Abstract
Repairing the lost or damaged mandible is very difficult and time-consuming, so there is a great hope for tissue engineering to accelerate it. At the present study, electrospinning was applied to fabricate polyvinylidene fluoride (PVDF) and PVDF-polyaniline (PANI) composite scaffolds. In addition, extremely low frequency pulsed electromagnetic field (PEMF) was applied for treating the stem cells derived from dental pulp (DPSCs) when cultured on the nanofibrous scaffolds. Osteoinductive property of the fabricated PVDF, PVDF-PANI scaffold at the presence and absence of the PEMF was investigated by evaluating the common osteogenic differentiation markers in seeded-DPSCs on the scaffold. Results demonstrated that cell attachment, protein adsorption and cells viability were increased when PEMF was applied. In addition, ALP activity, calcium content, osteogenic genes and protein evaluations confirmed that PEMF could significantly increase osteoinductivity of the PVDF while composite with PANI. According to the results, the use of polymers with piezoelectricity and conductivity features plus PEMF exposure has a promising potential to improve the current treatment methods in bone and mandibular defects.
Introduction
As a multi-disciplinary field, tissue engineering (TE) has attempted to introduce numerous polymeric implants for the treatment of the mandibular lesions over the past two decades [Citation1]. This trend is still evolving to produce biocompatible implants and scaffolds that are more similar to the natural tissue of the bone to imitate their physical activities. There are two options for preparing three-dimensional (3D) scaffolds including the use of decellularized native extracellular matrix (ECM) derived scaffolds and synthesized polymeric scaffolds [Citation2]. Current gold standard treatment method in the patients faced by bone lesions is implanting of the autologous bone grafts, which can also be used after decellularization or seeding by autologous stem cells [Citation3]. Polymeric scaffolds can have a natural or synthetic source, and the most important determinants of their choice are biocompatibility, degradability and mechanical strength similar to the target tissue [Citation4]. Piezoelectricity is a unique property of the bone tissue [Citation5], which related to the mechanical energy converting to the electrical and also vice versa. So far, different polymers have been studied alone or in combination with each other in bone-TE (BTE). Various methods are also available for the construction of these scaffolds, which also produce biopolymeric products in various forms [Citation6,Citation7]. Given that the extracellular matrix (ECM) is often comprised of compressed nanoscale fibrous proteins, one of the most important, cheapest and easiest methods with high manipulation capability is electrospinning [Citation8].
Using this method, several polymers can be used to fabricate scaffold. At the present study, polyvinylidene fluoride (PVDF) has selected to make scaffold based on its prepared nanofibres biocompatibility and also its piezoelectricity that are useful for BTE according to the similarity to the bone tissue characteristics [Citation9,Citation10]. In addition, to increase the conductivity of the fabricated scaffold, polyaniline (PANI) as the most famous conductive polymer has also selected to composite with PVDF [Citation11].
Regarding to the designed scaffold (PVDF-PANI), which has piezoelectric properties and electrical conductivity, and, on the other hand, the application of mechanical pressure in laboratory conditions is very complicated for the testing functionality of the scaffold, the electromagnetic field (EMF) was applied to examine the functional issues of the fabricated piezoelectric scaffold. Although, undesirable issues of the EMF on health or lifestyle have been fully confirmed, various studies have shown that a part of the EMF, which has been introduced as an extremely low frequency (ELF, 0–300 Hz), has therapeutic potential for several diseases and now commonly used in the treatment of the osteoporosis and bone fractures [Citation12,Citation13].
Cells/stem cells are another sideways of the dental-BET and the most accessible and easiest cellular source to the dental bone is the tooth pulp, which dental-pulp-derived stem cells (DPSCs) can be extracted from them. These cells have a great proliferation and osteogenic differentiation potential which can be applied in dental-BET [Citation14].
This study was performed with the aim to investigate the osteoinductive property of a new designed cell-polymer implant based on its piezoelectric and conductive features at the presence and absence of the PEMF. Human DPSCs was applied to evaluating scaffold capability for the use in BTE applications.
Methods and materials
Composite electrospun nanofibres preparation
Electrospun composite nanofibrous scaffold was prepared based on the previously reported protocol [Citation15]. Briefly, PVDF with 275000 Mw (Sigma-Aldrich, St. Louis, MO, USA) was dissolved in the DMF/acetone solution (4/6 v/v) supplemented with tween-80 (2%, v/v) while placed on a stirrer for 3 h. Another solution was prepared with PANI and CPSA (both 100 mg) solving in DMSO (10 ml) with a final concentration of 3% (w/v) and PVDF concentration was also 18% (w/v) for PVDF-PANI nanofibres. Electrospinning (Nano-Model, Tehran, IRAN) was performed with 0.5 ml/h as flow rate and 22 kV as voltage and snozzle distance with collector was 24 cm.
Characterization of the prepared nanofibres
Morphological evaluation
Fabricated PVDF and PVDF-PANI nanofibres were evaluated morphologically via SEM before and after culturing of the DPSCs. DPSCs seeded-nanofibres were rinsed in glutaraldehyde for 2 h. After removing scaffold from fixator, it was rinsed in PBS and then dehydrated through rinsing in serial degraded alcohol from 50 to 100. Un-seeded and cell-seeded scaffolds were covered by platinum and examined by SEM (S-4500; Hitachi, Japan).
Mechanical properties
Tensile test was applied to mechanical features evaluation of the nanofibrous scaffold based on the protocol reported by Rezaie et al. [Citation16]. A load-cell (200 N) was applied at a speed of 1 mm/min for testing while scaffold was cut in 30 × 5×1 mm3 as a tensile strip.
Protein adsorption
The ability of PVDF and PVDF-PANI nanofibrous scaffold to absorb protein at the presence and absence of the PEMF was quantified based on the reported protocol by Saburi et al. [Citation17], briefly, scaffolds were placed in the PBS supplemented with FBS (l% v/v) at 37 °C for 1 h, and then, samples were transferred to the PBS containing sodium dodecylsulfate (2.0 wt.%, 20 h) to adsorbed proteins releasing from their surfaces. Both solutions protein content was quantified using a BCA kit (Pierce, Rockford, IL, USA) and reading at 530 nm (Bio-Tek Instruments, Inc., Winooski, VT, USA).
Dental pulp stem cell culture
Human DPSCs (IBRC C10266) was obtained from Royan Institute (Royan Institute, Tehran, Iran). Cells were cultured in the medium (alpha-MEM) containing FBS (20%) and 2 mM L-Glutamine and cells were detached and passaged after a week that cells received to the 80% confluency. DPSCs at passage three were used at this study for MTT assay and osteogenic differentiation evaluation.
MTT assay
MTT assay was performed to cell adhesion measurement and cytotoxicity examination of nanofibrous scaffold and also PEMF (1 mT, 50HZ) effects on the cell viability after DPSCs seeding on the PVDF and PVDF-PANI scaffolds at the presence of the basal medium with and without PEMF exposure during 10 days. DPSCs were seeded with a cell number of 1.5 × 104 cells per cm2 and to do experiment, medium was replaced by MTT solution (5 mg/ml) 5 h after cell seeding for cell adhesion assay and on days 1, 3, 5, 7 and 10 for biocompatibility assay and then stored at incubator for 4 h. Then, supernatants removed and DMSO were replaced for formazan crystals dissolving and finally, solutions were read at 570 nm.
Osteogenic differentiation
DPSCs were seeded on the sterilized PVDF and PVDF-PANI nanofibrous scaffolds under osteogenic medium (basal medium supplemented with Ascorbic Acid (50 µgr/ml), Dexamethasone (10 µM) and β-glycerophosphate (10 µM)) in the presence and absence the PEMF (1 mT, 50 HZ) during 6 h per day by a device designed by Ardeshirylajimi [Citation18].
ALP activity and calcium assay
To ALP activity measurement of the differentiated DPSCs, ALP kit (Pars-Azmoon, Tehran, Iran) was applied based on the recently reported protocol by our group at days 7 and 14 after differentiation induction [Citation19].
Total calcium content of the differentiated DPSCs was measured by using a calcium content kit reagent (Pars-Azmoon, Tehran, Iran) at days 7 and 14 after differentiation induction based on the recently reported protocol by our group [Citation20].
Real-time polymerase chain reaction
Real-time quantitative reverse transcription PCR was carried out to evaluation of five important osteogenic-related genes expression in the differentiated DPSCs cultured on the nanofibrous PVDF and PVDF-PANI scaffold at the presence and absence of the PEMF 7 and 14 days after differentiation induction. Genes were included Runx2z, Collagen type-I (Col-I), Osteocalcin (OSC), Osteonectin (OSN) and Osteopontin (SSP1). The RNA content of the differentiated cells was obtained using Qiazol (Qiagen, Valencia, CA, USA) and reverse transcription was also performed for the cDNA synthesis by random hexamer and M-MLV Reverse Transcriptase (Vivantis, Kuala Lumpur, Malaysia) based on the kit’s protocols. is shown primers sequences, which used in this study. SYBR Premix Ex Taq Master mix (Takara, Tokyo, Japan) and Rotor Gene 6000 (Corbett, Life Science, Concorde, NSW, Australia) were applied to do a real-time experiment.
Table 1. Sequence of the primers used in the real-time RT-PCR analysis at the present study.
Immunocytochemistry
Immunocytochemistry (ICC) was applied to the post-translational investigation of the osteocalcin expression level in differentiated DPSCs after 21 days grown on PVDF and PVDF-PANI under ELF exposure. Briefly, cell-cultured scaffolds were fixed using 4% paraformaldehyde for 20 min. After that, scaffolds were rinsed in BSA for blocking and then incubated at 4 °C for 1 hour. After that, anti-osteocalcin (1:50 OPN, Proteintech Germany) was used as a primary antibody. Finally, PE-conjugated goat-anti-mouse IgG (1:200, Sigma Aldrich, St. Louis, MO. USA) was used as a secondary antibody. Before imaging, supernatant was removed, and then, DAPI was used to the cell nucleus staining.
Statistics
All Experiments were performed 3-times; obtained results were mentioned as means ± standard deviation. Obtained data from real-time RT-PCR were analyzed using REST 2009 software (Germany). Comparison of data between different groups was performed via one-way analysis of variance (ANOVA) method using SPSS. Probability values ≤.05 were mentioned as significant.
Results
Scaffold characterization
Fabricated PVDF and PVDF-PANI scaffolds were morphologically evaluated via SEM and images demonstrated that fibres were bead free, flat, nanometer-sized and with random orientation (, respectively). Mechanical properties of the prepared nanofibres were quantified by tensile-instrument and results were showed that peak stress of the PVDF nanofibres decreased while composite with PANI from 1.93 MPa to 1.29 MPa, but peak strain was increased from 15.23% to 29.56% in PVDF after blending with PANI (). Tensile strength was reduced in PVDF after composite with PANI _ 1.57 MPa to 0.9 MPa, which showed positive effects of the PANI on the mechanical features of the PVDF nanofibrous scaffold.
Figure 1. SEM was applied to morphological study of the fabricated PVDF (A) and PVDF-PANI (B) nanofibrous scaffold.
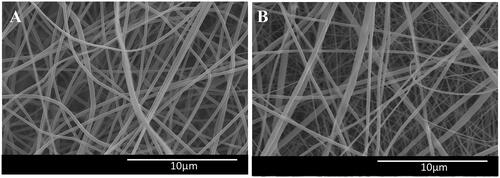
TABLE 2. Tensile results: mechanical properties of the PVDF nanofibrous scaffolds before and after composite with PANI.
After that, the biocompatibility of the nanofibrous scaffold was evaluated qualitatively using SEM and quantitatively using MTT by culturing of the human DPSCs () on nanofibres at the presence and absence of the PEMF. SEM photographs were demonstrated that DPSCs continuously grew on the surface of PDVF () and PVDF-PANI () nanofibres during two weeks after cell seeding, whereas DPSCs on PVDF-PANI was more confluent than PVDF. PEMF exposure showed an affirmative effect on the infiltration and proliferation of the cells into the scaffold whereas this effect was observed in PVDF-PANI group () higher than PVDF group (). To more characterization of the fabricated scaffold, cell adhesion () and protein adsorption () were also investigated and results were showed that the amount of the protein adsorbed by PVDF nanofibres was not changed significantly by treating with PEMF, but when PVDF combined with PANI (PVDF-PANI), its protein adsorption was increased significantly. It should be noted that protein adsorption in PVDF-PANI under PEMF treatment was significantly higher than when non-treated. In addition, the rate of the adhered cells 5 h after seeding on the surface of the PVDF nanofibres was increased significantly by combining with PANI and treating with PEMF synergistically, whereas highest cell adhesion was detected in ELF+(PVDF-PANI) group. Following scaffold characterization, MTT assay was done to quantify survival and proliferation rate of the DPSCs when grown on the PVDF and PVDF-PANI nanofibres with and without PEMF exposure (). Proliferation rate differences of the DPSCs at days 1, 3 and 5 were not noticeable when grown in different situations. But at days 7, the most proliferation rate of the DPSCs was found when grown on ELF+(PVDF-PANI) group that this difference was also notable compared with the others. Proliferation rate difference between DPSCs cultured on ELF + PVDF and PVDF-PANI was not significant, but DPSCs proliferation rate in both of them was significantly greater than those seeded on PVDF group. On days 10, DPSCs proliferation rate in ELF treated groups were significantly higher than those non-treated and highest DPSCs proliferation rate was also observed in those cells cultured on ELF+(PVDF-PANI) group.
Figure 2. Optical micrographs of the dental pulp stem cells (DPSCs) (A), SEM images of the DPSCs-seeded electrospun PVDF scaffold in the absence (B) and presence of the PEMF exposure (ELF + PVDF) (C), DPSCs-seeded electrospun PVDF-PANI scaffold in the absence (D) and presence of the PEMF exposure (ELF + PVDF) (E) at day 14 while cultured under osteogenic medium.
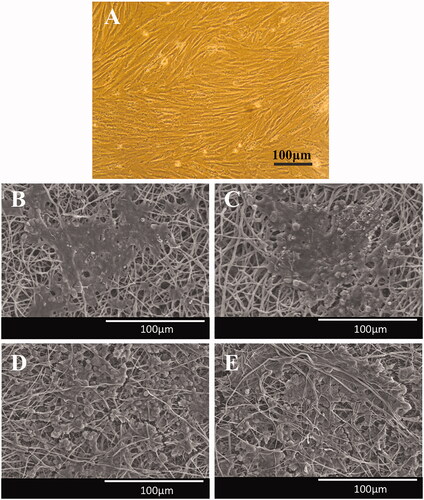
Figure 3. Cell attachment (A) and protein adsorption (B) assays of the fabricated PVDF and PVDF-PANI nanofibrous scaffold at the presence and absence of the PEMF exposure. The viability and proliferation rate of the DPSCs during 10 days to display non-toxicity and biocompatibility of the PVDF and PVDF-PANI scaffolds and PEMF exposure under basal medium. The significant differences (p<.05) between groups are indicated with star sign.
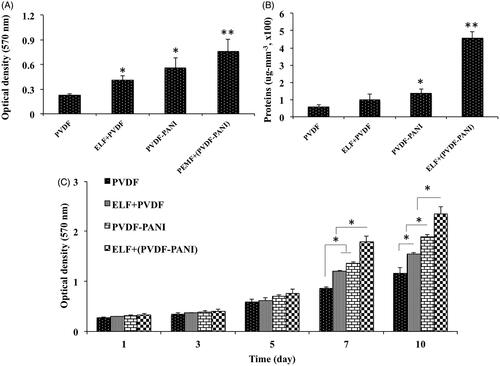
Osteogenic differentiation evaluation
ALP and calcium content
When we understand that proliferation rate of the DPSCs significantly affected by ELF exposure after a week, we continued osteogenic differentiation evaluation of the DPSCs when grown on four groups: DPSCs cultured on PVDF, ELF + PVDF, PVDF-PANI and ELF+(PVDF-PANI). ALP activity () and calcium content () as two critical osteogenic indicators were examined in DPSCs when grown on different groups under osteogenic medium 7 and 14 days after differentiation induction. Results were showed that PANI and ELF has a great impact on ALP activity and calcium content of the DPSCs during the period of study when grown on PVDF and this effect was synergistically increased while PVDF combined with both PANI and ELF exposure in ELF+(PVDF-PANI) group compared to the other groups such as PVDF, ELF + PVDF and PVDF-PANI.
Figure 4. The ALP activity (A) and calcium content (B) assays of the differentiated DPSCs while cultured on the PVDF and PVDF-PANI under osteogenic medium at the absence and presence of the PEMF at days 7 and 14 after cell seeding. The significant differences (p<.05) between groups are indicated with star sign.
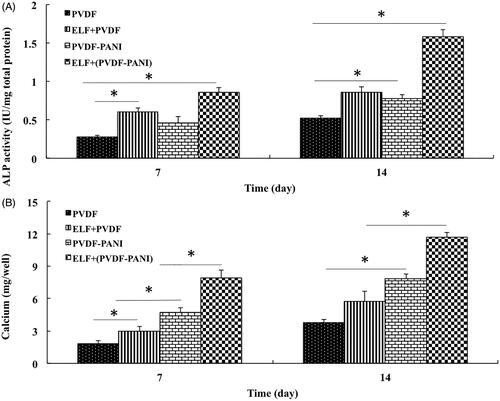
Gene expression
Osteogenic genes evaluation was carried out to more investigation of the synergism effect of the ELF and PVDF-PANI on the osteogenic differentiation potential of the DPSCs (. Results were showed that Runx2 gene expression as a critical primary osteogenic factor was up-regulated significantly in DPSCs when grown under PEMF exposure in PVDF or PVDF-PANI groups whereas highest Runx2 expression was detected in ELF+(PVDF-PANI) group at both days (7 and 14). Expression level of the Col-I in DPSCs seeded on ELF+(PVDF-PANI) was greater than PVDF, ELF + PVDF and PVDF-PANI but it was not significant on day 7, but on day 14 this difference was significant. Osteonectin and osteocalcin as another two critical osteogenic gene markers were expressed in DPSCs seeded on ELF + PVDF and ELF+(PVDF-PANI) significantly greater than those cells seeded on PVDF and PVDF-PANI nanofibrous scaffold at both 7 and 14 days after cells seeding. Although expression of these genes in DPSCs cultured on ELF + PVDF and PVDF-PANI on days 7 was not significantly different. Osteopontin (SSP1) gene expression was not up-regulated a lot on day 7, because this is a lately expressed osteogenic gene, but on day 14, the expression level of this gene in the DPSCs grown on the PVDF-PANI and ELF+(PVDF-PANI) was increased compared to the other groups, but this increase in ELF+(PVDF-PANI) was significant compared to the others.
Figure 5. Relative expression of Runt-related transcription factor 2 (Runx2), Collagen type-1 (Col-I), Osteonectin (OSN), Osteocalcin (OSC) and Osteopontin (SPP1) at days 7 and 14 for DPSCs cultured on the PVDF and PVDF-PANI under osteogenic medium at the absence and presence of the PEMF. The significant differences (p<.05) between groups are indicated with star sign.
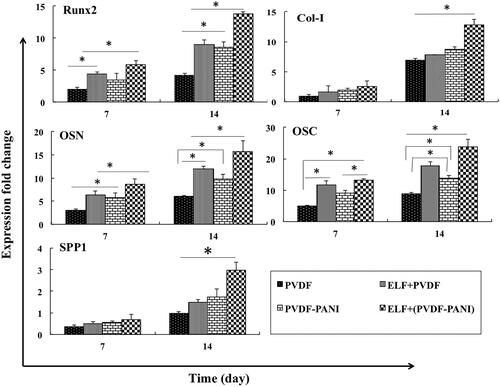
Protein expression
Two ELF + PVDF and ELF+(PVDF-PANI) groups were selected to more investigation by ICC according to the better results acquired using ALP activity, calcium content and gene expression assays. Osteocalcin protein expression as a lately expressed osteogenic marker was investigated using ICC at the end of study, and results were showed that this protein was expressed in cultured DPSCs in ELF+(PVDF-PANI) group () significantly higher than those DPSCs cultured in ELF + PVDF group (). DAPI staining was also carried out for nuclear detection () for samples.
Discussion
Bone lesions can be created at various parts of the body; one of the most important bone defects could be created in the mandible area, which can be caused by various reasons, including oral and dental diseases or tumours [Citation21]. When needed to remove a large portion of the mandibular bone, the most common method for replacing it is autologous bone grafting from the patient pelvic bone, which is very painful and causes a lot of restrictions for the patient lifespan [Citation22,Citation23]. Tissue engineering has been a major effort to introduce numerous implants to treat and reconstruct of the mandibular defects in these types of patients [Citation24,Citation25]. Several polymeric scaffolds have already been introduced as bio-implants for use in mandibular surgery, in order to fill the empty space created by the removal of the tumorous bone, or the bone fragmentation portion that each of them has advantages and disadvantages. To accelerate bone reconstruction process, stem cells could also be used as an important part of the mandibular TE [Citation26]. Polymers such as polycaprolactone, polyvinyl alcohol, poly (L-glycolic acid) and poly (L-lactic acid) are among the most important polymers that used in various forms, including nanofibres, spongy and 3 D printed alone or in combination with bioceramics such as beta-tricalcium phosphate and hydroxyapatite [Citation27]. Various stem cells were also studied in combination with these scaffolds at either the in vitro, in vivo or clinical levels, which most of them was mesenchymal stem cells (MSCs) derived from different tissues such as bone marrow and adipose tissues [Citation28,Citation29]. Although the use of adipose-derived MSCs has been more interested by researchers due to the its several unique characteristics such as the availability of its source, its non-invasive preparation method, its high proliferation and survival capacity, its direct differentiation potential to mature osteoblast, the paracrine effect on neighbour cells, and the secretion of microvesicles that can contain many beneficial factors [Citation30]. In this study, in addition to fabricating a composite polymeric scaffold with unique properties and use of the more available stem cells at the oral cavity, PEMF exposure were also used for introduce a multifactorial construct, which can have many benefit for use in mandibular tissue engineering. To achieve this aim, PVDF as a polymers with piezoelectric feature [Citation31] was selected as a main component of the nanofibrous scaffold and then PANI as a famous conductive polymer [Citation32] was selected to blending with PVDF to improve its biological and mechanical characteristics. Our results obtained from cell adhesion, protein adsorption and MTT assays demonstrated that PVDF biocompatibility was improved significantly while combined with PANI. After that PEMF treatment was also applied in addition to the PVDF combining with PANI and results were showed that this improvement was synergistically increased when both of them were applied. After characterization of the PVDF and PVDF-PANI scaffolds, ELF range of the EMF was also selected to irradiation as another part, for osteogenic induction. Since, dental pulp tissue is more available stem cells source in patients with mandibular defect [Citation33], DPSCs was also selected as stem cells in the current study to examine its osteogenic differentiation potential while cultured on the fabricated PVDF and PVDF-PANI nanofibrous scaffolds at the presence and absence of the PEMF exposure. ALP is a critical enzyme during the osteogenesis, which its function is cutting the phosphates groups of macromolecules and increased extracellular mineralization that is essential for bone formation [Citation34]. For this reason, ALP activity was evaluated at this study and results were displayed that this activity significantly enhanced in DPSCs while cultured on PVDF-PANI compared to the PVDF. In addition, this increase was also more significantly enhanced in DPSCs while both PANI and ELF treatment was applied at this study in ELF+(PVDF-PANI) group. It is concluded that PEMF has an acceleratory effect in ALP protein expression that can modulate osteogenesis, which can improve by the presence of a conductive polymer (PANI) at the scaffold. Tsai and et al. showed also positive effects of the PEMF treatment on ALP activity of the human MSCs while cultured under osteogenic medium [Citation35]. Increase in ALP activity was also reported by others followed by ELF irradiation to adipose-derived MSCs and human induced pluripotent stem cells during 2 D and 3 D culture [Citation36,Citation37]. As was predictable based on ALP activity increase, calcium content was also significantly enhanced in DPSCs seeded on ELF+(PVDF-PANI) group compared to the those DPSCs cultured on PVDF, ELF + PVDF and PVDF-PANI groups. It is well known that cell membrane channels and ion charges will affect by PEMF and it has also a great effect on the motion of the ions across the membrane [Citation38,Citation39], which can have a positive effect on ion outflow and increased mineralization. Our results were showed that this ability could also improve synergistically by the presence of the conductive polymer such as PANI in the nanofibrous scaffold. Gene expression evaluation was performed to more investigation of the differentiation level of the DPSCs on different groups. Runx2, osteonectin, osteocalcin and osteopontin genes expression was affected significantly by combining of the PVDF with PANI, although expression level of the Col-I and SPP1 was not significantly changed. But expression level of all genes was significantly upregulated, while both PANI and PEMF were applied at the experiment. However, changes in Col-I expression was not significant, while PEMF was applied that is reported by others also [Citation35]. To more investigation of the combination effects of PANI and PEMF on osteogenesis, osteocalcin protein expression level was examined using ICC and result showed that presence of the conductive polymer (PANI) could significantly increase PEMF positive effects on the osteocalcin expression in the stem cells during osteogenic differentiation.
Conclusion
Obtained results revealed that PVDF biocompatibility and osteoinductivity were increased while combined with PANI, and it can be considered as a suitable composite scaffold for osteogenic induction since it has two great features as piezoelectricity and conductivity that potentially could be very useful for protein adsorption and cell attachment. In addition, the presence of the conductive polymer could increase PEMF positive impact on osteogenic differentiation of the DPSCs. Based on the results, it was confirmed that the osteogenic differentiation potential of the human DPSCs was synergistically enhanced by PANI and PEMF. Regards to the mandibular defects and based on the results obtained from the present study, several clinical treatment options can be concluded including the use of DPSCs transplantation alone at the defect site while treating with PEMF from outside of the body. Implanting of the PVDF-PANI nanofibrous scaffold alone and exposing patients with PEMF irradiation, and/or the use of a combination strategy with both DPSCs and PVDF-PANI implanting that could be leading to a synergistic increase in the regeneration process.
Acknowledgement
The authors thank Alborz University of Medical Sciences and Shahid Beheshti University of Medical Sciences for their financial support to this project.
Disclosure statement
The authors declare that they have no conflict of interest.
References
- Neel EAA, Chrzanowski W, Salih VM, et al. Tissue engineering in dentistry. J Dent. 2014;42:915–928.
- Aulino P, Costa A, Chiaravalloti E, et al. Muscle extracellular matrix scaffold is a multipotent environment. Int J Med Sci. 2015;12:336.
- Paduano F, Marrelli M, Alom N, et al. Decellularized bone extracellular matrix and human dental pulp stem cells as a construct for bone regeneration. J Biomater Sci Polym. 2017;28:730–748.
- Sharma S, Srivastava D, Grover S, et al. Biomaterials in tooth tissue engineering: a review. JCDR. 2014;8:309.
- Jacob J, More N, Kalia K, et al. Piezoelectric smart biomaterials for bone and cartilage tissue engineering. Inflamm Regener. 2018;38:2.
- Hutmacher DW. Scaffold design and fabrication technologies for engineering tissues—state of the art and future perspectives. J Biomater Sci Polym. 2001;12:107–124.
- Ardeshirylajimi A. Applied induced pluripotent stem cells in combination with biomaterials in bone tissue engineering. J Cell Biochem. 2017;118:3034–3042.
- Pham QP, Sharma U, Mikos AG. Electrospinning of polymeric nanofibers for tissue engineering applications: a review. Tissue Eng. 2006;12:1197–1211.
- Ribeiro C, Correia DM, Rodrigues I, et al. In vivo demonstration of the suitability of piezoelectric stimuli for bone reparation. Mater Lett. 2017;209:118–121.
- Harrison JS, Ounaies Z, Bushnell DM. Piezoelectric polymers. Ueberschlag P. PVDF piezoelectric polymer. Sensor Review. 2001;21:118–126.
- Huang W-S, Humphrey BD, MacDiarmid AG. Polyaniline, a novel conducting polymer. Morphology and chemistry of its oxidation and reduction in aqueous electrolytes. J Chem Soc, Faraday Trans 1. 1986;82:2385–2400.
- Saliev T, Mustapova Z, Kulsharova G, et al. Therapeutic potential of electromagnetic fields for tissue engineering and wound healing. Cell Prolif. 2014;47:485–493.
- Haddad JB, Obolensky AG, Shinnick P. The biologic effects and the therapeutic mechanism of action of electric and electromagnetic field stimulation on bone and cartilage: new findings and a review of earlier work. J Altern Complement Med. 2007;13:485–490.
- Tatullo M, Marrelli M, Shakesheff KM, et al. Dental pulp stem cells: function, isolation and applications in regenerative medicine. J Tissue Eng Regen Med. 2015;9:1205–1216.
- Ardeshirylajimi A, Ghaderian S-H, Omrani MD, et al. Biomimetic scaffold containing PVDF nanofibers with sustained TGF-β release in combination with AT-MSCs for bladder tissue engineering. Gene. 2018;676:195–201.
- Rezaie Z, Ardeshirylajimi A, Ashkezari MD. Improved anticancer properties of stem cells derived exosomes by prolonged release from PCL nanofibrous structure. Gene. 2018;665:105–110.
- Saburi E, Atabati H, Kabiri L, et al. Bone morphogenetic protein‐7 incorporated polycaprolactone scaffold has a great potential to improve survival and proliferation rate of the human embryonic kidney cells. J Cell Biochem. 2019;120:9859–9868.
- Ardeshirylajimi A, Soleimani M. Enhanced growth and osteogenic differentiation of induced pluripotent stem cells by extremely low-frequency electromagnetic field. Cell Mol Biol. 2015;61:36–41.
- Hosseini FS, Soleimanifar F, Aidun A, et al. Poly (3‐hydroxybutyrate‐co‐3‐hydroxyvalerate) improved osteogenic differentiation of the human induced pluripotent stem cells while considered as an artificial extracellular matrix. J Cell Physiol. 2019;234:11537–11544.
- Hosseini FS, Soleimanifar F, Khojasteh A, et al. Promoting osteogenic differentiation of human‐induced pluripotent stem cells by releasing Wnt/β‐catenin signaling activator from the nanofibers. J Cell Biochem. 2019;120:6339–6346.
- Boyd JB, Gullane PJ, Rotstein LE, et al. Classification of mandibular defects. Plast Reconstr Surg. 1993;92:1266–1275.
- Abukawa H, Shin M, Williams WB, et al. Reconstruction of mandibular defects with autologous tissue-engineered bone. J Oral Maxillofac Surg. 2004;62:601–606.
- Lindström J, Brånemark P-I, Albrektsson T. Mandibular reconstruction using the preformed autologous bone graft. Scand J Plast Reconstr Surg. 1981;15:29–38.
- Meijer GJ, de Bruijn JD, Koole R, et al. Cell based bone tissue engineering in jaw defects. Biomaterials. 2008;29:3053–3061.
- Zhang W, Abukawa H, Troulis MJ, et al. Tissue engineered hybrid tooth–bone constructs. Methods. 2009;47:122–128.
- Mao JJ, Giannobile WV, Helms JA, et al. Craniofacial tissue engineering by stem cells. J Dent Res. 2006;85:966–979.
- Jagur‐Grodzinski J. Polymers for tissue engineering, medical devices, and regenerative medicine. Concise general review of recent studies. Polym Advan Technol. 2006;17:395–418.
- Polak JM, Bishop AE. Stem cells and tissue engineering: past, present, and future. Ann N Y Acad Sci. 2006;1068:352–366.
- Marion NW, Mao JJ. Mesenchymal stem cells and tissue engineering. Meth Enzymol. 2006;420:339–361.
- Paduano F, Marrelli M, Amantea M, et al. Adipose tissue as a strategic source of mesenchymal stem cells in bone regeneration: a topical review on the most promising craniomaxillofacial applications. IJMS. 2017;18:2140.
- Ueberschlag P. PVDF piezoelectric polymer. Sensor Rev. 2001;21:118–126.
- Roth S. Conducting polymers-present state of physical understanding. MSF. 1989;42:1–16.
- Egusa H, Sonoyama W, Nishimura M, et al. Stem cells in dentistry-part I: stem cell sources. J Prosthodont Res. 2012;56:151–165.
- Orimo H. The mechanism of mineralization and the role of alkaline phosphatase in health and disease. J Nippon Med Sch. 2010;77:4–12.
- Tsai MT, Li WJ, Tuan RS, et al. Modulation of osteogenesis in human mesenchymal stem cells by specific pulsed electromagnetic field stimulation. J Orthop Res. 2009;27:1169–1174.
- Arjmand M, Ardeshirylajimi A, Maghsoudi H, et al. Osteogenic differentiation potential of mesenchymal stem cells cultured on nanofibrous scaffold improved in the presence of pulsed electromagnetic field. J Cell Physiol. 2018;233:1061–1070.
- Ardeshirylajimi A, Khojasteh A. Synergism of electrospun nanofibers and pulsed electromagnetic field on osteogenic differentiation of induced pluripotent stem cells. Asaio J. 2018;64:253–260.
- Tenforde TS. Cellular and molecular pathways of extremely-low-frequency electromagnetic field interactions with living systems. Richland, WA (United States): Pacific Northwest Lab; 1992.
- Funk RHW, Monsees T, Özkucur N. Electromagnetic effects – from cell biology to medicine. Prog Histochem Cytochem. 2009;43:177–264.