Abstract
Cnidium lactone is effective in the maintenance of bone mass in various osteoporosis models; however, the precise molecular mechanisms are not understood. In this study, we investigated the effects and underlying mechanisms of action of cnidium lactone on receptor activator of NF-κB ligand (RANKL)-induced osteoclastogenesis. Cnidium lactone dose-dependently inhibited osteoclast differentiation and formation, decreased the bone-resorbing activity of osteoclasts, and downregulated the expression of osteoclast differentiation marker genes. Cnidium lactone treatment considerably reduced RANKL-induced p38 MAPK and PI3K-Akt signal activity in RAW264.7 cells. The cnidium lactone-induced osteoclastogenesis was significantly attenuated by inhibition of p38 and PI3K through pretreatment with SB203580 and LY294002, respectively. Furthermore, cnidium lactone inhibited the expression of c-Fos and NFATc-1 with dose-dependently and enhanced by SB203580 and LY294002. In conclusion, cnidium lactone inhibits osteoclast differentiation through p38 MAPK and PI3K-Akt signalling pathway/c-Fos/NFATc1 signalling pathway.
Introduction
Postmenopausal osteoporosis (PO) was a major disease and characterized by imbalanced bone formation and resorption [Citation1,Citation2]. Bone formation and resorption were precisely regulated by osteoblasts and osteoclasts, respectively [Citation3,Citation4]. Many drugs (oestrogen, bisphosphonates or parathyroid hormone (PTH)) have been used to prevent PO, but many adverse reactions prevented these drugs widely application in clinics [Citation5,Citation6]. Recent years, Chinese herbal medicine has been widely used in clinical practice to treat bone diseases and will most likely continue to be used as a cost-effective alternative medicine in China [Citation7]. Cnidium lactone was an effective Chinese herbal medicine and has a beneficial role in preventing bone loss in oestrogen-deficient ovariectomized rats [Citation8]. However, few studies have focused on the effects for osteoclastogenesis and its underlying mechanism.
Many studies have identified that p38 MAPK and PI3K-Akt signalling pathways play important roles in osteoclast differentiation and function [Citation9]. P38 MAPK and PI3K-Akt signalling pathways could activate the downstream osteoclasts formation genes, such as c-Fos and NFATc1 [Citation10]. In the current study, we first identify the optimal dose of cnidium lactone for osteoclastogenesis and whether cnidium lactone inhibits osteoclast differentiation through p38 MAPK and PI3K-Akt signalling pathway/c-Fos/NFATc1 signalling pathway.
Materials and methods
Materials
RAW264.7 cells (a murine macrophage cell line) were purchased from the Institute of Basic Medicine of Peking Union Medical College (Beijing, China). High glucose Dulbecco minimum essential medium (DMEM) and foetal bovine serum (FBS) were obtained from Gibco Company (Carlsbad, CA). Receptor activator of NF-κB ligand (RANKL) was purchased from Peprotech (Rocky Hill, CT). TRAP staining kit was purchased from Sigma Company (St. Louis, MO). Cnidium lactone (purity >99%) was purchased from Yousi Company (Shanghai, China). The following antibodies were purchased from Solarbio Company (Beijing, China): antibodies against phosphor-p38 and phosphor-Akt. Antibodies against NFATc-1 and c-Fos were purchased from Cell Signaling Technology (Boston, MA). SB203580 (p38-specific inhibitor) or LY294002 (pAkt-specific inhibitor) was purchased from Med-Chem-Express (MCE, Monmouth Junction, NJ).
Cell culture
The procedure of this experiment was approved by the Institutional Committee of Hospital. RAW264.7 cells were grown in DMEM supplemented with 10% heat-inactivated FBS and 1% penicillin–streptomycin, at 37 °C in a humidified atmosphere of 5% CO2. The differentiation of RAW264.7 cells was induced in high glucose DMEM containing RANKL (100 ng/mL). Many mature osteoclast-like cells were observed after four days of RANKL induced.
TRAP staining and TRAP activity
TRAP staining was used for detecting osteoclast formation. We performed TRAP staining according to the manufacturer’s instructions. Briefly, RAW264.7 cell lines (density = 1 × 104 cells) were placed in a 96-wells plate and then incubated with recombinant soluble RANKL (100 ng/mL) in the absence of presence of various concentrations of cnidium lactone (10−4 mmol/L, 10−5 mmol/L, 10−6 mmol/L, 10−4 mmol/L combined with SB203580 or LY294002). Concentration of SB203580 or LY294002 was referred to previous studies [Citation11,Citation12].
Bone resorption area
RAW 264.7 cells were seeded on bovine cortical bone slices (density = 1 × 104 cells). RAW264.7 were cultured with DMEM media containing 100 ng/mL RANKL and change the medium every two days. Five days later, the RAW264.7 cells were removed by the pancreatic enzyme. Then, bovine cortical bone slices were fixed with 2.5% glutaraldehyde for over 1 h. Then, all of the slices were subjected to ethanol gradient dehydration, drying and spraying, and observed with scanning electron microscopy (SEM).
Quantitative real-time PCR
Total RNA from RAW264.7 cells under the treated condition was collected by Trizol reagent according to the instructions of the manufacturer. cDNA was synthesized from total-RNA using Prime Script RT reagent Kit (Takara, Dalian, China). After reverse transcription reaction, qRT-PCR was performed by iQ5 real-time PCR detection system (Bio-Rad Laboratories, Hercules, CA). The primers summarized in are designed using the Roche ProbeFinder assay tool. On completion of the reaction, analysis of the amplification curve and melting curve analysis were carried out. Gene expression value is represented by 2−ΔΔCt of initial modulus board amount.
Table 1. Primers used for qRT-PCR analysis.
Western blot analysis
Cells were washed with PBS three times and then lysed in radioimmunoprecipitation assay (RIPA) buffer (Biyuntian, Beijing, China) with added phenylmethanesulfonyl fluoride (PMSF). Above samples were placed in ice for more than three hours and centrifuged with 13,000×g for 15 min at 4 °C. Extracted deposit was quantified by BCA Quantitation Kit (Bio-Rad, Hercules, CA). Equal amounts of protein (100 μg) were separated on SDS-PAGE and then transferred to polyvinylidene difluoride membrane (PVDM, Millipore, Billerica, MA). After blocking with 5% nonfat dry milk diluted in TBST at room temperature for 3 h, membranes were incubated with antibody overnight. After rinsed in TBST, membranes were incubated with appropriate horseradish peroxidase-conjugated secondary antibodies (1:5000; Santa Cruz, Santa Cruz, CA) at room temperature for 2 h, and were then visualized with enhanced chemiluminescence (Santa Cruz, Santa Cruz, CA).
Statistical analysis
Data are presented as mean value ± SD. Statistical analysis was performed with ANOVA followed (where significant) by Dunnett’s test for post hoc comparisons to identify significant pairwise differences. SPSS 20.0 (SPSS, Chicago, IL) was used for all of the comparisons. p Values <.05 were considered statistically significant.
Results
Effect of cnidium lactone on cell viability
RAW264.7 were cultured with various concentrations of cnidium lactone in the presence of RANKL for three days to assess cytotoxicity (). RAW264.7 viability without cnidium lactone treatment was comparable to that of RAW264.7 treated with up to 10−4 mol/L.
Effects of cnidium lactone on number of TRAP-positive multinucleated osteoclasts
Next, we investigated the effects of cnidium lactone on RANKL-induced osteoclast formation and activation. Cnidium lactone effectively inhibited osteoclast differentiation and TRAP(+) multinuclear mature osteoclast formation in a dose-dependent manner (). We also used TRAP activity to assess the effects of cnidium lactone on the osteoclastogenesis.
Figure 2. Cnidium lactone inhibited the osteoclast differentiation and TRAP(+) multinuclear mature osteoclast formation. (A) Control group, (B) cnidium lactone group (10−4 mol/L), (C) cnidium lactone group (10−5 mol/L), (D) cnidium lactone group (10−6 mol/L), (E) quantification of no. of TRAP(+) MNCs and (F) TRAP activity (A405 nm). *p < 0.05 compared with 0 cnidium lactone group; #p < 0.05 compared with 10-6 cnidium lactone group; &p < 0.05 compared with 10-5 cnidium lactone group.
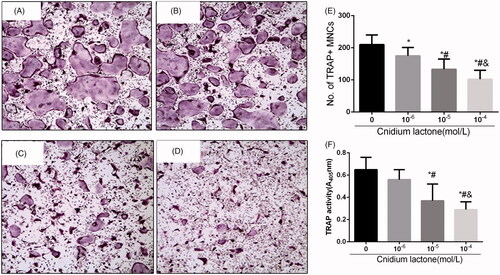
We next investigated whether MAPK and PI3K-Akt signal cascades participate in cnidium lactone inhibited RAW264.7 cells differentiation. The results showed that the mature osteoclasts promoted by RANKL were significantly blocked by SB203580 or LY294002 and similar phenomenon occurred about the TRAP activity in these groups ().
Figure 3. Cnidium lactone and SB203580 or LY294002 inhibited the osteoclast differentiation and TRAP(+) multinuclear mature osteoclast formation. (A) Control group, (B) cnidium lactone group (10−4 mol/L) combined with RANKL (100 ng/mL), (C) cnidium lactone group (10−4 mol/L) combined with RANKL (100 ng/mL) and SB203580, (D) cnidium lactone group (10−4 mol/L) combined with RANKL (100 ng/mL) and LY294002, (E) quantification of no. of TRAP(+) MNCs and (F) TRAP activity (A405 nm). *p < 0.05 compared with control group (0 mol/L cnidium lactone).
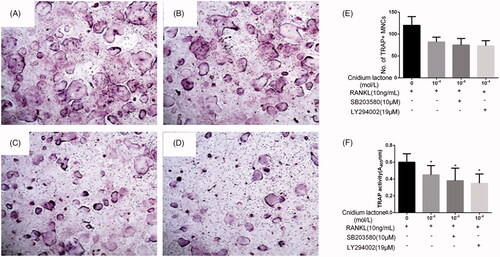
Cnidium lactone reduce resorption pit area
Resorption pit results are shown in , and results indicated that cnidium lactone could decrease the resorption pit in a dose-dependent manner, and 10−4 mol/L cnidium lactone showed the highest inhibition of the osteoclastogenesis than the control group and other doses of cnidium lactone groups.
Figure 4. Cnidium lactone inhibited the resorbed area on bone slices. (A) Control group, (B) cnidium lactone group (10−4 mol/L), (C) cnidium lactone group (10−5 mol/L), (D) cnidium lactone group (10−6 mol/L), (E) quantification of resorbed bone surface and (F) quantification of number of resorbed OCs. *p < 0.05 compared with control group (0 mol/L cnidium lactone).
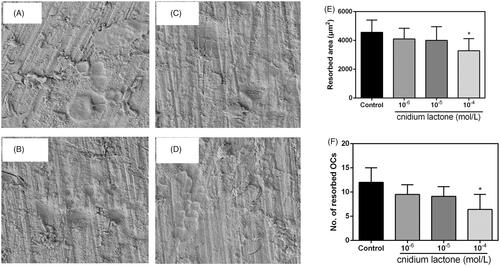
Cnidium lactone inhibited osteoclast differentiation-related genes
As shown in , we used the 10−4 mol/L cnidium lactone as the intervention group and found that cnidium lactone could significantly inhibit osteoclast differentiation genes (Acp5, CtsK, Atp6v0d2, Tm7sf4, Oscar and Nfatc1) with statistical significance (p<.05) at days 1, 2, 3 and 4.
Cnidium lactone inhibited the p38 MAPK and PI3K-Akt protein level
We further explored the activity of the p38 MAPK and PI3K-Akt signalling pathway. Compared with the control group, cnidium lactone significantly decreased the phosphorylation of p38 () and Akt () at a dose-dependent manner, and the most effective concentration was at 10−4 mol/L.
Figure 6. Immunoblotting showing the level of phosphorylated p38 (A) and Akt (B) in RAW 264.7 cells incubated within different concentration of cnidium lactone. The role of cnidium lactone in sustainment of the level of phosphorylated p38 and Akt in RAW264.7 cells inhibited by corresponding inhibitor SB203580 (C) and LY294002 (D).
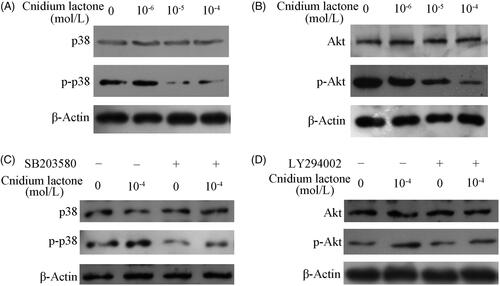
As demonstrated in , treatment with SB203580 or LY294002, in RAW264.7 decreased expression of p-p38 or p-Akt respectively is carried out with or without 10−4 mol/L cnidium lactone. However, the level of p-p38 or p-Akt in groups co-treated with 10−4 mol/L cnidium lactone and inhibitors was still higher than that of incubation within inhibitors alone. There was no significant difference between the total p-38 and total Akt.
Cnidium lactone inhibited the c-Fos and NFATc-1 protein level
In order to further analyze the down-stream gene of MAPK and PI3K-Akt, we tested the expression level of c-Fos and NFATc-1. Results show that the relative expression of c-Fos and NFATc-1 was decreased in cnidium lactone in a dose-dependent manner (). When cnidium lactone was co-cultured (10−4 mol/L) with SB203580 or LY294002, the relative expression of c-Fos and NFATc-1 was decreased when compared with cnidium lactone (10−4 mol/L) alone group.
Discussion
To the best of our knowledge, this study was the first to show that co-culturing with cnidium lactone significantly inhibited RANKL-induced osteoclast formation and bone resorption. In addition, we observed that cnidium lactone efficiently suppressed osteoclastogenesis-related marker genes expression in RANKL-stimulated RAW264.7 cell lines. More important, cnidium lactone suppressed RANKL-induced activation of p38 MAPK and PI3K-Akt induced c-Fos/NFATc1 signalling pathway in RAW264.7.
There were two key factors for osteoclast differentiation, macrophage-colony stimulating factor (M-CSF) and RANKL. In the current study, we only used 10 ng/mL RANKL for inducing osteoclast differentiation. Then, we first identify the optimal dose of cnidium lactone for inhibiting osteoclastogenesis. Li et al. [Citation13] used 10 ng/mL RANKL for inducing RAW264.7 differentiated into osteoclast in the absence of M-CSF. We identified TRAP(+) cells and bone resorption area as the observation target. Results show that cnidium lactone has a beneficial role in reducing the number of TRAP(+) cells and bone resorption area when compared with RANKL group. Zhao et al. [Citation14] revealed that cnidium lactone inhibits RANKL-mediated osteoclastogenesis and prevents bone loss in ovariectomized mice. However, underline mechanism of osthole for bone protective effects was unknown.
We measured six related genes associated with osteoclast differentiation (Acp5, CtsK, Atp6v0d2, Tm7sf4, Oscar and NFATc1). Results show that cnidium lactone inhibited the expressions of these genes. In these experiments, we administered the optimal dose of cnidium lactone (10−4 mmol/L). Next, we co-cultured cnidium lactone with SB203580 or LY294002. We found that when cnidium lactone was co-cultured with SB203580 or LY294002, it significantly reduced the number of TRAP(+) cells. These outcomes indicated that cnidium lactone may make p38 MAPK and PI3K-Akt signalling pathway to inhibit RANKL-mediated osteoclastogenesis.
The PI3K-Akt signalling pathway plays an important role in cell proliferation, growth and metabolism in osteoclasts. P38 MAPK and PI3K-Akt signalling pathway also plays a crucial function in osteoclast differentiation and activation. Lee et al. [Citation15] revealed that PI3K, p38 and extracellular signal-regulated kinase pathways are involved in osteoclast differentiation. Furthermore, Akt induces osteoclast differentiation through regulating the GSK3β/NFATc1 signalling cascade [Citation16]. Our results found that cnidium lactone could inhibit the phosphorylation of p38 and Akt in a dose–response manner.
NFATc-1 is a key transcriptional factor downstream of c-Fos. Recent studies have shown that NFATc1 has a central role in the process of osteoclast differentiation [Citation17]. When NFATc1 was knocked down, TRAP-positive cells could transfer into TRAP-negative cells [Citation18]. NFATc1 regulates the expression of various genes such as TRAP and MMP-9 [Citation19]. The expression of NFATc-1 and c-Fos in cnidium lactone group was lower than control group which was statistically significant. When cnidium lactone was co-cultured with SB203580 or LY294002, the relative expression of NFATc-1 and c-Fos was relatively lower than cnidium lactone group. These findings indicated that NFATc-1 and c-Fos were the downstream genes of p38 MAPK and PI3K-Akt.
Conclusions
In conclusion, the results of the current analysis indicate that cnidium lactone inhibits RANKL-induced osteoclastogenesis and bone resorption through the inhibition of p38 MAPK and PI3K-Akt induced c-Fos/NFATc1 signalling pathways (). Thus, cnidium lactone may be a new strategy for the treatment of osteoporosis.
Disclosure statement
No potential conflict of interest was reported by the authors.
References
- Schroder K. NADPH oxidases in bone homeostasis and osteoporosis. Free Radic Biol Med. 2018;132:67–72.
- Jin G, Wang FF, Li T, et al. Neogambogic acid suppresses receptor activator of nuclear factor kappaB ligand (RANKL)-induced osteoclastogenesis by inhibiting the JNK and NF-kappaB pathways in mouse bone marrow-derived monocyte/macrophages. Med Sci Monit. 2018;24:2569–2577.
- Li C, Yang Z, Li Z, et al. Maslinic acid suppresses osteoclastogenesis and prevents ovariectomy-induced bone loss by regulating RANKL-mediated NF-kappaB and MAPK signaling pathways. J Bone Miner Res. 2011;26:644–656.
- Zhu L, Wei H, Wu Y, et al. Licorice isoliquiritigenin suppresses RANKL-induced osteoclastogenesis in vitro and prevents inflammatory bone loss in vivo. Int J Biochem Cell Biol. 2012;44:1139–1152.
- Kalkan R, Tulay P. The interactions between bone remodelling, estrogen hormone and EPH family genes. Crit Rev Eukaryot Gene Expr. 2018;28:135–138.
- Tella SH, Gallagher JC. Prevention and treatment of postmenopausal osteoporosis. J Steroid Biochem Mol Biol. 2014;142:155–170.
- Xing LZ, Ni HJ, Wang YL. Quercitrin attenuates osteoporosis in ovariectomized rats by regulating mitogen-activated protein kinase (MAPK) signaling pathways. Biomed Pharmacother. 2017;89:1136–1141.
- Li XX, Hara I, Matsumiya T. Effects of osthole on postmenopausal osteoporosis using ovariectomized rats; comparison to the effects of estradiol. Biol Pharm Bull. 2002;25:738–742.
- Tai TW, Su FC, Chen CY, et al. Activation of p38 MAPK-regulated Bcl-xL signaling increases survival against zoledronic acid-induced apoptosis in osteoclast precursors. Bone. 2014;67:166–174.
- Sharma SM, Bronisz A, Hu R, et al. MITF and PU.1 recruit p38 MAPK and NFATc1 to target genes during osteoclast differentiation. J Biol Chem. 2007;282:15921–15929.
- Zhao Z, Ma X, Ma J, et al. Naringin enhances endothelial progenitor cell (EPC) proliferation and tube formation capacity through the CXCL12/CXCR4/PI3K/Akt signaling pathway. Chem Biol Interact. 2018;286:45–51.
- Choi J, Choi SY, Lee SY, et al. Caffeine enhances osteoclast differentiation and maturation through p38 MAP kinase/Mitf and DC-STAMP/CtsK and TRAP pathway. Cell Signal. 2013;25:1222–1227.
- Li F, Sun X, Ma J, et al. Naringin prevents ovariectomy-induced osteoporosis and promotes osteoclasts apoptosis through the mitochondria-mediated apoptosis pathway. Biochem Biophys Res Commun. 2014;452:629–635.
- Zhao D, Wang Q, Zhao Y, et al. The naturally derived small compound Osthole inhibits osteoclastogenesis to prevent ovariectomy-induced bone loss in mice. Menopause (New York, NY). 2018;25:1459.
- Lee SE, Woo KM, Kim SY, et al. The phosphatidylinositol 3-kinase, p38, and extracellular signal-regulated kinase pathways are involved in osteoclast differentiation. Bone. 2002;30:71–77.
- Moon JB, Kim JH, Kim K, et al. Akt induces osteoclast differentiation through regulating the GSK3beta/NFATc1 signaling cascade. J Immunol. 2012;188:163–169.
- Ono T, Nakashima T. Recent advances in osteoclast biology. Histochem Cell Biol. 2018;149:325–341.
- Chang EJ, Kim HJ, Ha J, et al. Hyaluronan inhibits osteoclast differentiation via Toll-like receptor 4. J Cell Sci. 2007;120:166–176.
- Shinohara M, Takayanagi H. Analysis of NFATc1-centered transcription factor regulatory networks in osteoclast formation. Methods Mol Biol (Clifton, NJ). 2014;1164:171–176.