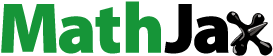
Abstract
Targeted delivery of chemotherapeutics by functionalized nanoparticles exhibits a wonderful prospect for cancer treatment. In this paper, selenium nanoparticles (SeNPs) was linked with hyaluronic acid (HA) to prepare tumor-targeted delivery vehicle HA-SeNPs, and HA-SeNPs was loaded with paclitaxel (PTX) to fabricate functionalized selenium nanoparticles HA-Se@PTX. HA-Se@PTX showed greater uptake in A549 cells in comparison with that in HUVEC, verifying HA-mediated specific uptake of HA-Se@PTX. HA-Se@PTX was capable of entering A549 cells via clathrin-associated endocytosis and showed faster drug release in cancer cell microenvironment in comparison with normal physiological environment. HA-Se@PTX could obviously inhibit the proliferation, migration and invasion of A549 cells and trigger A549 cells apoptosis. Moreover, active targeting functionalized selenium nanoparticles HA-Se@PTX showed greater in vivo antitumor activity compared with free PTX or passive targeting delivery system Se@PTX. In addition, HA-Se@PTX exhibited negligible toxicity on the major organs of mice. In a word, HA-Se@PTX may develop into a valuable nanoscale antitumor drug agent for lung cancer treatment.
Introduction
Non-small-cell-lung cancer (NSCLC) has become a serious health problem worldwide during the past decades [Citation1,Citation2]. It is reported that incidence and mortality ratio of NSCLC have been rising quickly and only 18% of the people who develop NSCLC can survive for over 5 years [Citation3,Citation4]. The main treatment strategy for NSCLC is chemotherapy [Citation5]. Paclitaxel (PTX) is one of the most common anticancer drugs in the clinic [Citation6]. Nevertheless, the application of PTX in the clinic is affected by its low-cellular uptake in cancer cells, which usually results in off-target side effects [Citation7]. Over the past decade, nanomedicine has been significantly developed in cancer therapy and a large number of nanoscale drug carriers were designed to deliver chemotherapeutic drugs for improving bioavailability and stability [Citation8–10]. Nevertheless, the delivery of chemotherapeutic drugs to the unintended sites may reduce the therapeutic efficacy and increase the risk of side effects [Citation11–13]. This shortcoming can be improved by using tumor-targeted drug delivery carriers [Citation14]. Hyaluronic acid (HA) is one kind of natural polysaccharides which have the structure of N-acetyl glucosamine and D-glucuronic acid. HA is reported to be a biodegradable, biologically active, and biocompatible material [Citation15]. Among the most attractive superiority of HA applied in the field of nanomaterials is its usage as an active tumor-targeted moiety, as HA receptor such as CD44 is abundantly present in cancer cells, including lung cancer A549 cells [Citation16]. Thus, HA can be used as tumor-targeted moiety by the specific bond between HA and CD44.
Selenium nanoparticles (SeNPs) have obtained a lot of attention in the field of anticancer drug carriers [Citation17,Citation18]. For one thing, selenium (Se) is a trace element and plays a key role in cancer prevention [Citation19,Citation20]. For another, Se aids in reducing drug toxicity, regulating the function of the thyroid gland and ensuringthe proper functioning of immune system, thus plays a major role in combating disease [Citation21]. These advantages make SeNPs superior to existing strategies including biogenetic (exosome, modularized extracellular vesicles, or extracellular vesicle-mimetic) and synthetic (inorganic nanoparticle and liposome). Therefore, SeNPs have got a lot of attention as potential chemotherapeutic carriers. However, the lack of active targeting capability is still an issue that needs to be solved [Citation22]. In order to acquire active targeting capability, many molecules (e.g. hyaluronic acid, folate, and RGD peptide) were linked to the surface of nanoparticles to function as tumor-targeted moieties [Citation23,Citation24].
In this paper, HA was linked with SeNPs to fabricate tumor-targeted delivery vehicle HA-SeNPs. Then HA-SeNPs nanoparticles were installed with PTX to prepare HA-Se@PTX. HA-Se@PTX exhibited significant cellular uptake and controlled release of PTX in A549 cells and could suppress the proliferation of A549 cells in vitro. HA-Se@PTX could also induce the apoptosis of A549 cells. Most importantly, HA-Se@PTX showed more favorable in vivo anticancer activity compared with Se@PTX or PTX, suggesting that HA-Se@PTX exhibits significant potential in non-small cell lung cancer treatment.
Materials and methods
Materials
Paclitaxel (PTX), sodium selenite (Na2SeO3), hyaluronic acid, vitamin C (Vc) and DAPI were purchased from Sigma (St. Louis, MO, USA). The antibodies were obtained from Cell Signaling Technology (Waltham, MA, USA). FBS and DMEM medium were purchased from Gibco (Paisley, United Kingdom).
Preparation and characterization of HA-Se@PTX nanoparticles
Selenium nanoparticles (SeNPs) was fabricated according to the previous literature [Citation25]. In brief, 4 ml vitamin C (Vc, 1 mM) solution and 0.5 ml Na2SeO3 (0.2 M) solution were mixed. The mixed solutions were gently stirred for 1 h at 25°C to manufacture SeNPs. After that, 1 ml hyaluronic acid was added to SeNPs solution. The mixtures were magnetically stirred for 5 h to prepare HA-SeNPs. Then 1.5 mg PTX was dissolved in a small amount of DMSO and dropped into HA-SeNPs solution. The mixed solutions were stirred for 6 h and dialyzed for 4 h to acquire pure HA-Se@PTX. Finally, the solutions were centrifuged at 12,000 rpm for 1.5 h and stored at 4°C. HA-Se@PTX containing coumarin-6 was fabricated by a similar method. The chemical characterization method was similar to the previous literature [Citation25].
A calibration curve against PTX was established to examine PTX-loading capability. The concentration of PTX was tested high-performance liquid chromatography (HPLC). The loading content and loading efficiency of PTX were calculated as follows:
The loading content and loading efficiency of PTX was found to be about 37 and 28%.
Cell culture
Non-small cell lung carcinoma A549 cells and human umbilical vein endothelial cells (HUVEC) were obtained from American Tissue Culture Collection (ATCC) and were cultivated in DMEM with 10% FBS in an incubator (80% humidity, Thermo Scientific, South Logan, USA) with 5% CO2 at 37°C.
Cellular uptake assay
About 0.8 ml A549 cell suspensions at the density of 2.5 × 104 cells/mL were added into 12-well plate and incubated at 37°C for 24 h.Afterward, A549 cells were co-cultured with 25 μg/mL HA-Se@PTX containing coumarin-6. The cells were processed as previously described [Citation11] and observed by a fluorescence microscope. The uptake of HA-Se@PTX in HUVEC was analyzed via a similar method. The quantitative uptake of SeNPs, HA-SeNPs, and HA-Se@PTX was tested by inductively coupled plasma-mass spectrometry. Briefly, A549 cells were incubated in 12-well plate for 18 h. After that, SeNPs, HA-SeNPs, and HA-Se@PTX nanoparticles at equivalent selenium concentration of 12 μg/mL were replaced and incubated in the well for different periods of time. To clearly reveal HA-mediated uptake by A549 cells, a control experiment was performed. Briefly, A549 cells were pretreated with free HA for 4 h. Subsequently, the cells were rinsed with cold PBS and co-cultured with HA-Se@PTX in fresh medium for 8 h. Finally, the cells were lysed and used for inductively coupled plasma-mass spectrometry analysis. Various uptake inhibitors were used to study the uptake mechanism of HA-Se@PTX in A549 cells. The cells were processed as previously reported [Citation13] and analyzed by flow cytometer (Becton, Dickinson & Company, BD FACSAria II, San Jose, CA, USA).
PTX release from nanoparticles
The release profile of PTX from nanoparticles at different pH values was examined. Briefly, 2 mg HA-Se@PTX nanoparticles were dissolved into 5 ml PBS and then transferred to a pre-swelled dialysis bag (cutoff size 3.5 kDa). The dialysis bag was dipped into 50 ml PBS at pH 5.4/6.8/7.4 at 25 °C. Samples were withdrawn from PBS at an indicated time interval, then equal volume fresh PBS was supplemented for the loss. PTX concentrations in the released samples were analyzed by HPLC method.
MTT assay
MTT assay was performed to examine the toxicity of nanoparticles. Briefly, A549 cells were incubated in 96-well culture plate for 24 h and then exposed to PTX or Se@PTX or HA-Se@PTX (various equivalent PTX concentration) or HA-SeNPs for 48 h, respectively. Then, the cells were processed as previously reported [Citation26].
In vitro wound healing analysis
Cell migration was determined by wound healing assay. Briefly, A549 cells were seeded in 24-well plate with each well containing 5 × 104 cells and incubated overnight to reach full confluence. The cells were scratched with a 10 µL tip and the residual cells were rinsed by PBS. Then, each well was added with DMEM containing 3% FBS. Then, A549 cells were incubated with PTX, Se@PTX, and HA-Se@PTX at PTX concentration of 4 µg/mL for 12 h, respectively. Then, scratched monolayer cells were photographed (Leica DMi8 digital microscope, Wetzlar, Germany ) at 0 h and after successive incubation for 12 h, respectively. The migration rate over 12 h period was calculated by using the following formula. Cell motilities (%) = (the average distance of wound at 0 h – the average distance of wound at 12 h/the average distance of wound at 0 h) ×100%. Experiments were conducted in triplicate.
In vitro transwell assay
The invasion assays were carried out using the transwell chamber (Corning, No. 3422, 8 μm). A549 cells were serum-starved for 24 h and were collected in serum-free medium. The cells then were added to the upper chamber and the complete medium was added to the bottom chamber. Then, A549 cells were incubated with PTX, Se@PTX and HA-Se@PTX at the equivalent PTX concentration of 4 µg/mL for 24 h, respectively. After 24 h, uninvaded cells on the upper filter were gently wiped. The invasive cells on the lower membrane surface were fixed with methanol for 10 min and stained with 0.1% crystal violet for 5 min. The invading cells in five independent views were counted and photographed using a microscope. Experiment was conducted in triplicate.
Flow cytometry assay
The cell cycle distribution was analyzed by flow cytometry (Becton, Dickinson & Company, BD FACSAria II). In brief, A549 cells were incubated with HA-Se@PTX, Se@PTX and PTX at PTX concentration of 4 µg/mL for 24 h, respectively and then washed with cold PBS. Finally, the cells were processed as previously reported [Citation17]. Flow cytometry was also used to analyze the effect of drugs on A549 cells apoptosis and the cells were processed as mentioned above.
Western blot assay
The protein level was examined via western blotting. A549 cells were seeded in the 6-well plate and incubated overnight. The cells were co-incubated with HA-Se@PTX at various equivalent PTX dose for 24 h. The cells were collected for further analysis according to the previous paper [Citation27].
Xenograft mouse model
Seven weeks old BALB/c nude mice were fed in SFP-grade animal center (day-night cycle) and used to investigate the antitumor activity of HA-Se@PTX in vivo. A549 cells (5 × 106 cells) were injected in the abdomen of the mice subcutaneously. When tumor volumes grew to 100 mm3, mice were categorized into four groups with six mice per group randomly. Then saline, PTX, Se@PTX and HA-Se@PTX (at the equivalent PTX of 1 mg/kg) were injected to tumor-bearing mice intravenously via tail vein once every other day, respectively. Tumor volume was calculated with the equation as follows: Tumor volume (mm3) = × length × width2.
Hematoxylin and eosin (H&E) staining was used for histologic analysis. The sections were observed under a microscope (Leica DMi8). All animal experiments were approved by the Ethics Committee of Guangzhou Medical University.
Statistical analysis
The data were expressed as mean ± standard deviations (SD). Statistical differences were analyzed via one-way analysis of variance (ANOVA). Differences with *p < .05 and **p < .01 were considered statistically significant.
Results and discussion
Synthesis and characterization of HA-Se@PTX
One novel tumor-targeted nanoparticles HA-Se@PTX was synthesized in this paper. Selenium nanoparticles (SeNPs) were linked with tumor-targeted molecular hyaluronic acid (HA) to prepare tumor-targeted carrier HA-SeNPs, then paclitaxel (PTX, one of the most common antitumor drugs) was loaded to the surfaces of HA-SeNPs to fabricate tumor-targeted functionalized nanoparticles HA-Se@PTX. Particle size, morphology and chemical structure of nanoparticles were characterized by various chemical methods. showed that HA-Se@PTX nanoparticles had an average size of 85 nm. Morphology of HA-Se@PTX nanoparticles was characterized in TEM image, which showed that HA-Se@PTX exhibited spherical particles with diameter from 60 nm to 90 nm (). Elemental analysis showed that an obvious signal of Se atom from the SeNPs and carbon and oxygen atom signal from PTX appeared in the spectrum of HA-Se@PTX (), suggesting that PTX was successfully loaded to the surfaces of SeNPs. Sizes distribution of HA-Se@PTX nanoparticles in water solution () and phosphate buffered solution (PBS) (Figure S1) indicated that HA-Se@PTX were stable with small size (<140 nm) during 16-day observation. This data showed that HA-Se@PTX had favourable stability in water solution and PBS. X-ray photoelectron spectroscopy (XPS) was used to verify the linking between PTX and HA-SeNPs (Figure S2). After loading PTX to HA-SeNPs, Se 3d, N 1S and O 1S peaks of HA-SeNPs were shifted from 50 eV, 400 eV and 532 eV (HA-SeNPs) to 52 eV, 401 eV and 533 eV (HA-Se@PTX), respectively. The chemical shift of Se 3d, N 1S and O 1S peaks in HA-SeNPs indicated that PTX was bond with the surface of HA-SeNPs via interaction of Se–N and Se–O. The zeta potentials of HA-Se@PTX, HA-SeNPs and SeNPs were presented in Figure S3. After loading with HA, zeta potential of SeNPs was slightly decreased from −20.3 mV to −22.6 mV, then increased to −16.3 mV after loading with PTX. The zeta potential of HA-SeNPs was increased from −22.6 mV to −16.3 mV after loading with PTX, indicating PTX exhibited slight positive charge. This result showed that electrostatic attraction also existed between PTX and HA-SeNPs. Thus, PTX was loaded to the surface of HA-SeNPs through chemical bonding (Se–N and Se–O) and electrostatic attraction.
Selective uptake of HA-Se@PTX nanoparticles
Drug delivery efficacy significantly depends on the uptake of drugs in cells [Citation28]. Effective uptake of drugs is a very crucial issue for favorable treatment outcoming. A lot of researches showed that the expression of hyaluronic acid receptor CD44 in cancer cells is much more than that in human normal cells [Citation29]. Therefore, the expression of CD44 in A549 cells should be examined to verify whether hyaluronic acid receptor CD44 contributes to the uptake of HA-Se@PTX in A549 cells. Seen from Figure S4, the expression level of CD44 was evidently higher in lung cancer A549 cells in comparison with human umbilical vein endothelial cells (HUVEC), suggesting that HA-Se@PTX has a superior guided selectivity for A549 cells to HUVEC. The selective HA-mediated uptake of HA-Se@PTX between A549 cells and HUVEC was analyzed via a fluorescence microscope. showed that the cellular uptake of HA-Se@PTX containing coumarin-6 in A549 cells was stronger than that in HUVEC at the same condition, verifying HA-mediated specific uptake. Quantitative cellular uptake of SeNPs, HA-SeNPs and HA-Se@PTX in A549 cells was conducted by ICP-MS. As shown clearly in , intracellular selenium concentrations in three groups were increased in one time-dependent way and the uptake ability of HA-SeNPs and HA-Se@PTX groups were stronger compared with that in SeNPs group. Higher uptake of HA-SeNPs and HA-Se@PTX in A549 cells further verified that HA-mediated targeting played a significant role in the uptakes of nanoparticles in cancer cells. In order to further verify whether HA-mediated uptake occurred in A549 cells, a control experiment was carried out. Free HA was added to A549 cells first to block the interaction between HA and CD 44, then HA-Se@PTX was added to the medium to study whether less HA-Se@PTX were taken by cells. As shown in , uptake of HA-Se@PTX in A549 cells without pretreatment with free HA is significantly greater in comparison with pretreated cells, suggesting HA-mediated targeting contributed to the enhanced uptake of HA-Se@PTX in A549 cells.
Figure 2. Cellular uptake of HA-Se@PTX in A549 cells (A) and HUVEC (B) was observed by fluorescence microscope, respectively. Scale bar is 20 μm. (C) Quantitative uptake of SeNPs, HA-SeNPs and HA-Se@PTX in A549 cells was analyzed by ICP-MS. (D) The uptake of HA-Se@PTX in A549 cells untreated/pretreated with HA were tested by ICP-MS.
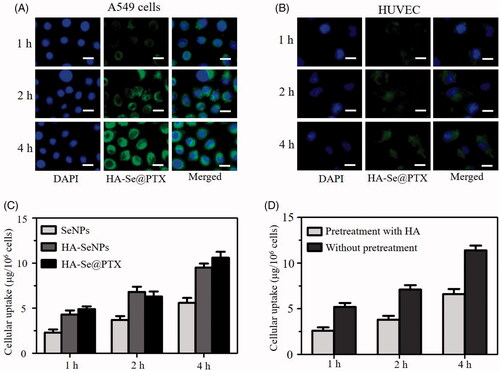
Endocytosis is an important transport mechanism for nanoparticles across the membrane [Citation30]. An uptake inhibition assay was performed to investigate the uptake pathways of HA-Se@PTX. Pretreatment with NaN3/DOG or incubation at 4 °C obviously decreased the uptake of HA-Se@PTX in A549 cells (), suggesting that the endocytosis of HA-Se@PTX is an energy-dependent process. Various endocytosis inhibitor was used to study the endocytosis mechanism of HA-Se@PTX. Chlorpromazine, amiloride and nystatin were the inhibitors of clathrin-associated endocytosis, macropinocytosis and caveolae-mediated endocytosis, respectively. As shown in , after pretreatment with nystatin or amiloride, uptake of HA-Se@PTX was reduced by 28.1 and 35.2%, respectively. However, chlorpromazine-pretreatment resulted in 55.7% reduction in the uptake of HA-Se@PTX, indicating that HA-Se@PTX entered cancer cells mainly by clathrin-associated endocytosis way.
In vitro release of PTX
As we know, the pH range in the tumor is from pH 6.5–7.2 in the extracellular medium to pH 4.5–6.0 in the lysosome/endosome. Thus, three types of pH values (pH 7.4, 6.8 and 5.4) were used to simulate normal physiological environment and cancer cell microenvironment, respectively. The release profiles of PTX from HA-Se@PTX nanoparticles were shown in , there was remarkable burst drug release in three pH values during the initial 4 h. Interestingly, HA-Se@PTX showed the fastest release of PTX in pH 5.4 during the initial 30 h and the release rate was about 84.2%. Nevertheless, the release rates were 45.7 and 59.4% in pH 7.4 and 6.8, respectively. The result showed that HA-Se@PTX exhibited a pH-dependent release of PTX in the pH range of 5.4–7.4. The faster release of PTX in acidic condition might be due to the increasing protonation of HA-SeNPs in acidic condition, which weakened electrostatic attraction between PTX and HA-SeNPs, thus acidic condition facilitated the release of PTX from HA-SeNPs. Such acid-dependent drug release features of HA-Se@PTX is quite favorable for cancer treatment.
HA-Se@PTX inhibits the proliferation of A549 cells
MTT assay was carried out to investigate whether HA-Se@PTX could inhibit the proliferation of A549 cells in vitro. Se@PTX (passive targeting nanoparticle) and PTX were set as a negative control. indicated that various formulations of PTX could obviously inhibit the proliferation of A549 cells. PTX, Se@PTX and HA-Se@PTX at 4 μg/mL PTX dose dramatically inhibited the proliferation of A549 cells and the cell viability rates were 64.8, 51.4 and 34.5%, respectively. The result indicated that HA-Se@PTX showed stronger proliferation inhibition activity on A549 cells compared with Se@PTX or PTX, which was possibly due to the higher cellular uptake ability of HA-Se@PTX. The above data showed that delivery of PTX by HA-SeNPs could significantly strengthen the anti-cancer ability of PTX. A549 cells exposed to HA-Se@PTX at the equivalent PTX dose of 4 μg/mL exhibited less than 50% viability, therefore such dose was applied forthe next study. Besides, HA-SeNPs at the used dose showed low cytotoxicity ().
Effect of HA-Se@PTX on the migration and invasion of A549 cells
Effective inhibition of tumor migration and invasion is considered as a promising way of delaying tumor growth. Wound healing and transwell assays were adopted to estimate whether HA-Se@PTX had the capability to inhibit the migration and invasion of A549 cells. Seen from , HA-Se@PTX obviously inhibited the migration and invasion of A549 cells. Moreover, HA-Se@PTX showed greater ability to suppress the migration and invasion of A549 cells in comparison with PTX or Se@PTX (). The anti-metastatic or anti-invasive mechanism of HA-Se@PTX needs further investigation.
Figure 5. (A) The wound width of A549 cells was photographed after 12 h of co-incubation with PTX, Se@PTX and HA-Se@PTX, respectively. Scale bar is 400 µm. (B) The quantitative analysis of cell motility in control, PTX, Se@PTX and HA-Se@PTX groups. (C) The effect of PTX, Se@PTX, and HA-Se@PTX on the migration of A549 cells. Scale bar is 200 µm. (D) The quantitative analysis of cell migration inhibition rate in PTX, Se@PTX, and HA-Se@PTX groups. *p < .05 vs. PTX group.
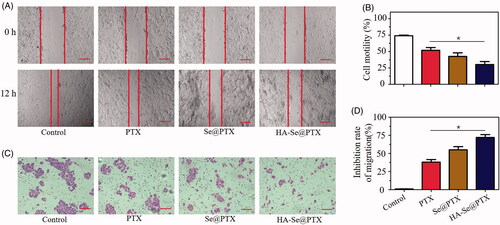
HA-Se@PTX trigger A549 cell apoptosis
PTX is one of the most effective chemotherapeutic agents with extensive activity against cancers in humans and it can induce the apoptosis of cancer cells [Citation31]. Flow cytometry was adopted to verify whether HA-Se@PTX could trigger A549 cells apoptosis. As shown in , Sub-G1 peak reflected the apoptosis cells. The result indicated that Sub-G1 peak in HA-Se@PTX group was more obvious (33.05%) than that in PTX group (10.67%) and Se@PTX group (20.75%), suggesting that HA-Se@PTX presented greater ability to trigger A549 cells apoptosis. Nevertheless, no obvious difference occurred in cell cycle distribution among different treatment groups.
Figure 6. (A) Effect of PTX, Se@PTX, and HA-Se@PTX on the cell cycle distribution and apoptosis in A549 cells, respectively. (B) Apoptosis percentage analysis of A549 cells after exposed to PTX, Se@PTX, and HA-Se@PTX, respectively.
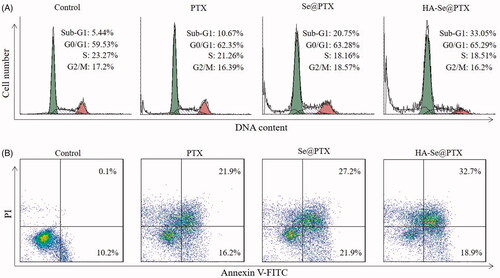
Flow cytometry was adopted to analyze the apoptosis of A549 cells by Annexin V-FITC/PI dual staining. As shown in , HA-Se@PTX could significantly induce A549 cells apoptosis and led to higher apoptosis rates (51.6%) compared with PTX (38.1%) or Se@PTX (49.1%) groups. The above data showed that HA-Se@PTX could strengthen the anticancer efficacy of PTX to trigger the apoptosis of A549 cells by loading PTX onto HA-SeNPs.
Western blotting analysis
MMP2 and MMP9 are considered as a key mediator of cell proliferation and migration [Citation32]. To explore whether HA-Se@PTX affected the migration of A549 cells through inhibiting the expression of MMP-9 and MMP-2 proteins, the protein level of MMP-9 and MMP-2 in A549 cells were analyzed. As shown in , the expression of MMP-2 and MMP-9 proteins were not obviously inhibited after A549 cells were exposed to different concentrations of HA-Se@PTX for 24 h. This result indicated that migration inhibition of A549 cells was not due to the effect of HA-Se@PTX on MMP-2 and MMP-9 proteins. The migration of A549 cells was slightly inhibited probably by decreasing the viability of A549 cells induced by HA-Se@PTX. Caspase-3 as a major executioner of cell apoptosis is responsible for the cleavage of various kinds of proteins [Citation33]. PARP is located in downstream of caspase family proteins and functioned as an important cleavage target of caspase-3 [Citation34]. In this study, protein expression of cleavage-caspase-3 and cleavage-PARP were examined via Western blot assay to estimate their contributions to cell apoptosis. As shown in , HA-Se@PTX-treatment led to the expression up-regulation of cleavage-caspase-3 and cleavage-PARP in comparison with the control group. This result showed that HA-Se@PTX might activate caspase-3-related apoptosis pathway.
In vivo anti-tumor efficacy
A549 tumor xenografts were adopted to confirm the antitumor potential of HA-Se@PTX in vivo. As shown in , tumor volume of saline group rapidly increased during treatment time. However, HA-Se@PTX-treatment group exhibited much higher growth delay compared with the saline control group, confirming the favorable antitumor effect of HA-Se@PTX. In addition, HA-Se@PTX exhibited an evident higher tumor inhibitory efficacy than other groups. As shown in , tumor weight of HA-Se@PTX-treated group further showed the good antitumor efficacy of HA-Se@PTX. In vivo antitumor efficacy results indicated that HA-Se@PTX had significant potential for lung carcinoma treatment.
In vivo toxicity assessment
To analyze the potential toxicity of HA-Se@PTX in vivo, heart, liver, spleen, lung, and kidneys of mice were harvested during the experiment and studied via H&E staining. As shown in Figure S5, no apparent abnormality in any of the five organs was observed in HA-Se@PTX-treatment group compared with the saline-treatment group. This result confirmed that HA-Se@PTX had good biocompatibility in vivo. Therefore, HA-Se@PTX showed the potential to be a promising tumor-targeted delivery system for non-small cell lung cancer therapy.
Conclusion
In the current research, a tumor-targeted nanoparticles HA-Se@PTX was prepared to deliver PTX to A549 cells for non-small cell lung carcinoma treatment. HA-Se@PTX exhibited excellent cellular uptake in A549 cells and showedthe faster release of PTX from nanoparticles in pH 5.4 which simulated acidic microenvironment of cancer cells. HA-Se@PTX exhibited high activity to inhibit the proliferation, migration and invasion of A549 cells and trigger A549 cells apoptosis. Furthermore, compared with free PTX and Se@PTX, HA-Se@PTX showed greater antitumor activity in vivo. The further anticancer mechanism of HA-Se@PTX should be explored in the following research. In a word, HA-Se@PTX holds significant potential for non-small cell lung carcinoma treatment.
Supplemental Material
Download ()Supplemental Material
Download ()Supplemental Material
Download ()Supplemental Material
Download ()Supplemental Material
Download ()Supplemental Material
Download ()Disclosure statement
The authors declare that they have no conflict of interest regarding this publication.
Additional information
Funding
References
- Chittasupho C, Athikomkulchai S. Nanoparticles of Combretum quadrangulare leaf extract induce cytotoxicity, apoptosis, cell cycle arrest and anti-migration in lung cancer cells. J Drug Deliv Sci Tec. 2018;45:378–387.
- Munaweera I, Shi Y, Koneru B, et al. Nitric oxide- and cisplatin-releasing silica nanoparticles for use against non-small cell lung cancer. J Inorg Biochem. 2015;153:23–31.
- Alibolandi M, Ramezani M, Abnous K, et al. In vitro and in vivo evaluation of therapy targeting epithelial-cell adhesion-molecule aptamers for non-small cell lung cancer. J Control Release. 2015;209:88–100.
- Kumar N, Salar RK, Prasad M, et al. Synthesis, characterization and anticancer activity of vincristine loaded folic acid-chitosan conjugated nanoparticles on NCI-H460 non-small cell lung cancer cell line. Egypt J Basic Appl Sci. 2018;5:87–99.
- Zhao Q-s, Hu L-l, Wang Z-d, et al. Resveratrol-loaded folic acid-grafted dextran stearate submicron particles exhibits enhanced antitumor efficacy in non-small cell lung cancers. Mat Sci Eng C-Mater. 2017;72:185–191.
- Wen ZM, Jie J, Zhang Y, et al. A self-assembled polyjuglanin nanoparticle loaded with doxorubicin and anti-Kras siRNA for attenuating multidrug resistance in human lung cancer. Biochem Bioph Res Co. 2017;493:1430–1437.
- Ibrahim HG, Attia N, Hashem F, et al. Cerium oxide nanoparticles: In pursuit of liver protection against doxorubicin-induced injury in rats. Biomed Pharm. 2018;103:773–781.
- Sun H, Liu Y, Bai X, et al. Induction of oxidative stress and sensitization of cancer cells to paclitaxel by gold nanoparticles with different charge densities and hydrophobicities. J Mater Chem B. 2018;6:1633–1639.
- Li M, Tang Z, Zhang Y, et al. LHRH-peptide conjugated dextran nanoparticles for targeted delivery of cisplatin to breast cancer. J Mater Chem B. 2014;2:3490–3499.
- Wang W, Chen T, Xu H, et al. Curcumin-loaded solid lipid nanoparticles enhanced anticancer efficiency in breast cancer. Molecules 2018;23:1578.
- Xia Y, Lin Z, Li Y, et al. Targeted delivery of siRNA using RGDfC-conjugated functionalized selenium nanoparticles for anticancer therapy. J Mater Chem B. 2017;5:6941–6952.
- Brown AL, Winter H, Goforth AM, et al. Facile synthesis of ligand-free iridium nanoparticles and their in vitro biocompatibility. Nanoscale Res Lett. 2018;13:208.
- Li Y, Guo M, Lin Z, et al. Polyethylenimine-functionalized silver nanoparticle-based co-delivery of paclitaxel to induce A549 cell apoptosis. Ijn. 2016;11:6693–6702.
- Yu B, Tai HC, Xue W, et al. Receptor-targeted nanocarriers for therapeutic delivery to cancer. Mol Membr Biol. 2010;27:286–298.
- Pedrosa SS, Pereira P, Correia A, et al. Targetability of hyaluronic acid nanogel to cancer cells: in vitro and in vivo studies. Eur J Pharm Sci. 2017;104:102–113.
- Lin T, Yuan A, Zhao X, et al. Self-assembled tumor-targeting hyaluronic acid nanoparticles for photothermal ablation in orthotopic bladder cancer. Acta Biomater. 2017;53:427–438.
- Xia Y, You P, Xu F, et al. Novel functionalized selenium nanoparticles for enhanced anti-hepatocarcinoma activity in vitro. Nanoscale Res Lett. 2015;10:1051.
- Zheng W, Yin T, Chen Q, et al. Co-delivery of Se nanoparticles and pooled SiRNAs for overcoming drug resistance mediated by P-glycoprotein and class III beta-tubulin in drug-resistant breast cancers. Acta Biomater. 2016;31:197–210.
- Sun D, Liu Y, Yu Q, et al. Inhibition of tumor growth and vasculature and fluorescence imaging using functionalized ruthenium-thiol protected selenium nanoparticles. Biomaterials. 2014;35:1572–1583.
- Li Y, Lin Z, Guo M, et al. Inhibition of H1N1 influenza virus-induced apoptosis by functionalized selenium nanoparticles with amantadine through ROS-mediated AKT signaling pathways. IJN. 2018;13:2005–2016.
- Zhou Y, Yu Q, Qin X, et al. Improving the anticancer efficacy of laminin receptor-specific therapeutic ruthenium nanoparticles (RuBB-loaded EGCG-RuNPs) via ROS-dependent apoptosis in SMMC-7721 cells. ACS Appl Mater Interfaces. 2016;8:15000–15012.
- Xia Y, Wang C, Xu T, et al. Targeted delivery of HES5-siRNA with novel polypeptide-modified nanoparticles for hepatocellular carcinoma therapy. RSC Adv. 2018;8:1917–1926.
- Yang C, Wang X, Yao X, et al. Hyaluronic acid nanogels with enzyme-sensitive cross-linking group for drug delivery. J Control Release. 2015;205:206–217.
- Xia Y, Guo M, Xu T, et al. siRNA-loaded selenium nanoparticle modified with hyaluronic acid for enhanced hepatocellular carcinoma therapy. IJN. 2018;13:1539–1552.
- Sun D, Liu Y, Yu Q, et al. The effects of luminescent ruthenium(II) polypyridyl functionalized selenium nanoparticles on bFGF-induced angiogenesis and AKT/ERK signaling. Biomaterials. 2013;34:171–180.
- Xia Y, Chen Q, Qin X, et al. Studies of ruthenium(ii)-2,2′-bisimidazole complexes on binding to G-quadruplex DNA and inducing apoptosis in HeLa cells. New J Chem. 2013;37:3706–3715.
- Yin T, Xie W, Sun J, et al. Penetratin peptide-functionalized gold nanostars: enhanced BBB permeability and NIR photothermal treatment of Alzheimer's disease using ultralow irradiance. ACS Appl Mater Interfaces. 2016;8:19291–19302.
- Jeong JY, Hong EH, Lee SY, et al. Boronic acid-tethered amphiphilic hyaluronic acid derivative-based nanoassemblies for tumor targeting and penetration. Acta Biomater. 2017;53:414–426.
- Lin JT, Du JK, Yang YQ, et al. pH and redox dual stimulate-responsive nanocarriers based on hyaluronic acid coated mesoporous silica for targeted drug delivery. Mat Sci Eng C-Mater. 2017;81:478–484.
- Xia Y, Xu T, Wang C, et al. Novel functionalized nanoparticles for tumortargeting co-delivery of doxorubicin and siRNA to enhance cancer therapy. IJN. 2018;13:143–159.
- Kozlu S, Sahin A, Ultav G, et al. Development and in vitro evaluation of doxorubicin and celecoxib co-loaded bone targeted nanoparticles. J Drug Deliv Sci Tec. 2018;45:213–219.
- Kalhori V, Tornquist K. MMP2 and MMP9 participate in S1P-induced invasion of follicular ML-1 thyroid cancer cells. Mol Cell Endocrinol. 2015;404:113–122.
- Kong L, Yuan Q, Zhu H, et al. The suppression of prostate LNCaP cancer cells growth by selenium nanoparticles through Akt/Mdm2/AR controlled apoptosis. Biomaterials. 2011;32:6515–6522.
- Laha D, Pramanik A, Maity J, et al. Interplay between autophagy and apoptosis mediated by copper oxide nanoparticles in human breast cancer cells MCF7. Biochim Biophys Acta. 2014;1840:1–9.