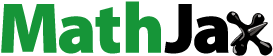
Abstract
Neurotoxicity of local anesthetics is often reported in the clinic, more and more people pay attention to them. CaMKIIβ, a subtype of CaMKII, is detected in the central nervous system. Previous study found that CaMKIIβ mRNA are up-regulated in DRG neurons treated with ropivacaine hydrochloride, as well as inhibition of Cav3.2 and Cav3.3 expression can improve the local anesthetics neurotoxicity. In this study, we observed the effect of CaMKIIβ on neurotoxicity injury induced by ropivacaine hydrochloride with DRG cell in vitro. We first constructed the pAd-shRNA-CaMKIIβ-DRG to inhibit CaMKIIβ mRNA expression and detected the cell viability, cell apoptosis rate, CaMKIIβ, Cav3.2 and Cav3.3 expression. The results showed that ropivacaine hydrochloride caused the DRG cell injury with cell viability decreased and cell apoptosis rate increased, CaMKIIβ, Cav3.2 and Cav3.3 expression up-regulated. Interestingly, inhibition of CaMKIIβ expression protected the DRG cell from the neurotoxicity injury induced by ropivacaine hydrochloride, increased the cell viability and decreased the apoptosis rate, as well as inhibition of CaMKIIβ expression down-regulated Cav3.2 and Cav3.3 expression. In other words, CaMKIIβ is involved with the DRG injury induced by ropivacaine hydrochloride. Inhibition CaMKIIβ expression improved DRG injury, increased the cell viability and decreased cell apoptosis rate.
Introduction
CaMKII is a multifunctional protease, which is widely distributed in nerve tissue [Citation1–3]. It plays an important role in the pathophysiological process such as cerebral ischemic injury, oxidative stress, excitatory amino acid toxicity and calcium overload [Citation4–6]. CaMKII can be divided into four subtypes: alpha, beta, gamma and delta (α,β,γ,δ). Previous studies have shown that the above four subtypes are expressed in dorsal root ganglion (DRG) neurons of rats [Citation7,Citation8]. Moreover, all those subtypes of CaMKII mRNA expression are up-regulated in DRG neurons treated with ropivacaine hydrochloride [Citation7]. At the same time, knockdown of CaMK IIγmRNA can alleviate the DRG neurons injury induced by ropivacaine hydrochloride. On the contrary, up-regulating the expression of CaMK IIγ can aggravate the DRG injury. Those data suggest that CaMK IIγ is closely related to the nerve injury induced by ropivacaine hydrochloride [Citation8].
CaMKIIβ, a subtype of CaMK II, is detected in the central nervous system [Citation9,Citation10]. It is closely related to the formation of synapses, learning and memory. Our previous study found that ropivacaine hydrochloride could up-regulate the expression of CaMKIIβmRNA in DRG cells [Citation7]. Similar to the low voltage dependent calcium channel, T-type calcium channel plays a key role in the local anesthetics neurotoxicity [Citation11–13]. Knockdown of the T-type calcium channel improves the neuron injury induced by the local anesthetics [Citation14]. Cav3.2 and Cav3.3 are the dominating subtypes of T-type calcium channel in the DRG neurons [Citation15]. CaMKIIγ, Cav3.2 and Cav3.3 are involved in nerve injury induced by local anesthetics. Moreover, calcium signaling regulated by CaMKIIγ, Cav3.2 and Cav3.3 are closely related with the local anesthetics neurotoxicity. CaMKII can regulate T-type calcium channel, activation of CaMKII up-regulates T-type calcium expression, as well as inhibition of CaMKII down-regulates T-type calcium channel expression [Citation16].
Therefore, we speculate that CaMKIIβ participates in the neurotoxicity of local anesthetics through Cav3.2 and Cav3.3 in DRG neurons. In this study, we investigated the effects of knockdown CaMKIIβ mRNA expression on the DRG neurotoxicity induced by ropivacaine hydrochloride, as well as detected the Cav3.2 and Cav3.3 expression of the DRG neurons with CaMKIIβ mRNA knockdown.
Materials and methods
Isolation of DRG neurons of rats
The protocols for the animal use were approved by the Ethics Committee of Affiliated Foshan Hospital of the Southern medical University. One-day-old SD rats (Animal Laboratory Center, the Southern Medical University of China) were anaesthetized with ketamine. Spinal cord and dorsal root ganglia were exposed. Dorsal root ganglia were separated from the intervertebral foramen. After separation, dorsal root ganglia were moved into 15 ml centrifugal tube for digestion with 0.125% trypsin at 37 °C for 20 min. After digestion, the dorsal root ganglia were added to neurobasal medium (containing 4.5 g/L-glucose, 2 mmol/L-glutamine, 1% FBS, 20 ml/L-27 additive, 10 μg/ml NGF, penicillin 100 U/ml, streptomycin 100 μg/ml) and filtered by 400 mesh stainless steel mesh. The cell suspension was inoculated into the cell culture plate. The cell density was 1–2 × 105/ml. After 48 h of incubation at 37 °C and 5% CO2 incubator, the cell culture medium was replaced and 5 mM cytarabine was added to inhibit the proliferation of non-neuron cells. After 96 h, the cell suspension was replaced by cytarabin-free culture medium. The medium was changed every 3 days.
Construction of pAd-shRNA-CaMKIIβ-DRG
In order to inhibit the mRNA expression of CaMKIIβ in dorsal root ganglion neurons, we constructed pAd-shRNA-CaMKIIβ-DRG neurons. In brief, shRNA primers were designed according to the rat CaMKIIβ gene (NM_00104232354.1). The primer sequence was as the following: 5′-TTTGCCAGCTATACGAGGATATTTTCAAGACGAATACCTCGTATAGCTGGTTTTTTG-3′;5′-AGCTCAAAAAACCAGCTATACGAGGATATTCGTCTTGAA AATACCTCGTATAGCTGG-3′.
The sequences underlined are the interference sequence. After it was annealed, the double chain primers were connected with pYr-1.1 vector. After sequenced correctly, the interfering sequence was constructed into pAd/PL-DEST adenovirus expression vector to form pAd-shRNA products. The pAd-shRNA was transfected into HEK 293 cells by Lipo 2000 (“Invitrogen Company”, CA, United States) system to amplify the virus and infect DRG neurons. Real-time PCR and WB were used to identify the expression of CaMK II beta in pAd-shRNA-CaMK II beta-DRG neurons. Primers annealed to form double chains with pYr-1.1 vectors were connected, the conjugates were transformed and sequenced correctly. The interfering sequence was constructed into pAd/PL-DEST adenovirus expression vector. The pAd-shRNA was transfected into HEK 293 cells by Lipo 2000 system to amplify the virus and infect DRG neurons to construct pAd-shRNA-CaMKIIβ-DRG neurons. Real-time PCR and WB were used to identify the expression of CaMKIIβ in pAd-shRNA-CaMKIIβ-DRG neurons.
Experimental protocol
After isolation, the DRG cells were cultured in vitro. The pAd-shRNA-CaMKIIβ-DRG cells and empty vector-DRG cells also were cultured in vitro. There were six groups in this experiment: normal DRG neurons(normal group), vector-DRG neurons (vector group), pAd-shRNA-CaMKIIβ-DRG neurons (pAd-shRNA-CaMKIIβ-DRG group), normal DRG neurons treated with 3 mM ropivacaine hydrochloride for 4 h (normal + R group), vector-DRG neurons treated with 3 mM ropivacaine hydrochloride for 4 h (vector + R group), pAd-shRNA-CaMKIIβ-DRG neurons treated with 3 mM ropivacaine hydrochloride for 4 h (pAd-shRNA-CaMKIIβ-DRG + R group). After the neurons in every group were treated with or without 3 mM ropivacaine hydrochloride, the cell viability, ultrastructural changes of cells, cell apoptosis rate, CaMKIIβ, Cav3.2 and Cav3.3 expression were detected to evaluate effects of CaMKIIβ on the neurotoxicity induced by ropivacaine hydrochloride. As a control, the pH value of cell culture medium in each group was 4.0–6.0, which was consistent with that of ropivacaine hydrochloride.
Cell viability detected
All neurons in every group were seeded on 96-well plate and treated with or without 3 mM ropivacaine hydrochloride for 4 h. Cell viability was measured by MTT method. In brief, 4 h after treated with or without ropivacaine hydrochloride, the neurons in every group were incubated with normal culture medium and MTT solution (5 mg/ml) were added to the medium to incubate the neurons for 4 h, discarding supernatant, adding DMSO 150 µl to every hole after full shake, detecting absorbance at 570 and 630 nm wavelength (OD570 and OD630), respectively. The difference of absorbance between (OD570 and OD630) were calculated to evaluate the cell viability. The difference of the neurons in normal group were regarded as 100%, cell survival rate was calculated by the ratio of the neurons in other groups to those in normal group. The mean value was calculated by taking 6 holes in every group.
Cell apoptotic rate detected
Neurons in every group were seeded on 24-well plates and treated with or without 3 mM ropivacaine hydrochloride for 4 h. Cells were digested and collected. Apoptotic rate was detected by flow cytometry according to the instructions of apoptotic detection kit. In short, the cells in every group were collected and incubated with Annexin-V antibody (1:20) for 15 min at room temperature and under light shielded. After the cell resuspension, propidium iodide (PI, 50 μg/ml) was added to the cells to incubate for 30 min at room temperature and under light shielded. Then, 190 µl × binding buffer was added to the supernatant. The apoptotic rate was detected by flow cytometry after 1 × binding buffer suspension. The apoptotic rate detection was repeated three times in every group. The average value was regarded as the cell apoptotic rate of every group.
Neurons change observed with electron microscopy
After treated with or without 3 mM ropivacaine hydrochloride for 4 h, the cells were collected, fixed with 2.5% glutaraldehyde and rinsed with PBS at 4 °C for 3 times. Then, the cells were fixed with 1% osmium tetroxide for 15 min, dehydrated with 50, 70 and 90% acetone, respectively and overnight at room temperature with embedding agent. The cell mass was moved into the center of the capsule bottom. Embedding agent was filled to capsule. Then, the capsule was baked in an oven at 60 °C for 2 h to solidify. The samples were cut with 1 µm thickness on the slicing machine and stained with hematoxylin-eosin method to determine the location of ultra-thin sections. Ultra-thin slices with 50–70 nm were cut on the ultra-thin slice machine. The slices were stored in a drying vessel for dyeing and cleaning with sodium acetate and lead citrate. After dyeing, the slices were dried and the mitochondrial structure of neurons was observed under transmission electron microscopy (HITACHI, HT7700, Tokyo, Japan).
Real-time PCR detected CaMK II β, Cav3.2 and Cav3.3 mRNA expression
After treated with or without ropivacaine hydrochloride, the cells were collected and total RNA were extracted. In brief, Trizol was used to lyse the cells at 44 °C, chloroform was added to the lysed cells concentrated with 14,000 rpm at 4 °C for 15 min. The upper RNA was extracted and precipitated with equal volume isopropanol. After centrifuged, the supernatant was discarded and the precipitate was washed with 70% ethanol 500 µl and centrifuged again. The precipitate was the total RNA. It was dried in air and dissolved with 30 ml nuclear enzyme free water. RNA 5 µg, 2 M dNTP 5 µl, random primer 1 µl and DEPC water were added to 37 µl volume to incubate at 65 °C for 5 min. 5 × first stand buffer 10 µl, 0.1 M DTT 2 µl, mLv reverse transcriptase 1 µl and DEPC water were added to 50 µl at 42 °C for 1 h, 70 °C for 15 min. The product DNA was used for PCR reaction. 5 µl DNA product, 6 µl sybergreen and 10 pmol/lCaMKIIβ, Cav3.2 and Cav3.3 primer upstream and downstream primer 0.5 µl (synthesized by Jinsirui Ltd., Nanjing, China, ), respectively. The fluorescence signals were collected and the amplification and melting curves were drawn. The expression of genes was expressed by 2−ΔCt (Ct stands for cycle threshold). ΔCt = [Ct (target gene in the test group)−Ct (β-actin)] − [Ct (target gene in the control group)−Ct (β-actin)].
Table 1. The primer sequence of CaMKIIβ, Cav3.2, Cav3.3 mRNA.
CaMKIIβ, Cav3.2 and Cav3.3 protein expression detected by Western blotting
After treated with or without ropivacaine hydrochloride, 200 ml lysate was added into each pore to incubate at 4 °C for 30 min. The total protein was collected. 20 µg proteins from every sample were mixed with 4 × SDS buffer solution. After boiling for 5 min, the samples were transferred to 4% concentrated gel and 12% separated gel for electrophoresis. According to the marker, the target protein was transferred to PVDF membrane and was sealed at room temperature for 1 h. The target band in PVDF membrane was incubated with CaMKIIβ, Cav3.2, Cav3.3 proteins antibody (1:200) (Sigma Company, St. Louis, MO, USA) at 4 °C and overnight, respectively. The second antibody (1:1000) was added to incubate at room temperature for 1 h. The target PVDF membrane was incubated with chemiluminescent reagent for 1 min and was quickly wrapped with a fresh-keeping film and exposed to KoDak X-film, Kodak Corp., New York, USA in a dark box. X-film was scanned and recorded after developing and fixing. Quantity one image analysis software (Bio-Rad Co., CA, USA) was used to analyze the target band. The β-actin band was regarded as 1. The ratio of the target protein and β-actin was the expression level.
Statistical analysis
The data are expressed as the mean ± SD. Factorial design was adopted for the statistical analysis. One-way analysis of variance (one-way ANOVA) was used for comparisons among groups and the LSD method was used for multiple comparisons.
Results
Cell viability
Compared with the cells in normal group, the cell viability of the cell in vector group and pAd-shRNA-CaMKIIβ-DRG group showed no significant difference. However, the cell viability of the cells treated with ropivacaine hydrochloride in normal + R group, vector + R group and pAd-shRNA-CaMKIIβ-DRG + R group significantly decreased, (49.8 ± 4.3)%,(48.8 ± 3.8)% and(65.1 ± 6.0)%, respectively. Compared with normal + R and vector + R group, the cell viability of the cells in pAd-shRNA-CaMKIIβ-DRG + R group increased, see .
Cell apoptotic rate
There was no significant difference in apoptotic rate among the cells in normal group, vector group and pAd-shRNA-CaMKIIβ-DRG group. Compared with the cells in normal group, the apoptotic rate of the cells after treated with ropivacaine hydrochloride significantly increased. The apoptotic rates of the cells in normal + R, vector + R group and pAd-shRNA-CaMKIIβ-DRG + R group were (39.0 + 4.1)%, (39.1 + 3.5)% and (26.6 + 2.9)%, respectively. However, the cell apoptotic rate of the cell in pAd-shRNA-CaMKIIβ-DRG + R group decreased compared with those in normal + R group, see .
Changes of the cell observed with transmission electron microscope
There was a lot of neurofilament in the cells in normal group, vector group and pAd-shRNA-CaMKIIβ-DRG group, as well as the shape of mitochondria was regular with intact mitochondrial ridge and the cytoplasm was abundant. There was no obvious difference among the cells in normal group, vector group and pAd-shRNA-CaMKIIβ-DRG group with the transmission electron microscope observation. However, those cells treated with ropivacaine hydrochloride in normal + R group, vector + group and pAd-shRNA-CaMKIIβ-DRG + R group showed organelle vacuolation, mitochondrial swelling and mitochondrial ridge breakage. The degree of cell damage in pAd-shRNA-CaMKIIβ-DRG + R group was less than that in normal + R group and vector + R group, .
CaMKIIβ, Cav3.2 and Cav3.3 mRNA expression
CaMKIIβ mRNA
Compared with normal group, there was no significant difference in expression of CaMKIIβ of the cell in vector group. The expression of CaMKIIβ in pAd-shRNA-CaMKIIβ-DRG group decreased. After ropivacaine hydrochloride treatment, the expression of CaMKIIβ in normal + R group and vector + R group increased significantly. Compared with pAd-shRNA-CaMKIIβ-DRG group, the expressions of normal + R group and vector + R group and pAd-shRNA-CaMKIIβ-DRG + R group increased, However, the expressions of pAd-shRNA-CaMKIIβ-DRG + R group decreased significantly compared with the cells in normal + R group, see .
Cav3.2 mRNA
Compared with the cells in normal group, the expression of Cav3.2 mRNA in vectors group had no difference. The expression of Cav3.2 mRNA in pAd-shRNA-CaMKIIβ-DRG group decreased. After treatment with ropivacaine hydrochloride, the expression of Cav3.2 in normal + R group and vector + R group increased. Compared with the cells in pAd-shRNA-CaMKIIβ-DRG group, the expression of pAd-shRNA-CaMKIIβ-DRG + R group increased, as well as the expression of pAd-shRNA-CaMKIIβ-DRG + R group decreased compared with the cells in normal + R group, see .
Cav3.3 mRNA
Compared with the normal group, the expression of Cav3.3 mRNA of the cells in vector group was no different. The expression of Cav3.3 of the cells in pAd-shRNA-CaMKIIβ-DRG group decreased, while that in normal + R group and vector + R group increased. Compared with pAd-shRNA-CaMKIIβ-DRG group, the expression of Cav3.3 mRNA in pAd-shRNA-CaMKIIβ-DRG + R group increased. Compared with normal + R group, the expression of those in pAd-shRNA-CaMKIIβ-DRG + R group decreased, see .
CaMKIIβ, Cav3.2 and Cav3.3 protein expression
CaMKIIβ protein expression
Compared with normal group, the protein expression of CaMKIIβin pAd-shRNA-CaMKIIβ-DRG group was down-regulated. Compared with untreated with ropivacaine hydrochloride group, the protein expression of CaMKIIβ in every group treated with ropivacaine hydrochloride was up-regulated. Compared with normal + R group, the protein expression of CaMKIIβ in pAd-shRNA-CaMKIIβ-DRG + R group was down-regulated, see .
Figure 5. The protein expression of CaMKIIβ (A), Cav3.2 (B) and Cav3.3 (C) protein (± s, n = 3). D: The represented band of every group. Lane 1: normal group; Lane 2: vector group; Lane 3: pAd-shRNA-CaMKIIβ-DRG group; Lane 4: normal + R group; Lane 5: vector + R group; Lane 6: pAd-shRNA-CaMKIIβ-DRG + R group. Compared with normal group, ap < .05, compared with pAd-shRNA-CaMKIIβ-DRG, bp < .05, compared wth normal + R group, cp < .05.
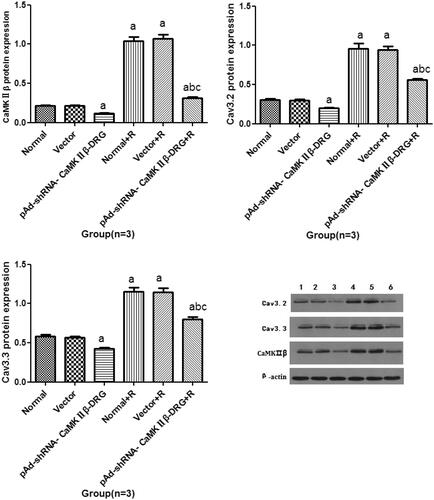
Cav3.2 protein expression
Compared with the normal group, the protein expression of Cav3.2 in the vector group showed no difference. The protein expression of Cav3.2 in the pAd-shRNA-CaMKIIβ-DRG group was down-regulated. Compared with the cell in untreated group, the protein expression of Cav3.2 in every group that was treated with ropivacaine hydrochloride was up-regulated. Compared with the pAd-shRNA-CaMKIIβ-DRG group, the protein expression of Cav3.2 in the pAd-shRNA-CaMKIIβ-DRG + R group was up-regulated. Compared with normal + R group, the protein expression of Cav3.2 in pAd-shRNA-CaMKIIβ-DRG + R group decreased, see .
Cav3.3 protein expression
There was no difference of the Cav3.3 protein expression between the cells in normal group and vector group. Compared with the cells in normal group, the protein expression of Cav3.3 of the cells in pAd-shRNA-CaMKIIβ-DRG down-regulated. After treated with ropivacaine hydrochloride, the Cav3.3 protein expression of the cells up-regulated. Compared with normal + R group, the expression of Cav3.3 in pAd-shRNA-CaMKIIβ-DRG + R group decreased, see .
Discussion
In this study, we adopted ropivaciane hydrochloride to induce the local anesthetics neurotoxicity. The pH value of ropivacaine hydrochloride was 4–6. In order to exclude the effect of adjuvant (hydrochloric acid) on this experiment, we titrated the pH value of the cell culture medium in every group as 4–6.
CaMKII is widely distributed in the nervous system and plays an important role in regulating the homeostasis of intracellular calcium ions [Citation17–19]. Calcium ion binds to calmodulin (CaM) to form Ca/CaM protein complex, which activates CaMK II. Activation of CaMKII can regulate intracellular calcium rebalancing [Citation20]. CaMKIIβ, a subtype of CaMK II, is widely distributed in the central nervous system. Studies have shown that CaMKIIβ is related to cerebral ischemia-reperfusion injury and the formation of learning and memory [Citation21,Citation22]. CaMKIIβ is one important factor for the neurons physiological activity. Dysfunction of CaMKIIβ may lead to neuronal dysfunction. In this study, we used the dorsal root ganglia neurons as the cell model of local anesthetics neurotoxicity to investigate the neurons injury induced by ropivacaine hydrochloride. According to our previous study, we determined the concentration of ropivacaine hydrochloride was 3 mM and the exposure time was 4 h. To observe the effects of CaMKIIβ on the neurotoxicity of ropivacaine hydrochloride, we down-regulated CaMKIIβ mRNA and protein expression by genetic engineering techniques.
In this study, we observed the neurotoxicity induced by ropivacaine hydrochloride, the cell viability of the DRG treated with ropivacaine hydrochloride decreased, cell apoptosis rate increased, as well as CaMKIIβ, Cav3.2 and Cav3.3 expression increased. Interestingly, CaMKIIβ knockdown can improve the DRG injury induced by the ropivacaine hydrochloride, cell viability increased and cell apoptosis rate decreased. Those data suggested that CaMKIIβ was closely related to the ropivacaine hydrochloride neurotoxicity.
T-type calcium channel is the low voltage dependent calcium channel. It is divided into Cav3.1, Cav3.2 and Cav3.3 [Citation23]. Cav3.2 and Cav3.3 are detected in DRG cells, they also are involved with the local anesthetics neurotoxicity [Citation8]. Inhibition expression of Cav3.2 and Cav3.3 can improve the local anesthetics neurotoxicity injury [Citation14]. It was interesting that down-regulation of CaMKIIβ can reduce Cav3.2 and Cav3.3 expression in DRG cells. Those data suggested that CaMKIIβ protective effect was related with Cav3.2 and Cav3.3.
In our previous study [Citation11], we found that local anesthetics increased the expression of caspase-3, and T-type calcium channel blockers could alleviate SH-SY5Y cell apoptosis induced by local anesthetics and reduce the expression of Caspase-3 protein. The results of this study showed that inhibiting the expression of CaMKIIβ to down-regulate the expression of T-type channel (Cav3.2 and Cav3.3) protein. Therefore, we speculated that CaMKIIβ regulates Caspase-3 expression through T-type calcium channels (Cav3.2 and Cav3.3) and induces apoptosis of DRG cells. At the same time, our previous study found that inhibiting T-type calcium channel in the neurotoxic injury model of local anesthetics resulted in the expression of P-38 MAPK decreased and apoptotic cells decreased, which suggested that T-type calcium channel participates in neurotoxic injury of local anesthetics through P-38 MAPK [Citation12]. From the results of this study, we speculated that CaMKIIβ may regulate the expression of Caspase-3 and p38MAPK through T-type calcium channel to participate in neurotoxic injury of local anesthetics.
There are some limitations in this study. We down-regulated the CaMKIIβ expression to investigate the ropivacaine hydrochloride neurotoxicity injury, however, we did not observe the effect of the DRG cells with CaMKIIβ up-regulation on the neurotoxicity induced by ropivacaine hydrochloride. We speculated that CaMKIIβ up-regulation may aggravate the DRG damage induced by local anesthetics. Although we proved that CaMKIIβ regulated Cav3.2 and Cav3.3 to participate in local anesthetics neurotoxicity, it remains unclear whether CaMKIIβ directly regulates Cav3.2 and Cav3.3 or not.
Conclusion
In other words, CaMKIIβ is involved with the DRG injury induced by ropivacaine hydrochloride. Inhibition CaMKIIβ expression improved DRG injury, increased the cell viability and decreased cell apoptosis rate.
Author contributions
Wen XJ designed and implemented the experiments and wrote the paper; Li YQ collected the data and performed the statistical analysis; Xingqing Liu, Can Sun, Jinbing Lin, Wenli Zhang, Yabin Wu implemented the experiments; Wang XP designed the experiment and performed the statistical analysis.
Disclosure statement
The authors declare no conflicts of interest.
Additional information
Funding
References
- Colbran RJ, Schworer CM, Hashimoto Y, et al. Calcium/calmodulin-dependent protein kinase II. Biochem J. 1989;258:313–325.
- Colbran RJ, Brown AM. Calcium/calmodulin-dependent protein kinase II and synaptic plasticity. Curr Opin Neurobiol. 2004;14:318–327.
- Colbran RJ. Targeting of calcium/calmodulin-dependent protein kinase II. Biochem J. 2004;378:1–16.
- O'Leary H. Regulation and functions of calcium/calmodulin dependent protein kinase II in excitatory neurons of the hippocampus [dissertations and Theses]. Gradworks; 2012.
- Liu Z, Huang Y, Liu L, et al. Inhibitions of PKC and CaMK-II synergistically rescue ischemia-induced astrocytic dysfunction. Neurosci Lett. 2017;657:199.
- Franklin RA, Rodriguez-Mora OG, Lahair MM, et al. Activation of the calcium/calmodulin-dependent protein kinases as a consequence of oxidative stress. Antioxid Redox Signal. 2006;8:1807–1817.
- Wen X, Lai X, Li X, et al. The effects of ropivacaine hydrochloride on the expression of CaMK II mRNA in the dorsal root ganglion neurons. Biomed Pharmacother. 2016;84:2014–2019.
- Wen XJ, Li XH, Li H, et al. CaMK II gamma down regulation protects dorsal root ganglion neurons from ropivacaine hydrochloride neurotoxicity. Sci Rep. 2017;7:5262.
- Lund LM, McQuarrie IG. Calcium/calmodulin-dependent protein kinase IIbeta isoform is expressed in motor neurons during axon outgrowth and is part of slow axonal transport. J Neurosci Res. 2002;67:720–728.
- Novak G, Seeman P, Tallerico T. Increased expression of calcium/calmodulin-dependent protein kinase IIbeta in frontal cortex in schizophrenia and depression. Synapse. 2006;59:61–68.
- Wen X, Xu S, Liu H, et al. Neurotoxicity induced by bupivacaine via T-type calcium channels in SH-SY5Y cells. PLOS One. 2013;8:e62942.
- Wen X, Xu S, Zhang Q, et al. Inhibitory gene expression of the Cav3.1 T-type calcium channel to improve neuronal injury induced by lidocaine hydrochloride. Europ J Pharmacol. 2016;775:43–49.
- Gong Q, Wen X, Li H, et al. Up-regulation of Cav3.1 expression in SH-SY5Y cells induced by lidocaine hydrochloride. Artif Cells Nanomed Biotechnol. 2018;46:1–8.
- Wen X, Liang H, Li H, et al. In vitro neurotoxicity by ropivacaine is reduced by silencing Cav3.3 T-type calcium subunits in neonatal rat sensory neurons. Artif Cells Nanomed Biotechnol. 2018;46:1617–1624.
- Wen XJ, Xu SY, Chen ZX, et al. The roles of T-type calcium channel in the development of neuropathic pain following chronic compression of rat dorsal root ganglia. Pharmacology. 2010;85:295–300.
- Wolfe JT, Wang H, Perez-Reyes E, et al. Stimulation of recombinant Ca(v)3.2, T-type, Ca(2+) channel currents by CaMKIIgamma(C). J Physiol (Lond). 2002;538:343–355.
- Byth LA. Ca(2+)- and CaMKII-mediated processes in early LTP. Ann Neurosci. 2014;21:151–153.
- Ghosh A, Giese KP. Calcium/calmodulin-dependent kinase II and Alzheimer's disease. Mol Brain. 2015;8:78.
- Erickson JR, Anderson ME. CaMKII and its role in cardiac arrhythmia. J Cardiovasc Electrophysiol. 2008;19:1332–1336.
- Hudmon A, Schulman H. Calcium/calmodulin-dependent protein kinase II. Encyclopedia Biol Chem. 2013;297–303.
- Sun B, Ou H, Ren F, et al. Propofol inhibited autophagy through Ca(2+)/CaMKKbeta/AMPK/mTOR pathway in OGD/R-induced neuron injury. Mol Med. 2018;24:58.
- Zalcman G, Federman N, Romano A. CaMKII isoforms in learning and memory: localization and function. Front Mol Neurosci. 2018;11:445.
- Cueni L, Canepari M, Adelman JP, et al. Ca(2+) signaling by T-type Ca(2+) channels in neurons. Pflugers Arch. 2009;457:1161–1172.