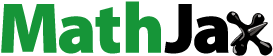
Abstract
The repair of periodontal bone tissue defects in patients with periodontitis is one of the major challenges for dentists. Stem cell-based bone regeneration has been considered as a promising strategy to restore the lost periodontal bone tissue. However, the local inflammatory environment of periodontal tissue affects stem cell-based periodontal bone regeneration. Toll-like receptor 2 (TLR2), a member of the TLR family, plays an important role in regulating immunoreaction. Previous studies have shown that the activation of TLR2 signaling pathway is involved in enhancing tissue vascularization and wound healing. However, the mechanisms underlying the therapeutic effects of TLR2 on regulating bone marrow stromal cells (BMSCs) mediated periodontal bone tissue regeneration still need to be further investigated. In this study, we tested the effect of TLR2 on regulating BMSCs mediated alveolar bone regeneration by establishing a TLR2 gene-modified canine BMSCs using a lentivirus. Activation of TLR2 significantly enhanced the expression of hypoxia-inducible factor-1α (HIF-1α) and bone morphogenetic protein 2 (BMP-2) and then upregulated the expression of their downstream osteogenic and angiogenic related gene in BMSCs. TLR2-BMSCs mediated bone regeneration in canine tooth extraction sockets under an inflammatory environment demonstrated that activation of the TLR2 signaling pathway significantly stimulated BMSCs meditated angiogenesis and osteogenesis.
Introduction
Inflammatory is one of the most common reasons causing the bone defect, especially in oral periodontium [Citation1]. Periodontitis is a chronic infective disease and can lead to the resorption of alveolar bone and tooth losing eventually [Citation2]. In addition, more and more evidence indicates that the osseointegration of dental implants can also be destroyed by peri-implant inflammatory (termed as peri-implantitis), which is also the major reason leading to implant failure [Citation3]. Both of the periodontitis and peri-implantitis are common diseases and frequently-occurring diseases. Due to the progressive bone resorption, many patients could suffer a serious consequence of losing their masticatory function. Therefore, the treatment of these patients’ bone defect is still an arduous task. Considering the limited source of autologous bone, Bio-oss and other artificial materials are often applied in the clinic to treat these bone defects. However, low osteogenic property prolongs the therapy time and decreases the success ratio of bone regeneration. The inflammatory microenvironment also greatly increases the difficulty of regeneration. To address these drawbacks, we provide a novel method of using toll-like receptor 2 (TLR2) modified BMSCs to adapt the inflammatory microenvironment and acquire better-vascularized bone regeneration.
Stem cells play an important role in promoting tissue regeneration. Different types of stem cells have been applied to construct bone tissue engineering complex for the treatment of bone defects. Bone marrow stem cells (BMSCs) and adipose tissue-derived stem cells (ADSCs) are two common sources of stem cells for bone regeneration and well-studied. For the repairing of bone defects in the oral maxillofacial region, BMSCs displayed faster and better capacity in enhancing bone regeneration when compared with ADSCs [Citation4,Citation5]. Moreover, BMSCs has been approved to be safe and accelerate bone regeneration for the treatment of alveolar bone defects, according to a randomized, controlled feasibility trial [Citation6]. However, bone tissue tends to absorb under the inflammatory environment, so the osteogenic capacity of BMSCs should be further enhanced to increase the total bone mass. Toll-like receptors (TLRs) are a class of proteins that work in the innate immune system [Citation7]. Like one of the TLRs, TLR2 protein is encoded by the TLR2 gene. TLR2 plays a fundamental role in pathogen recognition and can recognize many bacterial, viral, and certain endogenous substances [Citation8–10]. The activation of TLR2 was also reported to enhance tissue vascularization and wound healing [Citation11]. More importantly, both of ADSCs and BMSCs express various toll-like receptors, including TLR1, TLR2, TLR3, TLR4, TLR5, and TLR6 [Citation12,Citation13]. Also, the osteogenic differentiation of human ADSCs can be enhanced by lipopolysaccharide (LPS) and peptidoglycan (PGN) via TLRs [Citation14]. As we know, the natural agonist of TLR2 is peptidoglycan (PGN), which is the main component of the bacterial cell wall [Citation15]. We speculate that the activation of TLR2 might directly involve in the osteogenic differentiation of ADSCs [Citation14]. However, whether the expression of TLR2 in BMSCs also plays the same role still needs to be approved.
In this study, BMSCs were modified with TLR2 gene via lentivirus to enhance their angiogenic and osteogenic capacity in the inflammatory environment. In vitro, TLR2 modified cells were stimulated by PGN and the expression of angiogenesis and osteogenesis related makers were detected at both gene and protein levels. Then, the cells together with PGN were subcutaneously implanted in nude mice, vascularization and osteogenic differentiation of those implanted cells were measured by immunofluorescence method. Lastly, the TLR2 modified BMSCs were delivered by collagen gel and then were injected into canine tooth extraction sockets and new bone regeneration was detected after 2 weeks. The use of TLR modification will make use of the inflammatory microenvironment to acquire better bone regeneration.
Materials and methods
Isolation and culture of canine BMSCs
Canine BMSCs were isolated and cultured as we previously described [Citation16]. Briefly, the canine was anesthetized with ketamine (10 mg/kg) and xylazine (4 mg/kg) and then about 4 ml of bone marrow were harvested via needle aspiration from the iliac crest. The bone marrow was mixed with 10 ml phosphate buffered saline (PBS), following centrifuged at 1000 rpm for 15 min. The precipitated cells were resuspended in Dulbecco’s modified Eagle’s medium (DMEM; Gibco, New York, USA) containing 10% fetal bovine serum (FBS; Gibco, USA) and cultured at 37 °C in 5% CO2. The non-adherent cells were rinsed away after 5 days and fresh medium was added. The culture medium was then changed every 2–3 days. Cells at passage 2–4 were used in this study.
Isolated canine BMSCs were identified via the cell surface antigens CD29, CD34, CD44 and CD45 and CD105 using a flow cytometry assay as described previously [Citation4]. Briefly, cells were fixed in 4% phosphate-buffered paraformaldehyde and incubated with CD29-PE (Biolegend, San Diego, CA, USA), CD34-PE (Becton, Dickinson and Company, New Jersey, USA), CD44-PE (Becton Dickinson and Company, USA) CD45-PE (Biolegend, USA) or CD105-PE (eBioscience, California, USA) antibodies for 30 min. Then, the cells were washed twice with PBS and analyzed using a FACSCalibur flow cytometer (Becton Dickinson).
Multiple lineage differentiation
For adipogenic differentiation, canine BMSCs were cultured in adipogenic medium containing 0.5 mM isobutylmethylxanthine (Sigma, St. Louis, Mo, USA), 0.5 mM hydrocortisone and 60 mM indomethacin (Sigma), which was replaced every three days for 4 weeks. The cells were fixed using 4% paraformaldehyde and stained with oil red O in isopropanol for 30 min at room temperature [Citation17]. For chondrogenic differentiation, cells were cultured in chondrogenic medium (Cyagen, Guang Zhou, China) containing 1% penicillin/streptomycin, 1% ITS (Sigma, Guang Zhou, China), 0.1 mM L-ascorbate-2-phosphate, 0.4 mM proline (Sigma), 100 nM dexamethasone and 10 ng/ml transforming growth factor-β3 (TGFβ3, Sigma) for 4 weeks. Chondrogenic differentiation was detected by Alcian blue staining. For osteogenic differentiation, the cells were cultured in osteogenic medium (DMEM, 10% FBS, 1% penicillin/streptomycin, 50 μg/mL L-ascorbic acid, 10 mM glycerophosphate and 100 nM dexamethasone) for 4 weeks. The cells were fixed using 95% ethanol and stained with 1% Alizarin red S (Sigma) for 30 min at room temperature [Citation18,Citation19].
Gene transduction of canine BMSCs
Lentiviral vectors containing the toll-like receptor 2 (TLR2) and green fluorescent protein (GFP) or GFP was transfected into canine BMSCs at a multiplicity of infection (MOI) of 35 using polybrene at 8 μg/ml. The transduction efficiency was observed with a fluorescence stereomicroscope (Leica, Wetzlar, Germany) after 72 h transfection. The expression of TLR2 was detected by immunofluorescence and Western blot. For immunofluorescence analysis, the cells were fixed in 4% paraformaldehyde for 15 min at 4 °C, treated with 1% Triton X-100 for 30 min and then blocked with 10% goat serum for 1 h at room temperature, and then incubated with a primary antibody against TLR2 (Abcam, Cambridge, USA) at 1:400 overnight at 4 °C, following incubation with DyLight 549-conjugated anti-mouse antibody (Invitrogen, Carlsbad, CA, USA) for 1 h at 37 °C, and the nuclei were counterstained with 4, 6-diamino-2-phenyl indole (DAPI, Sigma, St.Louis, MO,USA). All samples were viewed with a fluorescence stereomicroscope (Leica, Germany).
Effects of TLR2 on regulating BMSCs mediated osteogenesis and angiogenesis in vitro
To investigate the effects of TLR2 on regulating BMSCs mediated osteogenesis and angiogenesis in vitro. PGN (Sigma) at a final concentration of 10 μg/ml was used to activate the TLR2 signaling pathway in TLR2-BMSCs. After culturing the cells in osteogenic differentiation medium with the administration of PGN, the total RNA and protein were collected at corresponding time points and the expression of osteogenic and angiogenic related markers was evaluated with real-time reverse transcriptional- polymerase chain reaction (RT-PCR) and Western blotting. At days 3 and 7, ALP staining was performed. Briefly, cells in each group were fixed with 4% paraformaldehyde for 30 min, washed with PBS three times and then stained with an ALP kit (Beyotime , Shanghai, China). The expression of osteocalcin (OCN) was evaluated using immunofluorescence at day 7. The cells were fixed with 4% paraformaldehyde for 30 min, washed with PBS, then incubated with 1% bovine serum albumin (BSA) and 0.1%TrixtonX-100 for 20 min at room temperature. After blocking and permeabilization, the cells were incubated with primary antibody OCN (1:400; Abcam, USA) overnight at 4 °C. Then, the cells were incubated secondary antibody labeled by DyLight549 (Invitrogen, USA) at room temperature for 1 h. The cell nuclei were stained with 4',6-diamidino-2-phenylindole (DAPI, Invitrogen, USA) The results were observed using a fluorescence microscope (Leica, Germany).
Real-time quantitative PCR
Total RNA was extracted from canine BMSCs of each group (BMSC-GFP, PGN + BMSCs-GFP, BMSC-TLR2, PGN + BMSCs-TLR2) using Trizol Reagent (Invitrogen) at day 2, 4, and 6 and quantified with a Thermo Scientific NanoDrop™ 1000 ultraviolet-visible spectrophotometer (NanoDrop Technologies, Wilmington, DE, USA). Then, cDNA was synthesized using a Prime-ScriptTM RT reagent kit (Takara Bio, Shiga, Japan) according to the manufacturer’s instructions. The related genes, including runt-related transcriptional factor 2 (Runx2), alkaline phosphatase (ALP), osteocalcin (OCN), and vascular endothelial growth factor (VEGF) were measured, and glyceraldehyde-3-phosphate dehydrogenase (GAPDH) was used as the housekeeping gene for the normalization of RNA expression levels. The PCR primer sequences are displayed in .
Table 1. Nucleotide sequences for real-time RT-PCR primers.
Western blot analysis
The canine BMSCs were cultured using osteogenic medium with administration of peptidoglycan (10 μg/ml) for 7 days, and then the total protein was harvested using a protein extraction reagent (Kangchen, Shanghai, China); the cell lysates were subsequently centrifuged at 12000 rpm for 15 min at 4 °C and the supernatant was collected for protein analysis. The protein concentration was determined using a BCA Protein Assay. Equal amounts of cell lysates were subjected to 10% SDS-polyacrylamide gel electrophoresis (PAGE) and transferred to polyvinylidene difluoride (PVDF, Pall, New York, USA) membranes. The membranes were blocked with 5% nonfat milk and incubated with primary antibodies against Hif-1α, VEGF or BMP-2 as well as GAPDH and were then incubated with HRP-conjugated secondary antibodies (Sigma). Finally, the protein bands were visualized using ECL Plus reagents (Amersham Pharmacia Biotech, New York, USA).
Subcutaneous transplantation
Before subcutaneous transplantation, the cell/collagen gel complex was prepared (Supplemental Figure S1). Cells from each group were separately collected and mixed with collagen gel at a final concentration of 2 × 106 cells/mL. The athymic nude mice were anesthetized with isoflurane, and then subcutaneously injected the following cell/collagen gel complex: BMSC-GFP/collagen gel complex; PGN + BMSC-GFP/collagen gel complex; PGN + TLR2-BMSC/collagen gel complex. Each group was injected 100 μl. The PGN concentration in the complex was 10 μg/ml. The implants of each group were harvested after two weeks transplantation and fixed in 4% paraformaldehyde. The fixed samples were dehydrated in 10, 20, and 30% sucrose solution, 15 min per each step. Cryo-embedding media (O.C.T, Tissue-Tek, Torrance, CA, USA) was used to cover the whole tissue; 12-μm-thick frozen sections were generated using a cryotome cryostat. For the immunostaining assay, the sections were incubated with the following primary antibodies: CD 31 (1:500; Abcam) or OCN (1:500; Abcam). Then, the sections were incubated with an anti-rabbit secondary antibody labeled by DyLight549 (Invitrogen, USA) and cell nuclei were stained with DAPI (Invitrogen, USA). All sections were observed using a fluorescence microscope
TLR2-BMSC mediated bone regeneration in vivo under an inflammatory environment
Canine periodontitis model
A total of six male adult labrador dogs, aged 2 years old, with an average weight of 25 kg, were used in this study. The experimental protocol was approved by the Animal Care and Experiment Committee of Ninth People’s Hospital affiliated with the Shanghai Jiao Tong University School of Medicine. To induce periodontitis, 3–0 silk sutures were placed at the cervix of the gingiva of the mandibular premolar [Citation20]. The selected experimental site of the mandible premolar was shown in Supplemental Figure S2 and then the dogs were fed with high sugar and low protein diet for 3 weeks to form chronic periodontitis.
Tooth extraction and cell/collagen gel complex transplantation
The premolars suffered periodontitis were extracted and the tooth extraction sockets were filled with one of the following implants: collagen gel alone (n = 6); BMSC-GFP/collagen gel complex (n = 6); BMSC-TLR2/collagen gel complex (n = 6). The mucoperiosteal flaps were sutured with to block the tooth extraction sockets. The animals were administered an intramuscular injection of penicillin (4000, 000 IU/ml, 0.1 ml/kg) postoperatively. The dogs were fed with a soft diet for one week after the operation.
Fluorochrome labeling
During the healing period, the new bone formation and mineralization were labeled using different fluorochromes. Three different fluorochromes including ARS, tetracycline (TE, Sigma), and calcein (CA, Sigma) were intraperitoneally administered with a corresponding dose 30 mg/kg, 25 mg/kg, and 20 mg/kg at days 7, 10, and 13 after the operation, respectively.
Micro-CT and histological analysis
All animals were sacrificed at 2 weeks post-operation. The samples of submandibular bone were fixed in 10% formalin solution. The newly formed bone in the tooth extraction sockets was detected and imaged using micro-CT (GE eXplore Locus SP Micro-CT, Boston, Massachusetts, USA). The scanning parameters were set at 80 kV and 80 mA with an exposure time of 3000 ms and the resolution of 15 mm as our previous study. After scanning, the bone mineral density (BMD), new bone area, and trabecular number (Tb.N) of new bone were quantified and analyzed.
After Micro-CT analysis, the submandibular bone samples were dehydrated with an ascending series of alcohol from 70 to 100% and then the samples were embedded by polymethymethacrylate (PMMA). Cross sections of the central segment were cut using a microtome (Leica, Germany) and polished to a final thickness of approximately 40 μm. For histological analysis, the sections of each group were stained with Van Gieson’s picro fuchsin to assess new bone formation. Red color staining area indicated new bone formation. For fluorescence labeling detection, the sections were observed using a confocal laser scanning microscope (CLSM, Leica). The parameters of excitation/emission wavelengths of chelating different fluorochromes were used 543/617 nm for Alizarin Red S (red), 405/580 nm for tetracyclin hydrochloride (yellow) and calcein (green), respectively.
Statistic analysis
All data are expressed as the mean ± standard deviation. Statistical analysis was performed using SPSS 10.0 software (SPSS Inc., Chicago, IL, USA) using ANOVA analysis of variance. Values of p < .05(★) or .01(★★) were considered statistically significant.
Results
BMSC isolation, culture and TLR2 transfection
Canine BMSCs was successfully isolated from bone marrow. Flow cytometry analysis showed that these cells were negative expression of the hematopoietic markers CD31 and CD45 and positive staining for stem cell markers including CD29, CD44, and CD105 (). The multipotent differentiation ability of canine BMSCs was also investigated. The results showed that canine BMSCs can differentiation into adipocytes, osteoblasts, and chondrocytes, positive staining for oil red O, alizarin red S and alcian blue, respectively (). These results indicated that the isolated BMSCs possessing stem cells’ characteristics and multipotent differentiation, and can be used for the following study. After gene transduction, the expression of TLR2 in BMSCs was evaluated by Western blotting and immunofluorescence. The protein expression level of TLR2 and red fluorescence staining of TLR2 in BMSC-TLR2 was significantly enhanced compared with that of the other three groups ().
Effects of PGN on regulating TLR2-modified BMSCs osteogenic differentiation
To investigate the potential effects of TLR2 on regulating BMSCs osteogenic differentiation, PGN was used to activate the TLR2 signaling pathway in TLR2-BMSCs. After culturing the cells with PGN in osteogenic medium for 3 days, real-time RT-PCR results showed that the expression of the osteogenic related gene including Runx2, ALP and OCN was significantly upregulated in PGN + BMSC-TLR2 group compared with other three groups (. Moreover, the expression of angiogenic related gene VEGF was also enhanced in PGN + BMSC-TLR2 group (. Only overexpression of TLR2 or treated with PGN was no effects on regulating BMSC osteogenic differentiation. ALP staining was performed on days 3 and 7. The staining was more intense in PGN + BMSC-TLR2 group than in BMSC-GFP, and PGN + BMSC-GFP group at both time points (). This result was consistent with the PCR result. To further investigate the mechanism of the TLR2 signaling pathway on regulating BMSCs differentiation, total protein was collected after administration of PGN for 7 days. Under the stimulation of PGN, the expression of Hif-1α, VEGF and BMP2 were significantly up-regulated in TLR2-modified BMSCs (). Hif-1α is known to play an important regulatory role in the coupling relationship between angiogenesis and osteogenesis [Citation21,Citation22]. PGN may promote the osteogenic and angiogenic ability of BMSCs through activation of the expression of Hif-1α. OCN expression was also further detected using immunofluorescence, the red fluorescent staining of OCN in cells of PGN + BMSC-TLR2 group was more intense compared with BMSC-GFP group and PGN + BMSC-GFP (.
Ectopic angiogenesis and osteogenesis of BMSC-TLR2
The implants were harvested after subcutaneous transplantation for 2 weeks. The histological analysis of the implants was performed using cryosection. The sections of all groups showed intense green fluorescence. This indicated that the transplanted cells survived well in vivo (). CD31 staining showed more intense red fluorescence compared with other groups (). This indicated that there was more blood vessels formation in PGN + BMSC-TLR2 group than in other groups. OCN staining showed the most intense staining in PGN + BMSC-TLR2 group and the green fluorescence of the transplanted cells was overlapped with red fluorescence of OCN staining (). These results indicated that stimulation of TLR2 signaling pathway by PGN enhanced BMSCs mediated angiogenesis osteogenesis.
Figure 4. Ectopic osteogenesis and angiogenesis of BMSC-TLR2. (a) Schematic illustration of the experiment process. (b) Blood vessels formation in the implants were detected using fluorescence staining of CD31. (c) Osteogenic differentiation of transplanted cells was detected using fluorescence staining of OCN.
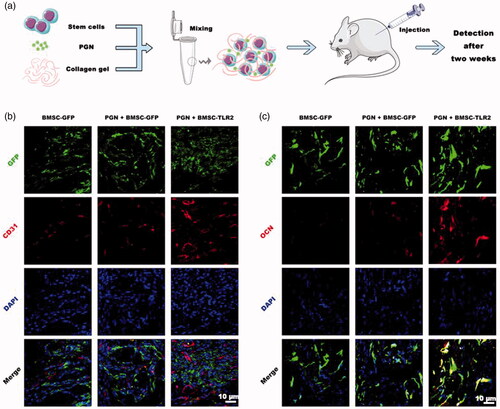
BMSCs-TLR2 mediated bone regeneration in tooth extraction sockets under inflammation environment
To investigate in vivo bone regeneration ability under an inflammatory environment, we first established a periodontitis canine periodontitis model, and then the TLR2-modified BMSCs/collagen gel complex was transplanted into tooth extraction sockets was shown in . After transplantation for 2 weeks, the samples were harvested and analyzed. Micro-CT analysis showed that there was more newly formed bone tissue in TLR2-modified BMSCs group than in both the control group and GFP-BMSCs group (). Van Gieson’s picro fuchsin staining of the undecalcified alveolar bone specimens showed that there was plenty of cancellous bone formation in the tooth extraction sockets of TLR2-modified BMSCs group compared with a control group and GFP-BMSC group (). The tooth extraction sockets were almost fulfilled by the newly formed bone tissue in TLR2-BMSC group (). The quantitative analysis result showed the same results (). The newly regenerated bone tissue was also labeled by three types of fluorochrome (ARS, TE, and CA) at different time points (days 7, 10, and 13). There was more fluorescence positive newly formed bone area in TLR2-BMSC group among all three groups.
Figure 5. BMSC-TLR mediated bone regeneration in tooth extraction sockets. (a) Schematic illustration of the experiment process. (b) Micro-CT and histological analysis of the new bone formation. Upper panel, coronal view of Micro-CT scanning; Lower panel, Van Gieson’s Picrofuchsin staining. The newly formed bone appears red. (c, d) BMD and Tb.N statistic results according to the micro-CT assay (n = 6). (e) Histomorphometric analysis of the newly formed bone area in the tooth extraction sockets. (f) Sequential fluorescence labeling of the new bone formation. Red, Alizarin Red S (ARS); Yellow, Tetracycline (TE); and Green, Calcein (CA). (★, p < .05; ★★, p < .01).
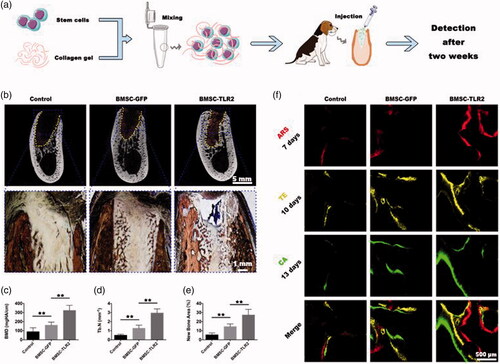
Discussion
TLR2, as an important member of the toll-like receptor family, recognizes the most ligands including peptidoglycan (PGN), lipid teichoic acid (LAT) of gram-positive bacteria, lipopolysaccharide, the main component of the cell wall of gram-negative bacteria and so on [Citation23]. It plays an important role in recognizing the innate immune response of various pathogenic microorganisms [Citation24–30]. The activation of TLR2 was also reported to enhance tissue vascularization and wound healing [Citation11]. In order to further confirm the effects of TLR2 on regulating BMSCs-based bone regeneration. In this study, we established a TLR-modified canine BMSCs line using a lentivirus vector to investigate the mechanism underlying TLR2 regulation of BMSCs-based bone regeneration in the inflammatory microenvironment.
When the stem cells are exposed to some harsh condition such as hypoxemia and inflammation, TLR receptors on the surface of BMSCs can bind with endogenous ligands or other inflammatory factor and then activating the relative signaling pathway to regulate BMSCs mediated tissue repair and regeneration. PGN, the main component of bacterial cell wall [Citation15,Citation31], was used to stimulate TLR2 in this study. After activation of the TLR2 signaling pathway, real-time PCR result showed that PGN can stimulate the high expression of osteogenic related marker Runx2, ALP, and OCN and also the angiogenic marker VEGF. The overexpression of TLR2 alone has no effect on the osteogenesis and angiogenesis differentiation of stem cells.
HIF-1α is a major regulator of hypoxic/ischemic vascular response, regulating the transcriptional activities of hundreds of genes, including vascular responses, angiogenesis, arterial angiogenesis, and bone marrow-derived angiogenesis [Citation32,Citation33]. VEGF is the major downstream target gene of HIF-1α [Citation34], HIF-1α-mediated tissue engineered bone has a greater advantage in promoting angiogenesis than VEGF or SDF-1. During bone regeneration and repair, angiogenesis is inseparable from bone formation and exists in a coupled form [Citation35]. Recent studies have shown that HIF-1α is an essential key factor in the process of angiogenesis coupled with bone formation [Citation33,Citation36]. In this study, we found that Hf-1α, VEGF and BMP2 were up-regulated in TLR2-modified BMSCs under PGN stimulation. Therefore, it can be speculated that PGN may promote the high-level expression of HIF-1α in BMSCs through TLR2 receptor, which in turn promotes high expression of VEGF and BMP2 and then enhance BMSCs mediated bone tissue regeneration.
For further investigating the effects of BMSCs-TLR2 mediated bone regeneration in vivo. Firstly, we utilized subcutaneous implants of BMSCs-TLR2/collagen gel in nude mice. The result of immunofluorescence staining for CD31 and OCN was more intense than other groups. These results indicated that stimulation of TLR2 signaling pathway enhanced BMSCs mediated osteogenesis and angiogenesis. Moreover, in situ bone regeneration of the canine tooth extraction sockets under inflammation environment also showed almost full bone regeneration in TLR2-modified BMSCs group. Since the microbiota routinely lives in the oral environment [Citation37], especially in periodontitis condition, the remained microbial antigen such as PGN and LPS in the tooth extraction sockets which suffered periodontitis may activate the TLR2 signaling pathway in the transplanted BMSC-TLR2 and then enhanced BMSCs mediated bone regeneration in the inflammatory environment. The survive of these TLR2 modified BMSCs in the defect region, they might be re-activated by local pathogenic factors of reinfection to enhance bone regeneration and maintain a long-term effect.
In conclusion, PGN can promote BMSCs mediated-vascularized bone regeneration through the TLR2 receptor. This finding may contribute to stem cell-based bone tissue regeneration in an inflammatory microenvironment, especially in periodontal bone regeneration ().
Disclosure statement
No potential conflict of interest was reported by the authors.
Additional information
Funding
References
- Cochran DL. Inflammation and bone loss in periodontal disease. J Periodontol. 2008;79:1569–1576.
- Oliver RC, Brown LJ. Periodontal diseases and tooth loss. Periodontol 2000. 1993;2:117–127.
- Heitz‐Mayfield LJ. Peri‐implant diseases: diagnosis and risk indicators. J Clin Periodontol. 2008;35:292–304.
- Zhang W, Zhang X, Wang S. comparison of the use of adipose tissue–derived and bone marrow–derived stem cells for rapid bone regeneration. J Dent Res. 2013;92:1136–1141.
- Niemeyer P, Fechner K, Milz S, et al. Comparison of mesenchymal stem cells from bone marrow and adipose tissue for bone regeneration in a critical size defect of the sheep tibia and the influence of platelet-rich plasma. Biomaterials. 2010;31:3572–3579.
- Kaigler D, Pagni G, Park CH, et al. Stem cell therapy for craniofacial bone regeneration: a randomized, controlled feasibility trial. Cell Transplant. 2013;22:767–777.
- Aderem A, Ulevitch RJ. Toll-like receptors in the induction of the innate immune response. Nature. 2000;406:782.
- Medzhitov R, Janeway C. The toll receptor family and microbial recognition. Trends Microbiol. 2000;8:452–456.
- Heumann D, Roger T. Initial responses to endotoxins and gram-negative bacteria. Clinica Chimica Acta. 2002;323:59–72.
- Hashimoto M, Asai Y, Ogawa T. Separation and structural analysis of lipoprotein in a lipopolysaccharide preparation from Porphyromonas gingivalis. Int Immunol. 2004;16:1431–1437.
- West XZ, Malinin NL, Merkulova AA, et al. Oxidative stress induces angiogenesis by activating TLR2 with novel endogenous ligands. Nature. 2010;467:972.
- Wu J-Y, Chen C-H, Wang C-Z, et al. Low-power laser irradiation suppresses inflammatory response of human adipose-derived stem cells by modulating intracellular cyclic AMP level and NF-κB activity. PLoS One. 2013;8:e54067.
- Opitz CA, Litzenburger UM, Lutz C, et al. Toll‐like receptor engagement enhances the immunosuppressive properties of human bone marrow‐derived mesenchymal stem cells by inducing indoleamine‐2, 3‐dioxygenase‐1 via interferon‐β and protein kinase R. Stem Cells. 2009;27:909–919.
- Hwa Cho H, Bae YC, Jung JS. Role of toll-like receptors on human adipose-derived stromal cells. Stem Cells. 2006;24:2744–2752.
- Schwandner R, Dziarski R, Wesche H, et al. Peptidoglycan- and lipoteichoic acid-induced cell activation is mediated by toll-like receptor 2. J Biol Chem. 1999;274:17406–17409.
- Zhang W, Wang G, Liu Y, et al. The synergistic effect of hierarchical micro/nano-topography and bioactive ions for enhanced osseointegration. Biomaterials. 2013;34:3184–3195.
- Zhang M, Jiang F, Zhang X, et al. The effects of platelet‐derived growth factor‐BB on human dental pulp stem cells mediated dentin‐pulp complex regeneration. Stem Cells Transl Med. 2017;6:2126–2134.
- Dai J, Wang J, Lu J, et al. The effect of co-culturing costal chondrocytes and dental pulp stem cells combined with exogenous FGF9 protein on chondrogenesis and ossification in engineered cartilage. Biomaterials. 2012;33:7699–7711.
- Chen B, Sun H-H, Wang H-G, et al. The effects of human platelet lysate on dental pulp stem cells derived from impacted human third molars. Biomaterials. 2012;33:5023–5035.
- Glowacki AJ, Yoshizawa S, Jhunjhunwala S, et al. Prevention of inflammation-mediated bone loss in murine and canine periodontal disease via recruitment of regulatory lymphocytes. Proc Natl Acad Sci. 2013;110:18525–18530.
- Schipani E, Maes C, Carmeliet G, et al. Regulation of osteogenesis‐angiogenesis coupling by HIFs and VEGF. J Bone MinerRes. 2009;24:1347–1353.
- Wan C, Shao J, Gilbert SR, et al. Role of HIF-1alpha in skeletal development. Ann N Y Acad Sci. 2010;1192:322–326.
- Vives‐Pi M, et al. Evidence of expression of endotoxin receptors CD14, toll‐like receptors TLR4 and TLR2 and associated molecule MD‐2 and of sensitivity to endotoxin (LPS) in islet beta cells. Clin Exp Immunol. 2003;133:208–218.
- Texereau J, Chiche J-D, Taylor W, et al. The importance of toll-like receptor 2 polymorphisms in severe infections. Clin Infect Dis. 2005;41:S408–S415.
- Satta N, Kruithof EK, Reber G, et al. Induction of TLR2 expression by inflammatory stimuli is required for endothelial cell responses to lipopeptides. Mol Immunol. 2008;46:145–157.
- McCurdy JD, Olynych TJ, Maher LH, et al. Cutting edge: distinct Toll-like receptor 2 activators selectively induce different classes of mediator production from human mast cells. J Immunol. 2003;170:1625–1629.
- Redecke V, Hacker H, Datta SK, et al. Cutting edge: activation of Toll-like receptor 2 induces a Th2 immune response and promotes experimental asthma. J Immunol. 2004;172:2739–2743.
- Medzhitov R. Toll-like receptors and innate immunity. Nat Rev Immunol. 2001;1:135.
- Takeda K, Akira S. Toll-like receptors in innate immunity. Int Immunol. 2004;17:1–14.
- Urbonaviciute V, Fürnrohr BG, Meister S, et al. Induction of inflammatory and immune responses by HMGB1–nucleosome complexes: implications for the pathogenesis of SLE. J Exp Med. 2008;205:3007–3018.
- Takeuchi O, Hoshino K, Kawai T, et al. Differential roles of TLR2 and TLR4 in recognition of gram-negative and gram-positive bacterial cell wall components. Immunity. 1999;11:443–451.
- Rey S, Semenza GL. Hypoxia-inducible factor-1-dependent mechanisms of vascularization and vascular remodelling. Cardiovasc Res. 2010;86:236–242.
- Zou D, Zhang Z, He J, et al. Blood vessel formation in the tissue-engineered bone with the constitutively active form of HIF-1α mediated BMSCs. Biomaterials. 2012;33:2097–2108.
- Fukuda R, Hirota K, Fan F, et al. Insulin-like growth factor 1 induces hypoxia-inducible factor 1-mediated vascular endothelial growth factor expression, which is dependent on MAP kinase and phosphatidylinositol 3-kinase signaling in colon cancer cells. J Biol Chem. 2002;277:38205–38211.
- Riddle RC, Khatri R, Schipani E, et al. Role of hypoxia-inducible factor-1α in angiogenic–osteogenic coupling. J Mol Med. 2009;87:583–590.
- Deng Y, Zhou H, Zou D, et al. The role of miR-31-modified adipose tissue-derived stem cells in repairing rat critical-sized calvarial defects. Biomaterials. 2013;34:6717–6728.
- Wade WG. The oral microbiome in health and disease. Pharmacol Res. 2013;69:137–143.