Abstract
Colon cancer is one of the most malignant cancers. Histone modification is closely related to tumour development. Our study explored the functions of anti-silencing function 1A (ASF1A) on H4Y72ph in colon cancer cells. Colon cancer cell lines and clinical specimens were obtained and/or transfected with full length ASF1A or interference mRNA to mimic or silence of ASF1A expression. Immunoprecipitation and GST pull down was used to target targeting ASF1A or H4Y72ph. Cells were transfected with H4WT- or H4Y72F-expressing. An in vitro kinase activity assay was set to determine whether ASF1A could phosphorylate H4. The severity of autophagy was measured by detecting number of autophagosomes, number of EGFP-LC3, LC3-II/I, percentage of degradation and expression of autophagy associated gene (ATG). ASF1A positively regulated H4Y72ph; Immunoprecipitation assay and GST pull down results showed that ASF1A interacted directly with H4. In addition, ASF1A silence inhibited autophagosomes number, EGFP-LC3 number, LC3-II/I, percentage of degradation and ATG expression. Moreover, H4Y72F impaired the promoting autophagy effects of ASF1A. The ASF1A-H4Y72ph axis promoted colon cancer autophagy via transcriptional regulation of ATG genes. ASF1A regulated H4Y72ph and promotes autophagy in colon cancer cells via a kinase activity through regulation of ATG.
Introduction
Colon cancer is one of the most catastrophic cancers with high incidence and mortality [Citation1]. Previous data revealed that there were more than one million population with colon cancer and around 700,000 patients subjected to colon-related death each year [Citation2]. This serious situation motivates scientists to inspect the reason behind and to seek for the possible effective treatments. Recent years, because the crucial roles of epigenetic modification, the relationship between epigenetic modifications and colon cancer starts to seize attention.
Epigenetic modifications recently received considerable attention all due to its important potential roles in human diseases [Citation3]. The main epigenetic modifications include DNA methylation, histone modifications and nucleosome positioning [Citation4]. Among these three categories, DNA methylation is the most well studied, and nowadays more and more experiments set to explore histone modification because of its deregulation is closely associated with cancer development [Citation5]. Histone modification is characterized by histone phosphorylation, acetylation and methylation [Citation6]. In addition, the core histones group into histone H2A, H2B, H3 and H4, which are the main component for histone octamers wrapping eukaryotic DNA [Citation7]. Hence, histone modification participates almost of the DNA-templated progression, such as replication, transcription and repair [Citation6]. Importantly, dysregulation of histone modification is subjected to a wide spectrum of tumour development [Citation8], such as prostate cancer [Citation9], gastric cancer [Citation10] and also include colon cancer [Citation11]. In our study, we investigated the role of one specific histone modification which was phosphorylation of tyrosine on histone H4 at residue H4Y72 (H4Y72ph) in colon cancer cell lines and clinical specimens.
Anti-silencing function 1A (ASF1A) is a key histone-remodelling chaperone which involved in diverse biological progression in cells [Citation12]. For example, ASF1A induced lineage-specific genes expression via modulating bivalent chromatin domains during mouse embryonic stem cell differentiation [Citation13]. Importantly, ASF1A was observed to have functions in multiple cancers. For example, previous literature found that ASF1A was involved in gastric cancer and colorectal cancer by regulation of genetics and epigenetics [Citation14]. In addition, a previous study has reported that ASF1A was associated with chromatin higher-order organization and was required for appropriate chromatin assembly [Citation15]. Particularly, ASF1A contributes to tumourigenesis [Citation16], but the functions of ASF1A in colon cancer cells have not been clearly illustrated.
Considering that autophagy is closely related to colon cancer [Citation17], therefore, in our study, experiments were designed to specifically determine whether ASF1A had effects on the autophagy of colon cancer cells and the underlying mechanisms. Our study might provide a new insight into the treatment of colon cancer.
Material and method
All experiments were performed in Jining No. 1 People’s Hospital or its cooperated laboratory.
Cell culture
Human colon cell line LoVo, SW48 and SNU-C1 cells were purchased from Shanghai Institute for Biological Science (Shanghai, China). Cells were maintained at 37 °C under 5% CO2 in Roswell Park Memorial Institute (RPMI)-1640 (Gibco Laboratories, Grand Island, NY) adding 100 units/ml penicillin, 100 μg/ml streptomycin and 10% foetal bovine serum (FBS, Life Science, Salt Lake City, UT).
Clinical specimens
In the time of clinical specimen, colon tissues were collected for complete clinicopathological characteristics information study. From the June 2009 to June 2010, our study collected information from 130 patients from Jining No. 1 People’s Hospital. No patients received any chemotherapy or radiation therapy. Informed consents from every individual patient were collected, and the Medical Ethics Committee of Jining No. 1 People’s Hospital was approved in this study. Our intraoperative diagnosis was according to the World Health Organization grading system and strictly followed Edmondson–Steiner grading standard to assess tumour histologic differentiation degree [Citation18]. All pathological specimens were detected by two or three pathologists independently.
Plasmids construction
Plasmids (Clontech Inc, Palo Alto, CA) expressing hexa-histidine (His6)-tagged (Invitrogen, Carlsbad, CA) full-length wild-type ASF1A was obtained and transfected into colon cell lines SW48 and SNU-C1 to mimic the expression of ASF1A. Cells transfected with pcDNA3.1 was treated as control. H4−/− SNU-C1 cells were transfected with H4WT- or H4Y72F-expressing plasmids, in which H4 was tagged with human influenza hemagglutinin (HA). H4WT- or H4Y72F-expressing plasmids were obtained by QuickChange Site-Directed Mutagenesis Kit (Thermo Fisher Scientific, Rockford, IL). Specific interference RNA (ASF1A siRNA-1/-2) which can silence ASF1A was purchased from GenePharma (Shanghai, China). The transfection approach were performed using Lipofectamine® 3000 (Invitrogen, Carlsbad, CA) according to the manufacture’s instruction.
Co-immunoprecipitation analysis
Co-immunoprecipitation analysis was performed as what the previously described [Citation1]. Cells were collected and washed using cold-PBS, then resuspended with CHIP buffer (Cell Signaling Technology, Beverly, MA). Then the liquid were pulse sonicated (BRANSON Digital Sonifier, Branson Ultrasonics Co, Danbury, CT) for 10 times with 5 s each. Collect the cell lysates and culture with mouse IgG or primary antibodies (Abcam, Cambridge, UK) for around 12 h overnight at 4 °C. Afterwards, the cell lysates were added with Protein A/G agarose beads (50 μl) and maintained for extending 3 h. Using CHIP buffer to wash the beads, and then centrifuge to collect the beads. Last, boil cell lysates to release the precipitated proteins. The final results were detected by western blot.
Immunohistochemistry (IHC)
IHC was performed as what described in the report [Citation19]. In brief, 5 µm sized paraffin embedded tissue sections were de-paraffinized with xylene. Then endogenous peroxidase activity was determined with 3% H2O2 in methanol for 30 min in the room temperature in the dark. Afterward, slides were washed by Tris-buffered saline (TBS) supplemented with 0.025% Triton X-100 for 2–3 min and blocked in 5% bovine serum albumin (BSA) for 1 h. Then slides were incubated with primary antibodies (Abcam, Cambridge, MA) and maintained for 1 h in room temperature. Then slides were incubated with secondary antibodies (Abcam, Cambridge, MA) for 1 h after 20 min maintained in Reaction Enhancer. In the end, all the samples were stained using 3,3′-diaminobenzide tetrahydrochloride) and haematoxylin. The final results were calculated by testing the intensity and extent of immunopositivity. All the chemical material aforementioned except antibodies for IHC was purchased from Sigma-Aldrich (St. Louis, MO).
Long-lived protein degradation during autophagy
Colon cells autophagy was induced by starvation. Cells were cultured in completed or starved Earle's Balanced Salt Solution (EBSS) medium for 5 h. Briefly, cell cultured for 1 h first, then the medium was changed into fresh medium and continued to culture for another 4 h. The culture was ended by cooling in ice. Trichloroacetic acid (TCA, 10% v/v) was utilized for precipitation assay after centrifugation at 12,000 rpm 10 min at room temperature. Long-lived protein degradation was detected via measuring the ration of the acid-soluble radioactivity by liquid scintillation counting.
Glutathione S-transferase (GST) pull-down
In brief, colon cancer cells were resuspended in binding buffer with bacterial lysate (phosphate buffer saline (PBS) + 1% Triton-100 + phenylmethylsulphonyl fluoride (PMSF)) (Beyotime, Shanghai, China). Then lysate were sonicated in cooling condition by ice until the lysate cool down. Then glutathione–Sepharose (GS)-4B beads (Amersham Biosciences, Uppsala, Sweden) were added to the tube and centrifuged at 4 °C at 2000 rpm. Afterwards, using cold binding buffer (PBS + 1% Triton-100) to wash the tube in order to remove the ethanol in the tube, then the beads need resuspended in cold PBS to generate 50% slurry. After centrifuged for 1 h, the beads were washed and resuspended in binding buffer, and then reacted with in vitro-expressed H4 protein for 2 h at 4 °C. Then, 0.5% Tween-20 buffer was used to wash the beads two times, and the binding proteins were analyzed by western blot.
Mononucleosome purification
Mononucleosome were obtained by digestion of the appropriate labelled nucleosome array with PSTI. Mononucleosomes were obtained by digestion of the appropriate labelled nucleosomal arrays with PstI. As described in the report from Jaskelioff et al. [Citation20], mononucleosomes which containing H4 were purified by 5–30% glycerol gradient centrifugation at 30,000 × g for 18 h at 4 °C.
In vitro kinase assay
Kinase assay was used to detect the activity of ASF1A in vitro. Method was referred to reports from Yang et al. [Citation21] that using 250 ng of the indicated recombinant protein(s) in 30 μL of kinase buffer. After maintained for 30 min at 30 °C, kinase reactions were quenched by the addition of 5× Laemmli buffer and by boiling the samples for 5 min. All the chemical materials aforementioned were purchased from Sigma-Aldrich (St Louis, MO).
Purification of recombinant proteins from Escherichia coli
ASF1A or H4 constructs were transfected into pET28 vectors and then transferred into E. coli expression strain BL21-(DE3) competent cells (Novagen, Madison, WI). We selected the positive strains using ampicillin added in LB medium to culture E. coli. 24 h later, chose three bacterial colonies and then resuspended and incubated overnight in shaking incubated bed. Cultured cells were treated with isopropyl β-d-1-thiogalactoside (IPTG, 0.8 mM) and then added 25 ml of lysis buffer and maintained for 15 min at 4 °C. The protein were collected and analyzed by western blot.
Western blot
Protein was obtained from LoVo, SW48 and SNU-C1 cells by RIPA lysis buffer (Cat. No. R0010, Solarbio, Beijing, China) and protease inhibitors (Thermo Fisher Scientific, Rockford, IL). The protein concentration was determined by BCA™ Protein Assay Kit (Pierce, Appleton, WI). Bio-Rad Bis-Tris Gel system was established for western blot system. Primary antibodies were prepared in 5% blocking buffer and diluted strictly following product instruction. These primary antibodies were incubated in membrane and maintained at 4 °C overnight. Then for secondary antibody incubation, incubate with HRP. Signals were collected and analyzed the intensity of the bandsby Image Lab™ Software (Bio-Rad, Shanghai, China).
Statistical analysis
Results are shown as mean + standard deviation (SD). Graphpad 6.0 statistical software (GraphPad, San Diego, CA) was used for statistical analyses. A one-way analysis of variance (ANOVA) and Student’s t-test was applied for p values calculated. p < .05 means significant difference.
Results
ASF1A positively regulated H4Y72ph in colon cancer cells
Histone Chaperone ASF1A has been reported to have correlation with different cancer developments [Citation14,Citation22]. Therefore, at the beginning of our work, data from the clinicopathological practice were collected to check whether ASF1A expression was involved in the progression of colon cancer. From the result of , it is obviously demonstrated that ASF1A expression was connected with histological grade, age, lymph node (LN) metastasis, tumour size and advanced tumour node metastasis (TNM) stage (p < .01, ), which indicated that ASF1A expression might play a vital role in the occurrence and development of colon cancer.
Table 1 Correlation of ASF1A and clinicopathological parameters in colon cancer
Furthermore, we evaluated the samples from the same patient to check the level of phosphorylation at H4Y72 by the other team members in our researching group. Surprisingly, combining the results from the two groups, we found that there was a closely and positively relationship between the level of H4Y72ph and ASF1A in the colon cancer (). Moreover, it is easier to conclude from the WB result in that the accumulated levels of ASF1A revealed the same trend along with the expression level of H4Y72ph in different colon cancer cell lines LoVo, SW48 and SNU-C1. Further experiments were performed to confirm this result. As shown in , transfection with full length wild-type ASF1A-expressing plasmid showing high expression level of ASF1A, which also coupled with the increasing accumulated level of H4Y72ph in different colon cancer cell lines SW48 (both p < .01) and SNU-C1 (p < .01 or p < .001). On the opposite, using siRNA to downregulate the expression of ASF1A, H4Y72ph levels were also markedly inhibited in in both SW48 and SNU-C1 cell lines (). These results demonstrated that the relationship between ASF1A and H4Y72ph are positively correlated, which means that ASF1A can enhance the expression of H4Y72ph in colon cancer cell lines SW48 and SNU-C1.
Figure 1. ASF1A expression is positively correlated with H4Y72ph levels in colon cancer cells. (A) Expression of H4Y72ph and ASF1A in different cell lines (LoVo, SW48 and SNU-C1) were detected by western blot. (B and C) Effects of ASF1A overexpression in regulation of H4Y72ph were detected by western blot. (D) Using interference RNA to silence ASF1A expression and detected the influence on H4Y72ph in different cell lines (SW48 and SNU-C1) by western blot. All the data were presented by mean + SD (n = 3). ** p < .01; *** p < .001 (to control in H4Y72ph/H4); ## p < .01 (to control in ASF1A/GAPDH).
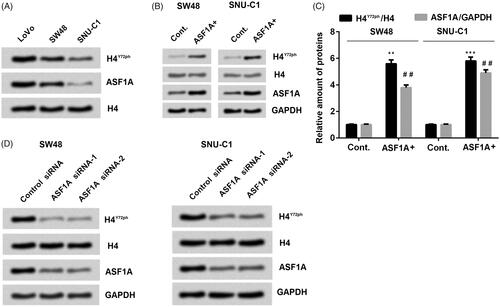
Table 2. Relationships between ASF1A expression and H4Y72ph in specimens of colon cancer.
ASF1A interacted directly with H4 in colon cancer cells
In order to reveal the underlying mechanism how ASF1A enhanced the phosphorylation of H4Y72, further experiments were performed to investigate whether ASF1A could interact with histone H4. Co-immunoprecipitation assay was used to evaluate the relationship of ASF1A and H4 in SW48 cells, and then the result was detected by western blot. Result in showed that there was an association between ASF1A and H4. Furthermore, we explored whether there was connection between the H4 and H4Y72ph, which might be access of ASF1A to H4Y72ph. Hence, we constructed special plasmids which were HA (human influenza haemagglutinin)-tagged WT and Y72F-mutant (a mutation that mimics dephosphorylation) H4-expressing and transfected into H4-/- SNU-C1 cells.
Figure 2. ASF1A has intrinsic tyrosine kinase activity. (A) H4 or ASF1A was analyzed by western blot using immunoprecipitation with antibodies targeting ASF1A or H4 in SW48 cells. (B) ASF1A and H4 expression was detected by western blot using immunoprecipitation with an anti-HA antibody. (C) H4 was detected using GST pull-down assay with tag in ASF1A full length. (D) An in vitro kinase activity assay was used to detect whether recombinant full-length ASF1A could phosphorylate H4. (E) Samples from the in vitro kinase activity assay were analyzed by western blot WT. wild-type; Y72F, dephosphorylated mutant. All experiments depends on replicates (n = 3).
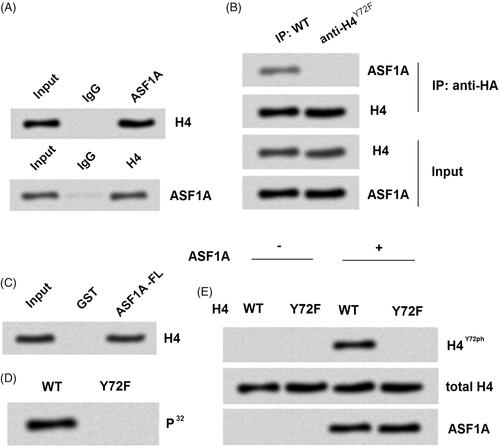
First, H4 and its corresponding proteins were precipitated by Co-immunoprecipitation assay using an antibody against the HA tag (). Western blot result demonstrated that the accumulated level of ASF1A bound to H4Y72F was obviously decreased compared to H4WT, which suggested that ASF1A could not connected with H4 in the Y72F-mutant group. The result stand in the same line with the association between ASF1A and H4 showed in .
To explore the deeper and detailed connection between ASF1A and H4, a GST pull-down assay was used with GST-tagged full-length ASF1A that were expressed in E. coli. In the GST tagged ASF1A full length group, H4 was strongly expressed, which indicated that ASF1A could directly bind to H4 (). Taken together, the data indicate that H4Y72ph may be directly regulated by ASF1A in colon cancer cells.
Based on the results above, we started to consider which is the exactly point for ASF1A worked on H4. We inferred that histone chaperone ASF1A might have kinase activity and through this point to phosphorylated H4Y72. Our next experiments were performed to determine this possible explanation. Isotope labelling P32 was used as the phosphate donor to check whether ASF1A could phosphorylate H4 between WT and Y72F mutant group. Results showed that H4 phosphorylated type only shown in the WT but not in the Y72F mutant group (). This finding demonstrated that the phosphorylated target point for the ASF1A on H4 was in Y72. Further experiment in showed that ASF1A administration could not phosphorylated H4Y72 if the Y72 mutant into Y72F, which confirmed that the target for ASF1A on H4 phosphorylation was in Y72. Taken together, all these findings suggested us that ASF1A could phosphorylate H4 in the target of Y72.
Silence of ASF1A inhibited autophagy in Colon cancer cells
Autophagy progression and situation in tumour cells could be used as a marker of the progression of tumour cancer [Citation23]. Therefore, we curious to determine whether ASF1A play a role in regulation of autophagy in colon cancer cells in vitro. Silence of ASF1A was achieved by using interference siRNA to downregulate ASF1A. Then we used starvation to induce autophagy in colon cancer cells. Surprisingly, we found that starved treatment significantly induced autophagy in the WT (p < .001, ). Meanwhile, we found that silence of ASF1A significantly decreased the number of autophagosomes in the starved groups (p < .001, ). LC-I and LC-II are two important markers for autophagy. Then we detected the effects of ASF1A on the expression of LC-I and LC-II. Results showed that silence of ASF1A both decreased LC-II expression (). EGFP-LC3 is the marker of the autophagosomes [Citation24]. Hence, we explored the reason how silence of ASF1A decreased cell autophagy in colon cancer cells. Results showed that an increased accumulation of EGFP-LC3 puncta was found in WT cells after the autophagy inhibitor chloroquine (CQ) administration (p < .01) but not in ASF1A−/− cells (p < 0.01) as relative to WT, suggesting that the silence of ASF1A inhibits autophagosome formation (). Then we detected the long-lived protein degradation ability and found that silence of autophagy is the main reason for the degradation of most long-lived proteins [Citation25]. ASF1A significantly increased protein degradation per cent (p < .01, ), which indicated that autophagy related protein degradation was suppressed by absence of ASF1A. Moreover, to determine the how the interference RNA work on the ASF1A to influence autophagy process. Interestingly, we found that after we added siRNAs, then the target gene of ATG5, ATG13 and ATG 14 was downregulated, as well as the decreased LC3-II (). These findings suggested us that silence of ASF1A was through silence of target gene of ATG5, ATG13 and ATG 14. In a word, silence of ASF1A decreased colon cell autophagy.
Figure 3. ASF1A is an essential factor that regulates autophagy in colon cancer cells. (A) In SW48 cells and (B) in SNU-C1 cells, effects of ASF1A silence on the number of autophagosome per field under complete or starved condition. (C) Effects of ASF1A on the expression of LC3-I and LC3-II under complete or starved condition. (D) Effects of ASF1A silence and chloroquine (CQ) on the number of enhanced green fluorescent protein (EGFP)-tagged LC3 under complete or starved condition. (E) Effects of WT and ASF1A silence on the degradation percentage. (F) SW48 cells harbouring doxycycline (Dox)-inducible siRNA targeting autophagy-associated gene (ATG) were transiently transfected with ASF1A-expressing plasmids under complete or starved condition. The results are shown as the mean + SD (n = 3). * p < .05, ** p < .01; *** p < .001.
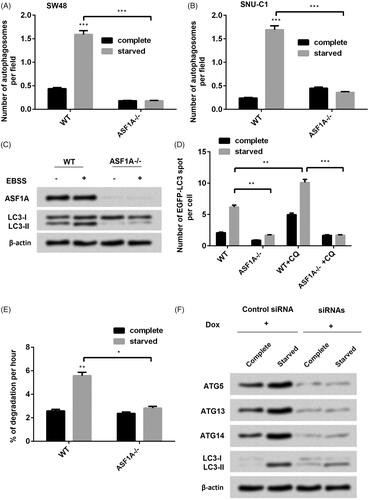
H4Y72ph was required for ASF1A-promoted autophagy in starvation-treated Colon cancer cells
We already known that ASF1A was bound with H4, then next we explored how H4 was joined the promoting autophagy of ASF1A on colon cancer cell line SW48. H4-/-, ASF1A+/+SW48 cells () were transfected with plasmids with empty vector, H4WT and H4Y72F (Y72 mutation) which can expression EGFP-LC3. Results showed that only transfection with H4WT increased the accumulation of EGFP-LC3 puncta but not in the H4Y72F, which suggested that H4Y72Fph exert crucial role in promoting cell autophagy. Further experiments were performed to investigate whether silence of ASF1A could decrease H4Y72Fph and the expression of light chain 3 (LC3)-II compared with the WT group. On the other hand, ASF1A silence inhibited the increasing trend of H4Y72Fph which was shown in WT in a time-dependent manner (). With co-treated with ASF1A and transfection with WT, accumulation level of H4Y72ph was upregulated as accompanied with increasing level of EGFP-LC3 in the starved group (p < .01, ) compare with transfection with empty vector. On the opposite, added with ASF1A and transfection with H4Y72F was no significant difference with the group transfected with empty vector, which suggested that ASF1A promoted autophagy through phosphorylation of H4Y72.
Figure 4. ASF1A-mediated H4Y72ph engages in autophagy in colon cancer cells. (A) Effects of H4WT and H4Y72F on the number of EGFP-LC3 puncta under complete or starved condition. (B) WT and ASF1A−/− SW48 cells were treated with complete medium or Earle's balanced salt solution (EBSS) for 30 min or 1 h, and whole cell protein samples were then obtained for western blot. (C) Total H4, H4Y72ph and ASF1A expression was detected by western blot. (D) Effects of H4WT and H4Y72F mutation on the number of EGFP-LC3 puncta were detected. The results are shown as the mean + SD (n = 3). * p < .05, ** p < .01.
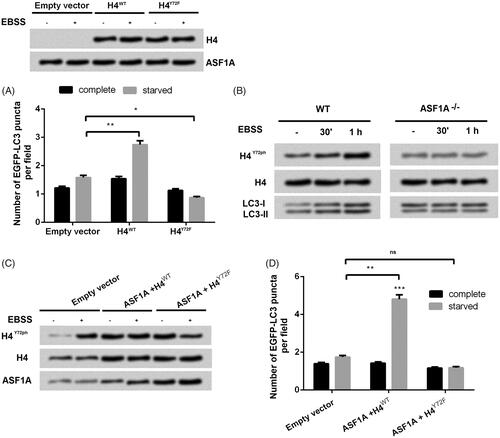
The ASF1A-H4Y72ph axis promoted colon cancer autophagy via transcriptional regulation of ATG genes
In order to determine how the ASF1A-H4Y72ph axis promoted autophagy in colon cancer cell line SW48, we co-transfected with ASF1A−/− with H4WT or H4Y72A or H4Y72E (both revealed overexpression of H4Y72ph). Result showed that in the staved condition, ATG5 and ATG7 expression was significantly upregulated as compared to the normal medium culture group (both p < .01, ). On the other side, interestingly, silence of ASF1A broke the significant difference between complete culture and stared culture. Similar results were shown in the western blot in . These findings indicated that ASF1A-H4Y72ph axis upregulates ATG mRNA and protein expression under starvation conditions. Then plasmids which containing the promotor of ATG5 and ATG7 were transfected into SW58 cells. Using the luciferase report, we found that ASF1A with the H4WT group which contains ASF1A-H4Y72ph axis has the positive effect on the on the transcriptional activity of ATG5 and ATG7 in SW48 cells under starvation conditions, and this effect was inhibited by H4Y72F (). Western blot results showed that starvation induced ATG5 and ATG7 expression in the H4WT group associated with upregulation of LC3-II (). These findings hinted that ASF1A-H4Y72ph axis worked in autophagy progress through targeting ATG5 and ATG7 in colon cancer cell line SW48.
Figure 5. ASF1A-H4Y72ph axis promotes colon cancer autophagy via transcriptional regulation of autophagy associated genes (ATG). The mRNA expression levels of (A) ATG5 and (B) ATG7 under ASF1A silence with H4WT or H4Y72A or H4Y72E. (B) The protein expression level of ATG5 and ATG7 were detected by western blot. (D) The relative light of (D) ATG5 and (E) ATG7 under co-treated ASF1A and H4WT and H4Y72F. (F) Protein expression level of ATG5 and ATG7 under co-treated ASF1A and H4WT and H4Y72F. The results are shown as the mean + SD (n = 3). ** p < .01 and *** p < .001.
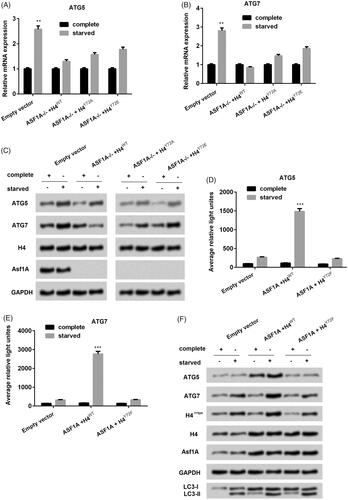
Discussion
Colon cancer ranks into the third and fourth in all the cancer-related diseases in incurrence and mortality, respectively [Citation26]. Nowadays, different explains and theories were shown to demonstrate the possible underlying mechanisms. For example, some related factors, such as vascular endothelial growth factor (VEGF), might directly mediate colon cancer growth via a non-angiogenic mechanism [Citation27]; some miRNAs might involve in colon cancer cell growth and metastasis [Citation26]. Recent years, epigenetic modification enriched major advances in our understanding of colon cancer [Citation28]. In our study, we investigated the effects of histone chaperone ASF1A in colon cancer cells via regulation of H4Y72.
Increasing evidence revealed that histone H3–H4 chaperone ASF1A played vital roles in regulating chromatin assembling and gene transcription. One report showed that the higher the ASF1A expressed in cells, the lower outcome the patients have, which suggested that ASF1A was a biological marker for the gastrointestinal cancer [Citation22]. On the other side, H4 modification exert fundamental functions in modulating damaged chromatin and create open chromatin structures, even though DNA repair machinery and chromatin remodelling complexes [Citation29]. Previous study identified a tyrosine phosphorylation site at Y72 of histone H4, which could promote DNA synthesis and repair [Citation30]. Combining that ASF1A is the H4 chaperone and H4 is so important in gene regulation, we inferred that ASF1A has effects via phosphorylation of H4Y72.
Interestingly, we found that in the three different colon cancer cell lines LoVo, SW48 and SNU-C1, the expression of H4Y72ph and ASF1A was always in the similar trend, which indicated that there might be an association between H4Y72ph and ASF1A. In order to confirm this hypothesis, further experiments were performed to investigate the exactly relationship between ASF1A and H4Y72ph and by which ASF1A regulates H4Y72ph in vivo. Surprisingly, immunoprecipitate results showed that the proteins from SW48 cells H4Y72ph may be directly regulated by ASF1A in colon cancer cells. A study from J et al. found that ASF1A really connected with ASF1A in regulating chromatin assembly [Citation31]. Our study undergone further to indicate that ASF1A directly connected with H4Y72ph in colon cancer cells, which lifted the veil of roles of ASF1A in colon cancer cells. In addition, we tried to detect the results in clinical specimens and found that the colon cancer cell lines and clinical specimens demonstrate the similar results about the relationship between ASF1A and H4Y72ph.
Next, we detected the roles of ASF1A in the autophagy of colon cancer cells. Autophagy is a process in which cellular material transferred into lysosomes for degradation and amount of studies illustrated that autophagy plays extremely important roles in cancer cells [Citation32]. In addition, LC3-I and LC3-II are two important marker factors in autophagy and autophagy promoted the conversion of LC3-I to LC3-II [Citation33]. Transfection with EGFP-LC-3, the phagosome-specific marker, could trace the change of LC-3, by which to determine the situation of autophagy [Citation34]. Hence, the autophagosomes numbers, the per cent of degradation and the number of EGFP-LC-3 could reveal the severity of autophagy to some extent. Results showed that silence of ASF1A decreased the number of autophagosomes, the expression of LC3-II, number of EGFP-LC-3 in colon cancer cells. In addition, a previous study showed that 18 autophagy-associated gene (ATG) translated proteins are necessary in autophagosome formation [Citation35]. In our study, we chose three ATG proteins ATG5, ATG13 and ATG14 and check their expression under the expression of silence of ASF1A and results showed that they all downregulated. All these findings indicated silence of ASF1A inhibited cell autophagy while ASF1A might promote cell autophagy.
For we already known that there was a strong relationship between ASF1A and H4Y72ph; therefore, we detected the roles of H4Y72ph in autophagy in colon cancer cells. Results showed that co-treated ASF1A with mutation of H4Y72F, the promoting autophagy effects of ASF1A was inhibited. These results demonstrated that H4Y72ph was required for ASF1A-promoted autophagy in starvation-treated colon cancer cells, meaning that the ASF1A-H4Y72ph axis promoted cell autophagy.
Then we intended to explore by which ASF1A-H4Y72ph axis promoted colon cancer cell autophagy. As we aforementioned that ATG family members were the key important proteins in autophagosome formation, therefore, we inferred that there was possibility that ASF1A-H4Y72ph axis worked through regulation of ATG proteins. In one literature reported about the autophagy-related histone modification, ASF1A, and ATG5 and ATG7 came to light [Citation36]. In our study, we found that the ASF1A and H4WT group increased the expression of ATG5 and ATG7 while mutation of H4Y72 point impaired this trend. These findings suggested that The ASF1A-H4Y72ph axis promotes colon cancer autophagy via transcriptional regulation of ATG genes.
Conclusions
In a word, our study revealed strongly relationship between ASF1A and H4Y72ph. Then we also investigated the role of ASF1A-H4Y72ph axis in regulating of colon cancer cell autophagy and found that ASF1A-H4Y72ph axis promoted colon cancer autophagy via transcriptional regulation of ATG5 and ATG7. Furthermore, our study introduced the clinical specimens to enrich our data, therefore, our study might provide a fundamental and novel insight in understanding the underlying mechanism and also helped to enrich the potential treatment for colon cancer in the future.
Disclosure statement
No potential conflict of interest was reported by the authors.
References
- Tamas K, Walenkamp AM, de Vries EG, et al. Rectal and colon cancer: not just a different anatomic site. Cancer Treatment Rev. 2015;41:671–679.
- Landreau P, Drouillard A, Launoy G, et al. Incidence and survival in late liver metastases of colorectal cancer. J Gastroenterol Hepatol. 2015;30:82–85.
- Portela A, Esteller M. Epigenetic modifications and human disease. Nat Biotechnol. 2010;28:1057–1068.
- Balakrishnan L, Milavetz B. Epigenetic regulation of viral biological processes. Viruses. 2017;9:346.
- Audia JE, Campbell RM. Histone modifications and cancer. Cold Spring Harb Perspect Biol. 2016;8:a019521.
- Tessarz P, Kouzarides T. Histone core modifications regulating nucleosome structure and dynamics. Nat Rev Mol Cell Biol. 2014;15:703–708.
- Lennartsson A, Ekwall K. Histone modification patterns and epigenetic codes. Biochim Biophys Acta. 2009;1790:863–868.
- Hattori N, Ushijima T. Compendium of aberrant DNA methylation and histone modifications in cancer. Biochem Biophys Res Commun. 2014;455:3–9.
- Dryhurst D, Ausio J. Histone H2A.Z deregulation in prostate cancer. Cause or effect? Cancer Metastasis Rev. 2014;33:429–439.
- Yang WY, Gu JL, Zhen TM. Recent advances of histone modification in gastric cancer. J Can Res Ther. 2014;10:240–245.
- Deb M, Sengupta D, Kar S, et al. Epigenetic drift towards histone modifications regulates CAV1 gene expression in colon cancer. Gene 2016;581:75–84.
- Gonzalez-Munoz E, Arboleda-Estudillo Y, Otu HH, et al. Cell reprogramming. Histone chaperone ASF1A is required for maintenance of pluripotency and cellular reprogramming. Science (New York, NY). 2014;345:822–825.
- Gao Y, Gan H, Lou Z, et al. Asf1a resolves bivalent chromatin domains for the induction of lineage-specific genes during mouse embryonic stem cell differentiation. Proc Natl Acad Sci USA. 2018;115:E6162–e6171.
- Henrique R. ASF1A in gastric and colorectal cancer: on the hinge between genetics and epigenetics? EBioMedicine. 2017; 21:45–46.
- Wang C, Chang JF, Yan H, et al. A conserved RAD6-MDM2 ubiquitin ligase machinery targets histone chaperone ASF1A in tumorigenesis. Oncotarget. 2015;6:29599–29613.
- Yang S, Liu L, Cao C, et al. USP52 acts as a deubiquitinase and promotes histone chaperone ASF1A stabilization. Nature Commun. 2018;9:1285.
- Wen J, Zhao Y, Guo L. Orexin A induces autophagy in HCT-116 human colon cancer cells through the ERK signaling pathway. Int J Mol Med. 2016;37:126–132.
- Asare EA, Compton CC, Hanna NN, et al. The impact of stage, grade, and mucinous histology on the efficacy of systemic chemotherapy in adenocarcinomas of the appendix: analysis of the National Cancer Data Base. Cancer. 2016; 122:213–221.
- Varghese F, Bukhari AB, Malhotra R, et al. IHC Profiler: an open source plugin for the quantitative evaluation and automated scoring of immunohistochemistry images of human tissue samples. PloS One. 2014;9:e96801.
- Jaskelioff M, Gavin IM, Peterson CL, et al. SWI-SNF-mediated nucleosome remodeling: role of histone octamer mobility in the persistence of the remodeled state. Mol Cell Biol. 2000;20:3058–3068.
- Yang JH, Song TY, Jo C, et al. Differential regulation of the histone chaperone HIRA during muscle cell differentiation by a phosphorylation switch. Exp Mol Med. 2016;48:e252.
- Liang X, Yuan X, Yu J, et al. Histone chaperone ASF1A predicts poor outcomes for patients with gastrointestinal cancer and drives cancer progression by stimulating transcription of beta-catenin target genes. EBioMedicine. 2017;21:104–116.
- Lu W, Ke H, Qianshan D, et al. Apatinib has anti-tumor effects and induces autophagy in colon cancer cells. Iran J Basic Med Sci. 2017;20:990–995.
- Drake KR, Kang M, Kenworthy AK. Nucleocytoplasmic distribution and dynamics of the autophagosome marker EGFP-LC3. PloS One. 2010;5:e9806.
- Yoshii SR, Mizushima N. Monitoring and measuring autophagy. Int J Mol Sci. 2017;18: E1865.
- Zeng M, Zhu L, Li L, et al. miR-378 suppresses the proliferation, migration and invasion of colon cancer cells by inhibiting SDAD1. Cell Mol Biol Lett. 2017;22:12.
- Ahluwalia A, Jones MK, Matysiak-Budnik T, et al. VEGF and colon cancer growth beyond angiogenesis: does VEGF directly mediate colon cancer growth via a non-angiogenic mechanism? Cpd. 2014;20:1041–1044.
- Okugawa Y, Grady WM, Goel A. Epigenetic alterations in colorectal cancer: emerging biomarkers. Gastroenterology. 2015; 149:1204–1225.e12.
- Dhar S, Gursoy-Yuzugullu O, Parasuram R, et al. The tale of a tail: histone H4 acetylation and the repair of DNA breaks. Philos Trans R Soc Lond B Biol Sci. 2017;372:20160284.
- Chou RH, Wang YN, Hsieh YH, et al. EGFR modulates DNA synthesis and repair through Tyr phosphorylation of histone H4. Dev Cell. 2014;30:224–237.
- Yoon J, Kim SJ, An S, et al. integrative structural investigation on the architecture of human Importin4_Histone H3/H4_Asf1a complex and its histone H3 tail binding. J Mol Biol. 2018;430:822–841.
- Levy JMM, Towers CG, Thorburn A. Targeting autophagy in cancer. Nat Rev Cancer. 2017;17:528–542.
- Lin WM, Li ZG. Blockage of cisplatin-induced autophagy sensitizes cervical cancer cells to cisplatin. Genet Mol Res. 2015;14:16905–16912.
- Muilenburg D, Parsons C, Coates J, et al. Role of autophagy in apoptotic regulation by Akt in pancreatic cancer. Anticancer Res. 2014;34:631–637.
- Hanada T, Noda NN, Satomi Y, et al. The Atg12-Atg5 conjugate has a novel E3-like activity for protein lipidation in autophagy. J Biol Chem. 2007; 282:37298–37302.
- Fullgrabe J, Heldring N, Hermanson O, et al. Cracking the survival code: autophagy-related histone modifications. Autophagy. 2014;10:556–561.