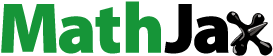
Abstract
Escherichia coli O157:H7 is considered as emerging foodborne pathogens that occur globally. Three major virulence protein factors; EspA(E), intimin(I), Tir(T) and Stx2 toxin have been found to be highly associated with bloody diarrhoea or, Haemolytic Uremic Syndrome. In this study, a trivalent recombinant EIT in combination with the binding domain of STX toxin were encapsulated with chitosan nanoparticles as a combination vaccine candidate. Mice were immunized either subcutaneously or orally with these antigens and challenged with E. coli O157:H7. Results of the binding inhibition assay with caco2 cell monolayer show a significant reduction in the adhesion percentage of pre-treated E. coli O157:H7 with immunized mice sera. Evaluation of neutralizing abilities of immune sera pre-incubated with CD50 dose of STX2 by Vero cells cytotoxicity neutralization assay shows less morphological reforms in comparison with the control groups. Results of mice mortality challenge with STX2 demonstrate around 66% of survived in immunized mice. In a challenge experiment with E. coli O157:H7, all the immunized mice showed a significant decrease in bacterial colonization and shedding. The results indicate that the use of multiple recombinant proteins in combination with natural nanostructure effectively evocated strong humoral and mucosal response, increasing the protection capacity of the synthetic antigen.
Introduction
Enterohaemorrhagic Escherichia coli (EHEC) O157:H7, the main serotype of this species due to the ability of fast colonization on the digestive system is an important zoonotic enteric pathogen that causes Haemorrhagic Colitis (HC) and Haemorrhagic Uremic syndrome (HUS) in humans [Citation1]. The occurrence of acute diarrhoea affected by E. coli strains is very wide-reaching, especially in children and is one of the most important causes of haemorrhages, mainly in areas with a lower level of health [Citation2]. Therefore study the methods by which further immunization against E. coli (EHEC) O157:H7 can be reached, will be crucial. In this regard, researchers initially intended to design EHEC vaccines by screening original antigens and virulence factors of this bacteria [Citation3]. Furthermore, with genetic engineering, a new face of biological knowledge was emerged making it no longer necessary to use the whole body of the pathogen as a vaccine, rather, specific genes and expressions can be used to produce these subunit vaccines reducing the general risks involved [Citation4,Citation5]. The major groups of EHEC virulence factors are the Locus of Enterocyte Effacement (LEE) encoded factors, toxins and adhesions [Citation6,Citation7]. Shiga toxins as Vero toxins are produced by several enteric pathogens, mostly by Enterohaemorrhagic E. coli (EHEC) O157: H7 that cause illness ranging from mild intestinal disease to severe kidney disease [Citation8–10]. They are classified into two distinct groups; Stx1 and Stx2, which are strong AB5 toxins [Citation11]. Stx2 is more potent in toxicity and lethality models in mice, also is more related to severe disease [Citation12]. Until now several Shiga toxin based subunit vaccine strategies using of sticky and nontoxic part of this toxin has been employed to reduce these relevant infections [Citation13–15]. Gao et al., 2011 by applying Stx2B-Stx1B fusion protein in mice model proved 93% survival rate of orally immunized mice challenged with E. coli O157:H7 [Citation16].
The EspA, Intimin and Tir are the three important proteins for bacterial binding to intestinal epithelial cells. EspA which is encoded by espa gene could provide a filamentary structure in the host cell using a bridging apparatus for transferring other factors involved in bacterial implantation [Citation17]. The Tir is processed on the host cell surface in order to get ready for receiving intimin as its own ligand [Citation18]. The eae gene encodes intimin as membrane protein for binding the bacteria to the host cell [Citation19]. Wan and colleagues by using intimin antigen demonstrated the reduction of bacterial attachment to Hep-2 cells and protection of immunized mice [Citation20]. Nowadays, studies have shown further methods for extended immunization against these three pathogens. Previous research showed that with the preparation of the trivalent gene construct, the mice immunogenicity is higher than the case of each of the above pathogens separately [Citation14,Citation21]. Results proved that immunization with a combination of several recombinant antigens can be more effective [Citation22]. Therefore, the joint use of toxin and attachment factors together can produce better protection responses against E. coli O157:H7. Further, since the transmission of this bacteria often occurs through faecal–oral routes, use of mucosal vaccination for prevention and controlling of its infection is highly recommended. To extend the above-reached immunization levels, enhancements of the mucosal routes by which the vaccine is introduced to the body becomes necessary. Nanocarriers are the best and modern approach for the safe transfer of antigens to mucosal surfaces [Citation23]. Some of the advantages of nanovaccine candidates are painless, more effective and safe needle-free routes of boosters [Citation24]. Chitosan as biodegradable and bio-friendly nanoparticles exhibit clear adjuvant properties [Citation25–27] and due to stimulation of the immune system by producing cytokines, will be able to enhance the immune system functionalities [Citation28]. Use of chitosan shall not only protect antigens but also promotes slow triggering and prolong exposure to the immune system, thus creating an improving effect on the mucosal and cellular immune responses [Citation29–31]. Doavi and colleagues studied on the intranasal route of vaccination with rEIT nanoparticulated with chitosan applied in mice and proved inhibition of EHEC colonization in immunized mice [Citation32]. For achieving a better result in immunogenicity, mixing of nanovaccine candidates as a combination vaccine can be ideal for providing protective immunity to several virulence factors of this bacteria [Citation14]. Zhang and colleagues used chimeric protein Tir-Stx1B-Stx2B combination with Zot adjuvant to provide protection of immunized mice against E. coli O157:H7 and obtained high titers of IgA and IgG antibodies and reduction of bacterial shedding in their feces [Citation33].
In the current study, rEIT and rStx2B [Citation14,Citation21] as the recombinant antigens, were nanoparticulated by chitosan separately. Mixing candidates as combination vaccines were applied in 36 BALB/c mice and their immunogenicity were evaluated.
Methods and materials
Bacterial strains, plasmids and media
Escherichia coli O157:H7 (strain ATCC 35218 from Bu Ali hospital), E. coli BL21 (DE3) and the constructed rEIT and Stx2B both in pET28a plasmids were provided by the NIGEB laboratory [Citation21].
All E. coli strains were grown in LB broth at 37 °C; media were supplemented when required with kanamycin (SIGMA, 40 µg/ml).
Expression of recombinant EIT and Stx2B
pET28-eit and pET28-stxB with the 6X-His-tag at the N-terminal under the control of the T7 promoter were transformed into E. coli BL21 (DE3) separately for expression of recombinant proteins. Frozen cells were inoculated into LB medium with Kanamycin. The grown cultures under shaking condition at 37 °C overnight were used to inoculate LB medium containing Kanamycin for each of antigens. The cultures were grown at 37 °C to an Optical Density (600 nm) of 0.5–0.7. Expression of the rEIT and rStx2B were achieved by the addition of 1 mM IPTG (SIGMA). Harvested cells were resuspended in lysis buffer (50 mM NaH2PO4, pH 8.0, 300 mM NaCl, 0.2 mg/ml Lysozyme for rEIT) (100 Mm NaH2 PO4, 10 mM Tris- Cl 8 M urea;pH 8.0 for rStx2 B). The samples were analyzed by 12% SDS-PAGE.
Purification of recombinant EIT and Stx2B protein
The recombinant 6 His-tagged antigens were purified using two separate Nickel- nitrilotriacetic acid (Ni-NTA agarose - Qiagen) resin under native condition for rEIT and denaturing condition for rStx2B. The rEIT and rStx2B were washed and eluted based on different concentration of imidazole and pH gradient respectively. After using the Bradford method for purified protein estimation, the samples were confirmed for recombinant protein bands by 12% SDS-PAGE.
Western blot analysis
Purified rEIT and rStx2B antigens from 12% SDS-PAGE were transferred to PVDF membrane using transfer buffer. The chimeric recombinant EIT and Stx2B were incubated with HRP conjugated anti- His-tag (1:2000; sigma) in PBS/T (PBS contain 0.05% Tween 20), via gentle shaking for 1 h at 37 °C. Detection finally was carried out using HRP staining solution (DAB). Chromogenic reaction was stopped by rinsing the membrane with distilled water.
Preparation of chitosan solution
Chitosan nanoparticles were prepared by ionic cross linking of chitosan solution with TPP anions. 500 µg of each rEIT and Stx2B antigens were mixed with 7.5 ml of solution of chitosan separately (2 mg/ml, pH 5.5) in 2% of acetic acid during 10 min. The solutions were mixed with drop wise addition of sodium tripolyphosphate (1 mg/ml) under magnetic stirring at room temperature for 1 h. The final mixture was centrifuged at 1300 rpm for 20 min at 4 °C. The pellet was collected for further characterization and kept at 4 °C.
Determination of particle size and the surface charge
Particle size and Zeta potential of nanoparticles with rEIT and Stx2B were measured by dynamic light scattering and Laser Doppler Electrophoresis using Zeta sizer (Nano-ZS, Malvern, UK) at wavelength of 633 nm at 25 °C
Encapsulation efficiency and in vitro antigen release
To check the encapsulation efficiency, the free rEIT and Stx2B that were present in the supernatant after centrifugation were measured in a spectrophotometer at 595 nm via Bradford protein assay [Citation34]. The encapsulation efficiency was calculated using the formula as follows[Citation35]:
(1)
(1)
The nanoparticled recombinant EIT and Stx2B with chitosan in PBS buffer were centrifuged at 13,000 rpm for 20 min at 4 °C and were placed in a shaker incubator at 3 °C. In the times period of 0, 1, 3, 12, 24, 48, 72, 96 and 120 h the quantity of each released antigen was determined by Bradford protein assay, separately.
Animal immunization
Thirty-six female five-week-old BALB/c mice (Pasteur Institute of Iran) were divided into four tests (T1, T2, T3, T4) and two control group (C). Each mouse in the T1 group was immunized directly by oral gavage administration of 100 µg chitosan nanoparticled rEIT protein mixed with 100 µg chitosan nanoparticled Stx2B four times at 2 weeks intervals. The T2 group was immunized by oral gavage with the same amount for three times and the intraperitoneal injection of 15 µg pure rEIT mixed with 15 µg pure rStx2B for last period. The mice from T3 group were injected subcutaneously with 15 µg recombinant EIT protein mixed with 15 µg pure rStx2B with complete Freund,s adjuvant (CFA) for the first time and with the same amount antigens using incomplete Freund adjuvant (IFA) for the second and third times. The last injection was performed without adjuvant. The mice from the T4 group were injected subcutaneously with 15 µg nanoparticled recombinant EIT protein mixed with 15 µg pure rStx2B. Control group was injected intraperitoneally with PBS for three times and administrated gavage through chitosan only without antigen for last time. The mice groups are shown in . Pooled blood samples from each group of mice were collected retro-orbitally after the third and fourth immunizations. In order to recover antibodies from feces, 1 gram of pooled samples collected from each group was mixed thoroughly with 500 µl of PBS containing 0.05% (w/v) sodium azide and 10 µl/mg protease inhibitor cocktail (Roche, Switzerland).The supernatant of the faecal after centrifugation (4 °C, 6000 rpm, 10 min), and sera samples were stored at −70 °C for further analysis. Animal study approvals are evaluable under ref number IR.PNU.REC.1397.39 on www.ethics.research.ac.ir.
Table 1. Immunization administered to the groups of mice.
Determination of serum IgG and faecal IgA antibody responses to rEIT and rStx2B individually
Antibody specific responses were determined by an indirect Enzyme-Linked Immunosorbent assay (ELISA). 96-well plates (Nunk) were coated with 5 µg of purified rEIT protein diluted in coating buffer (64 mM Na2CO3, 136 mM NaHCO3, pH 9.8) at 4 °C, overnight. The plates were washed three times with PBS containing 0.05% Tween 20 (PBS/T) and the non-specific sites were blocked with 3% skimmed milk in PBS/T. For determination of the relative IgG and IgA titers, sera samples were serially diluted in PBS/T from 1:100 to 1:400,000 for IgG and from 1:5 to 1:10,000 for IgA whereas faecal samples. The diluted samples were added to ELISA plates and incubated at 37 °C for 1 h. The plates were washed three times with PBS/T and HRP rabbit anti-mouse IgG (1/2000 in PBS/T) (Sigma) or HRP rabbit anti-mouse IgA (1/10,000 in PBST) (Sigma) were added to the ELISA plates as a conjugated secondary antibody. Plates were incubated (30 min at 37 °C) and washed three times in PBS/T. The wells added with 100 µl of citrate buffer containing 0.06% (W/V) of O-Phenylenediamine Dihydrochloride (OPD)(Sigma) and 0.06% (V/V) hydrogen peroxide were incubated at room temperature for 15 min. The reaction was stopped with 100 µl 0f 2 M H2SO4 and the OD492 was read on a microplate ELISA reader. All process repeated for determination of anti rStx2B by a coating of pure rStx2B antigen with same sera samples as well.
Challenging the immunized mice
To analyze the effect of antibodies on bacterial growth, 2 weeks after the last immunization, the mice were orally challenged with a lethal dose of E. coli O157:H7 All mice groups were pre-treated with streptomycin sulphate (5 mg/ml) for reduction of gut bacterial flora 2 days before. The fasted, antibiotic-treated mice were gavaged with 1010 CFU of E. coli O157:H7. The mice faecal samples were collected daily for 2 weeks. 0.1 g of faecal samples were suspended in 1 ml LB broth and incubated at room temp for 3–4 h, then spun for more homogenization, followed by plating onto Sorbitol MacConkey agar plates containing Cefixime and Tellurite. After incubation (37 °C overnight) the E. coli O157:H7 colonies were counted.
Binding inhibition assay for bacterial adhesion effect
Caco-2 cells (Pasteur Institute of Iran, Tehran) were grown to confluence in a culture flask containing DMEM medium and 10% FBS trypsinized and distributed onto a sterile round coverslip placed on the bottom of a 6-well cell culture plate (Nunk) and incubated in 5% CO2 at 37 °C for 48 h. An overnight culture of E. coli O157:H7 grown to exponential growth phase was washed three times with PBS (pH = 8) and adjusted to an OD: 0.5 at 600 nm (∼108 bacteria). The 300 µl of the bacterial suspension was pre-treated with 150 µl of immunized T1, T2 and T3 mice antisera. The Caco-2 cell line treated with unimmunized mice antisera were used as negative control. The bacteria/serum mixture was added to cells and incubated for 1 h at room temperature. After washing three times with PBS, the Caco-2 cells were fixed in (1 ml of 100% methanol for 5 min) and stained with Giemsa staining solution (SIGMA) for 5 min and then destained with PBS. The coverslips containing the Caco-2 cells bound with E. coli O157:H7 were observed under the optical microscope at 1000× magnification. The number of bacteria that adhered to each cell was counted and determined the percentage of positive cells by examining 100 Caco-2 cells on each coverslip.
Crude preparation of STX
Briefly, an overnight culture of E. coli O157:H7 (from NIGEB) in LB broth grown to exponential growth phase and the turbidity of the broth was adjusted to that of a 5 McFarland standard (109 bacteria). Then the broth was centrifuged (1700 g, 30 min, room temperature) and the supernatant was filter sterilized (0.22 nm) and used as a source of STX [Citation36].
Vero cells cytotoxicity assays and neutralization of Stx2 activity by antibody
The Vero cells were performed and seeded in a 96-well plate, and serial dilutions of rStx2B containing 100 µl of each dilution were added to wells of the microtiter plates. Incubation was continued at 37 °C in 5% CO2 for 3 days. The supernatant was removed, and fresh Dulbecco’s Modified Eagle’s medium (DMEM) (containing 0.5 mg/mL MTT (3–(4, 5-Dimethylthiazol-2-yl)-2, 5-Diphenyltetrazolium Bromide) was added (200 μL/well) and incubated at 37 °C for 4 H. The supernatant was removed, and DMSO was added (150 μL/well). After incubation for 10 min, the absorbance was read at 570 nm. The median Cytotoxic Dose (CD50) corresponded to the amount of toxin required to kill 50% of the cells in a well. The results were expressed as percent use viability compared with control culture viability (100%) from assays performed in the absence of Stx2. The cytotoxicity assay was done at least three times, and the average was used in the results. Neutralization cytotoxicity assay of the mouse anti-Stx2B serum was examined on Vero cells. The 50-μL volume of the Stx2 solution containing the CD50 of the toxin was pre-incubated with 50 μL of diluted the mouse anti-Stx2B solution at 4 °C for one night. The remaining unbound toxin-cytotoxicity was measured by cytotoxicity assay as described above. Cell viabilities were calculated according to the formula described previously [Citation35].
Mouse lethality assay
All groups of mice were inoculated intraperitoneally with the 150 µl of Stx2 as prepared. The mice were observed daily over 10 days and fatalities recorded. All animal experiments were done in accordance with the National Institute of Genetic Engineering and Biotechnology (NIGEB), Tehran, Iran for animal care and experimentation.
Statistical analysis
The data were representative of three separate experiments and described the mean ± standard deviation. Student t-test was performed to analyze the antibody data of the mice groups. Analysis for the significant differences of EHEC attachment inhibition on Caco-2 cells was also tested using Bonferroni multiple comparison test. Mice survival differences were studied by one-sided Fisher’s exact test. In all experiments, the p < .05 was considered statistically significant.
Results
Expression and purification of recombinant protein
The synthetic eit and stx2B genes were expressed separately in E. coli BL21 DE3, with the N-terminal His-tag. Purification of the rEIT was carried out under native condition and Stx2 B under denaturing condition with 12% SDS-PAGE analysis showed the presence of near 61 KD of chimeric antigen for rEIT and 8 KD of rStx2B as a major band in all the eluted fractions. The expression of recombinant EIT protein and Stx2B confirmed with western blotting. To verify the reactivity of the immune sera with antigens, the rEIT and rStx2B were blotted and incubated with antisera of immunized mice (.
Figure 1. Purification and Western blot analysis of recombinant protein (A) Purified rEIT, 6X-His-tagged protein after elution with 250 mM imidazole. Lane 1, protein weight marker, Lane 2, 3, 4, 5 – Purified protein after elution with 40 mM imidazole. (B) Purified rStx2B, 6X-His-tagged protein after elution based on pH gradient under denaturing condition. Lane 1, Protein weight marker. Lane 2, 3, 4, 5 Purified Stx2B after elution with denaturing buffer (pH = 4.5). (C) Western blot analysis of rEIT (∼60 KD) and Stx2B (8 KD) using anti 6X-His-tag antibodies. Lane1: control; Lane 2: rEIT; Lane 3: rStx2 B; Lane 4: protein weight marker. (D) Western blot carried out with serum EIT and Stx2B antibodies, Lane1: mixing of rEIT and rStx2B antigens; Lane 2: protein marker; Lane3: control.
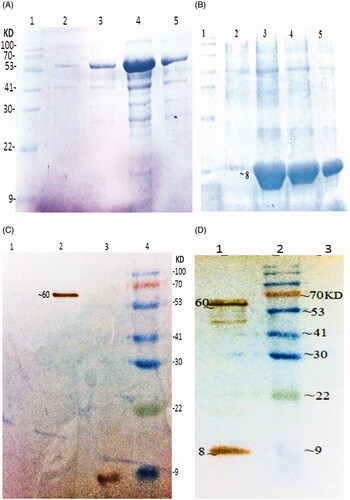
Physical properties of chitosan nanoparticles loaded with rEIT and rStx2B antigens
The measurement of particle size and Zeta potential of nanoparticles with rEIT and rStx2B results are shown in . The size of chitosan particles along with rEIT antigen was about 116.6 nm with a polydispersity index about 0.384 and for nanoparticled Stx2B was about 129.2 with PDI about 0.330. The surface charge of chitosan nanoparticles was measured and the value of +19.3 mV for rEIT and +17.9 mv for rStx2B indicated that the chitosan nanoparticles possessed positive surface charges with good mobility (). The encapsulation efficiency of rEIT and rStx2B in chitosan was calculated and showed that 91% of the rEIT and 95% of rStx2B antigens loaded into chitosan nanoparticles. The in vitro antigens release was studied. displayed a graph showing 76% of rEIT and 78% of rStx2B release of chitosan nanoparticles in PBS buffer during 120 h.
Figure 2. Measurement of physical property of nanoparticles with recombinant protein. Size distribution measured of; (A) rEIT and (B) rStx2B loaded with chitosan by Zetasizer. Zeta potential and Mobility of chitosan nanoparticles containing rEIT, (C) and rStx2B (D). Graph of in vitro antigens release of nanoparticled rEIT (E) and rStx2B (F) base on chitosan in PBS buffer.
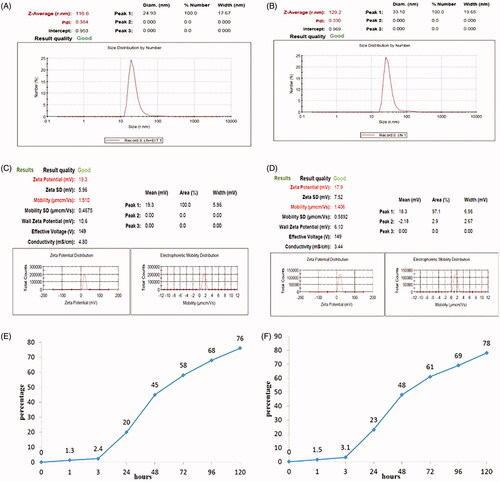
Determination of antibody responses to recombinant antigens
Titration of Faecal and Serum samples from immunized mice of T1 (oral), T2 (oral-injection), T3 (injection) and T4(nano-injection) groups compared to control group C showed significant specific IgA responses against rEIT and rStx2B. The titers of Serum anti-rEIT and anti-rStx2B specific IgG antibody were clearly detectable even at 1:400,000 dilution separately. The titers of rEIT and rStx2B specific IgA in serum and faecal of four immunized mice groups were compared with each other (). Data was shown that the higher titers of serum anti rEIT and rStx2B IgG could be achieved after the last vaccination in all of the groups ().
Figure 3. Results of Challenging tests in immunized and non-immunized mice against E. coli O 157:H7. (A) Reduction of shedding in immunized mice. Test and control groups were orally fed with 1010 E. coli O157:H7 and shedding was monitored daily in feces of each group for 2 weeks. Differences were considered significant whenever p < .05. (B) Number of surviving immunized and non-immunized mice infected with 150 µl of Stx2. Statistical analysis showed significant difference (p < .05) between test and control groups.
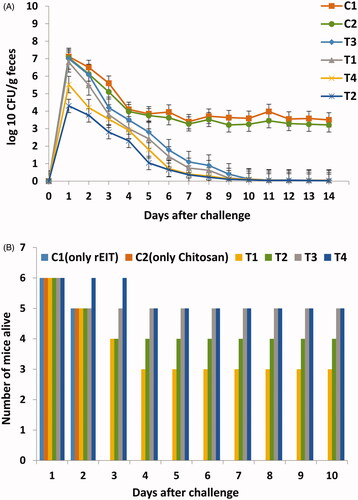
Figure 4. Comparisons of highest level of IgG (1/100) and IgA (1/5) titres against rEIT and rStx2B from immunized and control (non- immunized) groups of mice. (A) Faecal and serum IgA against EIT (B) Faecal and serum IgA against Stx2B (C) Serum IgG against rEIT of third and fourth vaccination (D) Serum IgG against rStx2B after third and fourth immunization.
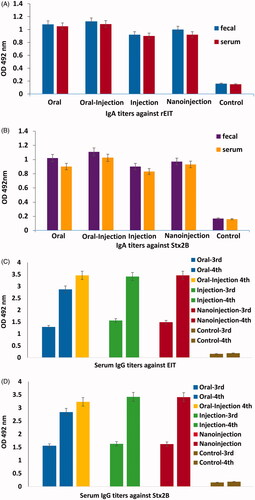
Protection of immunized mice against lethal toxin and E. coli O157:H7 with challenges
In order to determine the ability of rEIT-specific antibodies in immunized mice sera for prevention of the E. coli O157:H7 shedding in faeces, four immunized (T1, T2, T3, T4) and control group (C) were challenged orally with 1010 CFU of E. coli O157:H7 orally. Shedding was followed in faeces of each group for 2 weeks. The result showed that control mice group have high levels of bacterial shedding in their faeces during 2 weeks sampling period at a constant rate. All immunized mice exhibited a statistically significant decrease during colonization of bacteria compared to the control mice (p < .05). There was no significant difference in the duration of shedding between the four groups (. Furthermore, 4 weeks after the last antigen boost, all of the vaccinated groups of mice were challenged with a lethal dose of Stx2. As shown in , the 66% immunized mice with rStx2B survived after challenging for near 2 weeks. In contrast, all of the control mice died after challenging for 2 days. These results clearly demonstrated that the mice were protected against Stx2 toxin ().
3.5. Bacterial adhesion and growth inhibitory effect
Colony count of Caco-2 cell monolayers revealed that E. coli O157:H7 cells pre-treated with sera from the immunized and non-immunized mice have shown different adhesion percentage (). In non-immunized mice, almost all the Caco-2 cells (up to 90%) showed the binding of one or more bacteria with a mean number around 15 bacteria per cell. In contrast in immunized samples, the lower attachment rate was observed (less than 50%). That means the percentage of E. coli O157:H7 cells treated with different groups of immunized mice sera was significantly lower than that treated with non-immunized mice sera (p < .05). All experiments were carried out in duplicate on separate occasions ()
Figure 5. Light micrographs of Giemsa –stained Caco-2 cells with E. coli O157:H7 pre-treated with diferent sera (A) Negative control (only E. coli O157:H7, without pretreated by sera). (B) Non-immunized sera; (C, D, E, F) Immunized mice sera of test groups (T1, T2, T3, T4) for abbreviations see material and methods.
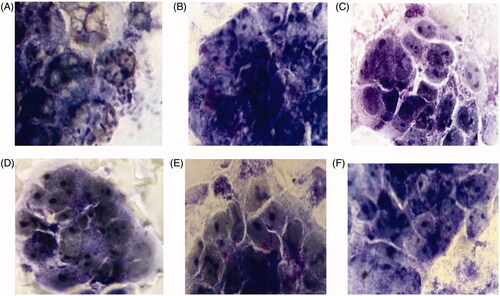
Table 2. Inhibition of E. coli O157:H7 binding to caco-2 cells.
3.6. Vero cells neutralization assay
The Vero cells cytotoxicity assay with the culture supernatant of E. coli O157:H7 as a source of Stx2 indicated that the CD50 of the toxin was equivalent to a 10−2 dilution of the prepared toxin. The Vero cells neutralization assay was performed with 1CD50, 2CD50, 5CD50 and 10 CD50 of Stx2 toxin. The pooled sera of all immunized mice groups can neutralize the activity of Stx2 but the sera of non-immunized mice had no neutralizing effect on Vero cells. The Stx2 caused Vero cell rounding and cells lysing and showed morphological changes (.
Figure 6. Effect of incubated Vero cells with Stx2 toxin. (A) Normal Vero cell control. (B) Morphological changes in Vero cells after treated with 1CD50 dilution of Stx2. Images were taken by phase contrast microscope (40×). (C) Determination of Cytotoxic dose (CD50) on Vero cells. (D) Percentage of neutralization of toxin (Stx2) with anti-sera.
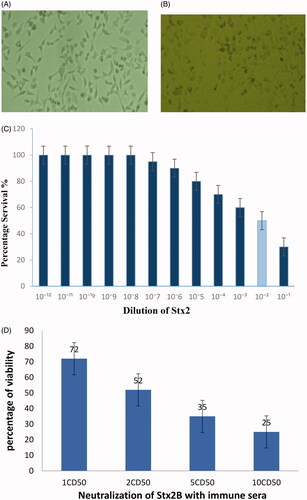
Discussion
Scientists have been actively working on developing safe effective subunit vaccines to reduce the worldwide prevalence of Enterohaemorrhagic E. coli infections [Citation37]. The massive study in the major attaching proteins and toxins have indicated effective properties in the induction of protective immunity and development of a subunit vaccine candidate against E. coli O157:H7 [Citation13,Citation14,Citation32,Citation38,Citation39]. It is necessary to consider that for operational vaccination, more attention is needed to be paid on antigens in the context of activating the proper immune response, immune stimulants for enhancing the immunogenicity, protection of antigens and finally the proper delivery methods for approaching the antigens to the target cells [Citation40].
Recently, the nanovaccines are considered as a new approach to the issue of immunization against E. coli: O157: H7 [Citation24]. Chitosan by functioning as adjuvant, protection and slow release of antigens can be used for transferring recombinant proteins to the target cells. The positive properties of chitosan such as mucosal adhesion, biocompatibility, biodegradability, harmlessness [Citation41,Citation42], controlling the time and rate of degradation and improving the immunogenicity [Citation42] and above all its ability to deliver the antigen to the M-cells in peyer’s patches for preparing better stimulation of mucosal membranes [Citation43] make it an exceptional case in comparison to other carriers.
In this research, two nanovaccine candidates containing chimeric antigen construct (EIT);the most immunogenic parts of three important attachment proteins from E. coli: O157: H7 (EspA, Intimin, Tir) [Citation21] in combination with recombinant Stx2B subunit; which is accountable for toxin binding to the target cell surface [Citation14]; were encapsulated with chitosan nanoparticles as combination vaccine candidate.
While studying the Physical properties of our nanovaccine, the size and positive zeta potential of nanoparticles with a gradual release of the recombinant proteins from the surface of chitosan indicate their better adhesion to the mucosal cells of the intestine and ensuring proper stimulation of the immune system with the help of released antigens [Citation44].
In analyzing the immunogenicity data, we concluded that due to first colonization of E. coli O157:H7 through the mucosa of the gastrointestinal tract, oral-injection routes of mucosal vaccination induced an effectual immune response since the systemic vaccination does not usually rise these antibody levels enough. By administration of three oral and one injection doses of nanoparticled antigens (rEIT-rStx2B), both specific IgG and IgA antibodies, in appropriate levels, were detected. Higher titration of IgG antibodies after final vaccination proved that the fourth round of injection was essential. Furthermore raising of IgG antibodies in oral-injection groups compared to the oral groups after final vaccination was considerable. The presented data are in agreement with those obtained previously by other researchers in mice with non nanopaticulated rEIT subcutaneously and with the intranasal route of vaccination by nanoparticulated rEIT with chitosan and also on cattle with intramuscular route by rEIT without the usage of nanoparticle for immunization [Citation21,Citation32,Citation38]. These studies, although demonstrating the significant results for reduced shedding of E. coli O157:H7 following an oral challenge, but didn’t show protective responses against Shiga toxin of this bacteria. Also, other researchers studied on Stx2B as subunit vaccine candidate and showed only the important results for neutralization of toxin [Citation14,Citation16]. Therefore, for achieving better results, using nanovaccine candidate with multiple factors can be more effective by neutralization of toxin and prevention of bacterial attachment to host cell. In that regard, similar oral-injection vaccination methods only with rEIT antigens (without rStx2B) were simultaneously applied on six BALB/c mice and satisfying results were achieved [Citation45]. These groups of mice were used as negative control in lethality challenges, which resulted in all of them getting sacrificed. These results clearly proved that the mentioned mice were not protected against the toxin of E. coli: O157:H7.
The reduction rate in bacterial shedding of oral-injection and nano-injection groups was more than the other groups over the period of 2 weeks which showed similar finding to that of the previous research [Citation46]. Results of neutralization test showed a significant percentage reduction of E. coli O157:H7 binding to Caco-2 cells after incubation of different groups of immunized mice sera treated with bacteria proving that mice were notably protected against E. coli: O157:H7 [Citation21,Citation32]. Also in Vero cells cytotoxicity assay, the results indicated that most of the Vero cells survived after incubated immunized mice sera was treated with this bacteria. Nearly 70 present of the immunized mice with both recombinant antigens survived after the mice fatality challenge. Data gathered from in vitro and in vivo assay of neutralization of toxin clearly demonstrated the mice were protected against Stx2. This process can inactive the function of Stx2A since without attachment to their receptor (Gb3) other steps such as Stx2A partition and inhibiting protein synthesis will not take place [Citation47–49].
Results from this study clearly showed the benefits of using combination nanovaccine of rEIT and rStx2B antigens for better immunization against EHEC E. coli O157:H7 infections. This mixed and novel nanovaccine candidate with multiple factors can be more effective by neutralization of toxin and prevention of bacterial attachment to the host cell. Moreover, the results revealed that by mixing of both encapsulated recombinant antigens in chitosan, the capacity of immunization was significantly enhanced in nano-injection and oral-injection groups of mice. As a consequence of humoral and mucosal immune responses obtained through several challenging assays, it was proved that with the utilization of this effective and potent nanovaccine candidate, mice were significantly protected against E. coli O157:H7.
Disclosure statement
The authors report no conflict of interest.
References
- Bach SJ, McAllister TA, Veira DM. Transmission and control of Escherichia coli O157:H7—a review. Can J Anim Sci. 2002;82:475–490.
- Pop M, Walker AW, Paulson J, et al. Diarrhea in young children from low-income countries leads to large-scale alterations in intestinal microbiota composition. Genome Biol. 2014;15:R76.
- Garcia-Angulo VA, Kalita A, Torres AG. Advances in the development of enterohaemorrhagic Escherichia coli vaccines using murine models of infection. Vaccine. 2013;31:3229–3235.
- Ellis RW, Brodeur BR. New bacterial vaccines. New York, NY: Springer Science & Business Media; 2012.
- Rojas-Lopez M, Monterio R, Pizza M, et al. Intestinal pathogenic Escherichia coli: insights for vaccine development. Front Microbiol. 2018;9:440.
- Karmali MA. Infection by verocytotoxin-producing Escherichia coli. Clin Microbiol Rev. 1989;2:15–38.
- Gyles C. Shiga toxin-producing Escherichia coli: An overview 1. J Animal Sci. 2007;85:E45–E62.
- Boerlin P, McEwen SA, Boerlin-Petzold F, et al. Associations between virulence factors of Shiga toxin-producing Escherichia coli and disease in humans. J Clin Microbiol. 1999;37:497–503.
- Obrig TG. Escherichia coli Shiga toxin mechanisms of action in renal disease. Toxins. 2010;2:2769–2794.
- Johannes L, Römer W. Shiga toxins—from cell biology to biomedical applications. Nat Rev Microbiol. 2010;8:105.
- Paton JC, Paton AW. Pathogenesis and diagnosis of Shiga toxin-producing Escherichia coli infections. Clin Microbiol Rev. 1998;11:450–479.
- Mohawk KL, Melton-Celsa AR, Robinson CM, et al. Neutralizing antibodies to Shiga toxin type 2 (Stx2) reduce colonization of mice by Stx2-expressing Escherichia coli O157:H7. Vaccine. 2010;28:4777–4785.
- Lu X, Skurnik D, Pozzi C, et al. A Poly-N-Acetylglucosamine − Shiga toxin broad-spectrum conjugate vaccine for Shiga toxin-producing Escherichia coli. MBio. 2014;5:e00974.
- Kazemi R, Akhavian A, Amani J, et al. Immunogenic properties of trivalent recombinant protein composed of B-subunits of LT, STX-2, and CT toxins. Microb Infect. 2016;18:421–429.
- Mejias MP, Ghersi G, Craig PO, et al. Immunization with a chimera consisting of the B subunit of Shiga toxin type 2 and brucella lumazine synthase confers total protection against Shiga toxins in mice. J Immunol. 2013;191:2403–2411.
- Gao X, Cai K, Shi J, et al. Immunogenicity of a novel Stx2B–Stx1B fusion protein in a mice model of Enterohaemorrhagic Escherichia coli O157:H7 infection. Vaccine. 2009;27:2070–2076.
- Dziva F, Vlisidou I, Crepin VF, et al. Vaccination of calves with EspA, a key colonisation factor of Escherichia coli O157:H7, induces antigen-specific humoral responses but does not confer protection against intestinal colonisation. Veter Microbiol. 2007;123:254–261.
- Fan H-y, Wang L, Luo J, et al. Protection against Escherichia coli O157:H7 challenge by immunization of mice with purified Tir proteins. Mol Biol Rep. 2012;39:989–997.
- Dean-Nystrom EA, Bosworth BT, Moon HW, et al. Escherichia coli O157:H7 requires intimin for enteropathogenicity in calves. Infect Immun. 1998;66:4560–4563.
- Wan C-s, Zhou Y, Yu Y, et al. B‐cell epitope KT‐12 of enterohaemorrhagic Escherichia coli O157:H7: A novel peptide vaccine candidate. Microbiol Immunol. 2011;55:247–253.
- Amani J, Salmanian AH, Rafati S, et al. Immunogenic properties of chimeric protein from espA, eae and tir genes of Escherichia coli O157:H7. Vaccine. 2010;28:6923–6929.
- Eko FO, Witte A, Huter V, et al. New strategies for combination vaccines based on the extended recombinant bacterial ghost system. Vaccine. 1999;17:1643–1649.
- Hajizade A, Ebrahami F, Salmanian A-H, et al. Nanoparticles in vaccine development. J Appl Biotechnol Rep. 2015;1:125–134.
- Sekhon BS, Saluja V. Nanovaccines-an overview. Int J Pharm Front Res. 2011;1:101–109.
- Metcalfe SM, Fahmy TM. Targeted nanotherapy for induction of therapeutic immune responses. Trends Mol Med. 2012;18:72–80.
- Vinsova J, Vavrikova E. Chitosan derivatives with antimicrobial, antitumour and antioxidant activities-a review. Cpd. 2011;17:3596–3607.
- Xu Y, Du Y. Effect of molecular structure of chitosan on protein delivery properties of chitosan nanoparticles. Int J Pharmac. 2003;250:215–226.
- van der Lubben IM, Verhoef JC, Borchard G, et al. Chitosan and its derivatives in mucosal drug and vaccine delivery. Eur J Pharma Sci. 2001;14:201–207.
- Qi L, Xu Z, Jiang X, et al. Preparation and antibacterial activity of chitosan nanoparticles. Carbohydr Res. 2004;339:2693–2700.
- Zhao L, Seth A, Wibowo N, et al. Nanoparticle vaccines. Vaccine. 2014;32:327–337.
- Sahdev P, Ochyl LJ, Moon JJ. Biomaterials for nanoparticle vaccine delivery systems. Pharma Res. 2014;31:2563–2582.
- Doavi T, Mousavi SL, Kamali M, et al. Chitosan-based intranasal vaccine against Escherichia coli O157:H7. Iranian Biomed J. 2016;20:97.
- Zhang X-h, He K-w, Zhang S-x, et al. Subcutaneous and intranasal immunization with Stx2B–Tir–Stx1B–Zot reduces colonization and shedding of Escherichia coli O157:H7 in mice. Vaccine. 2011;29:3923–3929.
- Kruger NJ. The Bradford method for protein quantitation. In: The protein protocols handbook. Totowa (NJ): Springer; 2002. p. 15–21.
- Tu W, Li T, Wang Q, et al. A simple method for expression and purification of Shiga toxin 1 (Stx1) with biological activities by using a single‐promoter vector and native signal peptide. Biotechnol Appl Biochem. 2016;63:539–545.
- McFarland J. The nephelometer: an instrument for estimating the number of bacteria in suspensions used for calculating the opsonic index and for vaccines. J Am Med Assoc. 1907;49:1176–1178.
- Mehr KS, Mousavi SL, Rasooli I, et al. A DNA vaccine against Escherichia coli O157:H7. Iranian Biomed J. 2012;16:133.
- McNeilly TN, Mitchell MC, Rosser T, et al. Immunization of cattle with a combination of purified intimin-531, EspA and Tir significantly reduces shedding of Escherichia coli O157:H7 following oral challenge. Vaccine. 2010;28:1422–1428.
- Amani J, Mousavi SL, Rafati S, et al. Immunogenicity of a plant-derived edible chimeric EspA, Intimin and Tir of Escherichia coli O157:H7 in mice. Plant Sci. 2011;180:620–627.
- Xing L, Fan Y-T, Zhou T-J, et al. Chemical modification of chitosan for efficient vaccine delivery. Molecules. 2018;23:229.
- Liu M. DNA vaccines: a review. J Intern Med. 2003;253:402–410.
- Nandedkar TD. Nanovaccines: recent developments in vaccination. J Biosci. 2009;34:995–1003.
- Yoo M-K, Kang S-K, Choi J-H, et al. Targeted delivery of chitosan nanoparticles to Peyer’s patch using M cell-homing peptide selected by phage display technique. Biomaterials. 2010;31:7738–7747.
- Bhattarai N, Gunn J, Zhang M. Chitosan-based hydrogels for controlled, localized drug delivery. Adv Drug Deliv Rev. 2010;62:83–99.
- Khanifar J, Hosseini RH, Kazemi R, et al. Prevention of EHEC infection by chitosan nano-structure coupled with synthetic recombinant antigen. J Microbiol Methods. 2019;157:100–107.
- Potter AA, Klashinsky S, Li Y, et al. Decreased shedding of Escherichia coli O157:H7 by cattle following vaccination with type III secreted proteins. Vaccine. 2004;22:362–369.
- Mejias MP, Cabrera G, Fernández-Brando RJ, et al. Protection of mice against Shiga toxin 2 (Stx2)-associated damage by maternal immunization with a Brucella lumazine synthase-Stx2 B subunit chimera. Infect Immun. 2014;82:1491–1499.
- Ishikawa S, Kawahara K, Kagami Y, et al. Protection against Shiga toxin 1 challenge by immunization of mice with purified mutant Shiga toxin 1. Infect Immun. 2003;71:3235–3239.
- Watarai S, Isogai E, Oguma K. Induction of intestinal IgA and IgG antibodies preventing adhesion of verotoxin‐producing Escherichia coli to Caco‐2 cells by oral immunization with liposomes. Lett Appl Microbiol. 2003;36:135–139.