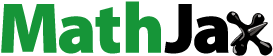
Abstract
Brucellosis has always been a threat to the health and economics of societies. We report a new colorimetric immunoassay based on colored silica nanoparticles for detection of Brucella abortus. An immunosensor was designed based on blue-SiNPs and paramagnetic nanoparticles (PMNPs). The synthesized immunosensor was conjugated with a polyclonal antibody against B. abortus, which was activated by 1-Ethyl-3–(3-dimethylaminopropyl)-carbodiimide (EDC) and N-hydroxysuccinimide (NHS) to form detection and capture probes, respectively. After adding the conjugates to the bacterial suspension, sandwich structure of PMNPs B. abortus-blue-SiNPs was formed and then separated by a magnet. The blue dye was released from the silica structure and its absorbance was measured at 670 nm with a spectrophotometer. Under optimal conditions, results showed a wide dynamic range from 1.5 × 103 to 1.5 × 108 cfu mL−1 with a detection limit of 450 cfu mL−1. The specificity of the sensor was confirmed in comparison with 5 other bacteria. Also, during the 120-days period, the complex was stable. The results suggested that it can be used in real samples (R2 = .9865). This designed colorimetric immunoassay strategy can be used as an alternative, user-friendly and on-site tool for the rapid detection of Brucella spp. compared to other common methods with high sensitivity and specificity in a short time.
Introduction
Brucellosis (Malta fever) is an ancient disease and one of the five most common bacterial zoonosis in the world that is caused by the Brucella spp. and threatens the health and the economy of the community [Citation1,Citation2]. It reduces lactation and power of fertility in animals. Infection is contagious and there is no effective treatment for bacteria. Also, it can infect various organs in human and causes a wide range of asymptomatic clinical manifestations until meningitis and endocarditis [Citation3,Citation4]. The survival power within phagocytosis cells is an important factor in the pathogenicity of the bacterium [Citation5].
Human brucellosis is rarely fatal and the transmission of the disease is not person-to-person but it can be transmitted through respiration with a small infective dose. Wide geographical distribution, use of multiple antibiotic and long-term treatment, lack of effective vaccine, as well as the limitations of common detection methods, are factors that describes the Brucella spp. as a threat to the health and the economy of the community [Citation6]. Various methods have been used for the detection of Brucella spp. from a long time such as culture-based methods, immuno-serologic tests, molecular and complex methods. Time-consuming, deficiency or lack of high sensitivity or specificity, need for expensive equipment, and trained personnel are their weaknesses [Citation7]. Using an immunosensor based on nanoparticles can be helpful. Since the selection of a suitable antibody is of importance, three characteristics should be considered in selecting antibodies for the identification of pathogen bacteria: First, the ability of the antibody to detect the smallest number of target bacterial cells (sensitivity). Second, identification of a particular strain in a mixture of different bacteria (specificity) and finally, high affinity for bonding with target bacteria antigen [Citation8]. Polyclonal antibodies can be a good choice for this purpose. They are a complex of immunoglobulin molecules that can react against a specific antigen and secrete through various B cells lineages [Citation9,Citation10].
Colorimetric immunoassay is a technique, which is based on the quantitative identification of the analytic by measuring the amount of light absorbed at a particular wavelength. In fact, changing the colour is the key to this technique. It is used for environmental monitoring, detection of pathogenic bacteria and food safety [Citation11,Citation12]. Enzymes and gold nanoparticles are mostly used in colorimetric technique but the critical operating situation, high price and short lifetime prevent them from being used as a routine test in laboratories [Citation13,Citation14]. Paramagnetic nanoparticles and Silica nanoparticles with fast kinetics in solution, quick and easy separation by the magnet, excellent compatibility and ease of synthesis, low cost of production and the presence of a large hydrophilic surface, have more applicability [Citation15]. In most studies, SiNPs have been used in white or colourless, which are not suitable for signal amplification and signal conversion [Citation16]. Therefore, organic dyes like C.I. Blue 21 can be used to compensate for this deficiency. These dyes are stable and do not fade in unfavourable conditions such as acid, alkali, light and heat. Wenchow Dou et al. used, successfully, magnetic and blue-silica nanoparticles in several studies for detection of Salmonella pullorum, 4.4 × 102 to 4.4 × 107 cfu mL−1, and Salmonella gallinarum, 8.8 × 101 to 8.8 × 108 cfu mL−1 [Citation14,Citation16]. Hemadi et al. detected Entamoeba histolytica successfully based on fluorescent silica nanoparticles [Citation17].
The purpose of the present study was to design an immunosensor based on coloured silica nanoparticles and using colorimetric strategy for rapid detection of B. abortus with high sensitivity and specificity. We employed coloured SiNPs as detection probes. Paramagnetic nanoparticles (PMNPs) have been used as capture probes and C.I. Blue 21 as reporter probes. Visible colour changing in samples justifies the presence of bacteria qualitatively and the absorbance of C.I. Reactive Blue 21 quantitatively shows a linear correlation with the concentration of B. abortus at 670 nm.
Materials and methods
Materials
Triton X-100, cyclohexane, hexanol, ammonia (25–28 wt%) were purchased from Merck (Merck, Germany). 3-[2-(2aminoethylamino) ethylamino] propyl-trimethoxysilane (APTMS), Tetraethyl Orthosilicate (TEOS), Bovine Serum Albumin (BSA), Fe2O3, Fe3O4, 1-Ethyl-3-(3-dimethylaminopropyl)-carbodiimide (EDC) and N-hydroxysuccinimide (NHS) were obtained from Sigma-Aldrich (Sigma-Aldrich, Merck, USA). C.I. Reactive Blue 21 was prepared by Santa Cruz (Santa Cruz, USA). Polyclonal antibody against Brucella abortus was purchased from Biorbyt, orb10564 (Biorbyt, USA). Brucella abortus 544, Brucella melitensis 16M, Escherichia coli O:157 ATCC4 3895, Salmonella typhimurium ATCC14028, Yersinia enterocolitica O:9 ATCC700823, Staphylococcus aureus ATCC49775 and Stenotrophomonas maltophilia ATCC13637 were purchased from Razi Vaccine and Serum Research Institute, Hesarak, Karaj, Iran, the water used was doubly distilled.
Synthesis of blue-SiNPs and blue-SiNP-NH2
The inverse microemulsion method was used for the synthesis of coloured silica nanoparticles. 2 mL surfactant Triton X-100, 8 mL oil phase cyclohexane, and 2 mL co-surfactant n-hexanol, 150 C.I. Reactive Blue 21 solution (100 mg mL−1) and 400
of distilled water were mixed in room temperature and stirred for 15 min with magnetic force. 100
TEOS, 33
APTMS and 100
ammonia were added to the complex. The reaction was completed after 48 h stirring. To break the microemulsion and recover the particles, 10 mL acetone was added for 30 min. Finally, the blue-SiNPs were washed several times with ethanol and water.
Introduction of amino groups onto the surface of blue-SiNPs was done overnight at room temperature. 30 mg of blue-SiNPs, 10 mL ethanol, 150 APTMS and 150
ammonia were mixed and stirred. Amino-modified blue-SiNPs (blue-SiNPs-NH2) collected after 10 min centrifugation at 8000 rpm, were washed three times with 0.01 M phosphate buffered saline solution (PBS, pH 7.3). They were dispersed in 1 mL of PBS and stored at 4 °C. Nanoparticles were characterized by Fourier transform infrared (FTIR) and scanning electron microscope (SEM) [Citation14,Citation18,Citation19].
Synthesis of PMNPs
1.6 g Fe3O4 and 0.8 g Fe2O3 dissolved in 20 mL of distilled water. The mixture was stirred at 80 °C for 20 min. 2 mL propanol and 2 mL ethanol were added to the complex for half an hour. Also, 20–30 mL ammonia was added dropwise for over an hour. The mixture condition was kept constant for 2 h after adding 10 mL TEOS and finally, PMNPs were formed by adding 5 mL APTMS after 2 h. PMNPs were washed 5–6 times with distilled water and characterized by FTIR and SEM and kept at 4 °C [Citation20].
Immobilization of polyclonal antibodies on the surface of blue-SiNPs-NH2 and PMNPs
0.0005 g EDC, 0.0002 g NHS, 5 polyclonal antibodies against Brucella abortus and 500 mL PBS were added to 30 mg blue-SiNPs-NH2 and PMNPs, respectively. After incubating at 37 °C and washed by PBS, IgG-blue-SiNPs and IgG-PMNPs were again incubated with 1 mL BSA 1% for 1 h. During this period, unreacted active groups were blocked on nanoparticles. Antibody modified nanoparticles were washed by PBS and stored at 4 °C [Citation19].
Culture of bacteria
At first, Brucella spp. were incubated for 72 h at 37 °C on Brucella broth medium and then transferred to Brucella agar medium for 48 h at 37 °C, and re-incubated. Other bacteria were cultured in nutrient-agar. After 24 h incubation at 37 °C, colonies were confirmed by a biochemical test, and stored at −70 °C before use [Citation21].
Colorimetric immunoassay and optimization
10 mg IgG-blue-SiNPs, 5 mg IgG-PMNPs with 1 mL microbial suspension containing Brucella abortus were mixed and incubated for 1 h at 37 °C with gentle shaking. During this period, IgG-blue-SiNPs and IgG-PMNPs formed a sandwich structure with bacteria by immune reaction. The final sandwich immune complexes were separated by a magnet and washed several times to remove unbound IgG-nanoparticles. C.I. Reactive Blue 21 was desorbed from the complex with 100 of NaOH aqueous solution (5 mol L−1) for 15–60 min. Afterwards, supernatants including an organic dye were separated. The absorbance of the dye was measured with a spectrophotometer at 670 nm [Citation14,Citation16]. The colorimetric immunoassay was repeated with different concentrations of antibody, coloured silica and PMNPs to achieve optimal analytical performance. Also, all incubation times were evaluated for conjugation of each nanoparticle with activators and bacteria.
Sensitivity and specificity
According to the 0.5 McFarland standard, (mixing 0.05 mL of 1.175% barium chloride dehydrate (BaCl2 2H2O) with 9.95 mL of 1% sulfuric acid (H2SO4); ∼cell density of 1.5 × 108 cfu mL−1 and the absorbance at a wavelength of 625 nm should be 0.08 to 0.13 ), serial dilutions of the B. abortus, which were descending from 1.5 × 108 to 1.5 × 10 cfu mL−1, were prepared. Sensitivity was evaluated by dilutions assay. In order to determine the specificity, a colorimetric immunoassay was done by B.abortus 544, B. melitensis 16M, E. coli O:157, S. typhimurium, Y. enterocolitica O:9, S. aureus, S. maltophilia and PBS as a negative control [Citation14].
Stability and applicability
Performance of PMNPs B. abortus-coloured-SiNPs complex was measured on days 7, 15, 30, 60, 90 and 120 to investigate the stability of the conjugated nanoparticles with the antibody. Applicability was evaluated by real samples of whey and milk. Serial dilutions of B. abortus, 1.5 × 108 to 1.5 × 10 cfu mL−1 were prepared and added to each sterilized sample. The colorimetric immunoassay was repeated. Dye absorbance was recorded separately for each dilution and the performance was calculated finally [Citation14].
Results
Characterization of nanoparticles
Coloured silica nanoparticles were physically bright with uniform dispersion rate in aqueous solution and according to the SEM had a smooth surface, spherical shape with an average size of 43–56 nm ().
Figure 1. (A) The SEM image of blue-SiNPs. Spherical shape and 43–56 nm; (B) FTIR spectrum of blue-SiNPs (top) and blue-SiNP-NH2 (bottom). The band at 2945 cm−1 in the blue-SiNP-NH2 is assigned to the N–H of the silica; (C) The SEM image of PMNPs, 58–72 nm, uniform and spherical; (D) FTIR spectrum of Fe3O4-TEOS (top) and Fe3O4-TEOS-APTMS (bottom). The band at 2923 cm−1 is assigned to C–H of the Fe3O4-TEOS-APTMS. The band at 3450 cm−1 refers to the N–H stretching vibration. Bands around 3430.7 and 1630 cm−1 are assigned to amide groups.
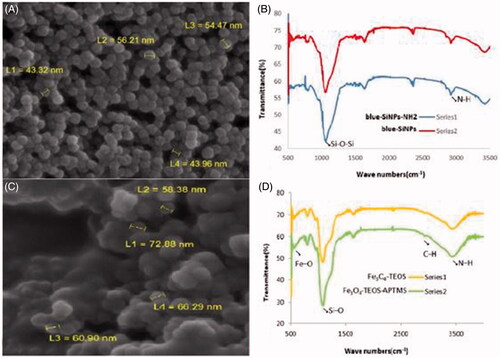
On the other hand, the FTIR spectrum was used for confirming the presence of amine groups on the surface of these nanoparticles. Dried samples from blue-SiNPs and blue-SiNP-NH2 were prepared by KBr pellet method in the range of 400–4000 cm−1. The band at the region 980–1220 cm−1 is corresponding to the Si–O–Si of the silica core. The band at 2945 cm−1 in the blue-SiNP-NH2 is assigned to the N–H of the silica ().
Based on the SEM results, size of PMNPs was determined to be 58–72 nm with uniform, spherical, and smooth surface dispersion, which is consistent with the findings of Amini et al. ().
Also, FTIR analysis was done for these particles in the region 400–4000 cm−1. The band at 580–630 cm−1is corresponding to the Fe3O4-TEOS vibration, which is related to the magnetite phase, Fe–O. The band at 2923 cm−1is assigned to C–H of the Fe3O4-TEOS-APTMS ().
The band at 3450 cm−1is referred to the N–H stretching vibration. Bands around 3430.7 and 1630 cm−1 were assigned to amide groups. Bands at 1017.3 and 1030 cm−1 were assigned to the Si–O stretching vibration of 3- Aminopropyltriethoxysilane (APTES) on the surface of PMNPs.
Optimization
Different amounts of IgG-PMNPs (0.25, 0.5, 1, 3, 5 and 7 mg), IgG-blue-SiNPs (1, 5, 10 and 15 mg) and antibody (1, 3, 5, 7 and 10 μL) were prepared and the experiment was repeated. According to the results, the best concentration was determined for IgG-PMNPs 5 mg, IgG-blue-SiNPs 10 mg, and antibody 5 μL. ().
Figure 2. Condition optimization results for rapid colorimetric detection of B. abortus, (A) PMNPs and blue-SiNP-NH2 optimization results, optimum concentration of PMNPs 5 mg and blue-SiNP-NH210 mg were determined; (B) 5 μL was the optimized concentration of the antibody; (C) Time optimization results, best time for conjugation of antibody with EDC/NHS complex was calculated as 240 min; (D) Optimized time for conjugated of bacteria with nanoparticles was 60 min.
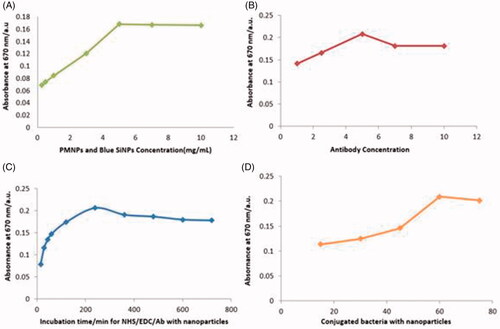
No significant changes were observed in the results with increasing of concentrations. Incubation times for antibody with EDC/NHS were optimized. Antibody and activators were incubated in 37 °C for periods of time 15, 30, 45, 60, 120, 240, 360, 480, 600 and 720 min. Binding between the antibody and activators increased at 240 min and the trend remained constant with a slight decrease in 600 and 720 min (). The 240 min period was chosen as the optimal time for conjugation between the antibody and EDC/NHS. Nanosensors and microbial suspension were incubated at 37 °C under an optimized situation for 15, 30, 45, 60 and 75 min. Results at 60 min showed that maximum interaction occurred between bacteria and nanoparticles. Then the curve decreased slightly and finally stayed linear ().
Sensitivity
To explore the sensitivity of nanosensors, serial dilutions of B. abortus were prepared according to 0.5 McFarland standard from 1.5 × 108 to 1.5 × 10 cfu mL−1. A colorimetric assay was performed based on optimal conditions with serial dilutions ().
Figure 3. (A) Visual results of the colorimetric assay by serial dilutions of the B. abortus from 1.5 × 108 to 1.5 × 10 cfu mL−1, by reducing the concentration of bacteria the intensity of the color decreases; (B) A linear dependence between the dye absorbance and logarithm of B. abortus concentration.
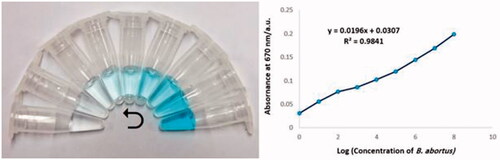
Absorption was measured for each dilution and compared with each other (). Based on results, maximum detection was in the range of 1.5 × 103 to 1.5 × 108 cfu mL−1, (y = 0.0197x + 0.0306; R2 = .9841), a linear dependence between the absorbance and logarithm of B. abortus concentration. Limit of detection (LOD) area was between two dilutions 1.5 × 102 and 1.5 × 103 cfu mL−1. This area was divided into smaller components. New dilutions were prepared from 100–900 cfu mL−1 and the test was repeated. LOD was determined at 1.5 × 300 (450) cfu mL−1.
Specificity
Identification of specific bacteria was evaluated in a mixture of different bacteria. For this, the assay was performed in the presence of B. abortus 544, B. melitensis 16M, E. coli, S. typhimurium, Y. enterocolitica, S. aureus, S. maltophilia and PBS as a negative control, respectively. The results demonstrated the highest rate of absorption belonged to the B. abortus and B. melitensis, although a slight increase was seen in the absorption of E. coli and Y. enterocolitica samples (.
Figure 4. Specificity of the colorimetric immunoassay. From left to right, PBS (0.01 mol mL−1), Brucella abortus 544 (1.5 × 108 cfu mL−1), Brucella melitensis 16 M (1.5 × 108 cfu mL−1), Escherichia coli O:157 (1.5 × 108 cfu mL−1), Salmonella typhimurium (1.5 × 108 cfu mL−1), Yersinia enterocolitica O:9 (1.5 × 108 cfu mL−1), Staphylococcus aureus (1.5 × 108 cfu mL−1), Stenotrophomonas maltophilia (1.5 × 108 cfu mL−1).
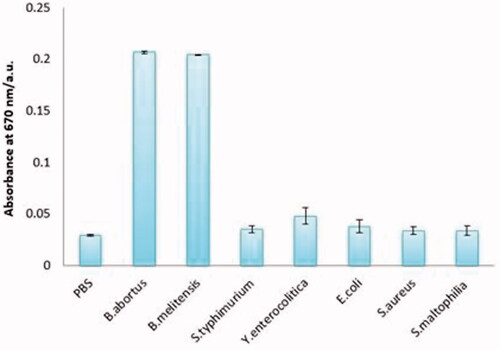
Stability
Stability of conjugated nanoparticles with antibody and organic dye in the long-term can be considered as one of the important factors in the evaluation of biosensor performance. Therefore, these factors were investigated in a 4-month period. The colorimetric assay was repeated on days 7, 15, 30, 60, 90 and 120. There was no significant change in performance of immunosensor (.
Applicability
Biosensor efficiency was calculated by different dilutions of B. abortus according to 0.5 McFarland standard. After the preparation, different dilutions were added to sterile samples of whey and milk. Colorimetric assay results showed the correlation coefficient (R2) was equal to .9865, (y = 0.019x + 0.030).
Discussion
The colour changing is our strategy in identification of pathogen in this study, on the other hand, transfer of dye into silica particles has always been a major challenge because of hydrophilic environments that do not have the ability of inserting the hydrophobic dye into the particles, so in this study, C.I. Reactive Blue 21, a polar and organic dye was used to increase the electrostatic attraction of the dye molecules with a negative charge in the silica matrix [Citation22]. Among the various available methods for the synthesis of coloured silica nanoparticles, w/o method was selected for easy control of the particles size, cost-effectiveness, thermodynamic and isotropic properties [Citation14,Citation23]. The particle size in this method is influenced by the nature of the surfactant molecules, the concentration of tetraethyl orthosilicate and ammonium, as well as, the molar ratio of water to the surfactant [Citation18]. Our sanitized blue silica nanoparticles were consistent with the above methods. FTIR spectrum analysis confirmed that blue-SiNPs have been successfully coated with amine silica shells and APTMS have been successfully introduced onto the surface of the silica modified nanoparticles. Comparison of the absorbance spectra showed a significant difference in the region 2900–3450 cm−1. These results are in accordance with the descriptions by Yu et al. and Sun et al. [Citation14,Citation16,Citation19,Citation24]. Synthesis of PMNPs was also done by a co-precipitation method, ease of work and economic viability of this method are important advantages. Temperature plays a significant role in this method. At below 60 °C the Fe2O3 production is higher but Fe3O4 will produce more at temperatures higher than 80 °C. Here, our manufactured nanoparticles are consistent with Bordbar et al. and Hariani and Amini et al’s descriptions [Citation20,Citation25,Citation26]. By investigating the FTIR spectra results, the introduction of amine groups onto the surface of paramagnetic nanoparticles was confirmed.
The sensitivity of immunosensor was compared with other similar studies. According to this, Wen et al., by colorimetric technique and immunomagnetic capture, detected Shewanella oneidensis with a wide dynamic range between 5.0 103 and 5.0
106 cfu mL−1 toward target cells [Citation27]. Sun et al. detected S. pullorum by polyclonal antibody and blue-silica nanoparticles ranging from 4.4 × 102 to 4.4 × 107 cfu mL−1 [Citation14], and also detected S. pullorum and S. gallinarum by silica and magnetic nanoparticles in milk powder 8.8 × 102 cfu mL−1 [Citation16]. Song et al. detected Brucella by using polyclonal antibody-conjugating quantum dots and antibody modified magnetic beads, 102–105 cfu mL−1 [Citation28]. Wu et al. determined B. melitensis by sensitivity method and using gold nanoparticles-screen-printed carbon electrode 1 × 104 to 4 × 105 cfu mL−1 [Citation29]. Bayramoglu et al. detected Salmonella in milk samples by Fe3O4/SiO2/pGMA and MCM-41 particles equal to 103 cfu mL−1 [Citation30].
Li et al. based on immunomagnetic beads and quantum dots detected Brucella spp. through a sandwich immunoassay [Citation31]. Wu et al. were able to detect as low as 1 × 104 and 4 × 105 cfu mL−1 of Brucella melitensis in pure culture and milk samples by a label-free impedance immunosensor based on a gold nanoparticle-Modified Screen-Printed Carbon Electrode [Citation29]. Liu et al., by colorimetric immunoassay and using core gold nanoparticles, silver nanoclusters as oxidase mimetics, and aptamer-conjugated magnetic nanoparticles, detected Listeria monocytogenes in the10 to 106 cfu mL−1 concentration range without pre-enrichment [Citation32]. In the present work, we used a polyclonal antibody against B. abortus, blue-SiNPs, PMNPs and UV spectroscopy detection range was from 1.5 × 103 to 1.5 × 108 cfu mL−1 and LOD determined 450 cfu mL−1. Some of these methods are better for identification of bacteria but they are not recommended for routine laboratory because of limitations such as complexity, expensive prices and inability to use them at any location and any time with the minimum equipment and trained personnel.
In the evaluation of specificity, the highest level of reaction was related to B. abortus and B. melitensis, which is an important advantage for the prepared immunosensor because both species are the most prevalent cause of Brucellosis and they have similar preventive and therapeutic measures. On the other hand, a slight increase occurred in the absorbance of E. coli and Yersinia because of similarity in the O-antigenic side chain of LPS of Brucella and other organisms like Y. enterocolitica O:9, Vibrio cholerae, E. coli O:157, and Francisella tularensis [Citation33,Citation34]. The nearest non-specific absorbance to Brucella spp. was Yersinia O:9. So, based on OD, diagnostic cut off in this method was calculated to be equal to 0.078877 (Mean ± SD for Yersinia O:9 and B. abortus were determined to be equal to 0.056188 and 0.078877, respectively).
Compared to the enzyme-coated nanoparticles, immuno-nano-biosensor had better stability and its performance did not change significantly on day 120 compared to the first day. The important reasons are the formation of covalent bonds on the surface of the nanoparticles with anti-Brucella antibodies, as well as the replacement of labelled enzyme antibodies with modifying antibodies in the silica surface [Citation14,Citation16,Citation24].
Conclusions
Conventional methods for diagnosis of B. abortus requires a laboratory and they are not capable of detecting the bacteria in a short time period and some of them lack high sensitivity and specificity despite economic efficiency; while the immuno-nano-biosensor, which was designed, has high sensitivity and specificity for detection of B. abortus. In addition, qualitative and quantitative identification is carried out, which is the greatest advantage and an important factor in situations where there is no access to a lab. The maximum time for detection of B. abortus in real samples was 90 min. In summary, this designed colorimetric immunoassay strategy can be used as an alternative, user-friendly and on-site tool for the rapid diagnosis of Brucella spp. compared to other common methods, with high sensitivity and specificity in a short time.
Disclosure statement
No potential conflict of interest was reported by the authors.
Correction Statement
This article was originally published with errors, which have now been corrected in the online version. Please see Correction (http://dx.doi.org/10.1080/21691401.2019.1674496)
References
- El-Diasty M, Wareth G, Melzer F, et al. Isolation of Brucella abortus and Brucella melitensis from seronegative cows is a serious impediment in brucellosis control. Vet Sci. 2018;5:28.
- Minda AG, Gezahegne MK. A review on diagnostic methods of brucellosis. J Vet Sci Technol. 2016;7:1–8.
- Alamian S, Esmaelizad M, Zahraei T, et al. A novel PCR assay for detecting Brucella abortus and Brucella melitensis. Osong Public Health Res Persp. 2017;8:65.
- Petrović M, Ž. Cvetnić IOP conference series earth and environmental science. Bristol, England: IOP Publishing; 2017. Brucellosis-the past, the present, the future; p. 012019.
- Jahandeh N, et al. Different methods for diagnosis of Brucella and Legionella spp. in various samples. J Appl Biotechnol Rep. 2015;2:187–190.
- Higgins J, et al. Molecular epidemiology of Brucella abortus isolates from cattle, elk, and bison in the United States: 1998–2011. Appl Env Microbiol. 2012;78:3674–3684.
- Kaden R, et al. A novel real-time PCR assay for specific detection of Brucella melitensis. BMC Infect Dis. 2017;17:230.
- Byrne B, Stack E, Gilmartin N, et al. Antibody-based sensors: principles, problems and potential for detection of pathogens and associated toxins. Sensors 2009;9:4407–4445.
- Actor JK. Introductory immunology: basic concepts for interdisciplinary applications.Waltham, MA: Academic Press; 2014.
- Sharma S, Byrne H, O’Kennedy RJ. Antibodies and antibody-derived analytical biosensors. Essay Biochem. 2016;60:9–18.
- Alamer S, Chinnappan R, Zourob M. Development of rapid immuno-based nanosensors for the detection of pathogenic bacteria in poultry processing plants. Procedia Technol. 2017;27:23–26.
- Wang X, Niessner R, Knopp D. Magnetic bead-based colorimetric immunoassay for aflatoxin B1 using gold nanoparticles. Sensors 2014;14:21535–21548.
- Shahbazi R, Salouti M, Amini B, et al. Highly selective and sensitive detection of Staphylococcus aureus with gold nanoparticle-based core-shell nano biosensor. Mol Cell Probes. 2018;41:8–13.
- Sun Q, Zhao G, Dou W. Blue silica nanoparticle-based colorimetric immunoassay for detection of Salmonella pullorum. Anal Meth. 2015;7:8647–8654.
- Narmani A, Kamali M, Amini B, et al. Highly sensitive and accurate detection of Vibrio cholera O1 OmpW gene by fluorescence DNA biosensor based on gold and magnetic nanoparticles. Proc Biochem. 2018;65:46–54.
- Sun Q, Zhao G, Dou W. An optical and rapid sandwich immunoassay method for detection of Salmonella pullorum and Salmonella gallinarum based on immune blue silica nanoparticles and magnetic nanoparticles. Sens Actuat B: Chem. 2016;226:69–75.
- Hemadi A, Ekrami A, Oormazdi H, et al. Bioconjugated fluorescent silica nanoparticles for the rapid detection of Entamoeba histolytica. Acta Tropica. 2015;145:26–30.
- Bagwe RP, Yang C, Hilliard LR, et al. Optimization of dye-doped silica nanoparticles prepared using a reverse microemulsion method. Langmuir 2004;20:8336–8342.
- Yu H, Zhao G, Dou W. Simultaneous detection of pathogenic bacteria using agglutination test based on colored silica nanoparticles. CPB. 2015;16:716–723.
- Amini B, Kamali M, Salouti M, et al. Fluorescence bio-barcode DNA assay based on gold and magnetic nanoparticles for detection of Exotoxin A gene sequence. Biosens Bioelectron. 2017;92:679–686.
- Siadat SD, Vaziri F, Eftekhary M, et al. Preparation and evaluation of a new lipopolysaccharide-based conjugate as a vaccine candidate for brucellosis. Osong Publ Health Res Persp. 2015;6:9–13.
- Farias S, de Oliveira D, de Souza AAU, et al. Removal of reactive blue 21 and reactive red 195 dyes using horseradish peroxidase as catalyst. Braz J Chem Eng. 2017;34:701–707.
- Kong L, Uedono A, Smith SV, et al. Synthesis of silica nanoparticles using oil-in-water emulsion and the porosity analysis. J Sol-Gel Sci Technol. 2012;64:309–314.
- Sun Q, Zhao G, Dou W. A nonenzymatic optical immunoassay strategy for detection of Salmonella infection based on blue silica nanoparticles. Analytica Chimica Acta. 2015;898:109–115.
- Bordbar AK, Rastegari AA, Amiri R, et al. Characterization of modified magnetite nanoparticles for albumin immobilization. Biotechnol Res Int. 2014;2014:1.
- Loekitowati Hariani P, et al. Synthesis and properties of Fe3O4 nanoparticles by co-precipitation method to removal procion dye. IJESD. 2013;4:336–340.
- Wen J, Zhou S, Chen J. Colorimetric detection of Shewanella oneidensis based on immunomagnetic capture and bacterial intrinsic peroxidase activity. Sci Rep. 2014;4:5191.
- Song D, et al. A rapid detection method of Brucella with quantum dots and magnetic beads conjugated with different polyclonal antibodies. Nanoscale Res Lett. 2017;12:179.
- Wu H, Zuo Y, Cui C, et al. Rapid quantitative detection of Brucella melitensis by a label-free impedance immunosensor based on a gold nanoparticle-modified screen-printed carbon electrode. Sensors 2013;13:8551–8563.
- Bayramoglu G, et al. Fast and sensitive detection of Salmonella in milk samples using aptamer-functionalized magnetic silica solid phase and MCM-41-aptamer gate system. ACS Biomater Sci Eng. 2018;4:1437–1444.
- Li L, Yin D, Xu K, et al. A sandwich immunoassay for brucellosis diagnosis based on immune magnetic beads and quantum dots. J Pharmac Biomed Anal. 2017;141:79–86.
- Liu Y, et al. Colorimetric immunoassay for Listeria monocytogenes by using core gold nanoparticles, silver nanoclusters as oxidase mimetics, and aptamer-conjugated magnetic nanoparticles. Microchimica Acta 2018;185:360.
- Christopher S, Umapathy B, Ravikumar K. Brucellosis: review on the recent trends in pathogenicity and laboratory diagnosis. J Lab Phys. 2010;2:55.
- Maldonado RF, Sa-Correia I, Valvano MA. Lipopolysaccharide modification in Gram-negative bacteria during chronic infection. FEMS Microbiol Rev. 2016;40:480–493.