Abstract
The aim of the present study was to investigate the effect of Lycopus lucidus Turcz (LT) on diabetic retinopathy (DR) and its underlying mechanisms. SD rats and human retinal microvascular endothelial cells (HRECs) were applied for establishment DR model. HE and TUNEL staining were used to evaluate the pathological changes and apoptosis of retinal ganglion cells. Additionally, retinal vessels were detected by immunofluorescence staining with CD31 and VEGF. The function of BRB was observed using Evans blue. Moreover, the oxidative stress, inflammation and angiogenesis associated factors were measured respectively. The expression of p38-MAPK/NF-κB signalling proteins were detected by Western blot. The results demonstrated that pathological changes and retinal optic disc cells apoptosis in retinas of diabetic rats, both of which were reduced in the LT-treated group. And LT treatment attenuated the levels of oxidative stress, inflammation and angiogenesis factors. Importantly, the expression levels of p-p38, p-ERK, p-JNK and NF-κB were decreased. After treatment with TNF-α combined with LT, the levels of inflammatory factors were decreased but higher than the negative control. Taken together, the results suggested that LT treatment is of therapeutic benefit by ameliorating oxidative stress, inflammation and angiogenesis of DR via p38-MAPK/NF-κB signaling pathway.
Introduction
Diabetic retinopathy (DR) is one of the most common and serious micro-vascular complications of the eye disease due to diabetes mellitus throughout the world, which greatly attenuates the survival and quality of life of diabetic patients attributing to its high incidence [Citation1,Citation2]. Therefore, it is getting increasingly important and relevant to elucidate the underlying mechanisms of DR in order to develop new therapeutic agents.
An increasing body of literature shows that persistent hyperglycaemia leads to advanced glycation end products (AGEs) accumulation, which subsequently results in oxidative stress and the development of diabetic retinopathy via enhancing the proliferation and migration of endothelial cells and neoangiogenesis [Citation3,Citation4]. It has been well documented that inflammation plays a crucial role in the occurrence and development of DR [Citation5–7]. The persisting inflammation leads to the development of numerous inflammatory factors including tumour necrosis factor-α (TNF-α), interleukin (IL)-6 and IL-1β. These factors cause retinal macular oedema, ischaemia and angiogenesis, which contribute to the development of proliferative DR (PDR) from non-proliferative DR (NPDR) [Citation8,Citation9]. Hence, anti-inflammation and anti-angiogenesis have been considered as potential therapeutic strategies for treating DR [Citation10].
One of the inflammatory markers, TNF-α, is up-regulated in the expression of vascular endothelial growth factor (VEGF). VEGF is a marker for regulatory factor of pathogenic angiogenesis in DR [Citation11]. VEGF acts as an angiogenic and permeability factor through its interaction with the receptors, VEGFR1 (flt-1) and VEGFR2 (KDR/flk-1) [Citation12,Citation13]. VEGF-induced leukocyte adhesion to the retinal vessels is shown to result in the breakdown of blood–retinal barrier (BRB) [Citation14]. Emerging evidence supports the notion that the activation of mitogen-activated protein kinase (MAPKs) is involved the BRB breakdown of the retina in diabetic rats [Citation15–17].
Lycopus lucidus Turcz (LT), a perennial herb, has been used as a traditional medicine widely in Korea, China and Japan due to its well-known effects of reducing inflammation, oedema, menstrual cramps, abdominal pain, rheumatic arthritis and cardiovascular diseases [Citation18]. Increasing evidence supports that LT could suppress high glucose-induced vascular inflammatory process via inhibition of reactive oxygen species (ROS) and NF-κB activation in human umbilical vein endothelial cells [Citation19]. In addition, the aqueous extract of LT was found to reduce streptozotocin-induced diabetic renal damage [Citation20]. In this current study, we aimed to investigate the protective effect of LT on DR.
Material and methods
Preparation of lycopus lucidus turcz (LT) exstract
The LT extract was obtained from Jiangsu Research Institute of Traditional Chinese Medicine. Ten times the amount of distilled water was added to 100 g dried LT and the mixture was incubated for 2 h at 100 °C. Then, eight times the amount of water was added to the following mixture for 1.5 h. After centrifugation, the combined supernatants were concentrated to 100 ml using a rotary vacuum evaporator. The aqueous suspension was processed into a freeze-dried powder using a vacuum freeze dryer. The freeze-dried powder was stored at −80 °C. During experiments, the LT powder was dissolved in deionized distilled water and filtered through a 0.22-μm disk filter.
UPLC/MS analysis
The contents of caffeic acid, luteolin-7-O-β-D glucoside and rosmarinic acid in LT were measured by a Waters Acquity UPLC system coupled with a Waters Micromass Quattro Premier tandem quadrupole mass spectrometer as described in the previous studies [Citation20,Citation21].
Animals and treatment
Male SD rats (Eight-week old, body weight 220–250 g) were purchased from Shanghai SLAC Laboratory Animal Company Limited (Shanghai, China). Rats were raised in a suitable environment with 24 ± 3 °C and 12-h light/dark cycle. All the rats were given access to food and water and were allowed to acclimate to the environment for at least three days before the experiment. All of the study protocols were approved by the Ethics Committee on Animal Experiments of China Medical University. A total of 30 rats were randomly divided into control (n = 5) and DR (n = 25) groups. The control rats were fed ordinary diet, whereas the DR rats were fed high-fat diet for five weeks. Then, 35 mg/kg Streptozotocin (STZ, Sigma, MO) was given to DR rats by intraperitoneal injection to induce diabetes, while control rats were injected with the same volume of natrium citricum buffer solution (0.1 mol/l, pH 4.5). Both the groups were fed their corresponding diets for one week. Blood glucose levels were measured by caudal vein blood. When the glucose concentration reached 16.7 mmol/L or greater, it represent that the DR model was successfully established. These eligible rats were arbitrarily assigned with five groups: model, LT 3 mg/kg, 6 mg/kg, 12 mg/kg and doxium 150 mg/kg (positive control group) groups, with 5 in each one group. After treatment with relative drugs for the extra 18 weeks by gavage, all rats were sacrificed by abdominal aortic method. Subsequently, their retinas were isolated and snap frozen in liquid nitrogen and were stored at −80 °C for further analysis.
Cell culture and treatment
Human retinal microvascular endothelial cells (HRECs) were obtained from The Cell Bank of Type Culture Collection of Chinese Academy of Sciences (Shanghai, China). HRECs cells were cultured in RMPI supplemented with 10% foetal bovine serum at 37 °C in 5% CO2. Cells were treated with different doses of glucose and assigned to six groups: negative control (5 mmol/L), high glucose (HG) model (30 mmol/L), HG + LT low, middle, high doses and calcium dobesilate (CD) groups.
Measurement of body weight and blood glucose
Body weight of the rats was measured every 4 week. Their blood glucose levels were measured at one-week intervals (Roche blood-glucose monitoring system, USA).
Haematoxylin and eosin staining
The retinas tissues were conventionally fixed in 4% paraformaldehyde over night at 4 °C. Then the paraffin-embedded posterior segment which contained the optic disc was sectioned into 5 μm slices. The sections were deparaffinized with graded ethanol together with xylene. Then, followed by staining with haematoxylin and eosin (HE), the sections were dehydrated with graded ethanol and xylene. Finally, the pictures were obtained from OLYMPUS DP73 microscope (Olympus Corp., Tokyo, Japan).
Terminal deoxynucleotidyl transferase dUTP nick end labelling (TUNEL) staining
TUNEL fluorescein kit (Promega, Madison, WI, USA) was used to investigate retinas tissues apoptosis. The paraffin-embedded posterior segment which contained the optic disc were sectioned into 5 μm slices. The retinal sections were further processed according to the manufacturer’s protocols. The apoptosis positive cells in optic disc were observed under OLYMPUS DP73 microscope with high magnification field (200×). Subsequently, five fields were randomly chosen in each slice to calculate the number of apoptosis positive cells. Apoptosis rate was expressed as the percentage of apoptosis positive cells relative to the total cell count.
Immunofluorescence (IF) analysis
To explore the change in neurons, immunofluorescence staining was employed in our study. The retinas were fixed in 4% paraformaldehyde solution at 4 °C for 24 h. Then, 0.1% Triton X-100 in PBS was applied for blocking at room temperature for 2 h. Subsequently, the retinas were incubated with anti-CD31 (1:1000; cat. no. ab24590; abcam; Cambridge; MA; USA) at 4 °C for 24 h. Following three times washing with PBS, the retinas were incubated with DyLight™ 488-conjugated secondary antibody (Thermo Scientific) for 2 h. Immunofluorescence was detected using a fluorescence microscopy (Olympus, Japan).
Measurement the breakdown of blood-retinal barrier (BRB) using Evans blue
To assess the function of BRB, 2% Evans blue was dissolved in PBS was injected into rats intraperitoneally. Blood was extracted from the left ventricle after 2 h. Subsequently, PBS was injected into the left ventricle to perfuse and eliminate the Evans blue dye in blood vessels thoroughly. Then, the retinas were dissected and the weight was measured after completely drying. Next, the retinas were routinely treated with 120 μl formamide at 70 °C for 18 h for the sake of collecting Evans blue dye. Then, the extract was centrifuged at 10,000 g twice for 1 h at 4 °C. The supernatant was collected to measure the absorbance with a spectrophotometer at 620 nm. The concentration of Evans blue dye in extracts was analysed using an Evans blue standard curve. Each sample was measured three times.
ELISA
The contents of TNF-α, IL-6, IL-1β and VEGF in the HRECs culture supernatant and rats’ retinas were measured by ELISA kits using the manufacturer’s instructions. The ELISA kits of TNF-α (F02810), IL-6 (F01310), IL-1α (F01210), IL-1β (F01220) used for HRECs culture supernatant and TNF-α (F16960), IL-6 (F15870), IL-1β (F15810) and VEGF (F17120) applied to rats’ retinas were all purchased from Shanghai Xitang Biotechnology Co., Ltd. (Shanghai, China).
Western blotting
Proteins were extracted from HRECs and rats’ retinas using RIPA lysis buffer (Beyotime, China). BCA protein assay kit (Bio-rad, China) was used for the analysis of proteins concentration. Subsequently, total protein (50 μg) was loaded on 8-12% SDS-PAGE gels, and transferred to PVDF membranes. Then, membranes were blocked with 5% skimmed milk for 90 min at room temperature. Following the incubation with primary antibodies overnight at 4 °C, the goat anti-rabbit horseradish peroxidase-conjugated secondary antibodies (1:1,000; cat. no. A0208; Beyotime Institute of Biotechnology, Haimen, China) were added at room temperature and incubated for 1 h. The immunoreactive bands were measured using ECL (Thermo Scientific). The expression of GAPDH was an endogenic control in all samples. Anti-p38 (1:1000; cat. no. 8690 T), anti-JNK (1:1000; cat. no. 9258 T), anti-p-p38 (1:1000; cat. no. 4511 T), anti-p-ERK (1:1000; cat. no. 4370 T), anti-p-JNK (1:1000; cat. no. 4668 T), anti-NF-κB p65 (1:1000; cat. no. 8242 T) and anti-GAPDH (1:1000; cat. no. 5174S) antibodies were purchased from Cell Signaling Technology (Boston, MA, USA). Anti-ERK (1:1000; cat. no. sc-514302) was obtained from Santa Cruz Biotechnology (CA, USA). Anti-Nox2 (1:1000; cat. no. ab31092) and anti-Nox4 (1:1000; cat. no. ab154244) were the products of Abcam Company (Cambridge, UK). The parallel test was repeated for three times.
Quantitative reverse transcription-PCR(RT-qPCR)
After treatment, the TRIzol Reagent (Invitrogen, Carlsbad, CA, USA) was employed to extract the total RNA from the HRECs of each group. Subsequently, RNA was reverse-transcribed to cDNA using the QuantiTect RT kit (Qiagen) following the manufacturer’s instructions. Real-time PCR was performed using a System 7500 instrument (Applied Biosystems, Carlsbad, CA, USA), and the following thermocycling conditions for PCR was used: 95 °C for 5 min and then 40 cycles of 94 °C for 15 s, 55 °C for 20 s, 72 °C for 20 s, followed by 72 °C for 7 min. Relative expression of target genes were normalized to GAPDH, which was the endogenous control. The primer sequences for qPCR were as follows: VEGF, forward 5′- GGGCCTCGGTTCCAGAAG-3′ and reverse 5′- AACTTCACCACTTCATGGGCT-3′; FLT-1, forward 5′-CAAGGGACTCTACACTTGTC-3′ and reverse 5′-CCGAATAGCGAGCAGATTTC-3′; KDR, forward 5′-CACCATGCAGACGCTGACAT-3′ and reverse 5′-TCTAGCTGCCAGTACCATTGGA-3′; GAPDH, forward 5′-AACGGATTTGGTCGTATTG-3′ and reverse 5′-GGAAGATGGTGATGGGATT-3′. Expression levels were analysed following the 2-ΔΔCq calculation method.
Statistical analysis
All statistical analyses were conducted using SPSS 14.0 software (Chicago, IL). All experimental results are expressed as mean ± SD. Statistical comparisons were made by two-tailed Student’s t-test or one-way analysis of variance (ANOVA). p < .05 was considered statistically significant.
Results
After treatment with LT, there was an obvious decrease in blood glucose levels and increase in body weight compared to untreated diabetic rats. These results indicate that LT treatment may improve blood glucose levels and body weight in diabetic rats.
LT retards the apoptosis of diabetic rats’ retinal ganglion cells
The results displayed that the blood glucose level of model group was significantly increased while body weight was decreased compared to control group (), which indicated the successful DR rat model was established. In order to evaluate the pathological changes, HE staining and TUNEL staining were employed to measure the apoptosis of diabetic rats’ retinal ganglion cells. The HE staining results demonstrated that retinal ganglion cells were neatly arranged accompanied with the smooth retinal surface and complete structure in every layer in the control group (). However, the retina was thin and vasodilated and the arrangement of retinal ganglion cells was disorganized in the model group. After treatment with LT at doses of 12 mg/kg and 6 mg/kg, there was an obvious decrease of the number of disorganized retinal ganglion cells. The retinal vascular networks were outlined by DAPI counterstaining of cell nuclei. From the results, no apoptotic cell death was detected in the control group, whereas apoptotic retinal ganglion cells were notably increased in the retinas of model group (). After treatment with different doses of LT, the number of apoptotic cell was significantly reduced. Taken together, these results indicate that LT decreases the apoptosis of retinal ganglion cells in diabetic rats.
Figure 1. Analysis of body weight, blood glucose level and HE staining of retinal ganglion cell in STZ-induced DR rats. (A) Bodyweight; (B) blood glucose level; (C) Representative pictures of H&E staining of retinas. ***p < .001: model versus control; ###p < .001: 12 mg/kg versus model; △△p < .01, △△△p < .001: 6 mg/kg versus model.
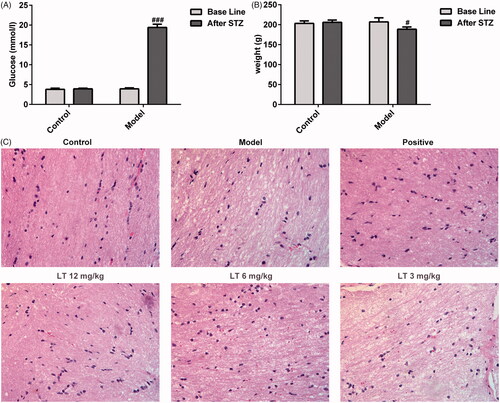
Figure 2. The apoptosis of retinal ganglion cell and the expression of angiogenesis remarks in STZ-induced DR rats. (A) TUNEL staining of retinal ganglion cell and (B) its quantified. (C) Immunofluorescence staining of retinas with CD31, and the enlarged partial view of CD31-stained retina was showed (original magnification ×100). (D) The expression of CD31 was qualified. (E) Immunofluorescence staining of retinas with VEGF, and the enlarged partial view of VEGF-stained retina was showed (original magnification ×100). (F) The expression of VEGF was qualified. ###p < .001 versus control; ***p < .001 versus model; △△p < .01, △△△p < .001 versus positive.
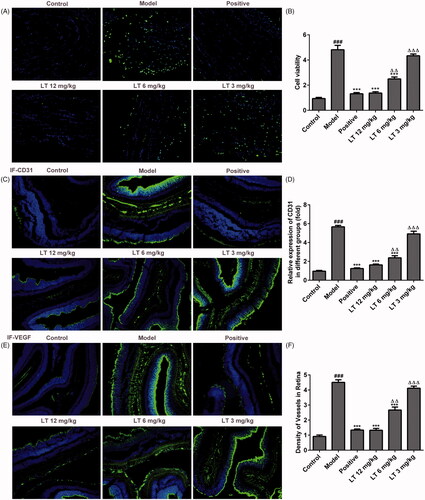
LT decreased capillary permeability of STZ-induced DR rats
The levels of angiogenic marker proteins CD31 and VEGF, measured by IF staining, were markedly increased in the retinas of diabetic rats compared to that of the normal rats. However, the expression of CD 31 and VEGF protein markers were significantly reduced after treatment with LT (). At the same time, the level of VEGF in vitreum has the same results as above (). In addition, DR is characterized by increased vascular permeability. In order to investigate the retinal disruption, Evans blue leakage assay was employed to measure the function of BRB (). The results revealed that the BRB permeability in the model group was elevated compared to that of the control group. After treatment with LT, especially in the LT 6 mg/kg group, the BRB permeability was lower than the model group. These results denote that LT treatment could inhibit the disruption of BRB in DR.
Figure 3. The levels of capillary permeability, anti-inflammation and antioxidant. (A) The level of VEGF in vitreum DR rats. (B) BRB breakdown was detected by using Evans blue leakage assay in STZ-induced DR rats. The levels of (C) TNF-α (D) IL-6 (E) IL-1β in serum of STZ-induced DR rats were measured by Elisa. (F) The level of LDH in HRECs culture supernatant was evaluated using Elisa. (G–I) The expression levels of Nox2 and Nox4 in HRECs were measured by Western blot. ###p < .001 versus control; **p < .01, ***p < .001 versus model; △p < .05, △△p < .01, △△△p < .001 versus positive.
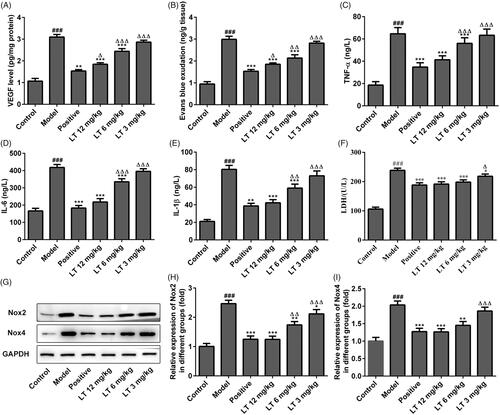
LT ameliorated the oxidative stress
We examined the oxidative stress factor LDH levels in high glucose treatment HRECs. As shown in , the LDH content was notably increased after HRECs being induced by high glucose compared to the control group. However, the LDH content was decreased dose-dependently in LT treatment group. At the same time, the protein expression levels of Nox2 and Nox4 were reduced after treatment with LT (). These data suggest that LT treatment may reduce the oxidative stress due to diabetes mellitus.
LT attenuated the inflammatory responses induced by oxidative stress
To investigate the inflammatory responses induced by oxidative stress, the levels of TNF-α, IL-6 and IL-1β in high glucose induced HRECs and serum of DR rats were measured. The results revealed that the TNF-α, IL-6 and IL-1β levels were lowered significantly in LT treatment group compared to the model group in rats’ serum () and HRECs culture supernatant (). These results demonstrate that LT might reduce the release of inflammatory cytokines.
Figure 4. The levels of anti-inflammation, angiogenesis and p38-MAPK/NF-κB signalling pathway in HRECs. The levels of (A) TNF-α (B) IL-6 (C) IL-1β in HRECs were measured by Elisa. The mRNA expression levels of (D) VEGF (E) FLT-1 and (F) KDR were measured by RT-qPCR. (G-K) The protein expression levels of p-p38, p-ERK, p-JNK and NF-κB p65 were evaluated using Western blot. ###p < .001 versus control; *p < .05, **p < .01, ***p < .001 versus model; △p < .05, △△p < .01, △△△p < .001 versus positive.
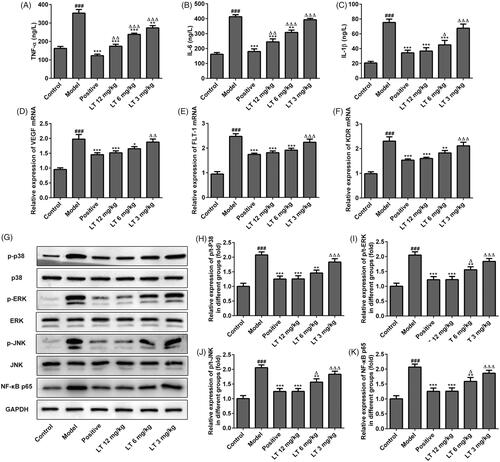
LT impedes the angiogenesis induced by inflammation
As exhibited in , the mRNA expression levels of VEGF, FLT-1 and KDR were elevated in high glucose-induced HRECs, whereas these levels were reduced dose-dependently after LT treatment. These results show that LT inhibits the generation of angiogenic factors and the progression of DR both in vitro which was in accordance with the study in vivo.
LT ameliorates the DR from p38-MAPK signalling
To further investigate the underlying protective mechanism of LT in DR, the expression levels of p38, ERK, JNK, p-p38, p-ERK, p-JNK and NF-κB were measured by Western blot. We found that the p-P38, p-ERK, p-JNK and NF-κB expression levels were increased in the model group, while these inflammatory markers were reduced in LT-treated group (). In addition, after treatment with TNF-α combined with LT 6 mg/kg in high glucose-induced HRECs, the levels of IL-6 and IL-1β were significantly reduced compared to the model group but higher than the positive control (). These results indicate that the protective effects of LT is associated with the inhibition of p38-MAPK/NF-κB signalling.
Figure 5. The levels of anti-inflammatory factors after treatment with TNF-α combined with LT in high glucose induced HRECs. The level of (A) TNF-α (B) IL-6 (C) IL-1α and (D) IL-1β were measured by Elisa. ###p < .001 versus control; ***p < .001 versus model; △△p < .01, △△△p < .001 versus LT 6 mg/kg.
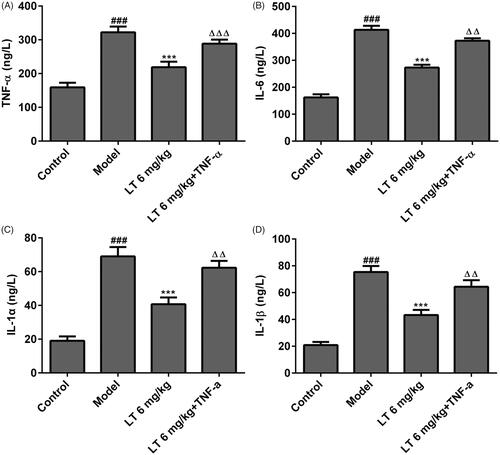
Discussion
DR is a microvascular complication of diabetes that affects the blood vessels of retina, leading to vision loss [Citation22]. There are many anti-inflammatory and antiangiogenic agents are deemed as the underlying treatment of DR [Citation23,Citation24]. Emerging evidence supports the notion of the anti-inflammatory effects of LT and its potential application in the improvement of streptozotocin-induced diabetic renal damage [Citation20,Citation21]. In our study, our results show that LT reduces the inflammatory responses and angiogenesis in DR, which in turn inhibits the progression of DR via MAPK signalling.
It has been well documented that persistent hyperglycaemia in diabetic patients lead to the AGEs accumulation and result in oxidative stress and inflammatory responses. The presence of oxidative stress increased the levels of lipid peroxidation end products MDA, Nox2 and Nox4. In addition, the increasing oxidative stress induce the secretion of pro-inflammatory cytokines, which leads to leukostasis and further promotes breakdown of BRB. In this study, TNF-α, IL-6 and IL-1β levels in HRECs supernatant and DR rat serum were significantly increased, which correlates with the previous research [Citation25,Citation26].
In recent years, accumulating evidences demonstrate that constant inflammatory stimulation of retina plays a critical role in the pathogenesis of DR, which contributes to break down of BRB and accelerates the development of oedema and neovascularization [Citation27,Citation28]. VEGF, a primary angiogenic factor in DR, promotes neovascularization and vascular leakage in retina through its interaction with the FLT-1 and KDR receptors [Citation29]. Therefore, several anti-VEGF drugs agents are reported as potential therapeutic agents of DR alone or as an assist participating in other therapies [Citation30,Citation31]. Therefore, there are several anti-VEGF drugs under investigation for treating DR including macugen and avastin [Citation32]. The previous studies reported that CD31 is an angiogenic marker protein that contributes to neovascularization in DR rats’ retina [Citation33]. In our study, the levels of VEGF in vitreous, retinas and serum from diabetic rats were apparently increased. In vitro, the mRNA expression of VEGF, FLT-1 and KDR in HRECs were elevated significantly. Further results suggest that the number of vessels in DR rats was increased whereas that of in the LT-treated group were decreased especially in LT 6 mg/kg group which could be seen from the expression of CD31. Our results indicate that LT inhibits retinal angiogenesis via inhibiting VEGF-signalling pathway and therefore reduces the effects of DR in diabetic rats.
Based on the well-known report that apoptosis of retinal ganglion cells is increased in the retinas of diabetic rats [Citation34]. The HE staining and TUNEL staining confirmed the apoptosis of ganglion cells in our study, which supports the findings of previous studies [Citation35]. The p38-MAPK signalling is an important pro-apoptosis pathway, and several studies have shown the involvement of this pathway in the development of DR [Citation36,Citation37]. NF-κB signalling as a downstream signal molecules of MAPK signalling was implicated in the occurrence of inflammation in DR. Our study shows that the expression levels of p-p38, p-ERK, p-JNK and NF-κB were increased both in vitro and in vivo of DR models whereas LT treatment reduces these expression levels in both the models. To further explore the protective mechanisms of LT on DR, TNF-α combined with LT 6 mg/kg was added to the high glucose treated HRECs. Our results show that the levels of IL-6 and IL-1β were decreased as compared to the high glucose group but higher than the negative control, which shows LT inhibited inflammation.
Conclusion
To our knowledge, the present study is the first time to investigate the protective effects of LT against DR. From out study, we found that LT treatment may be useful in attenuating the progression of DR by attenuating the inflammation and retinal angiogenesis of DR via p38-MAPK/NF-κB-signalling pathway. While further studies need to be done to further investigate the protective effects of LT on DR patients, our study provides experimental and scientific evidences for the potential of using LT as a therapeutic agent in clinical DR patients.
Disclosure statement
The authors declare no conflict of interest.
Availability of data and materials
The analysed data sets generated during the present study are available from the corresponding author on reasonable request.
Additional information
Funding
References
- Abu El-Asrar AM, Midena E, Al-Shabrawey M, et al. New developments in the pathophysiology and management of diabetic retinopathy. J Diabetes Res. 2013;2013:1.
- Robinson R, Barathi VA, Chaurasia SS, et al. Update on animal models of diabetic retinopathy: from molecular approaches to mice and higher mammals. Dis Model Mech. 2012;5:444–456.
- Stone JR, Collins T. The role of hydrogen peroxide in endothelial proliferative responses. Endothelium. 2002;9:231–238.
- Heng LZ, Comyn O, Peto T, et al. Diabetic retinopathy: pathogenesis, clinical grading, management and future developments. Diabet Med. 2013;30:640–650.
- Adamis AP. Is diabetic retinopathy an inflammatory disease? Br J Ophthalmol. 2002;86:363–365.
- Kastelan S, Tomic M, Gverovic Antunica A, et al. Inflammation and pharmacological treatment in diabetic retinopathy. Mediators Inflamm 2013;2013:8.
- Rangasamy S, McGuire PG, Franco Nitta C, et al. Chemokine mediated monocyte trafficking into the retina: role of inflammation in alteration of the blood-retinal barrier in diabetic retinopathy. PLoS One. 2014;9:e108508.
- Wilkinson CP, Ferris 3rd FL, Klein, RE, et al. Proposed international clinical diabetic retinopathy and diabetic macular edema disease severity scales. Ophthalmology. 2003;110:1677–1682.
- Tang J, Kern TS. Inflammation in diabetic retinopathy. Prog Retin Eye Res. 2011;30:343–358.
- Gologorsky D, Thanos A, Vavvas D. Therapeutic interventions against inflammatory and angiogenic mediators in proliferative diabetic retinopathy. Mediators Inflamm. 2012;2012:1.
- Aldebasi YH, Rahmani AH, Khan AA. The effect of vascular endothelial growth factor in the progression of bladder cancer and diabetic retinopathy. Int J Clin Exp Med. 2013;6:239–251.
- Ferrara N, Gerber HP, LeCouter J. The biology of VEGF and its receptors. Nat Med. 2003;9:669–676.
- Tammela T, Zarkada G, Wallgard E, et al. Blocking VEGFR-3 suppresses angiogenic sprouting and vascular network formation. Nature. 2008;454:656–660.
- Joussen AM, Poulaki V, Qin W, et al. Retinal vascular endothelial growth factor induces intercellular adhesion molecule-1 and endothelial nitric oxide synthase expression and initiates early diabetic retinal leukocyte adhesion in vivo. Am J Pathol. 2002;160:501–509.
- Liu X, Ye F, Xiong H, et al. IL-1beta induces IL-6 production in retinal Muller cells predominantly through the activation of p38 MAPK/NF-kappaB signaling pathway. Exp Cell Res. 2015;331:223–231.
- Zhang X, Lai D, Bao S, et al. Triamcinolone acetonide inhibits p38MAPK activation and neuronal apoptosis in early diabetic retinopathy. CMM. 2013;13:946–958.
- Cai Y, Li W, Tu H, et al. Curcumolide reduces diabetic retinal vascular leukostasis and leakage partly via inhibition of the p38MAPK/NF-kappa B signaling. Bioorg Med Chem Lett. 2017;27:1835–1839.
- Dzau VJ, Braun-Dullaeus RC, Sedding DG. Vascular proliferation and atherosclerosis: new perspectives and therapeutic strategies. Nat Med. 2002;8:1249–1256.
- Lee YJ, Kang DG, Kim JS, et al. Lycopus lucidus inhibits high glucose-induced vascular inflammation in human umbilical vein endothelial cells. Vascul Pharmacol. 2008;48:38–46.
- Yao Y, Yang J, Wang D, et al. The aqueous extract of Lycopus lucidus Turcz ameliorates streptozotocin-induced diabetic renal damage via inhibiting TGF-beta1 signaling pathway. Phytomedicine. 2013;20:1160–1167.
- Lu YH, Huang JH, Li YC, et al. Variation in nutritional compositions, antioxidant activity and microstructure of Lycopus lucidus Turcz. root at different harvest times. Food Chem. 2015;183:91–100.
- Elsherbiny NM, Ahmad S, Naime M, et al. ABT-702, an adenosine kinase inhibitor, attenuates inflammation in diabetic retinopathy. Life Sci. 2013;93:78–88.
- Jeganathan VS. Anti-angiogenesis drugs in diabetic retinopathy. Curr Pharm Biotechnol. 2011;12:369–372.
- Kumar B, Gupta SK, Saxena R, et al. Current trends in the pharmacotherapy of diabetic retinopathy. J Postgrad Med. 2012;58:132–139.
- Demircan N, Safran BG, Soylu M, et al. Determination of vitreous interleukin-1 (IL-1) and tumour necrosis factor (TNF) levels in proliferative diabetic retinopathy. Eye (Lond). 2006;20:1366–1369.
- Kowluru RA, Odenbach S. Role of interleukin-1beta in the development of retinopathy in rats: effect of antioxidants. Invest Ophthalmol Vis Sci. 2004;45:4161–4166.
- Noda K, Nakao S, Ishida S, et al. Leukocyte adhesion molecules in diabetic retinopathy. J Ophthalmol. 2012 ;2012:1.
- Rangasamy S, McGuire PG, Das A. Diabetic retinopathy and inflammation: novel therapeutic targets. Middle East Afr J Ophthalmol. 2012;19:52–59.
- Spranger J, Pfeiffer AF. New concepts in pathogenesis and treatment of diabetic retinopathy. Exp Clin Endocrinol Diabetes. 2001;109:S438–S50.
- Salam A, Mathew R, Sivaprasad S. Treatment of proliferative diabetic retinopathy with anti-VEGF agents. Acta Ophthalmol. 2011;89:405–411.
- Nicholson BP, Schachat AP. A review of clinical trials of anti-VEGF agents for diabetic retinopathy. Graefes Arch Clin Exp Ophthalmol. 2010;248:915–930.
- Tremolada G, Del Turco C, Lattanzio R, et al. The role of angiogenesis in the development of proliferative diabetic retinopathy: impact of intravitreal anti-VEGF treatment. Exp Diabetes Res. 2012;2012:1.
- Abu El-Asrar AM, Mohammad G, Allegaert E, et al. Matrix metalloproteinase-14 is a biomarker of angiogenic activity in proliferative diabetic retinopathy. Mol Vis. 2018;24:394–406.
- Asnaghi V, Gerhardinger C, Hoehn T, et al. A role for the polyol pathway in the early neuroretinal apoptosis and glial changes induced by diabetes in the rat. Diabetes. 2003;52:506–511.
- Chao HM, Hu L, Cheng JM, et al. Chi-Ju-Di-Huang-Wan protects rats against retinal ischemia by downregulating matrix metalloproteinase-9 and inhibiting p38 mitogen-activated protein kinase. Chin Med. 2016;11:39.
- Xie MS, Zheng YZ, Huang LB, et al. Infliximab relieves blood retinal barrier breakdown through the p38 MAPK pathway in a diabetic rat model. Int J Ophthalmol. 2017;10:1824–1829.
- Li Y, Bai YJ, Jiang YR, et al. Apelin-13 is an early promoter of cytoskeleton and tight junction in diabetic macular edema via PI-3K/Akt and MAPK/Erk signaling pathways. Biomed Res Int. 2018;2018:3242574.