Abstract
Numerous differentially expressed long non-coding RNAs (lncRNAs) have been identified in cerebral ischemia-reperfusion (I/R) injury using RNA-Seq analysis. However, little is known about whether and how lncRNAs are involved in cerebral I/R injury. In this study, we investigated the function of the lncRNA Oprm1 in cerebral I/R injury and explored the underlying mechanism. An oxygen-glucose deprivation model in N2a cells was utilized to mimic cerebral I/R injury in vitro. Trypan blue staining, terminal deoxytransferase-mediated dUTP-biotin nick end labelling and caspase-3 were measured to evaluate apoptosis. Middle cerebral artery occlusion was performed in mice to evaluate the function of lncRNA Oprm1 in vivo. Real-time PCR and western blotting were used to measure the expression levels of lncRNA Opmr1, caspase-3, miR-155, GATA binding protein 3 (GATA3) and nuclear factor (NF)-κB. lncRNA Oprm1 was mainly located in the cytoplasm. Overexpression of lncRNA Oprm1 alleviated the apoptosis induced by oxygen-glucose deprivation and significantly reduced cleaved caspase-3 levels. Infarct size was distinctly decreased in the lncRNA Oprm1-overexpression group. The neurological score was also improved. Our findings showed that the lncRNA Oprm1/miR-155/GATA3 axis plays an important role in cerebral I/R injury. lncRNA Oprm1 may attenuate cerebral injury through the NF-κB pathway. lncRNA Oprm1 may serve as a potential target for new therapeutic interventions in patients with ischemic stroke.
Keywords:
Introduction
Ischemic stroke accounts for approximately 87% of all strokes [Citation1] and seriously affects the quality of life. Ischemia can lead to a series of neurological events including hypoxia [Citation2], oxidative stress [Citation3], endoplasmic reticulum stress [Citation4], inflammation [Citation5] and edema formation [Citation6], triggering the death of neuronal cells in the ischemic core [Citation7–9]. Although great advancements have been made in characterizing the specific mechanism of stroke-induced neuronal cell death, few effective approaches exist that can reverse this process. Thus, exploring new intervention techniques will provide new clues and insights for the treatment of stroke.
Long non-coding RNAs (lncRNAs) are a series of transcripts longer than 200 bp without coding potential [Citation10–12]. An increasing number of studies have demonstrated that lncRNAs serve significant functions in biological and pathological processes, including heart disease [Citation13], myocardial infarction [Citation14], stroke [Citation11], obesity [Citation15], and tumour development [Citation16–19]. The mechanisms underlying the actions of lncRNAs are also diverse, including regulation of the neighbouring gene [Citation20], microRNA (miRNA)-sponge action [Citation21], binding to a target protein, and DNA repair [Citation22]. However, the relationship between lncRNAs and stroke remains largely unknown. These observations indicate that identifying new mechanisms underlying the action of lncRNAs in stroke will also shed new light on the clinical treatment of stroke.
Numerous differentially expressed lncRNAs were identified by Haitao et al. in cerebral ischemia-reperfusion (I/R) injury using RNA-Seq analysis [Citation23]. However, little is known about whether and how lncRNAs are involved in cerebral I/R injury. In the present study, we investigated the function of the lncRNA Oprm1 and explored its possible mechanism in cerebral I/R injury. An oxygen-glucose deprivation (OGD) model in N2a cells was utilized to mimic cerebral I/R injury in vitro. Trypan blue staining, terminal deoxytransferase-mediated dUTP-biotin nick end labelling (TUNEL), and caspase-3 were measured to evaluate apoptosis. Middle cerebral artery occlusion (MCAO) was performed in mice to evaluate the function of lncRNA Oprm1 in vivo. LncRNA Oprm1 was located mainly in the cytoplasm. Overexpression of lncRNA Oprm1 alleviated the apoptosis induced by OGD and significantly reduced the levels of cleaved caspase-3. Infarct size was distinctly decreased in the lncRNA Oprm1-overexpression group. The neurological score was also improved. We found that that the lncRNA Oprm1/miR-155/GATA binding protein 3 (GATA3) axis plays an important role in cerebral I/R injury. Our findings indicate that lncRNA Oprm1 may serve as a potential target for new therapeutic interventions. Undoubtedly, exploring the precise role of lncRNA Oprm1 in cerebral I/R injury will provide new insights into the ischemic stroke.
Materials and methods
Cell culture and OGD/reoxygenation injury
The mouse neuroblastoma cell line Neuro-2a (N2a) was cultured in Dulbecco’s modified Eagle’s medium (DMEM; Gibco, Carlsbad, CA) containing 10% fetal bovine serum (Gibco) in 5% CO2 at 37 °C. For the OGD model, the culture medium was replaced with glucose-free DMEM, and the cells were placed in an anaerobic chamber saturated with 95% N2 and 5% CO2 at 37 °C for 3 h. After exposure for 3 h, the medium was replaced with normal culture medium for 4, 8 and 12 h to reoxygenate the cells. The control group was cultured continuously in a normal medium under normal conditions.
Gene transfection
lncRNA Oprm1, miR-155 mimics and GATA3 were synthesized by Shanghai GenePharma (Shanghai, China). Briefly, N2a cells were transfected with Oprm1 or negative control (NC) when cell confluence reached 70%. After transfection for 48 h, the cells were exposed to the OGD model with reoxygenation for different lengths of time.
Cell death rate assay
The rate of cell death was measured using trypan blue (C0011; Beyotime, Shanghai, China) staining. The cells were digested for 3 min and then pipetted thoroughly.
Nuclear and chromatin RNA fractionation
The nuclear and cytoplasmic fractions of N2a cells were isolated using a PARIS Kit (Thermo Fisher Scientific, Rockford, IL). A total of 1.0 × 107 cultured cells were collected, placed on ice, and resuspended in 500 µL ice-cold cell fractionation buffer. All samples were centrifuged at 500 × g for 5 min, and the cytoplasmic fraction was removed carefully from the nuclear pellet fraction. The pellet was washed twice in ice-cold cell fractionation buffer to prevent contamination of the nuclear fraction with components from the cytoplasmic fraction.
TUNEL staining
A TUNEL assay was performed to detect the level of apoptosis. Cells were washed with phosphate-buffered saline twice and fixed in 4% paraformaldehyde. The apoptotic cells were visualized with TUNEL staining according to the manufacturer’s instructions (C1090; Beyotime, China). Fluorescence density was assessed using ImageJ software.
Organ collection
Six C57/B6 mice were sacrificed and the brain, heart, liver, spleen, lung, kidney, and pancreas were collected. The samples were treated with the TRIzol reagent (Thermo Fisher Scientific, Waltham, MA) and total RNA was extracted according to the manufacturer’s instructions.
Animals and MCAO model
Focal cerebral ischemia was performed by MCAO using an intraluminal monofilament technique as described previously [Citation24]. Briefly, the mice were anesthetized with isoflurane. After isolating the right common carotid artery, a 6–0 suture was tied at the origin of the right external carotid artery (ECA) and at the distal end of the ECA. The left middle cerebral artery was occluded by inserting a 6–0 nylon monofilament with a thin silicon coat. After 2 h occlusion, it was removed to allow reperfusion for 6, 12, or 24 h with the ECA tied permanently.
After anesthetization, lentivirus Ad-Oprm1 and its control Ad-NC (109 TU/mL, GenePharma, Shanghai, China) were mixed with the cationic lipid polybrene (4 μg/μL, GenePharma) and incubated at 37 °C for 15 min before MCAO. Subsequently, the mixture was delivered into the cortex of ischemia region by microliter syringes (Hamilton CO., Reno, NV) for 30 min. After reperfusion, the mice were sacrificed and the brains were sliced for further research.
Neurobehavioral evaluation
After reperfusion for 24 h, a neurobehavioral score was calculated as reported previously [Citation24]. A neurobehavioral score of less than 2 was considered normal and such animals were eliminated from the study.
2,3,5-Triphenyltetrazolium chloride monohydrate staining
After reperfusion, 2,3,5-triphenyltetrazolium chloride monohydrate (TTC) was used to evaluate infarct size. The mice were sacrificed and their brains were isolated quickly and frozen in isopentane for cryostat sectioning. Serial coronal sections (1 mm apart) were stained with TTC to assess infarct size. Infarct volume was calculated as described previously [Citation24].
Luciferase reporter assay
The 3′-untranslated region (UTR) of GATA3, with wild-type (WT) or mutant (mut) binding sites for miRNA-155, was amplified and cloned into the pGL3 vector (Promega, Madison, WI) to generate the plasmid pGL3-WT-GATA3-3′-UTR or pGL3-mut-GATA3-3′-UTR. HEK 293 cells were used to perform the luciferase reporter assay, and the miR-155 mimics or inhibitor and the GATA3 vector were transfected using the Lipofectamine 2000 reagent (Thermo Fisher Scientific, Waltham, MA). Luciferase activity was analyzed using the Dual-Luciferase Reporter Assay System following the manufacturer’s protocol.
Real-time PCR
Amplification of cDNA was performed with SYBR Green Real-time qPCR SuperMix UDG reagents (Invitrogen, Carlsbad, CA) and the MX3000P system (Stratagene, La Jolla, CA). The following cycling conditions were used: 95 °C for 15 s, then 40 cycles of 95 °C for 15 s, 60 °C for 15 s and 68 °C for 20 s. The fold-change of lncRNA Oprm1 expression was calculated by the 2−ΔΔCt method following normalization to GAPDH.
Western blotting
Proteins from N2a cells were extracted by using RIPA buffer. A bicinchoninic acid assay was utilized to measure protein concentration. The extracted proteins were subjected to 10% sodium dodecyl sulfate-polyacrylamide gel electrophoresis and transferred onto 0.22-µm polyvinylidene fluoride membranes (REF 03010040; Roche, Pleasanton, CA). The membranes were blocked with 5% non-fat milk and incubated with anti-caspase-3, anti-GATA3, anti-p65, anti-IκBα, anti-histone H1, anti-tubulin (all from Santa Cruz Biotechnology, Santa Cruz, CA) and anti-β-actin antibodies (Abcam, Cambridge, MA) overnight at 4 °C. After washing five times (6 min each time), the membranes were incubated with horseradish peroxidase-conjugated secondary antibodies (Abcam, Cambridge, MA) for 1 h at room temperature. The bands were visualized by using an enhanced chemiluminescent kit (Thermo Fisher Scientific, Waltham, MA). Immunopositive bands were detected using a FluorChem M system (ProteinSimple, San Jose, CA).
RNA immunoprecipitation (RIP)
RIP experiments were conducted using the Magna RIP RNA-Binding Protein Immunoprecipitation kit (Catalog No. 17–700, Millipore, Burlington, MA). N2a cells were lysed and the whole cell lysates were cultured with protein magnetic beads, which were conjugated using 2 μg of GATA3 antibodies (Abcam, Cambridge, MA) or control IgG at 4 °C for overnight. The immunoprecipitated RNA was purified and performed by RT-qPCR.
Statistical analysis
All graph plotting and data analyses were performed by using SPSS software 21.0 (SPSS Inc., Chicago, IL). All data are shown as the mean ± standard deviation. Significant differences between groups were analyzed by a t-test (comparison of two groups) or χ2-test or one-way analysis of variance (comparison of more than two groups). p < .05 was considered to be statistically significant.
Results
Characteristics of lncRNA Oprm1
To study the function of lncRNA Oprm1, firstly, we detected the distribution of lncRNA Oprm1 in different mouse organs. To our surprise, lncRNA Oprm1 was mainly distributed in heart tissue (), suggesting that it may possess a potential function in the heart. Next, we examined the location of lncRNA Oprm1. Cell fractionation analysis demonstrated that lncRNA Oprm1 was mainly located in the cytoplasm ().
lncRNA Oprm1 alleviates OGD-induced apoptosis in N2a cells
To explore the possible function of lncRNA Oprm1 in cerebral stroke, we first constructed an overexpression vector for Oprm1. Oprm1 expression was increased by approximately 8-fold compared with the NC (). Next, we used the OGD model to detect changes in lncRNA Oprm1 expression. Similarly, lncRNA Oprm1 expression was decreased as reperfusion time was increased (), suggesting that it may function in the OGD model. To verify the function of lncRNA Oprm1, trypan blue staining was used to evaluate the rate of cell death after OGD exposure. Cell death was significantly decreased in the lncRNA Oprm1-overexpression group (). TUNEL staining revealed similar results, suggesting that lncRNA Oprm1 exerted an anti-apoptotic effect (). We also detected the expression of cleaved-caspase-3 using western blotting, and cleaved-caspase-3 was manifestly reduced in the lncRNA Oprm1-overexpression group (). Moreover, we used flow cytometry to analyze the apoptosis rates after Oprm1 overexpression. Overexpression of Oprm1 significantly reduced the cell apoptosis (). These results suggested that lncRNA Oprm1 may possess an anti-apoptosis function in cerebral ischemic injury.
Figure 2. Functional analysis of lncRNA Oprm1 in vitro. (A) Overexpression of Oprm1 was verified by qPCR. (B) Oprm1 was down expressed with the development of reperfusion. (C) Overexpression of Oprm1 significantly alleviated cell apoptosis induced by OGD. (D) Tunel assay suggested that Tunel positive cells were significantly reduced in Oprm1 group. (E) Cleaved-caspase3 was decreased in Oprm1 overexpressed group. (F) Overexpression of Oprm1 significantly reduced the cell apoptosis using flow cytometry. **p < .01, ***p < .001.
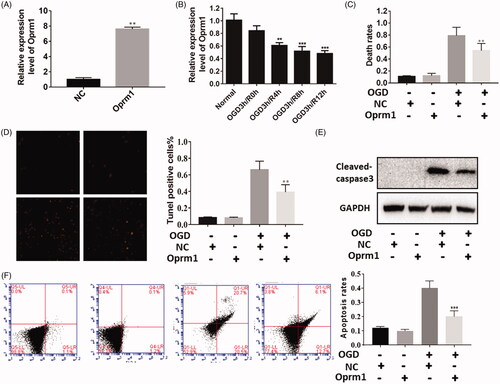
Effect of lncRNA Oprm1 in MCAO-induced injury in vivo
To elucidate further the function of lncRNA Oprm1, animal experiments were performed. First, overexpression efficiency was verified through real-time PCR, and lncRNA Oprm1 expression was increased by approximately 4-fold (). Next, an MCAO-induced injury model was used to evaluate the function of lncRNA Oprm1. Infarct size, which was measured by TTC staining, was significantly decreased in the lncRNA Oprm1-overexpression group, whereas no change was detected in the NC group (). We also evaluated the neurological score, and lncRNA Oprm1 clearly reduced the score (). We also performed a TUNEL assay to analyze the apoptosis effect in vivo. Overexpression of Oprm1 significantly reduced the apoptosis rates (). Next, we used real-time PCR to elucidate the expression level of lncRNA Oprm1 in different tissues with the development of reperfusion in vivo. Interestingly, lncRNA Oprm1 expression was decreased in ischemic core tissue, whereas no manifest difference was detected in the penumbra region, suggesting that lncRNA Oprm1 may function in ischemic core tissue (). Lastly, we collected ischemic tissue and analyzed the levels of cleaved-caspase-3 using western blotting. lncRNA Oprm1 overexpression significantly decreased the activation of cleaved-caspase-3 (). These results demonstrated that lncRNA Oprm1 could alleviate MCAO-induced injury in vivo.
Figure 3. Functional analysis of lncRNA Oprm1 in vivo. (A) Overexpression of lncRNA Oprm1 was verified through qPCR. (B) Infarct size was measured via TTC staining, and infarct size was significantly reduced in Oprm1 overexpressed group. (C) There is no difference in penumbra. (D) Overexpression of Oprm1 significantly reduced the cell apoptosis. (E) The expression of Oprm1 was manifestly reduced in the development of reperfusion. (F) Cleaved-caspase3 was distinctly decreased in Oprm1 overexpressed group in vivo. **p < .01.
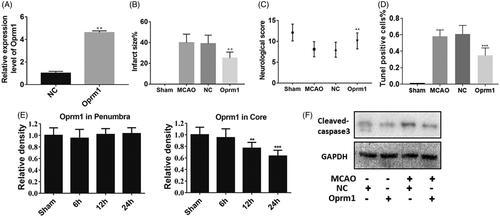
miR-155 is a target of lncRNA Oprm1
To better understand the mechanism of lncRNA Oprm1 in cerebral ischemic injury, we used TargetScan to predict potential miRNAs that may bind to lncRNA Oprm1 because it is mainly located in the cytoplasm. TargetScan predicted numerous miRNAs, and we selected the top 5 putative miRNAs for further analysis. Interestingly, miR-155 was significantly increased under OGD exposure, suggesting that miR-155 may function in cerebral stroke (). Next, we used a dual-luciferase reporter assay to verify whether lncRNA Oprm1 could bind directly to miR-155. In the Oprm1-3′-UTR-WT transfection group, the relative luciferase activity of lncRNA Oprm1 was significantly decreased in the miR-155 mimics group compared with the mimics NC group, whereas the relative luciferase activity of lncRNA Oprm1 was increased after transfection with the miR-155 inhibitor (). No difference was detected in the Oprm1-3′-UTR-mut transfection group following transfection with miR-155 mimics or miR-155 inhibitor (). We examined the expression of miR-155 in the lncRNA Oprm1-overexpression group and miR-155-knockdown group and found that lncRNA Oprm1 expression was inversely associated with the expression of miR-155 (). In addition, miR-155 expression was increased with the progression of reperfusion after OGD exposure (). We also measured miR-155 expression in the ischemic penumbra and core tissues. We found that miR-155 expression was significantly increased in ischemic core tissue when reperfusion reached 6 h and was reduced at 12 and 24 h. However, there was no difference in miR-155 expression in penumbra tissue (). Next, rescue experiments were performed to identify the direct relationship between miR-155 and lncRNA Oprm1. Overexpression of miR-155 and lncRNA Oprm1 significantly increased the rate of cell death compared with the lncRNA Oprm1-overexpression group (). TUNEL staining revealed that the number of apoptosis-positive cells was clearly increased (). We also detected increased levels of cleaved-caspase-3, and co-transfection rescued the anti-apoptotic effect of lncRNA Oprm1 (). These results demonstrated that miR-155 is a direct target of lncRNA Oprm1.
Figure 4. miR-155 is potential target of lncRNA Oprm1. (A) miR-155 is potential target of lncRNA Oprm1. The expression of miR-155 was increased in Oprm1 group, wheras other predicted miRNA was not changed. (B) Luciferase activity was detected to evaluate the binding potential between miR-155 and Oprm1. (C) miR-155 was negatively regulated by Oprm1. (D) The expression of Oprm1 was manifestly increased with the development of reperfusion. (E) The expression of miR-155 was significantly increased in core tissues when reperfusion reached 6 h and reduced at 12 and 24 h. (F) Rescue experiment was performed to detect the regulatory relationship between miR-155 and Oprm1. Death rates were rescued by overexpression of miR-155. (G) Tunel-positive cells were increased in co-transfection group. (H) The cleaved-caspase 3 was rescued by overexpression of miR-155. **p < .01, ***p < .001.
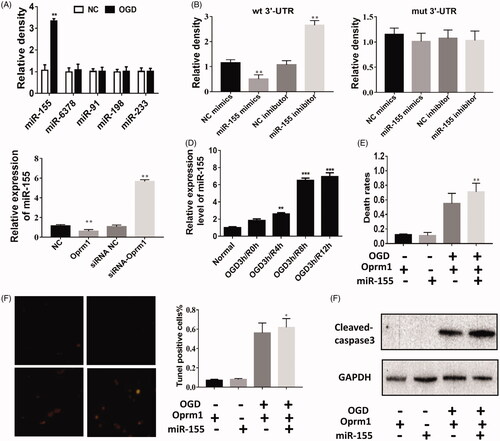
GATA3 is a target of miR-155
In a previous study, GATA3 was shown to regulate the expression of miR-155 as a target gene in prostate cancer [Citation25]; however, it is unknown whether GATA3 regulates miR-155 expression in cerebral ischemic injury (). The relative luciferase activity of GATA3 was significantly decreased in the miR-155 mimics group compared with the mimics NC groups (). No difference was detected in the GATA3-3′-UTR-mut group transfected with miR-155 mimics (). We examined GATA3 expression in the miR-155 mimics group and miR-155 inhibitor group via real-time PCR and found that GATA3 expression was inversely associated with the expression of miR-155 (). To further prove that GATA3 was the direct target of miR-155, we performed RNA-pulldown assay. As shown in and , miR-155 could directly interact with GATA3. Western blotting revealed similar results (). Next, rescue experiments were performed to identify the relationship between miR-155 and GATA3. Overexpression of miR-155 and GATA3 manifestly decreased the rate of cell death compared with the miR-155-overexpression group (). TUNEL staining revealed that the number of apoptosis-positive cells was clearly reduced (). We also detected increased levels of cleaved-caspase-3, and co-transfection rescued the pro-apoptotic effect of miR-155 (). These results demonstrated that GATA3 is a direct target of miR-155.
Figure 5. GATA3 is the target of miR-155. (A) The potential binding sequence was predicted using Target Scan Software. (B) Luciferase activity was detected to evaluate the binding potential between miR-155 and GATA3.(C) GATA3 was negatively regulated by miR-155 using qPCR. (D) GATA3 could directly interacted with miR-155. (E) lncRNA Oprm1 could combine with miR-155 and GATA3. (F) GATA3 was negatively regulated by miR-155 using western blotting. (G) Rescue experiment was performed to detect the regulatory relationship between miR-155 and GATA3. Death rates were rescued by overexpression of GATA3. (H) Tunel-positive cells were decreased in co-transfection group. (I) The cleaved-caspase 3 was rescued by overexpression of GATA3. **p < .01, ***p < .001.
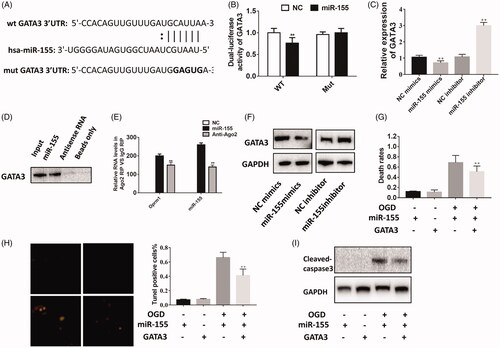
lncRNA Oprm1/miR-155/GATA3 axis in ischemic brain injury
Given that miR-155 regulated GATA3 expression in cerebral ischemic injury, we wondered whether lncRNA Oprm1 functioned by regulating the expression of miR-155 and GATA3. The overexpression of GATA3 and miR-155 partially rescued the protective effect of lncRNA Oprm1 (). The number of TUNEL-positive cells was slightly decreased in the co-transfection group compared with the miR-155 and lncRNA Oprm1 group (). Western blotting revealed similar results, that is, the level of cleaved-caspase-3 was increased compared with the lncRNA Oprm1 and miR-155 group. Taken together, these results confirmed that the lncRNA Oprm1/miR-155/GATA3 axis is important in ischemic brain injury and Oprm1 play a protective role in cerebral stroke by regulating the expression of miR-155 and GATA3.
Figure 6. Oprm1/miR-155/GATA3 axis in cerebral stroke. (A) Death rates were measured via trypan blue staining. (B) Tunel-postive cells were modestly decreased through rescue experiment. (C) The cleaved-capase3 was detected by western blotting. (D) p-p65 was decreased compared with control. (E) IkBα was decreased compared with control. *p < .05, **p < .01.
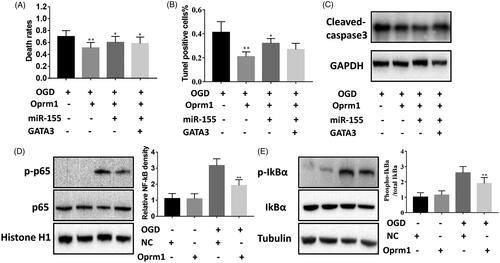
In previous studies, OGD was shown to activate the nuclear factor (NF)-κB-dependent signaling pathway [Citation26]. We analyzed the levels of p65, a major subunit of NF-κB, which was significantly increased in OGD cells compared with normal cultured cells and was inhibited in the lncRNA Oprm1 group (). Phosphorylation-dependent degradation of IκBα in the cytosol is required for the nuclear translocation of NF-κB. We found that lncRNA Oprm1 suppressed the level of phosphorylated IκBα under OGD conditions (). These data indicated that lncRNA Oprm1 may function by regulating the NF-κB signaling pathway.
Discussion
In a previous study, numerous differentially expressed lncRNAs were identified in cerebral I/R injury using RNA-Seq analysis [Citation23]. However, little is known about whether and how lncRNAs are involved in cerebral I/R injury. In the present study, we investigated the function of lncRNA Oprm1 and explored its possible mechanism in cerebral I/R injury. We found that lncRNA Oprm1 overexpression alleviated the apoptosis induced by OGD and the level of cleaved-caspase-3 was significantly reduced. Infarct size was distinctly decreased in the Oprm1-overexpression mouse group. The neurological score of the mice was also improved. We provided further evidence that the lncRNA Oprm1/miR-155/GATA3 axis plays an important role in cerebral I/R injury. Taken together, our findings show that lncRNA Oprm1 may serve as a potential target for novel therapeutic interventions in patients with cerebral I/R injury.
Dysregulated lncRNAs have been found to play important roles in biological and pathological processes [Citation27–29]. Previous studies have shown that the lncRNA N1LR exerts a neuroprotective effect against ischemic stroke by inhibiting p53 phosphorylation. The lncRNA SNHG12 inhibits miR-199a to upregulate SIRT1 and attenuates cerebral I/R injury by activating the AMPK signaling pathway [Citation30]. The lncRNA MEG3 functions as a competing endogenous RNA to regulate ischemic neuronal death by targeting the miR-21/PDCD4 signaling pathway [Citation11]. Our study proved that lncRNA Oprm1 was down-regulated in ischemic brain injury. We found that lncRNA Oprm1 had an anti-apoptotic function and protected neuronal cells from OGD injury. Moreover, animal experiments verified the function of lncRNA Oprm1 in vivo and showed that it could alleviate MCAO-induced injury. In addition, we proved that the lncRNA Oprm1/miR-155/GATA3 axis is important in ischemic brain injury, and lncRNA Oprm1 play a protective role in cerebral stroke as a competing endogenous RNA to regulate GATA3 expression by sponging miR-155. These findings revealed that lncRNA Oprm1 may be a novel target for the treatment of stroke.
This study found that miR-155 was increased with the development of reperfusion, which was negatively regulated by lncRNA Oprm1. In addition, we observed that miR-155 mimics blocked the protective effect of lncRNA Oprm1 in OGD-induced injury. Our study is the first to report that lncRNA Oprm1 regulated miR-155. Meanwhile, we also demonstrated that GATA3 regulated miR-155 in cerebral stroke. GATA3 overexpression partially rescued the effects of miR-155 and lncRNA Oprm1. Our study revealed that overexpressed lncRNA Oprm1 exerted its biological action in ischemic stroke by binding to miR-155.
In summary, we have studied the biological features and function of lncRNA Oprm1, which was decreased in ischemic brain injury, and provided a possible mechanism by which lncRNA Oprm1 exerts a protective effect by regulating miR-155 and GATA3 levels. We also proved that lncRNA Oprm1 may function by regulating the NF-κB signaling pathway. Thus, our study provides new insights into the cerebral stroke, demonstrating potential applications as a novel intervention target in clinical treatment. Understanding the precise role of lncRNA Oprm1 during cerebral I/R injury might ultimately be used to develop novel therapeutics for patients with cerebral stroke.
Disclosure statement
No potential conflict of interest was reported by the authors.
Additional information
Funding
References
- Wang Y, Li Z, Zhao X, et al. Effect of a multifaceted quality improvement intervention on hospital personnel adherence to performance measures in patients with acute ischemic stroke in China: a randomized clinical trial. JAMA. 2018;320:245–254.
- Vieira HL, Alves PM, Vercelli A. Modulation of neuronal stem cell differentiation by hypoxia and reactive oxygen species. Prog Neurobiol. 2011;93:444–455.
- Matsuda T, Zhai P, Sciarretta S, et al. NF2 activates hippo signaling and promotes ischemia/reperfusion injury in heart. Circ Res. 2016;119:596.
- Binet F, Mawambo G, Sitaras N, et al. Neuronal ER stress impedes myeloid-cell-induced vascular regeneration through IRE1α degradation of netrin-1. Cell Metab. 2013;17:353–371.
- Liesz A, Suri-Payer E, Veltkamp C, et al. Regulatory T cells are key cerebroprotective immunomodulators in acute experimental stroke. Nat Med. 2009;15:192–199.
- Perez-de-Puig I, Miró-Mur F, Ferrer-Ferrer M, et al. Neutrophil recruitment to the brain in mouse and human ischemic stroke. Acta Neuropathol. 2015;129:239–257.
- Ludewig P, Sedlacik J, Gelderblom M, et al. Carcinoembryonic antigen-related cell adhesion molecule 1 inhibits MMP-9-mediated blood-brain-barrier breakdown in a mouse model for ischemic stroke. Circ Res. 2013;113:1013–1022.
- Albert-Weißenberger C, Sirén AL, Kleinschnitz C. Ischemic stroke and traumatic brain injury: the role of the kallikrein-kinin system. Prog Neurobiol. 2013;101-102:65–82.
- Langhauser F, Gob E, Kraft P, et al. Kininogen deficiency protects from ischemic neurodegeneration in mice by reducing thrombosis, blood-brain barrier damage, and inflammation. Blood 2012;120:4082–4092.
- Ren W, Yang X. Pathophysiology of long non-coding RNAs in ischemic stroke. Front Mol Neurosci. 2018;11:96.
- Yan H, Rao J, Yuan J, et al. Long non-coding RNA MEG3 functions as a competing endogenous RNA to regulate ischemic neuronal death by targeting miR-21/PDCD4 signaling pathway. Cell Death Dis 2017;8:3211.
- Wei C, Wu M, Wang C, et al. Long noncoding RNA Lnc-SEMT modulates IGF2 expression by sponging miR-125b to promote sheep muscle development and growth. Cell Physiol Biochem. 2018;49:447–462.
- Cheng Z, Zhang Q, Yin A, et al. The long non-coding RNA uc.4 influences cell differentiation through the TGF-beta signaling pathway. Exp Mol Med. 2018;50:e447.
- Wang K, Long B, Zhou LY, et al. CARL lncRNA inhibits anoxia-induced mitochondrial fission and apoptosis in cardiomyocytes by impairing miR-539-dependent PHB2 downregulation. Nat Comm. 2014:5:3596.
- Chen J, Liu Y, Lu S, et al. The role and possible mechanism of lncRNA U90926 in modulating 3T3-L1 preadipocyte differentiation. Int J Obes. 2017;41:299–308.
- Zhang Y, Zhang D, Lv J, et al. miR-410-3p promotes prostatecancer progression via regulating PTEN/AKT/mTOR signaling pathway. Biochem Biophys Res Commun. 2018:503;2459–2465.
- Shen X, Bai H, Zhu H, et al. Long non-coding RNA MEG3 functions as a competing endogenous RNA to regulate HOXA11 expression by sponging miR-181a in multiple myeloma. Cell Physiol Biochem. 2018;49:87–100.
- Gu L, Lu L, Zhou D, et al. Long noncoding RNA BCYRN1 promotes the proliferation of colorectal cancer cells via up-regulating NPR3 expression. Cell Physiol Biochem. 2018;48:2337–2349.
- Huang Y, Zhou Y, Wong H-C, et al. Calmodulin Regulates Ca2+-sensing Receptor-mediated Ca2+ Signaling and Its Cell Surface Expression. J Biol Chem. 2010;285:35919.
- Zhang B, Arun G, Mao YS, et al. The lncRNA malat1 is dispensable for mouse development but its transcription plays a cis-regulatory role in the adult. Cell Rep. 2012;2:111–123.
- Shao Y, Ye M, Li Q, et al. LncRNA-RMRP promotes carcinogenesis by acting as a miR-206 sponge and is used as a novel biomarker for gastric cancer. Oncotarget 2016;7:37812–37824.
- Liu Z, Hui Y, Shi L, et al. Efficient CRISPR/Cas9-mediated versatile, predictable, and donor-free gene knockout in human pluripotent stem cells. Stem Cell Rep. 2016;7:496–507.
- Li H, Wu Y, Suo G, et al. Profiling neuron-autonomous lncRNA changes upon ischemia/reperfusion injury. Biochem Biophys Res Communic. 2017;495:104–109.
- Wu Z, Wu P, Zuo X, et al. LncRNA-N1LR enhances neuroprotection against ischemic stroke probably by inhibiting p53 phosphorylation. Mol Neurobiol. 2016;54:1–16.
- Li B, Jin X, Meng H, et al. Morin promotes prostate cancer cells chemosensitivity to paclitaxel through miR-155/GATA3 axis. Oncotarget. 2017;8:47849–47860.
- Wen Y, Yu Y, Fu X. LncRNA Gm4419 contributes to OGD/R injury of cerebral microglial cells via IκB phosphorylation and NF-κB activation. Biochem Biophys Res Comm. 2017;487:923.
- Xu Q, Deng F, Xing Z, et al. Long non-coding RNA C2dat1 regulates CaMKIIδ expression to promote neuronal survival through the NF-κB signaling pathway following cerebral ischemia. Cell Death Dis. 2016;7:e2173.
- Zhang T, Wu D-m, Deng S-h, et al. Integrated analysis reveals that long non-coding RNA TUBA4B can be used as a prognostic biomarker in various cancers. Cell Physiol Biochem. 2018;49:530–544.
- Feng W, Liang C, Wang C, et al. The dysregulated expression of KCNQ1OT1 and its interaction with downstream factors miR-145/CCNE2 in breast cancer cells. Cell Physiol Biochem. 2018;49:432–446.
- Yin WL, Yin WG, Huang BS, et al. LncRNA SNHG12 inhibits miR-199a to upregulate SIRT1 to attenuate cerebral ischemia/reperfusion injury through activating AMPK signaling pathway. Neurosci Lett. 2019:690;188–195.