Abstract
Background
Angelica polysaccharide (AP) is disengaged from the roots of Angelica sinensis. The extensive pathological activities of AP have been discovered in disparate diseases. Nevertheless, the impression of AP in epilepsy (EP) remains unaware. The research attempted to probe the impact of AP on lipopolysaccharide (LPS)-evoked inflammatory injury in HT22 cells.
Methods
AP were exploited to stimulate HT22 cells, cell cytotoxicity was monitored by CCK-8 assay. LPS was utilized to administrate HT22 cells to evoke inflammatory injury, meanwhile the involvements of AP in cell proliferation, apoptosis and inflammatory cytokines productions were examined. MicroRNA-10a (miR-10a) inhibitor and its negative control were one by one transfected into HT22 cells, the effect of miR-10a inhibition on LPS- and AP-treated cells was determined. NF-κB and JAK2/STAT3 pathways were ultimately detected.
Results
AP promoted cell proliferation, inhibited apoptosis and suppressed IL-1β, TNF-α and IL-6 productions in LPS-stimulated HT22 cells. Additionally, AP raised miR-10a expression in HT22 cells administration with LPS. These functions of AP in LPS-disposed cells were conversed by miR-10a suppression. Further, AP interdicted NF-κB and JAK2/STAT3 pathways via enhancing miR-10a.
Conclusions
Data corroboarted that AP mitigated LPS-evoked inflammatory injury through repression of NF-κB and JAK2/STAT3 pathways by regulating miR-10a in HT22 cells.
Introduction
Epilepsy (EP) is a familiar nervous system disease, which is a manifestation of brain dysfunction characterized by recurrent seizures leading up to abnormal discharge of brain neurons [Citation1,Citation2]. It is reported that nearly 80% of EP cases take place in developing countries [Citation3]. In 2015, approximately 39 million people were diagnosed with EP, and 12,500 people were died due to EP. The incidence of EP is related to age and is more common in older children and young people [Citation4]. Current studies show that 70% of EP patients can be controlled by drugs, and the use of anti-epileptics has become the main methods for the treatment of EP [Citation5]. Although drug treatment has made great progress for EP, the side effects and adverse reactions of anti-epileptics are unavoidable [Citation6]. Considering about the limitation of the anti-epileptics, the effective and lower side effects drugs are still needed to be developed.
Mounting evidences have demonstrated that the traditional Chinese medicines (TCMs) contain plentiful anti-epileptic active ingredients [Citation7,Citation8]. Angelica sinensis is a widely used TCM, which has multiple functions, such as nourishing, blood generating and anti-inflammation [Citation9,Citation10]. Angelica Polysaccharide (AP) is the main water-soluble component of A. sinensis, which can obviously improve blood system, promote hematopoietic function, regulate immune system and exert anti-tumor effect [Citation11–13]. In vivo experiment study revealed that AP could inhibit inflammation and reduce the damage of endotoxin to mice [Citation14]. Additionally, the neuro-protective effect of AP has been reported in the existing researches. For example, Zhang et al. summed up the impacts of AP in nervous system and stated the important effect on nerve cells apoptosis [Citation15]. Further, study from Lin uncovered the protective effect of AP against cerebral ischemia-reperfusion (I/R)-evoked injury in hippocampal neuron of rats [Citation16]. However, the whether AP could protect hippocampal neuron cells against inflammatory injury in EP remains indistinct.
MicroRNAs (miRNAs) have been certified to show a crucial role in EP [Citation17]. MiR-10a is a member of miR-10 family, the importance has been testified in various ailments, such as acute myeloid leukaemia [Citation18], diverse cancers [Citation19,Citation20] and rheumatoid arthritis [Citation21]. More importantly, recent research corroborated that repression of miR-10a was discovered in lupus and inflammatory bowel diseases [Citation22,Citation23]. Moreover, a momentous research revealed that miR-10a mediated the production of various inflammatory cytokines, proliferation and migration in fibroblast-like synoviocytes [Citation24]. But, the functions of miR-10a in EP are still unclear. Therefore, we selected miR-10a as an object of this study to further exploration of the impacts of miR-10a on EP.
We previously utilized lipopolysaccharide (LPS) to induce hippocampal neuron HT22 cells injury, and the regulatory effect of AP on LPS-evoked HT22 cell inflammatory injury was ascertained. Exploration of NF-κB and JAK2/STAT3 pathways to ferret out the potential mechanisms. This study might clarify the protective effect of AP on LPS-evoked HT22 cells injury. The research might afford a neoteric sight for EP.
Materials and methods
Cell culture and treatment
Mouse hippocampal neuronal HT22 cells (5 × 106) obtained from American Type Culture Collection (ATCC, Rockville, MD, USA) were cultivated at 37 °C in a humidified incubator with 5% CO2 using DMEM (Gibco-BRL, Carlsbad, CA, USA) supplied with 10% heat-inactivated foetal bovine serum (FBS, Gibco-BRL) and 100 mg/mL penicillin/streptomycin double resistant (Gibco-BRL). The cells between passages 15 and 25 were disposed with 1 μg/mL LPS for 24 h to evoke inflammatory injury. AP (>95%, Sigma-Aldrich, St. Louis, MO, USA) was configured the different solutions for the concentration range from 0 to 100 μg/mL. HT22 cells were preconditioned with AP for 48 h before LPS administration.
Cell counting kit-8 (CCK-8) assay
Cells toxicity and viability of HT22 cells were checked according to the specifications of CCK-8 kit (Dojindo, Gaithersburg, MD). Briefly, HT22 cells with 5 × 103 cells/well were cultivated in 96-well plate, and were preconditioned with diverse concentrations of AP, and subsequently managed with LPS for 24 h. After administration, 10 μL of CCK-8 solution was put into the culture plate and was co-cultured with HT22 cells for 1 h in a CO2 incubator at 37 °C. The optical density at 450 nm wavelength (OD450) was executed by a Microplate Reader (Multiscan EX; Labsystems, Helsinki, Finland).
Apoptosis assay
Cell apoptosis was appraised through utilizing FITC/PI apoptosis kit (Solarbio, Beijing, China). HT22 cells were preconditioned with 80 μg/mL AP, and then disposed with 1 μg/mL LPS for 24 h. After poaching with PBS, HT22 cells were re-suspended in 1 × binging buffer. Cells suspension (100 μL) was transferred to a flow tube, simultaneously mixed 10 μL Annexin-V and 5 μL PI for 15 min dyeing at room temperature without light. Cell apoptosis was straightway determined by flow cytometry analysis (Beckman Coulter, Fullerton, CA, USA).
Enzyme-linked immunosorbent assay (ELISA)
HT22 cells were pre-treated with AP, and subsequently disposed with LPS, after 24 cultivation, the culture supernatants were gathered from different groups, and the productions of IL-1β, TNF-α and IL-6 were assessed by the corresponding ELISA kits (British Biotechnology Products, Abingdon, UK) based on the kits manufacturer.
Cell transfection
MiR-10a inhibitor (GenePharma Co (Shanghai, China) was transfected into HT22 cells according to the experience of liposome transfection. The negative control (NC) transfected into HT22 cells was regarded as a black control. The lipofectamine 3000 reagent (Invitrogen) and above plasmids were mixed and rest for 20 min at room temperature. HT22 cells were gathered after 48 h transfection and utilized for the following research.
Reverse transcriptase-quantitative polymerase chain reaction (RT-qPCR)
On the basis of Trizol Reagent (Invitrogen) specifications, total RNA was isolated from HT22 cells along with AP and LPS administration. Using 1 μg total RNA as template to prepare the reverse transcription system according to PrimeScript® RT reagent kit (Takara Bio, Inc., Tokyo, Japan) instructions. The cDNA product was utilized to configure RT-qPCR system referring to the specifications of SYBR green Master Mix accompanied by Exicycler 96 Real-Time Quantitative Thermal Block (Bioneer Corporation, Daejeon, South Korea) detection. U6 was regarded as the reference gene for miR-10a expression analysis, and β-actin served as the reference gene for determination of IL-1β, TNF-α and IL-6 mRNA expression. These data were gauged by 2−ΔΔCT method [Citation25].
Western blot assay
After processing with AP and LPS or transfection with miR-10a inhibitor, HT22 cells were splitted in RIPA lysis buffer (Beyotime). The content of protein was quantified by the common used BCA™ Protein Assay Kit (Pierce, Appleton, WI, USA). The total protein samples were resolved by SDS-polyacrylamide gel electrophoresis (SDS-PAGE). Afterward, these proteins were transferred to PVDF membranes (Millipore, Billerica, MA, USA). The membranes were sealed in 5% BSA as well as co-cultivated with the diluted primary antibodies at 4 °C overnight. The primary antibodies include p53 (ab131442), p21 (ab188224), poly ADP-ribose polymerase (PARP, ab32138), cleaved-PARP (ab32064), pro-caspase-3 (ab32150), cleaved-caspase-3 (ab13847), pro-caspase-9 (ab69514), cleaved-caspase-9 (ab2324), t-IκBα (ab178846), phosphorylated (p)-IκBα (ab133462), t-p65 (ab16502), p-p65 (ab76302), t-JAK2 (ab39636), p-JAK2 (ab195055), t-STAT3 (ab68153), p-STAT3 (ab76315) and β-actin (ab8227, Abcam, Cambridge, UK). Subsequently, the membranes were co-incubated with the second antibody (ab205718, Abcam) for 1 h, and the specific bindings were visualized using ECL reagent (GE Healthcare, Braunschweig, Germany). The protein bands and the gay values of the internal parameters were quantified by ImageJ software (Bio-Rad).
Statistical analysis
Based on the three independent experiments, all results were expressed by mean ± standard deviation (SD), and SPSS 19.0 statistical software (SPSS, Inc., Chicago, IL, USA) was implemented to analyze the results in disparate groups. The data were exploited by one-way or two-way ANOVA followed by Sidak’s or Tukey’s multiple comparisons test. A p values of <.05 was considered to represent a statistically significant result.
Results
AP alleviated LPS-diminished cell proliferation and LPS-induced apoptosis
To study the potential cytotoxicity of AP, we first examined the impact of AP on the viability of HT22 cells by CCK-8 assessment. Four different concentration gradients of AP (20, 40, 80 and 100 μg/mL) were utilized to dispose HT22 cells, and CCK-8 solution was then used to incubate HT22 cells for another 4 h at 37 °C. The results in revealed that AP at the concentrations of 20, 40 and 80 μg/mL made no difference in the viability of HT22 cells. Nevertheless, AP at 100 μg/mL repressed cell viability in HT22 cells (p < .05). According to the above results, we selected 80 μg/mL of AP for using the following experiments.
Figure 1. Impact of AP on cell proliferation and apoptosis in HT22 with LPS administration. (A) HT22 cells were disposed with the diverse concentrations of AP (0, 20, 40, 80 and 100 μg/mL), cell viability measured through CCK-8; HT22 cells were preconditioned with 80 μg/mL AP and then disposed with LPS (1 μg/mL), (B) cell viability and (C and D) p53 and p21 were assessed through CCK-8 and western blot; (E) cell apoptosis and (F) apoptosis-associated factors were determined through flow cytometry and western blot . *p < .05, **p < .01, ***p < .001 vs Control; #p < .05, ###p < .001 vs LPS group.
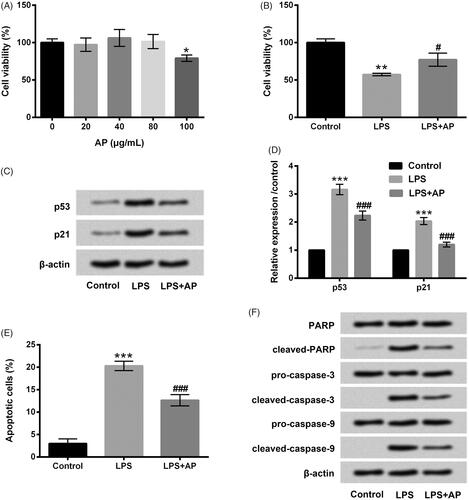
Impacts of AP on HT22 cell viability, apoptosis and their associated factors under inflammatory conditions induced by LPS were then examined. In , we observed that cell viability was markedly decreased (p < .01) in LPS-exposed HT22 cells, and enhancement of p53 and p21 were both observed in LPS-stimulated HT22 cells (p < .001). However, AP significantly increased cell viability (p < .05) concurrently down-regulated p53 and p21 levels in LPS-treated HT22 cells (p < .001). LPS administration memorably aggrandized the percentage of apoptotic cells as relative to control group (p < .001). But, AP treatment inversed the promoting effect of LPS on cell apoptosis (p < .001, ). Cleaved-PARP and cleaved-caspase-3/-9 were all aggrandized by LPS, whereas, AP decreased these factors protein levels in LPS-exposed HT22 cells (). Data from above-mentioned experiments indicated that AP alleviated LPS-diminished cell proliferation as well as weakened LPS-induced apoptosis in HT22 cells.
AP suppressed LPS-evoked inflammatory cytokines secretions in HT22 cells
HT22 cells were preconditioned with AP (80 μg/mL) and subsequently disposed LPS, IL-1β, TNF-α and IL-6 mRNA levels and concentrations were probed. In , IL-1β, TNF-α and IL-6 levels were significantly enhanced in HT22 cells following with LPS stimulation (p < .001). In HT22 cells pre-treated with AP, we observed the inhibition of IL-1β, TNF-α and IL-6 in these cells (p < .001). Similarly, AP signally repressed the productions of IL-1β, TNF-α and IL-6 in HT22 cells administrated with LPS (p < .001, ). Data from above experiments indicated that AP could inhibit LPS-evoked HT22 cells inflammatory injury.
Figure 2. Impact of AP on the productions of pro-inflammatory cytokines in HT22 cells with LPS administration. HT22 cells were preconditioned with 80 μg/mL AP prior to dispose with LPS (1 μg/mL), (A) the mRNA levels and (B) concentrations of IL-1β, TNF-α and IL-6 were tested through RT-qPCR and ELISA. ***p < .001 vs Control; ###p < .001 vs LPS group.
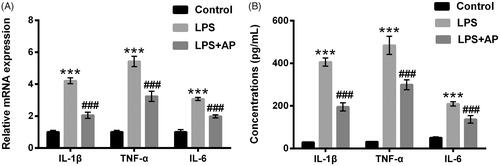
AP up-regulated miR-10a expression in HT22 cells with LPS stimulation
MiR-10a expression in LPS-treated or LPS together with AP-treated HT22 cells determined via RT-qRCR assessment. The reduction of miR-10a was discovered in HT22 accompanied by LPS stimulation as relative to control (p < .05, ). However, HT22 cells pre-treated with AP explained the enhancement of miR-10a as compared with LPS administration group (p < .001, ). In short, AP could up-regulate miR-10a expression in LPS-treated HT22 cells.
AP relieved LPS-evoked inflammatory injury through enhancement of miR-10a
MiR-10a inhibitor and its NC were respectively transfected into HT22 cells, and the transfection efficiency was ensured by RT-qPCR determination. Repression of miR-10a was observed after miR-10a inhibitor transfecting into HT22 cells (p < .001, ). After stimulation with LPS and AP, showed that the viability of HT22 cells was dramatically restrained by miR-10a inhibition (p < .001) in LPS and AP co-treated cells. Additionally, p53 and p21 expression were notably up-regulated by miR-10a inhibition in LPS and AP co-stimulated HT22 cells (p < .001, ). Induction of cell apoptosis was discovered in LPS and AP co-stimulated HT22 cells after miR-10a inhibitor transfection (p < .001, ). Beyond that, cleaved-PARP, cleaved-caspase-3 and cleaved-caspase-9 were all raised by miR-10a inhibition in LPS and AP co-administrated cells (). Furthermore, the enhancement of IL-1β, TNF-α and IL-6 were also observed in LPS and AP co-stimulated HT22 cells after transfection with miR-10a inhibitor (p < .001, ). Data indicated that miR-10a inhibition abolished the influence of AP in HT22 cells along with LPS stimulation.
Figure 4. Impact of miR-10a inhibition on cell proliferation and apoptosis in HT22 cells co-stimulated with LPS and AP. HT22 cells were respectively transfected with miR-10a inhibitor and NC, (A) miR-10a expression in these cells tested through RT-qPCR; these cells were preconditioned with 80 μg/mL AP prior to dispose with LPS (1 μg/mL), (B) cell viability (C and D) p53 and p21 were gauged through CCK-8 and western blot; (E) cell apoptosis and (F) apoptosis-associated factors were assessed through flow cytometry and western blot. ***p < .001 vs Control; ###p < .001 vs LPS group; &&&p < .001 vs LPS + AP + NC.
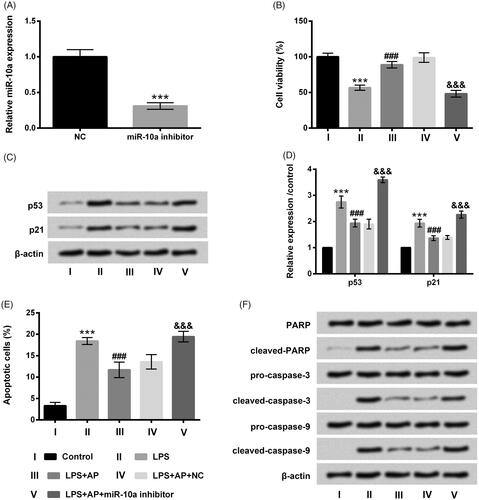
Figure 5. Impact of miR-10a inhibition on the productions of pro-inflammatory cytokines in HT22 cells co-stimulated with LPS and AP. HT22 cells were respectively transfected with miR-10a inhibitor and NC, and then were preconditioned with 80 μg/mL AP prior to dispose with LPS (1 μg/mL), (A) the mRNA levels and (B) the concentrations of IL-1β, TNF-α and IL-6 were assessed through RT-qPCR and ELISA. ***p < .001 vs Control; #p < .05, ###p < .001 vs LPS group; &&&p < .001 vs LPS + AP + NC.
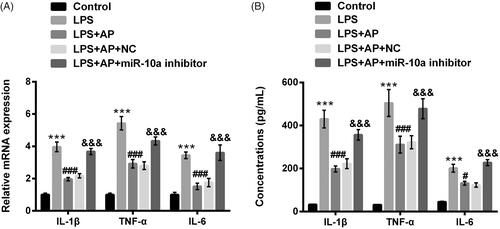
AP interdicted NF-κB and JAK2/STAT3 pathways through up-regulation of miR-10a
The key signalling pathways of NF-κB and JAK2/STAT3 were finally probed in HT22 cells. revealed that p-IκBα, p-p65, p-JAK2 and p-STAT3 were up-regulated (p < .001) in HT22 cells with LPS stimulation. After precondition with AP, the enhancement of p-IκBα, p-p65, p-JAK2 and p-STAT3 evoked by LPS were obviously decreased (p < .001). However, miR-10a suppression obviously up-regulated p-IκBα, p-p65, p-JAK2 and p-STAT3 levels in LPS and AP co-stimulated HT22 cells (p < .001). In the different treatment groups, t-IκBα, t-p65, t-JAK2 and t-STAT3 did not significantly changes. Collectively, AP interdicted NF-κB and JAK2/STAT3 through increasing of miR-10a expression.
Figure 6. Impact of AP and miR-10a on NF-κB and JAK2/STAT3 pathways in HT22 cells with LPS treatment. HT22 cells were respectively transfected with miR-10a inhibitor and NC, and then were preconditioned with 80 μg/mL prior to dispose with LPS (1 μg/mL), (A) NF-κB pathway associated factors and (B) JAK2/STAT3 pathway associated factors examined through western blot. ***p < .001 vs Control; ###p < .001 vs LPS group; &&&p < .001 vs LPS + AP + NC.
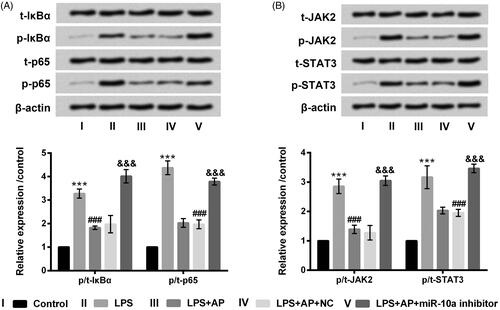
Discussion
The study corroborated that AP promoted cell proliferation and repressed apoptosis and suppressed IL-1β, TNF-α and IL-6 productions, indicating the protective effect of AP on LPS-injured HT22 cells. Interestingly, the enhancement of miR-10a was found in AP and LPS co-stimulated HT22 cells. The protective effect of AP on LPS-evoked inflammatory injury in HT22 cells was inversed after transfection with miR-10a inhibitor. Besides, AP obviously interdicted NF-κB and JAK2/STAT3 by enhancement of miR-10a.
AP is a main components of A. sinensis, which is purified from the fresh root of A. sinensis [Citation26]. The activities of AP, including antitumor, antioxidant, anticoagulant, anti-radiation, and immunomodulation have been widely reported in the existing researches [Citation27,Citation28]. Recently, several studies reported the important regulatory effect on inflammatory injury diseases. Wang et al. corroborated that AP attenuated concanavalin A-evoked liver injury in mice [Citation29]. Lei et al. displayed that AP could weaken oxidative stress-evoked neuronal cells injury [Citation30]. Nonetheless, the involvement of AP in LPS-evoked inflammatory injury in HT22 cells remains unclear. Our research discovered that AP facilitated cell proliferation, reduced apoptosis, meanwhile decreased pro-inflammatory cytokines productions in LPS-treated HT22 cells. These findings testified that AP could lighten LPS-evoked inflammatory injury in HT22 cells, suggesting the neuro-protective effect of AP on EP.
There is an emerging view that various miRNAs are implicated in mediating the pathogenesis of EP and the maintenance of EP conditions [Citation31]. In an acute seizure mouse model, Kretschmann et al. found that 146 miRNAs were up-regulated in this model [Citation32]. Functional studies have confirmed that miR-34a and miR-132 could affect seizure-evoked neuronal death, and suppression of miR-134 convictively suppressed status epilepticus and seizure damage [Citation33,Citation34]. MiR-10a is attached to the miR-10 family, and is located between HOXB4 and HOXB5 genes on the short arm of chromosome 17 [Citation35]. The abnormal expression of miR-10a has been discovered in manifold cancers, which shows a momentous role in the occurrence and development of these cancers [Citation20,Citation36,Citation37]. Our study tried to explore whether miR-10a affected the effect of AP on HT22 cells after LPS administration. Important results revealed the enhancement of miR-10a in AP and LPS co-stimulated HT22 cells, and the impact of AP on LPS-evoked HT22 cells inflammatory injury was reduced after miR-10a inhibitor transfection. Data in our research implicated the participation of miR-10a in the process of AP protecting HT22 cells resist LPS-evoked inflammatory injury.
To further confirm the underlying regulatory mechanism, two key signaling pathways of NF-κB and JAK2/STAT3 are examined. NF-κB is a momentous transcription factor, which is involved in multiple physiological processes including neurological diseases [Citation38,Citation39]. Zhou et al. reported that increased the activation of NF-κB in seizure rat hippocampus could promote the development of hippocampus injury [Citation40]. JAK2/STAT3 signalling pathway has also been reported to play a central role in different biological processes of multitudinous diseases [Citation41,Citation42]. Jia et al. revealed that Propofol postconditioning attenuated hippocampus I/R injury through modulating JAK2/STAT3 signaling pathway [Citation43]. Furthermore, Zeng et al. certified the protective effect of MC13 on neurons from microglia-mediated neuro-inflammatory injury through interdicting JAK2/STAT3 signaling pathway [Citation44]. Our research discovered that AP obviously interdicted NF-κB and JAK2/STAT3 pathways, and the inhibitory effect of AP on NF-κB and JAK2/STAT3 might via promotion of miR-10a. These observations indicated that AP weakened LPS-evoked HT22 cells inflammatory injury might through adjusting NF-κB and JAK2/STAT3 pathways.
Taken together, the study discovered that AP weakened LPS-evoked inflammatory injury, and its function might through interdicting NF-κB and JAK2/STAT3 pathways by enhancement of miR-10a. The discoveries offered a novel strategy for the clinical remedy of EP. More detailed researches merited further confirm to uncover the impact of AP on EP.
Disclosure statement
No potential conflict of interest was reported by the authors.
References
- Musteata M, Mocanu D, Stanciu GD, et al. Interictal cardiac autonomic nervous system disturbances in dogs with idiopathic epilepsy. Vet J. 2017;228:41–45.
- Dubey D, Singh J, Britton JW, et al. Predictive models in the diagnosis and treatment of autoimmune epilepsy. Epilepsia. 2017;58:1181–1189.
- Schmitz B. Effects of antiepileptic drugs on mood and behavior. Epilepsia. 2006;47:28–33.
- Naghavi M, Wang H, Lozano R, et al. Global, regional, and national age–sex specific all-cause and cause-specific mortality for 240 causes of death, 1990–2013: a systematic analysis for the Global Burden of Disease Study 2013. Springer; 2015.
- Pilotto C, Liu JF, Walker DA, et al. Seizure characteristics and the use of anti-epileptic drugs in children and young people with brain tumours and epileptic seizures: analysis of regional paediatric cancer service population. Seizure. 2018;58:17–21.
- Perucca P, Gilliam FG. Adverse effects of antiepileptic drugs. Lancet Neurol. 2012;11:792–802.
- Chen LC, Chou MH, Lin MF, et al. Lack of pharmacokinetic interaction between valproic acid and a traditional Chinese medicine, Paeoniae Radix, in healthy volunteers. J Clin Pharm Ther. 2000;25:453–459.
- Tian L, Wang Z, Wu H, et al. Evaluation of the anti-neuraminidase activity of the traditional Chinese medicines and determination of the anti-influenza A virus effects of the neuraminidase inhibitory TCMs in vitro and in vivo. J Ethnopharmacol. 2011;137:534–542.
- Hua Y, Xue W, Zhang M, et al. Metabonomics study on the hepatoprotective effect of polysaccharides from different preparations of Angelica sinensis. J Ethnopharmacol. 2014;151:1090–1099.
- Zhang WQ, Hua YL, Zhang M, et al. Metabonomic analysis of the anti-inflammatory effects of volatile oils of Angelica sinensis on rat model of acute inflammation. Biomed Chromatogr. 2015;29:902–910.
- Zhuang C, Xu NW, Gao GM, et al. Polysaccharide from Angelica sinensis protects chondrocytes from H2O2-induced apoptosis through its antioxidant effects in vitro. Int J Biol Macromol. 2016;87:322–328.
- Qin T, Chen J, Wang D, et al. Optimization of selenylation conditions for Chinese angelica polysaccharide based on immune-enhancing activity. Carbohydr Polym. 2013;92:645–650.
- Cao W, Li XQ, Wang X, et al. Characterizations and anti-tumor activities of three acidic polysaccharides from Angelica sinensis (Oliv.) Diels. Int J Biol Macromol. 2010;46:115–122.
- Zhuang H, Zhou ZY, Song CJ. Angelica sinensis polysaccharides promotes apoptosis in human breast cancer cells via CREB-regulated caspase-3 activation. Biochem Biophys Res Commun. 2015;467:562–569.
- Zhang HH, Wang S, Wang J, et al. Antioxidative and neuroprotective effects of Angelica sinensis polysaccharide. Acta Neuropharmacologica. 2014;4:39–45.
- Lin GF. Protective effects of angelica polysaccharide on hippocampal neuron of rats with cerebral ischemia-reperfusion injury. Chinese Trad Herbal Drugs. 2015;46:558–561.
- Jimenez-Mateos EM, Henshall DC. Epilepsy and microRNA. Neuroscience. 2013;238:218–229.
- Bryant A, Palma CA, Jayaswal V, et al. miR-10a is aberrantly overexpressed in Nucleophosmin1 mutated acute myeloid leukaemia and its suppression induces cell death. Mol Cancer. 2012;11:8.
- Wang Y, Liu Z, Yao B, et al. Long non-coding RNA TUSC7 acts a molecular sponge for miR-10a and suppresses EMT in hepatocellular carcinoma. Tumor Biol. 2016;37:11429–11441.
- Weiss FU, Marques IJ, Woltering JM, et al. Retinoic acid receptor antagonists inhibit miR-10a expression and block metastatic behavior of pancreatic cancer. Gastroenterology. 2009;137:2136–2145.e1.
- Hussain N, Zhu W, Jiang C, et al. Down-regulation of miR-10a-5p in synoviocytes contributes to TBX5-controlled joint inflammation. J Cell Mol Med. 2018;22:241–250.
- Xue X, Feng T, Yao S, et al. Microbiota downregulates dendritic cell expression of miR-10a, which targets IL-12/IL-23p40. J Immunol. 2011;187:5879–5886.
- Wu W, He C, Liu C, et al. miR-10a inhibits dendritic cell activation and Th1/Th17 cell immune responses in IBD. Gut. 2015;64:1755–1764.
- Mu N, Gu J, Huang T, et al. A novel NF-kappaB/YY1/microRNA-10a regulatory circuit in fibroblast-like synoviocytes regulates inflammation in rheumatoid arthritis. Sci Rep. 2016;6:20059.
- Livak KJ, Schmittgen TD. Analysis of relative gene expression data using real-time quantitative PCR and the 2(-Delta Delta C(T)) method. Methods. 2001;25:402–408.
- Wang KP, Zeng F, Liu JY, et al. Inhibitory effect of polysaccharides isolated from Angelica sinensis on hepcidin expression. J Ethnopharmacol. 2011;134:944–948.
- Zhao Y, Shi Y, Yang H, et al. Extraction of Angelica sinensis polysaccharides using ultrasound-assisted way and its bioactivity. Int J Biol Macromol. 2016;88:44–50.
- Cao P, Sun J, Sullivan MA, et al. Angelica sinensis polysaccharide protects against acetaminophen-induced acute liver injury and cell death by suppressing oxidative stress and hepatic apoptosis in vivo and in vitro. Int J Biol Macromol. 2018;111:1133–1139.
- Wang K, Song Z, Wang H, et al. Angelica sinensis polysaccharide attenuates concanavalin A-induced liver injury in mice. Int Immunopharmacol. 2016;31:140–148.
- Lei T, Li H, Fang Z, et al. Polysaccharides from Angelica sinensis alleviate neuronal cell injury caused by oxidative stress. Neural Regen Res. 2014;9:260–267.
- Song YJ, Tian XB, Zhang S, et al. Temporal lobe epilepsy induces differential expression of hippocampal miRNAs including let-7e and miR-23a/b. Brain Res. 2011;1387:134–140.
- Kretschmann A, Danis B, Andonovic L, et al. Different microRNA profiles in chronic epilepsy versus acute seizure mouse models. J Mol Neurosci. 2015;55:466–479.
- Reschke CR, Henshall DC. microRNA and Epilepsy. Vol. 888. Springer International Publishing; 2015. ((eds) ISG, editor.).
- Wang XM, Jia RH, Wei D, et al. MiR-134 blockade prevents status epilepticus like-activity and is neuroprotective in cultured hippocampal neurons. Neurosci Lett. 2014;572:20–25.
- Woltering JM, Durston AJ. MiR-10 represses HoxB1a and HoxB3a in zebrafish. PLoS One. 2008;3:e1396.
- Hoppe R, Achinger-Kawecka J, Winter S, et al. Increased expression of miR-126 and miR-10a predict prolonged relapse-free time of primary oestrogen receptor-positive breast cancer following tamoxifen treatment. Eur J Cancer. 2013;49:3598–3608.
- Hudson J, Duncavage E, Tamburrino A, et al. Overexpression of miR-10a and miR-375 and downregulation of YAP1 in medullary thyroid carcinoma. Exp Mol Pathol. 2013;95:62–67.
- Teng W, Wang L, Xue W, et al. Activation of TLR4-mediated NFkappaB signaling in hemorrhagic brain in rats. Mediators Inflamm. 2009;2009:473276.
- Kaltschmidt B, Kaltschmidt C. NF-kappaB in the nervous system. Cold Spring Harb Perspect Biol. 2009;1:a001271
- Zhou Q, Li GQ. Role of TLR4/NF-κB signal pathway in the hippocampus injury of status epileptic rats. Chinese J Neurol. 2008;41:689–694.
- Li L, Li H, Li M. Curcumin protects against cerebral ischemia-reperfusion injury by activating JAK2/STAT3 signaling pathway in rats. Int J Clin Exp Med. 2015;8:14985–14991.
- Minli LI, Zhu R, Zhang X, et al. The role of JAK2/STAT3 signaling pathway in the lung injury rat with severe acute pancreatitis. Med J Chinese Peoples Liberation Army. 2011;36:611–613.
- Jia L, Wang F, Gu X, et al. Propofol postconditioning attenuates hippocampus ischemia-reperfusion injury via modulating JAK2/STAT3 pathway in rats after autogenous orthotropic liver transplantation. Brain Res. 2017;1657:202–207.
- Zeng KW, Yu Q, Liao LX, et al. Anti-neuroinflammatory effect of MC13, a novel coumarin compound from condiment murraya, through inhibiting lipopolysaccharide-induced TRAF6-TAK1-NF-kappaB, P38/ERK MAPKS and Jak2-Stat1/Stat3 pathways. J Cell Biochem. 2015;116:1286–1299.