Abstract
This study aimed to investigate the potential effect of Scutellaria barbata D. Don (SBD) on oxygen glucose deprivation/reperfusion (OGD/R)-injured PC12 cells. PC12 cells were pretreated with various concentrations of 0.1–0.8 mg/ml SBD for indicated times (12–48 h) and then subjected to OGD/R injury. Cell viability, apoptosis and proliferation were detected using MTT assay, flow cytometry, Ki67 staining and western blot. Oxidative damage was assessed by detecting MDA content, SOD activity and GSH levels. The mitochondrial membrane potential (Δψm) was measured by Rh123 staining. Western blot was performed to assess the expression levels of Nrf2 and PI3K/AKT pathway-related proteins. We found that SBD pretreatment promoted cell viability and proliferation but inhibited apoptosis of OGD/R-injured PC12 cells in dosage- and time-dependent manner. Meanwhile, SBD attenuated oxidative damage and restored mitochondria dysfunction, as evidenced by the reduced MDA content, the increased SOD and GSH levels, and the increased Δψm. Furthermore, SBD induced the expression of Nrf2 in a PI3K/AKT-dependent signalling. Knockdown of Nrf2 blocked the protective effects of SBD on PC12 cells. In conclusion, this study demonstrates that SBD pretreatment protects PC12 cells against OGD/R-induced injury. The potential mechanism may be through up-regulating the expression of Nrf2 in a PI3K/AKT-dependent pathway.
Introduction
Oxidative stress-induced neuronal damage is critical for the development of ischemic stroke [Citation1]. Ischemic stroke is a devastating disease and has been considered as the predominant cause of mortality and chronic disability worldwide [Citation2]. In clinic, patients with ischemic stroke are usually treated by thrombolysis or surgery in combination with special psychological and diet nursing. Due to the large amount of body oxygen, the relatively poor antioxidant defense system and the high concentration of readily pro-oxidant molecules, brain is deemed highly susceptible to oxidative stress [Citation3]. Therefore, protection of neurons against oxidative injury is considered to be a promising therapeutic strategy for ischemia stroke.
The transcription factor NF-E2-related factor 2 (Nrf2) is a member of the cap “n” collar (CNC) subfamily. Nrf2 has been well-known as the major mechanism of cellular defense against oxidative stress that regulates the expression of numerous reactive oxygen species (ROS), showing antioxidative activity [Citation4,Citation5]. When cells suffer from oxidative stress, Nrf2 is activated and further alters the expression of antioxidant response element (ARE)-dependent genes [Citation6,Citation7]. Previous studies have demonstrated that Nrf2 pathway could enhance cellular resistance to ischemic/reperfusion-induced oxidative stress through regulating the downstream antioxidant factors [Citation7,Citation8]. Nrf2 can be activated through several signalling pathways, such as the phosphatidylinositol 3-kinase (PI3K)/AKT pathway [Citation9].
Scutellaria barbata D. Don (SBD), also known as Ban-Zhi-Lian, is a perennial herb with multiple pharmacological effects, including anti-inflammation, anti-oxidation and anti-tumour activity [Citation10–12]. It has been demonstrated that SBD has a strong inhibitory effect on numerous human cancers, such as lung cancer, hepatocellular carcinoma and skin cancer [Citation13–15]. While the antioxidant effect of SBD has been widely accepted, its functional effects on neuronal oxidative injury have not been reported. In the present study, we aimed to explore the protective effects of SBD on PC12 cells against oxygen glucose deprivation/reperfusion (OGD/R)-induced injuries. Furthermore, this study analyzed which pathways can be modulated during OGD/R injury in PC12 cells. The findings of this study revealed a newly discovered function of SBD and suggested SBD as a potential agent for preventing and treating ischemic stroke.
Materials and methods
Cell culture and treatment
Rat adrenal pheochromocytoma PC12 cells were obtained from the Cell Bank of the Chinese Academy of Sciences (Beijing Gold Amethyst Pharm & Bio-Tech Co. Ltd, Beijing, China). Cells were maintained in Dulbecco’s modified Eagle’s medium (DMEM; Gibco, Carlsbad, CA, USA) supplemented with 10% fetal bovine serum (FBS; Sigma-Aldrich, St. Louis, MO, USA), 100 U/ml penicillin and 100 μg/ml streptomycin (Amresco, OH, USA). Cells were grown in 75 cm2 flask in a humidified atmosphere with 5% CO2 at 37 °C. The growth medium was changed every day.
SBD extracts were purchased from Shanghai Ha Ling Biological Technology (Shanghai, China). SBD was diluted in 50% dimethyl sulfoxide (DMSO; Sigma-Aldrich) to a stock concentration of 500 mg/ml and stored at −20 °C. To make a working solution, SBD stock solution was further diluted by the culture medium until the concentration of the vehicle was less than 0.1%. PC12 cells were pre-treated with various concentrations of SBD for 12–48 h prior to OGD/R. The same concentration of DMSO was used to treat cells as a blank control.
LY294002, an inhibitor of PI3K, was purchased from Calbiochem (La Jolla, CA). PC12 cells were pre-treated by 20 μM of LY294002 for 1 h [Citation16].
Procedure of OGD/R
The in vitro ischemia-reperfusion model was set up by OGD/R treatment of PC12 cells as described previously [Citation17]. In brief, PC12 cells were cultured in the DMEM without glucose and subsequently incubated in a humidified atmosphere of 5% CO2, 94% N2 and 1% O2 at 37 °C for 6 h. After the OGD treatment, PC12 cells were replaced with normal culture medium and returned to the normoxic incubator for a further 18 h.
si-RNA transfection
When PC12 cells were reached to 50% confluence in normal culture condition, cells were transfected with Nrf2 specific small interfering RNA (si-Nrf2) or scrambled random sequence negative control siRNA (si-NC) (Invitrogen, Carlsbad, CA, USA). Transfection was performed with the mediation of Lipofectamine 2000 reagent (Invitrogen). After transfection for 48 h, cells were collected for use in the following experiments.
Cell viability assay
Measurement of cell viability was evaluated using an MTT colourimetric assay. Briefly, PC12 cells were seeded in 96-well plates at a concentration of 1 × 104 cells/well and grown in DMEM for 24 h. After the treatment of SBD and OGD/R injury, cells were treated with 5 mg/ml MTT (Beyotime Biotechnology, Shanghai, China) for 3 h at 37 °C, followed by the addition of 150 μl of DMSO. The absorbance was measured at a detection wavelength of 570 nm, with a reference wavelength of 650 nm on a microplate reader (Bio-Rad Laboratories, Orlando, FL, USA).
Apoptosis assay
To measure apoptotic cells, the treated cells were collected and washed with cold phosphate buffer saline (PBS). Then, cells were resuspended in binding buffer and stained with 5 μl of Annexin V-FITC and 5 μl of PI (Beyotime Biotechnology) for 30 min at room temperature in the dark. Flow cytometry analysis was performed using a FACS can (Beckman Coulter, Fullerton, CA, USA), and the data were analyzed with FlowJo software (TreeStar, Ashland, OR, USA).
Ki67 positive cell detection by flow cytometry assay
Treated cells were fixed with 70% ethanol for 30 min and then incubated with 1% bovine serum albumin (BSA; Roche, Indianapolis, IN, USA) for another 30 min. Next, cells were incubated with anti-Ki67-antibodies (#11882; Cell Signaling Technology, Beverly, MA, USA) at a dilution of 1:50 for 60 min at 4 °C, followed by incubation with 1:1000-diluted Alexa Fluor 488–conjugated goat anti-Rabbit IgG (Invitrogen) for another 30 min. Flow cytometric analysis was performed using FACScan (Beckman Coulter) and the data were analyzed using FlowJo software (Tree Star).
Measurements of superoxide dismutase (SOD) activity, malondialdehyde (MDA) content and glutathion (GSH) level
After the treatment, PC12 cells were harvested and washed with cold PBS for three times. Then, cell lysis buffer was added to dissociate cells and the supernatant was separated and was used to determine SOD, MDA and GSH. Cellular MDA content, total SOD activity, and GSH levels were determined using commercial assay kits (Jiancheng Institute of Biotechnology, Nanjing, China) according to the specified manufacturer's instructions. The absorbance was read at 450 nm for SOD activity, 532 nm for MDA content, and 412 nm for GSH levels. The bicinchoninic acid disodium (BCA) protein assay kit (Beyotime Biotechnology) was used to quantify the concentration of protein.
Determination of mitochondrial membrane potential (δψm)
The Δψm was detected by a fluorescence probe Rh123 staining. Briefly, the treated cells were collected and rinsed with PBS. Next, cells were stained with 500 μl Rh123 (Beyotime Biotechnology) for 30 min in the dark. Then, cells were washed and resuspended in 500 μl PBS. The uptake of Rh123 was analyzed through a FACSCalibur flow cytometer (Beckman Coulter).
Western blot
The protein samples were extracted from cells using RIPA lysis buffer containing a protease inhibitor cocktail (Vazyme Biotech, Nanjing, China). The quantification of proteins was analyzed using BCA protein assay kit (Beyotime Biotechnology). Equal amounts of protein samples (30 μg) were separated and transferred into a polyvinylidene fluoride membrane (PVDF; Millipore, Bed-ford, MA, USA) using a Bio-Rad Bis-Tris Gel system according to the manufacturer’s instructions. Then, the membranes were blocked in 5% BSA for 2 h. Primary antibodies were prepared in 5% BSA at a dilution of 1:1000. The blocked membranes were incubated with corresponding antibody overnight at 4 °C, followed by the incubation with secondary antibody marked by horseradish peroxidase for 1 h at room temperature. The signals were captured and analyzed using Image Lab™ software (Bio-Rad). The primary antibodies against Bax (#4223), cleaved Caspase 3 (#9662), Cyclin D1 (#2978), Cyclin E (#20808), p-AKT (#4060), AKT (#4685), Nrf2 (#12721) and β-actin (#4970) were purchased from Cell Signaling Technology; p-PI3K (ab182651), PI3K (ab86714) from Abcam (Cambridge, MA) and Bid (sc-6538) from Santa Cruz Biotechnology (Santa Cruz, CA, USA).
Statistical analysis
All experiments were repeated three times. The results of multiple experiments are presented as the mean ± standard deviation (SD). Statistical analyses were performed using Graphpad Prism 6 software (GraphPad Software, San Diego, CA, USA). The p values were calculated using a one-way analysis of variance (ANOVA) or Student t-test. p value of < 0.05 was considered to indicate a statistically significant result.
Results
SBD promoted PC12 cells viability after OGD/R injury
To explore the effect of SBD on OGD/R injured PC12 cells, we firstly determined the cytotoxicity of SBD on untreated PC12 cells. As shown in , treatment of 0.1–0.8 mg/ml SBD for 24 and 48 h had no effects on the viability of PC12 cells. It suggested that SBD had no toxicity on PC12 cells. Then, the effects of SBD on OGD/R-injured PC12 cells were studied. As shown in , viability of PC12 cells was significantly reduced by OGD/R exposure (p < .01). However, pretreatment of SBD for 24 h dramatically increased cell viability in a dose-dependent manner (p < .01). Among these dosages, 0.4 and 0.8 mg/ml induced the highest viability of PC12 cells, thus 0.4 mg/ml was selected as an SBD-treating condition for use in the follow-up experiments. Results in revealed that cell viability was increased by pretreatment of 0.4 mg/ml SBD in a time-dependent manner (p < .05). These results suggested that SBD might have a protective effect on OGD/R-injured PC12 cells by promoting cell viability.
Figure 1. SBD promoted PC12 cells viability after OGD/R injury. (A) PC12 cells were incubated with different concentrations of SBD (0.1–0.8 mg/ml) for 24 h. Cell viability was measured using MTT assay. (B) PC12 cells were pretreated with different concentrations of SBD (0.1–0.8 mg/ml) for 24 h and then were subjected to OGD/R injury. Cell viability was detected using MTT assay. **p < .01 compared to Normal group. ##p < .01 compared to DMSO + OGD/R group. (C) PC12 cells were pretreated with 0.4 mg/ml SBD for different times (12, 24 and 48 h), followed by OGD/R injury. Cell viability was measured using MTT assay. NS: no significance; *p < .05 compared to 12 h. Data presented as mean ± SD (n = 3).
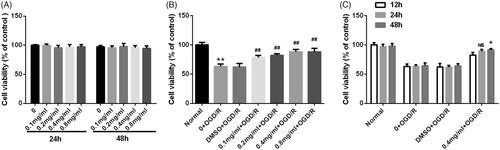
SBD inhibited PC12 cells apoptosis after OGD/R injury
We then investigated the effects of SBD on PC12 cells apoptosis triggered by OGD/R. Results in indicated that OGD/R induced a significant increase in apoptotic cell rate (p < .01). However, 24 h of SBD pretreatment significantly reduced apoptotic cell rate in a dosage-dependent manner (p < .01 or p < .001). showed that 0.4 mg/ml SBD pretreatment reduced apoptotic cell rate also via a time-dependent manner (p < .05 or p < .01), which suggested that the effect of SBD on PC12 cells was related with times of pretreatment.
Figure 2. SBD inhibited PC12 cells apoptosis after OGD/R injury. (A and B) PC12 cells were pretreated with different concentrations of SBD (0.1–0.8 mg/ml) for 24 h and then were subjected to OGD/R injury. Apoptotic cells were detected by flow cytometry. **p < .01 compared to Normal group. ##p < .01, ###p < .001 compared to DMSO + OGD/R group. (C and D) PC12 cells were pretreated with 0.4 mg/ml SBD for different times (12, 24 and 48 h), followed by OGD/R injury. Apoptotic cells were detected using flow cytometry. *p < .05, **p < .01 compared to 12 h. Data presented as mean ± SD (n = 3).
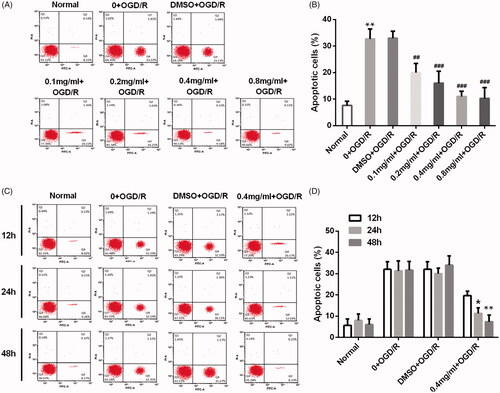
Similar results were observed in the protein expression of apoptosis-related factors. Results in showed that the expression levels of pro-apoptotic factors, including Bax, cleaved Caspase 3 and Bid, were increased by OGD/R injury in PC12 cells (p < .01). These increases induced by OGD/R were reversed by SBD pretreatment for 24 h. And, it seems that higher dosage of SBD resulted in lower protein levels. Also, showed that the expression levels of Bax, cleaved Caspase 3 and Bid were inhibited by 0.4 mg/ml SBD pretreatment in a time-dependent manner in OGD/R-injured PC12 cells. These results indicated that SBD protected PC12 cells against OGD/R injury through inhibiting cell apoptosis.
Figure 3. SBD altered the expression of pro-apoptotic proteins in PC12 cells after OGD/R injury. (A and B) PC12 cells were pretreated with different concentrations of SBD (0.1–0.8 mg/ml) for 24 h and then were subjected to OGD/R injury. Western blot was performed to assess the expression of pro-apoptotic factors. **p < .01 compared to Normal group. #p < .05, ##p < .01 compared to DMSO + OGD/R group. (C–F) PC12 cells were pretreated with 0.4 mg/ml SBD for different times (12, 24 and 48 h), followed by OGD/R injury. Western blot was performed to assess the expression of pro-apoptotic factors. **p < .01, ***p < .001 compared to 12 h. Data presented as mean ± SD (n = 3).
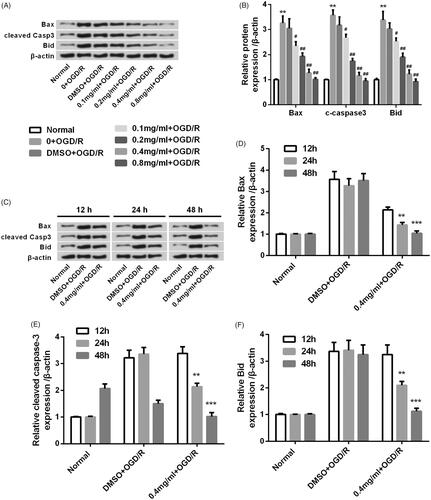
SBD promoted PC12 cells proliferation after OGD/R injury
We further determined the effects of SBD on the proliferation of OGD/R-injured PC12 cells. As shown in , OGD/R remarkably reduced Ki67 positive cell rate (p < .001). The reduction of Ki67 positive cell rate induced by OGD/R injury was significantly elevated by 24 h of SBD pretreatment with an increasing dosage from 0.1 mg/ml to 0.8 mg/ml (p < .05, p < .01 or p < .001). We also found that the effect of SBD on cell proliferation was correlated with incubated time of SBD. As shown in , the rate of Ki67 positive cells was significantly increased in OGD/R injured PC12 cells when pretreated with 0.4 mg/ml SBD in a time-dependent manner (p < .05).
Figure 4. SBD promoted PC12 cells proliferation after OGD/R injury. (A and B) PC12 cells were pretreated with different concentrations of SBD (0.1–0.8 mg/ml) for 24 h and then were subjected to OGD/R injury. Ki67 positive cells were determined by flow cytometry. ***p < .001 compared to Normal group. #p < .05, ##p < .01, ###p < .001 compared to DMSO + OGD/R group. (C and D) PC12 cells were pretreated with 0.4 mg/ml SBD for different times (12, 24 and 48 h), and then subjected to OGD/R injury. Flow cytometric analysis was used to measure the Ki67 positive cells. NS: no significance; *p < .05 compared to 12 h. Data presented as mean ± SD (n = 3).
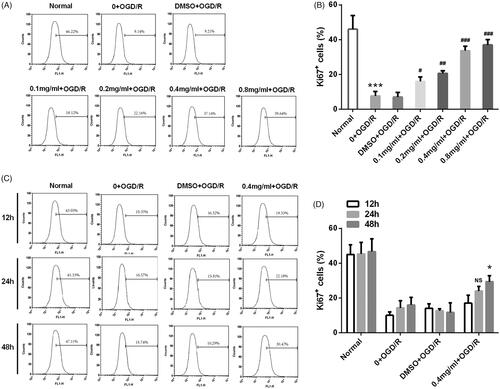
Western blot analysis revealed that the expression levels of proliferation-related proteins (Cyclin D1 and Cyclin E) were down-regulated in OGD/R-treated group (p < .01, ). However, the expression of Cyclin D1 and Cyclin E declined by OGD/R was reversed by 24 h SBD pretreatment in a dosage-dependent manner. Besides, the expression of Cyclin D1 and Cyclin E was increased by 0.4 mg/ml SBD pretreatment in OGD/R-injured PC12 cells in an increasing pretreated time (). These results suggested that SBD protected PC12 cells against OGD/R injury through promoting cell proliferation.
Figure 5. SBD altered the expression of proliferation-related proteins in PC12 cells after OGD/R injury. (A and B) PC12 cells were pretreated with different concentrations of SBD (0.1–0.8 mg/ml) for 24 h and then were subjected to OGD/R injury. The expression levels of proliferation-related proteins were assessed using western blot. **p < .01 compared to Normal group. NS: no significant; #p < .05; ##p < .01 compared to DMSO + OGD/R group. (C–E) PC12 cells were pretreated with 0.4 mg/ml SBD for different times (12, 24 and 48 h), and then subjected to OGD/R injury. Western blot was performed to assess the expression levels of proliferation-related proteins. NS: no significant; *p < .05; **p < .01 compared to 12 h. Data presented as mean ± SD (n = 3).
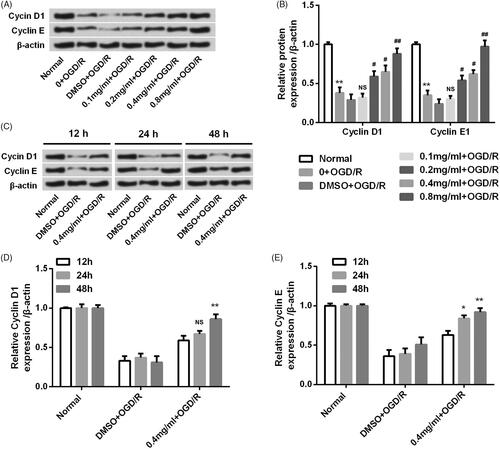
SBD attenuated oxidative damage and restored mitochondria dysfunction in PC12 cells after OGD/R injury
To further explore the antioxidant effects of SBD on PC12 cells, MDA content, SOD activity and GSH levels were measured. The concentration of MDA was significantly increased by OGD/R injury in PC12 cells (p < .001, ). However, with the pretreatment of SBD at a series of concentrations (0.1–0.8 mg/ ml), the contents of MDA was dose-dependently reduced (p < .05 or p < .01). Meanwhile, SBD pretreatment significantly enhanced the SOD levels which were reduced by OGD/R stimulation in PC12 cells with a dosage-dependent manner (p < .05 or p < .001, ). Similar results were observed in the GSH levels that SBD pretreatment dramatically increased the GSH levels in an increasing dosage (p < .01 or p < .001, ). Also, we found that the pretreatment of SBD significantly increased Δψm, which was collapsed by OGD/R injury (p < .01 or p < .001, ). Overall, these results suggested that SBD had an antioxidant effect and it could restore mitochondria dysfunction triggered by OGD/R in PC12 cells.
Figure 6. SBD attenuated oxidative damage and restored mitochondria dysfunction in PC12 cells after OGD/R injury. PC12 cells were pretreated with different concentrations of SBD (0.1–0.8 mg/ml) for 24 h, followed by OGD/R injury. (A) The MDA content, (B) SOD activity and (C) GSH levels were determined by special commercial assay kits. (D) The mitochondrial membrane potential (Δψm) was measured by Rh123 staining. **p < .01, ***p < .001 compared to Normal group. #p < .05, ##p < .01, ###p < .001 compared to DMSO + OGD/R group. Data presented as mean ± SD (n = 3).
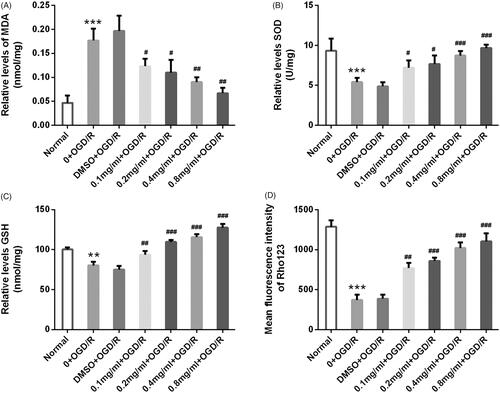
SBD promoted Nrf2 expression in PC12 cells
Nrf2, a CNC transcription factor, is a key transcription factor that regulates the expression of antioxidant genes in response to oxidative stress or enzyme inducers [Citation18]. Our findings showed that SBD had an antioxidant effect on PC12 cells, thus we further investigated the regulatory effects of SBD on Nrf2 expression. As shown in , the expression of Nrf2 in OGD/R-injured PC12 cells was up-regulated by 24 h of SBD pre-treatment with a dosage-dependent manner (p < .05, p < .01 or p < .001). Also, 0.4 mg/ml SBD increased Nrf2 expression in a time-dependent manner (p < .05 or p < .01, ). We further investigated whether Nrf2 acted as a downstream gene of SBD in OGD/R-injured PC12 cells. By performing siRNA transfection, the expression of Nrf2 in PC12 cells was knocked down successfully (p < .001, ). Then, we found that the effect of SBD on cell viability was abolished by si-Nrf2 transfection. As shown in , SBD pretreatment could not increase cell viability in si-Nrf2 transfected cells (p > .05). Meanwhile, the protein expression of Nrf2 was increased by SBD pretreatment in siNC transfected cells (p < .001, ). Not surprisingly, SBD pretreatment had no impacts on Nrf2 expression in si-Nrf2 transfected cells (p > .05). These results collectively suggested that SBD conferred its function possibly through regulating Nrf2.
Figure 7. SBD promoted Nrf2 expression in PC12 cells. (A) PC12 cells were pretreated with different concentrations of SBD (0.1–0.8 mg/ml) for 24 h and then were subjected to OGD/R injury. The expression of Nrf2 was measured using western blot. (B) PC12 cells were pretreated with 0.4 mg/ml SBD for different times (12, 24 and 48 h) and then were subjected to OGD/R injury. The expression of Nrf2 was measured using western blot. (C) PC12 cells were transfected with siNC or siNrf2 for 48 h. The transfected efficiency was verified using western blot. Then, transfected cells were pretreated with 0.4 mg/ml SBD for 24 h, followed by OGD/R injury. (D) Cell viability was measured using MTT assay. (E and F) The expression of Nrf2 was detected using western blot. NS: no significant; *p < .05; ***p < .001 compared to the corresponding control group. NS: no significant; #p < .05; ##p < .01; ###p < .001 compared to DMSO + OGD/R group. Data presented as mean ± SD (n = 3).
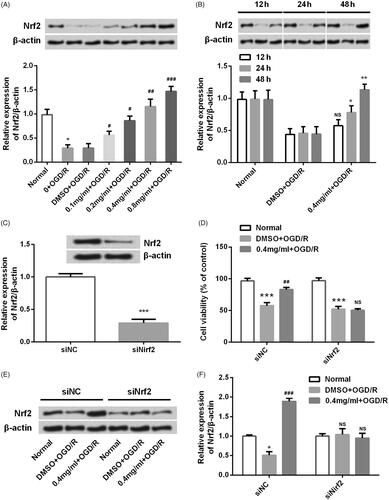
SBD regulated Nrf2 expression in PI3K/AKT-dependent signalling
To identify the potential upstream protein kinases involved in the activation of Nrf2 by SBD pretreatment, PI3K/AKT signalling pathway was investigated. As shown in , the phosphorylation levels of AKT and PI3K was increased by SBD pretreatment in both time- and dosage-dependent manner (p < .05, p < .01 or p < .001). Total levels of AKT and PI3K were unaffected. Thus, we hypothesized that the activation of PI3K/AKT might contribute to the regulatory effect of SBD on Nrf2 expression. To test this hypothesis, LP294002 was used to inhibit the activation of PI3K/AKT pathway. Interestingly, the elevated level of Nrf2 induced by SBD was inhibited in the presence of LY294002 (p < .05, ). Furthermore, we found that the promoting effect of SBD on cell viability was abolished by LY294002 treatment (p < .01, ). These results implied that SBD protected PC-12 cells against OGD/R via activating PI3K/AKT signalling and subsequently up-regulating Nrf2 expression.
Figure 8. SBD regulated Nrf2 expression in PI3K/AKT-dependent signalling. (A and B) PC12 cells were incubated with different concentrations of SBD (0.1–0.8 mg/ml) for 24 h and then were subjected to OGD/R injury. The expression of PI3K/AKT pathways related core factors was tested using western blot. (C and E) PC12 cells were pretreated with 0.4 mg/ml SBD for different times (12, 24 and 48 h) and then were subjected to OGD/R. Western blot was used to determine the expression of PI3K/AKT pathway-related factors. *p < .05, **p < .01, ***p < .001 compared to the corresponding controls. Then, PC12 cells were pretreated with 0.4 mg/ml SBD single or in combination with LY294002 and then were subjected to OGD/R injury. (E) The expression of Nrf2 and (F) cell viability was respectively measured using western blot and MTT assay. *p < .05, **p < .01, ***p < .001 compared to Normal group. ##p < .01 compared to DMSO + OGD/R group. &p < .05, &&p < .01 compared to 0.4 mg/ml + OGD/R group. Data presented as mean ± SD (n = 3).
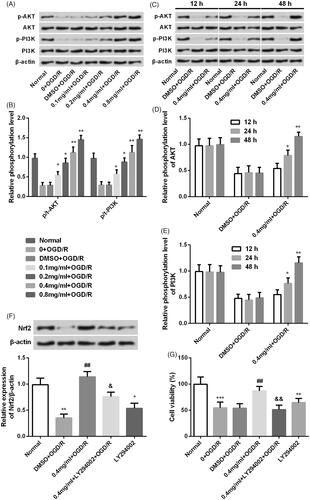
Discussion
Several lines of evidence have implicated that SBD exerts a strong activity of anti-tumour, anti-oxidative stress and anti-inflammation [Citation10–12]. Recent studies mainly focused on its anti-tumour effects which have been reported in many kinds of cancers [Citation13–15]. Besides, SBD had prominent antioxidant activity and free radical-scavenging property [Citation19]. Due to the role of oxidative stress and ROS in ischemic stroke, we hypothesized that SBD may also have an influence on OGD/R injured-PC12 cells. The present study showed that SBD pretreatment significantly enhanced cell viability, suppressed cell apoptosis and facilitated cell proliferation of PC12 cells suffering from OGD/R injury. Furthermore, SBD functioned to PC12 cells in dosage- and time-dependent manner. The regulatory effect of SBD on cell viability, apoptosis and proliferation might be based on its antioxidant activity.
We further investigated the effects of SBD on OGD/R-induced oxidative damage and mitochondria dysfunction. Oxidative damage was assessed by detecting the products of lipid peroxidation with the levels of MDA, enzymatic activity of SOD and content of GSH. MDA is a breakdown product of lipid peroxidases that is used as a biomarker to measure the level of oxidative damage of cell membranes. SOD and GSH are two important endogenous free radical scavengers, which catalyze the degradation of superoxide radical into oxygen and hydrogen peroxide and scavenge free radicals to protect cells from oxidative damage [Citation20,Citation21]. The increased activity of SOD and GSH enhances the ability to eliminate oxygen free radicals of cells. In our current study, the level of MDA was significantly increased in PC12 cells after OGD/R injury while SOD and GSH levels were dramatically reduced. However, different concentrations of SBD pretreatment reversed the effects of OGD/R, as evidenced by the reduced MDA levels, and the increased activity of SOD and GSH. These data confirmed the antioxidant effect of SBD on OGD/R injured PC12 cells. As a major production site of ROS, mitochondria are susceptible to oxidative damage and play a critical role in oxidative stress-induced cell apoptosis [Citation22]. Meanwhile, it has been reported that SBD had an effect on mitochondria function and regulated mitochondria-apoptosis pathway in several cancer cells [Citation23–25]. Thus, the present study investigated the effect of SBD on Δψm. We found that SBD pretreatment significantly inhibited the collapse of Δψm induced by OGD/R injury, which suggested that SBD had an effect on mitochondria function in PC12 cells. More detailed mechanisms need to be further explored.
Nrf2 is a pivotal regulator in antioxidative stress that can be activated by various phytochemicals or Chinese herbal medicine formula [Citation26,Citation27]. For example, Tao Hong Si Wu decoction (THSWD) and Z-ligustilide have been reported to activate PI3K/AKT and Nrf2/HO-1 signalling to protect against cerebral ischemia–reperfusion injury [Citation28,Citation29]. Our results showed that SBD could up-regulate the expression of Nrf2 in dosage- and time-dependent manner. Further, knockdown of Nrf2 abolished the protective effects of SBD on cell viability of ODG/R-injured PC12 cells. These results suggested that SBD conferred its protective function against ODG/R injury possibly via up-regulating Nrf2.
PI3K/AKT is a vital signalling pathway that promotes cell survival and cellular tolerance against multiple apoptotic insults [Citation30,Citation31]. PI3K/AKT pathway plays an important biological role in cell apoptosis, survival, proliferation, cytoskeleton change and other biological processes [Citation32]. It has been proved that the PI3K/AKT signalling pathway participates in the regulation of the pathological process of cerebral ischemia injury [Citation33–35]. In addition, several studies have reported that Nrf2 is a downstream regulator of PI3K/AKT pathway [Citation18, Citation36]. Thus, we further explored the effect of SBD pretreatment on the activation of PI3K/AKT pathway. We found that SBD pretreatment promoted the activation of PI3K/AKT pathway. Furthermore, the inhibitor of PI3K, LY294002, inhibited the expression of Nrf2 and blocked the function of SBD pretreatment on PC12 cells injured by OGD/R. These results implied that PI3K/AKT pathway was involved in the SBD elevated Nrf2 expression.
Conclusions
In conclusion, the present study indicated that SBD pretreatment had a protective effect on OGD/R-induced injury in PC12 cells. The neuroprotective effect of SBD may be partially through up-regulating the expression of Nrf2 in PI3K/AKT-dependent signalling. Our findings might provide a new insight into SBD’s effect on neural cells and a better understanding of the usage of SBD.
Disclosure statement
No potential conflict of interest was reported by the authors.
References
- Ni Z, Yao C, Zhu X, et al. Ailanthone inhibits non-small cell lung cancer cell growth through repressing DNA replication via downregulating RPA1. Br J Cancer. 2017;117:1621–1630.
- Yang P, Sun D, Jiang F. Ailanthone promotes human vestibular schwannoma cells apoptosis and autophagy by down-regulation of miR-21. Oncol Res. 2018;26:941–948.
- Chen Y, Zhu L, Yang X, et al. Ailanthone induces G2/M cell cycle arrest and apoptosis of SGC7901 human gastric cancer cells. Mol Med Rep. 2017;16:6821–6827.
- Peng S, Yi Z, Liu M. Ailanthone: a new potential drug for castration-resistant prostate cancer. Chinese J Cancer. 2017;36:25.
- Zhuo Z, Hu J, Yang X, et al. Ailanthone inhibits Huh7 cancer cell growth via cell cycle arrest and apoptosis in vitro and in vivo. Sci Rep. 2015;5:16185.
- Kato T, Suzumura Y, Fukushima M, et al. Antitumor activity of novel ailanthone derivatives in vitro and in vivo. Anticancer Res. 1988;8:573–579.
- Trino S, Lamorte D, Caivano A, et al. MicroRNAs as new biomarkers for diagnosis and prognosis, and as potential therapeutic targets in acute myeloid leukemia. Int J Mol Sci. 2018;19:E460.
- Wang XX, Zhang R, Li Y. Expression of the miR-148/152 family in acute myeloid leukemia and its clinical significance. Med Sci Monit. 2017;23:4768–4778.
- Elhamamsy AR, El Sharkawy MS, Zanaty AF, et al. Circulating miR-92a, miR-143 and miR-342 in plasma are novel potential biomarkers for acute myeloid leukemia. Int J Mol Cell Med. 2017;6:77–86.
- De Luca L, Trino S, Laurenzana I, et al. Knockdown of miR-128a induces Lin28a expression and reverts myeloid differentiation blockage in acute myeloid leukemia. Cell Death Dis. 2017;8:e2849.
- Chen J, Zhou J, Chen X, et al. miRNA-449a is downregulated in osteosarcoma and promotes cell apoptosis by targeting BCL2. Tumor Biol. 2015;36:8221–8229.
- Sandbothe M, Buurman R, Reich N, et al. The microRNA-449 family inhibits TGF-beta-mediated liver cancer cell migration by targeting SOX4. J Hepatol. 2017;66:1012–1021.
- Li Q, Peng J, Li X, et al. miR-449a targets Flot2 and inhibits gastric cancer invasion by inhibiting TGF-beta-mediated EMT. Diagn Pathol. 2015;10:202.
- Luo W, Huang B, Li Z, et al. MicroRNA-449a is downregulated in non-small cell lung cancer and inhibits migration and invasion by targeting c-Met. PLoS One. 2013;8:e64759.
- You J, Zhang Y, Li Y, et al. MiR-449a suppresses cell invasion by inhibiting MAP2K1 in non-small cell lung cancer. Am J Cancer Res. 2015;5:2730–2744.
- Zhi-Kun S, Hong-Qi Y, Zhi-Quan W, et al. Erythropoietin prevents PC12 cells from beta-amyloid-induced apoptosis via PI3KAkt pathway. Transl Neurodegener. 2012;1:7.
- Okunade AL, Bikoff RE, Casper SJ, et al. Antiplasmodial activity of extracts and quassinoids isolated from seedlings of Ailanthus altissima (Simaroubaceae). Phytother Res. 2003;17:675–677.
- Zheng Z, Zhao B. Astragalus polysaccharide protects hypoxia-induced injury by up-regulation of miR-138 in rat neural stem cells. Biomed Pharmacother. 2018;102:295–301.
- Nalla AK, Gorantla B, Gondi CS, et al. Targeting MMP-9, uPAR, and cathepsin B inhibits invasion, migration and activates apoptosis in prostate cancer cells. Cancer Gene Ther. 2010;17:599–613.
- Redondo-Munoz J, Ugarte-Berzal E, Garcia-Marco JA, et al. Alpha4beta1 integrin and 190-kDa CD44v constitute a cell surface docking complex for gelatinase B/MMP-9 in chronic leukemic but not in normal B cells. Blood. 2008;112:169–178.
- Lazarides E. Intermediate filaments: a chemically heterogeneous, developmentally regulated class of proteins. Annu Rev Biochem. 1982;51:219–250.
- Bouamrani A, Ramus C, Gay E, et al. Increased phosphorylation of vimentin in noninfiltrative meningiomas. PLoS One. 2010;5:e9238.
- Cripe LD. Adult acute leukemia. Curr Probl Cancer. 1997; 21:1–64.
- Whitman SP, Maharry K, Radmacher MD, et al. FLT3 internal tandem duplication associates with adverse outcome and gene- and microRNA-expression signatures in patients 60 years of age or older with primary cytogenetically normal acute myeloid leukemia: a Cancer and Leukemia Group B study. Blood. 2010;116:3622–3626.
- Gerloff D, Grundler R, Wurm AA, et al. NF-kappaB/STAT5/miR-155 network targets PU.1 in FLT3-ITD-driven acute myeloid leukemia. Leukemia. 2015;29:535–547.
- Zebisch A, Hatzl S, Pichler M, et al. Therapeutic resistance in acute myeloid leukemia: the role of non-coding RNAs. Int J Mol Sci. 2016;17:2080.
- Wu D, Liu J, Chen J, et al. MiR-449a suppresses tumor growth, migration and invasion in non-small cell lung cancer by targeting HMGB1-mediated NF-kappaB signaling way. Oncol Res. 2018;27:227–235.
- Chen J, Zhou J, Chen X, et al. miRNA-449a is downregulated in osteosarcoma and promotes cell apoptosis by targeting BCL2. Tumour Biol. 2015;36:8221–8229.
- Lobry C, Oh P, Mansour MR, et al. Notch signaling: switching an oncogene to a tumor suppressor. Blood. 2014;123:2451–2459.
- Xu X, Zhao Y, Xu M, et al. Activation of Notch signal pathway is associated with a poorer prognosis in acute myeloid leukemia. Med Oncol. 2011;28:483–489.
- Ono M, Takimoto R, Osuga T, et al. Targeting Notch-1 positive acute leukemia cells by novel fucose-bound liposomes carrying daunorubicin. Oncotarget. 2016;7:38586–38597.
- Xu Q, Simpson SE, Scialla TJ, et al. Survival of acute myeloid leukemia cells requires PI3 kinase activation. Blood. 2003;102:972–980.
- Jia HZ, Liu SL, Zou YF, et al. MicroRNA-223 is involved in the pathogenesis of atopic dermatitis by affecting histamine-N-methyltransferase. Cell Mol Biol (Noisy-le-Grand). 2018;64:103–107.
- Feng Z, Qi S, Zhang Y, et al. Ly6G + neutrophil-derived miR-223 inhibits the NLRP3 inflammasome in mitochondrial DAMP-induced acute lung injury. Cell Death Dis. 2017;8:e3170.
- Sharaf-Eldin WE, Kishk NA, Gad YZ, et al. Extracellular miR-145, miR-223 and miR-326 expression signature allow for differential diagnosis of immune-mediated neuroinflammatory diseases. J Neurol Sci. 2017;383:188–198.
- Martinez SR, Ma Q, Dasgupta C, et al. MicroRNA-210 suppresses glucocorticoid receptor expression in response to hypoxia in fetal rat cardiomyocytes. Oncotarget. 2017;8:80249–80264.