Abstract
The engineering of multifunctional therapeutics in an integrated single platform is demonstrated using three-dimensional SBA-16 (S-16). 10 wt% iron oxide nanoparticles (Fe) were loaded into the cage type of cubic pores through enforced adsorption technique. Fe/S-16 is then functionalized with amine-based silane (A), polyacrylic acid (P) and cisplatin (Cp). The physicochemical textural analysis showed the formation of nano metal oxide distributions at pore walls of S-16 with magnetization of 2.39 emu/g. S-16 based nanoformulations showed high percentage of Cp adsorption (90%) and percentage cumulative release (60%). in vitro study of Fe/S-16-A-Cp showed high toxicity against breast cancer cell line MCF-7 and normal cell line Human foreskin fibroblast (HFF-1) compared to Fe/S-16 indicating cisplatin profusion inside the cells than free cisplatin. While skin fibroblast seems to be resistant to Fe/S-16-AP-Cp with very high LC50 in compare to MCF-7. This indicates the unrelease of cisplatin in skin fibroblast after Fe/S-16-AP-Cp treatment due to effective encapsulation inside the cubic pores and core blockage due to pH-sensitive polyacrylic acid. Also, these treatments resulted in morphological changes in the cells such as DNA condensation and nuclear fragmentation.
Introduction
Cancer in various forms remains a deadly disease for centuries. The cancer burden is expected to increase to about 24 million by 2035 [Citation1]. Structured mesoporous silica nanoplatforms are most widely researched support in biomedical application such as diagnostic purpose, targeted oriented drug therapy, bioengineering and stem cell [Citation2]. The nanoparticle utilization has evolved from the monotonous role of drug delivery system to multifunctional role of labelling, drug and gene transportations, detection of pathogens and proteins, as RNA and DNA probe, optical imaging enhancer, tissue designing, biomolecules and cell isolation and as tumour destructor [Citation3]. Nanotechnology driven therapeutic chances have been broadened on chronic cancer, diabetic, autoimmunity, neurological disorders and other metabolic disorders [Citation4]. Particularly, magnetic-based nanocomposites were interesting in precision oncology therapy. The most striking features of magnetic/silica-based nanotherapeutics is the ability of nanoparticle to accommodate several components into a nano structure to generate multifunctional modality. In particular, superparamagnetic iron oxide nanoparticles (SPIONs) uses in biomedical applications are advantageous due to human tolerance level and are approved by the Food and Drug Administration (FDA) [Citation5]. Nonetheless, designing such multifunctional drug carriers are still marred by certain critical issues like passivation due to multiple inorganics, low pH-sensitive and toxicity at high dose level. Designing of diagnostic and drug-loading protocol for drug delivery in a single entity is cumbersome and challenging. Even though such modification is effective, the targeted diffusion of such nanoformulations and bioavailability are rather lower (5–10%).
The release rate of biomolecules was found to depend on the type and pore architectures of silica. Two dimensional straight channels of MCM-41 and SBA-15 has been shown to release the drug at a faster rate, while three-dimensional shaped structure (MCM-48) shown to slow down the drug release due to restricted cubic-shaped pores. Several magnetically active structured mesoporous molecular silicas were reported to exhibit dual-modal theranostic applications. Aval et al. [Citation6] have described about the preparation of pH responsive (pH change from 7.4 to 5.5) release of DOX (93%) using Fe3O4 SPIONs coated hydroxyapatite drug delivery system. The nanoformulation reduced the cell viability of breast cancer cell lines SKBR3 (54.7%) and T47D (57.3%) that is almost similar to conventional free DOX. Egodawatte et al. [Citation7] have reported the Fe3O4 impregnated over amine functionalized MCM-41 for 5-Fluorouracil drug release. Highest loading was found over DMSO than other solvents and showed the vital role of solvents polarity for the interaction between the amino group of silane and drug molecules. Zelenak et al. [Citation8] have reported the indomethacin delivery effect over unmodified Fe/3D SBA-16 and silane (aminopropyltriethoxysilane) modified SPIONs/SBA-16. The presence of drug shown to un effect the super paramagnetic property of Fe/SBA-16. However, indomethacin release ability has been found to be strongly influenced by the silane functionalization. The presence of hydrogen bonding between the amine group of silane and COOH group of drug shown to decrease the release in a sustainable manner (63%) compared to Fe/SBA-16 (68%) and unmodified SBA-16 (91%).
In our present study, three-dimensional SBA-16 was chosen as nanocarrier. The presence of 3 D cubic pore architecture with magnetic behaviour is proposed to reduce the aggregation and respective toxicity. Large surface area of SBA-16 is proposed to accommodate magnetically active species and exhibit high cisplatin loading-release capabilities for targeted anticancer efficiency in in vitro cancer cell line MCF-7. Cisplatin (cis-diamminedichloroplatinum (II) is a therapeutic drug used for cancer therapy (testicular, cervical, lung, ovarian and malignant mesothelioma). The drug excellent cytotoxic action is let down by adverse side effects such as developing resistance, nephrotoxicity (kidney failure), neuropathy, dose toxicity and hearing loss. Currently, several nanocarriers such as polymers, structured silicas, liposomes are reported to facilitate controlled and sustained drug release of cisplatin for a longer time period. However, studies related to synthesis, characterization of SPIONs/3D SiSBA-16 and silane functionalized SPIONs/SiSBA-16 for cisplatin (platinum-based drug) release effect, toxicity study using MTT on the cell line MCF-7 has not been presented in open literature. The present nanoformulation shows that very less dose of cisplatin loaded over multifunctional nanocarrier is sufficient to kill the cancer cells. By this way, the dose of cisplatin reduced, while the therapeutic effect is maintained.
Methods
SBA-16 was synthesized based on our earlier reported method [Citation9].
Preparation of 10 wt%SPIONs/S-16-AP-Cp (Fe/S-16-AP-Cp)
The support SBA-16 was predried at 120 °C for 24 h. 10 wt% of iron oxide was loaded over each support by enforced adsorption technique. In brief, 0.72 g of iron (III) nitrate nonahydrate was dissolved in 80 ml water for 10 min. Then, S-16 (1 g) was added and stirred overnight. Then, the aqueous mixture was dried at 120 °C for 12 h and then calcined at 550 °C for 2 h. Depending on the support, the loaded formulations were labelled as Fe/S-16, Fe/HYPS and Fe/MSU-F (). The aqueous mixture was stirred for 10 min, then dried at 120 °C for 12 h and calcined at 550 °C for 2 h. Fe/S-16 was then functionalized with 1.5 ml of aminopropyltriethoxysilane silane (A) using anhydrous toluene (100 ml) solution mixture under the refluxing condition in an argon atmosphere. The solution mixture was then filtered using what paper in glass funnel and then vacum dried for 3 h to produce Fe/S-16-A. Cisplatin loading was carried out by dissolving 30 mg of Cp with 600 mg of Fe/S-16-AP in normal saline solution (NSS) following our previously published literature to produce Fe/S-16-A-Cp [Citation10]. The cisplatin content determined to be 0.12 mmol/g of the nanocarrier.
Table 1. LC50 values for Fe/S-16, cisplatin, Fe/S-16-AP-Cp and Fe/S-16-A-Cp on cancer (MCF-7) and normal (HFF-1) cell lines.
Nanomaterial characterization
The phase analysis of SPIONs was measured using Rigaku Multiplex system (Rigaku, Japan). Textural characteristics involving surface area and pore size distributions of parent and formulations was analyzed by ASAP-2020 plus (Micromeritics, Norcross, GA, USA). Cisplatin functional groups over silica was identified using FT-IR spectroscopy (Perkin Elmer, Waltham, Massachusetts, USA).
Cell culture
MCF-7 (Breast cancer cell line) and on non-cancerous cell Human foreskin fibroblast (HFF-1) (ATCC® SCRC-1041™) were used. The MCF-7 cell line was gifted from (Dr Khaldoon M. Alsamman) Clinical Laboratory Science, Imam Abdulrahman Bin Faisal University. A 75-cm2 culture flasks were used to grow the cells in RPMI medium supplemented with penicillin/streptomycin (1%) (Thermo Fisher, Waltham, USA) and fetal calf serum (10%) (HyClone, GE Healthcare, Chicago, IL, USA) at 37 °C in a humidified incubator (Heracell 150i, Thermo Scientific, Waltham, MA, USA) with 5% CO2. The cells were cultured in a 96-well plate (Thermo Fisher, Waltham, MA, USA) (1 × 104 cells per well). At 80% confluent, the cells were treated with varying concentrations of nanoformulated drugs, along with the control. After 24 h, the cells were tested for cell survival using MTT assay, a dye reduction test.
MTT assay
MTT ((3–(4, 5-Dimethylthiazol-2-yl)-2,5-Diphenyltetrazolium Bromide), 20 µl (10 mg/ml)) was added over cultured cells in each well of 96-well plate. The cells were incubated for 4 h at 37 °C, then washed to remove the dye using 1x PBS. MTT solvent (150 µl) was added in each well to solubilize the formed formazan dye, the plate was then agitated for 15 min on a plate shaker. At 590 nm, the color intensity of solubilized dye was measured using a multiplate reader (Tecan Infinite® 200 PRO, Männedorf, Switzerland). The intensity of color was calculated and compared with control, the results were presented as the percentage of cell survival.
Hoechst staining
Cells viability of treated and control cells were tested by Hoechst staining (Sigma Aldrich). Pretreated MCF-7 and HFF-1 cells with cisplatin (Cp), Fe/S-16-A-Cp, Fe/S-16-AP-Cp or vehicle control were washed with 1x PBS, fixed with ice-cold methanol for 5 min and stained with Hoechst (Sigma Aldrich, St. Louis, MO, USA) for 10 min in the dark. Cells were washed again with 1× PBS, dried up and mounted with ProLong ® Gold Antifade reagent (Thermo Fisher, Waltham, MA, USA). Images were developed by ZEN software using confocal microscopy (Zeiss, Oberkochen, Germany).
Statistical analysis
The results of MTT cell cytotoxicity assay were subjected to one-way ANOVA followed by Dunnett’s post hoc test of GraphPad Prism software (GraphPad, La Jolla, CA, USA) on three independent sets of experiments conducted in triplicates. p values < .05 were considered significant
Preparation of Fe/S-16-A-Cp (G-IV)-treated cells for TEM
MCF-7 cells were cultured in six-well culture plates with a media containing: RPMI, 10% FBS, L-glutamine, 120 μg/mL streptomycin, and 120 μg/mL penicillin at 37 °C in an incubator with 5% CO2 environment (Heracell 150i, Thermo Scientific, Waltham, MA, USA). The MCF-7 cells were then treated with 32 µg/mL of Fe/S-16-A-Cp (G-IV) nanoparticles for 60 min. the control and treated cells were processed with chemicals prior to transfer into the TEM. A well-established preparation method was adopted [Citation11,Citation12]. Briefly, the pellets of the cells were made by centrifugation. The pellets were then fixed and rinsed 2–3 times in a first fixative: a mixture of glutaraldehyde and paraformaldehyde buffered with PIPES. Next, a secondary fixative: buffered osmium tetra-oxide were applied on the cells. The processed cells were dehydrated by graded ethanol (30, 50, 70, 90%) and then with absolute ethanol. Now, the cells were moved through the propylene oxide and infiltered by resin mixture. The cells were embedded in a resin mixture in beam capsule and cured in the oven at 60–70 0 C for 48 h. The specimen in the beam capsule were trimmed and sectioned by ultramicrotomy instrument to obtain the ultra-thin sections of the specimens. The specimen sections were then transferred into the TEM grids and stained with uranyl acetate to enhance the contrast of the TEM micrographs. The grids were examined under TEM at an operating voltage of 80 KV. Note, the samples in triplicate were prepared to highlight the difference between control cells and nanoparticles-treated cells by performing a statistical analysis.
Results and discussion
Nanoporous 3 D SBA-16 (S-16) with cubic pores loaded with magnetic nanoparticles (SPIONs) has been investigated for potential multifunctional therapeutics. shows the physicochemical characteristics of SPIONs loaded SBA-16 analyzed using XRD diffraction patterns, vibrating sample magnetometer and nitrogen adsorption isotherms. 10 wt% of SPIONs loadings were carried out using enforced adsorption technique. X-ray diffraction between 15–30○ shows the presence of characteristics amorphous cubic framework. The presence of sharp Fe3O4 peaks was expected to be observed at 2 theta value of 35.45○. Fe/S-16 showed weak and broad diffraction indexed to that of non-crystalline Fe3O4 nanoparticles magnetite (PDF # 88–0866) (). Such transformation of iron oxides was expected considering the large surface area of SBA-16 that drive the finer distribution of particles inside the cage type of 3 D pores. The magnetic nature of Fe/S-16 was analyzed using vibrating sample magnetometer (VSM) (). The study confirmed the presence of super para magnetization with a magnetization value of 2.39 emu/g. An earlier study has shown that the presence of nanoclusters in a small batch at the pore walls forms superparamagnetic behaviour due to interactions between Fe3+ species, while large nanoclusters generate ferromagnetism [Citation13]. The presence of superparamagnetic behaviour with narrow hysteresis over SBA-16 shows the presence of small-sized nanoclusters at the walls of ink bottle-shaped cubic pores.
Figure 1. X-ray diffraction (a), vibrating sample magnetometer (b), nitrogen adsorption isotherm and pore size distributions of (c, e) SBA-16 and (d, f) Fe/S-16, respectively.
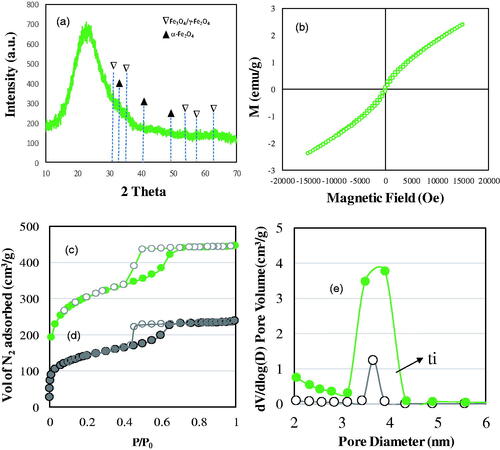
In order to confirm the textural characteristic change before and after SPIONs impregnation, the sample SBA-16 and Fe/S-16 was analyzed using nitrogen adsorption technique (). The isotherm pattern of S-16 shows a typical spinodal hysteresis pattern (type IV-H2) for ink bottle type of pores. After SPIONs impregnation, a significant decrease in the volume of nitrogen adsorption occurs indicating the occupation of SPIONs at the pore walls. This is reflected in the surface area and pore size variations. An initial surface area of SBA-16 decreases from 988 m2/g to 471 m2/g. Similarly, the cumulative surface area and pore volume decreased from 590 m2/g to 297 m2/g and 0.69 cm3/g to 0.37 cm3/g, respectively. Overall, about 50–52% decrease in the textural characteristics occurs after SPIONs impregnation. The cumulative pore volume showed a similar occupation (46%) compared to parent S-16. The textural modification after SPIONs impregnation shows the occupation at the pore walls that significantly affects the adsorption of nitrogen molecule leading to both surface and pore volume reduction behaviour. The abrupt decrease in the pore size distribution of SBA-16 showed the presence of SPIONs majorly at the pores averaged at 3.8 nm ().
shows the X-ray diffraction and FT-IR spectroscopy analysis of (a), Fe/S-16 (b) cisplatin and (c) Fe/S-16/A/Cp, respectively. Fe/S-16 showed broad diffractions characteristics of amorphous silica framework (). In case of cisplatin, the crystalline phase of Pt complex was clearly observed with characteristics diffraction patterns (). After the loading of cisplatin over Fe/S-16, the spectra remain similar to that of Fe/S-16 (). The absence of cisplatin peaks shows the effective phase transition from crystalline to amorphous phase during confinement into ink bottle-shaped pores of SBA-16. The TEM analysis shows that SPIONs deposition is unique based on the nanocarriers pore architecture. The average SPIONs particle size of the Fe/SBA-16 measured from TEM images was found to be in the range of 17 ± 1.83 nm.
Figure 2. X-ray diffraction and FT-IR spectroscopy analysis of (a) Fe/S-16 (b) cisplatin and (c) Fe /S-16/A/Cp, respectively.
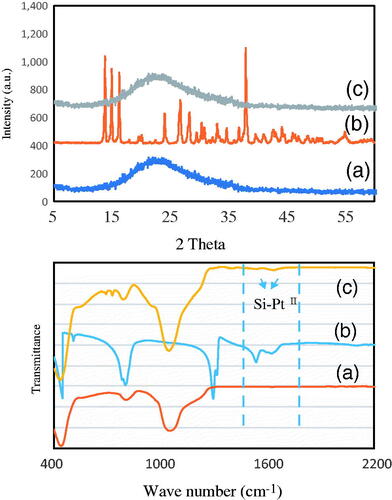
In order to study the cisplatin adsorption and release capabilities, different types of nanoformulations based on SBA-16, spherical silica, and mesocellular foam were used. In specific, (1) Cp/S-16 physical mix, (2) Cp-S-16, (3) Cp-F127/S-16, (4) Fe/S-16-A-Cp, (5) spherical silica-A-Cp, (6) Mesocellular foam-A-Cp and (7) Fe/S-16-AP-Cp (polyacrylic acid bound SBA-16) in normal saline at pH 5 for 24 h. In case of cisplatin adsorption, physical mixing with S-16 showed lowest Cp adsorption of ∼81% cisplatin. However, loading cisplatin directly over SBA-16 and difunctional copolymer Pluronic F127 containing S-16 slightly improved the adsorption to about 87%, respectively. On the other hand, functionalization of SBA-16 and spherical silica with 3-aminopropyltriethoxysilane silane showed increased adsorption to 91%, while mesocellular foam showed slightly reduced cisplatin adsorption of about 86%, respectively. The release profile of different nanoformulations was analyzed at similar cisplatin loading measured in terms of mmol of cisplatin per gram of nano support. The result shows that all the studied nanoformulations contains similar loadings of cisplatin per gram of nanomaterial in the absence and presence of 10 wt%SPIONs. However, the percentage cumulative cisplatin release profile shows a clear increasing trend, where Fe/S-16-A-Cp and Fe/S-16-AP-Cp nanoformulation exhibited highest cisplatin adsorption and release capacity ().
Figure 3. Cisplatin adsorption capacity and percentage cumulative release over different types of nanoformulations in normal saline pH 5 for 24 h.
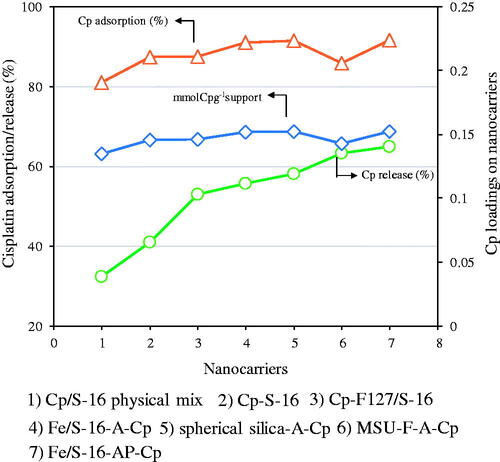
In vitro anti-cancer studies
Antitumour effects of Fe/S-16-A-Cp and cisplatin were studied in in vitro cancer cell lines, breast cancer (MCF-7) and normal cells (HHF-1). The LC50 values vary between the drug cisplatin and nano formulated drugs (Group-III, Group-IV and their control (Group-I)) (Figure S2, ). The cytotoxicity of Group-I is very low indicating their insignificant effect when they used in nanoparticle's construction. The sensitivity of MCF-7 and HFF-1 cells toward Group-IV is approximately the same, while the MCF-7 sensitivity to Group-III is higher than HFF-1 by 10.6 fold. On the other hand, HFF-1 is more sensitive towards cisplatin by 2.3-fold than MCF-7. In MCF-7, the sensitivity towards Group-IV is approximately 71.8-fold higher than its sensitivity towards Group-III, whereas it is more sensitive towards cisplatin by 5.36-fold than Group-IV. In normal cell line HFF-1, the cells were more resistant towards Group-III, the sensitivity to Group-IV is 741.8-fold higher than Group-III, but less by 13.37-fold than cisplatin. HFF-1 showed a resistant towards Group-III compare to MCF-7 cancer line.
Cells morphological studies
The treatment of HFF-1 and MCF-7 cells with Group-II showed that more than 80% of the cells were dead () compare to Group-I (control) (). HFF-1 treatment with Group-III () showed normal cell viability, even of the entry of the SPIONs particles inside the cells, which are pointed out by the characteristics iron red to brown colour. Whereas, in MCF-7 treatment () Group-III showed about 15% reduction in cell number. These data indicate a higher release of cisplatin in MCF-7 compare to HFF-1 and showed the poor release of cisplatin over all that might be due to the presence of pH-sensitive polyacrylic acid. Group-IV SPIONs were also detected inside the cells and showed a high number of cells killing (more than 60%) after 24-h treatment () in both cell lines. This indicates the effective release of cisplatin in Group-IV rather than in the presence of polyacrylic acid at pH 7.4.
Figure 4. Cell morphology of treated HFF-1 and MCF-7 cells. (a, e) Fe/S-16 (Control), (b) Cisplatin treatment (G-II) (0.018 mg/ml), (c) Fe/S-16-AP-Cp treatment (G-III) (0.24 mg/ml), (d) Fe /S-16-A-Cp treatment (G-IV) (0.24 mg/ml). (f) Cisplatin treatment (G-II) (0.041 mg/ml), (g) Fe/S-16-AP-Cp treatment (G-III) (0.22 mg/ml), (h) Fe/S-16-A-Cp treatment (G-IV) (0.22 mg/ml). Yellow arrow shows the SPIONs inside the cells.
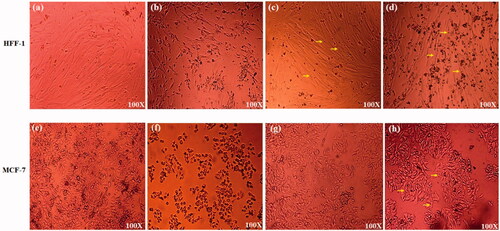
Nuclear morphology study
Confocal microscopic images for treated HFF-1 and MCF-7 with Group-I (control) showed normal nucleus () indicating the minor effect of Group-I on these cells. While treating these cells with cisplatin (Group-II) showed nuclear condensation (White arrow) and cell number reduction in both cell lines (). Treated cells with Group-III showed a reduction in cell number in both cell lines (). In addition, Group-III showed nuclear fragmentation/apoptotic bodies (red arrow), condensed DNA (White arrow) and the ring A stage of the disassembly of DNA (yellow arrow) in MCF-7 cells but not in HFF-1. Indicating the higher sensitivity of MCF-7 to Group-III than HFF-1. On the other hand, when the cells treated with Fe/S-16-A-Cp (G-IV) the cell number was dramatically reduced to less than 20% with disintegrated nuclear morphology (red arrows) (). The control cells showed the normal nuclear structure and no sign for apoptosis, while treated cells with Group-IV showed high nuclear fragmentation, chromatin condensation, ring A stage of the of DNA disassembly and reduction in cell number. These results indicate that Group-IV based nanoformulation is effective against breast cancer cell line MCF-7.
Figure 5. Hoechst staining of treated HFF-1 and MCF-7 cells, the density stained areas are cell nuclei (blue color). (a, e) Group-I treatment (control), (b) Cisplatin treatment (G-II) (0.018 mg/ml), (c) Fe/S-16-AP-Cp treatment (G-III) (0.24 mg/ml), (d) Fe/S-16-A-Cp treatment (G-IV) (0.24 mg/ml), (f) Cisplatin treatment (G-II) (0.041 mg/ml), (g) Fe/S-16-AP-Cp treatment (G-III) (0.24 mg/ml), (h) Fe/S-16-A-Cp treatment (G-IV) (0.24 mg/ml). The arrows indicate the nucleus structural changes. White arrow: DNA condensation and red arrow: nuclear fragmentation/apoptotic bodies and yellow arrow: the ring A stage of the DNA disassembly.
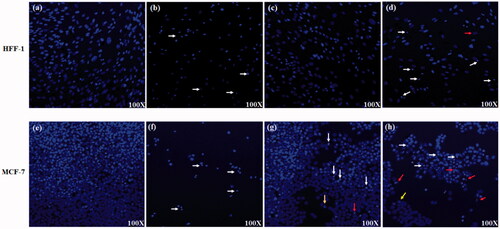
TEM analysis of nanoparticles treated cells
In order to underline the damage done by nanoparticles, the nanoparticles treated cancer cells were examined by TEM. For the sake of comparison, the structure and morphology of untreated cancer cells (control) were examined at two magnification (). The MCF-7 cell membrane was normal, regular and smooth, as described in another study [Citation14]. The average size of the MCF-7 is 5 μm and 1 μm for the nucleus, which appeared compact and darker than the other features of the cell. The control cells maintained their morphology [Citation15], for example, nonuniform and dotted contrast of cytoplasm and undamaged cell wall; see dark circular contrast around the cell as marked by red arrows. The thickness of the cell wall is estimated safely under 100 nm ().
Figure 6. Morphology of the nanoparticle-treated MCF-7 cells examined by TEM. (a, b) Morphology and structure of the controlled MCF-7 cells examined by TEM at two magnifications. The scale bars are 2 and 1 µm. The nucleus and cell wall show dark contrast within the cell. (a) The cell wall was marked with a pair of red arrows in the magnified image. (b) The average size of the cell was ∼ 5 μm and cell wall thickness ∼100 nm. (c–h) MCF-7 treatment with Fe/S-16-A-Cp treatment (G-IV). (c–d) TEM images are showing the severe damage of the cells, especially the cell walls. (e–g) The particles were penetrated inside the cells (cytoplasm) either with silica scaffold (red arrow) or after releasing from the carrier (black arrows). (h) TEM of nanoparticles penetrating the nucleus (red arrow) and lead to the cell death, Cy (cytoplasm), N (nucleus).
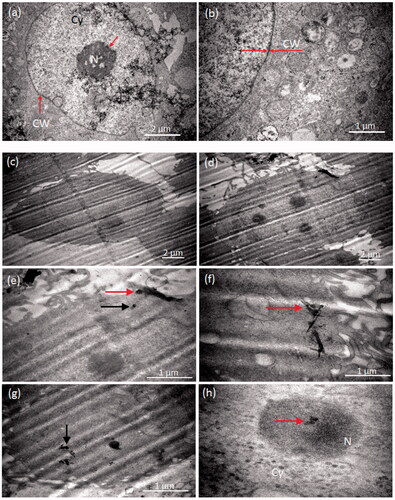
The cancer cell lost their native morphology when examined after treatment with the nanoparticles (). TEM micrographs shown in display the substantial damage of the cells especially the cell walls. The wall was ruptured severely and lost its circular morphology compared to the control cells (). The particles were penetrated inside the cells either with silica scaffold (red arrow) or after release from the carrier (black arrows). The nanocarrier was also penetrated inside the cell in a few cases ( and Citation7). The maximum width of the carrier was found around 100 nm and longitudinal length under one micrometre (). Few nanoparticles were seen attached to the scaffold as highlighted by red arrow. shows the silica carrier inside the cell as well as inside the nucleus (). Interestingly, it was found that the particles were entered in the cell nucleus (as marked with red arrow) and lead to cell death. showed the severe damage of the cell wall and high penetration of nanoparticles into the cell cytoplasm.
Figure 7. TEM observation on silica carrier inside the cell at different magnifications. The nanocarrier was penetrated inside the cell. The maxim width of the carrier was estimated around 100 nm and longitudinal length under one micrometre. Few nanoparticles were seen attached to the scaffold as highlighted by red arrows.
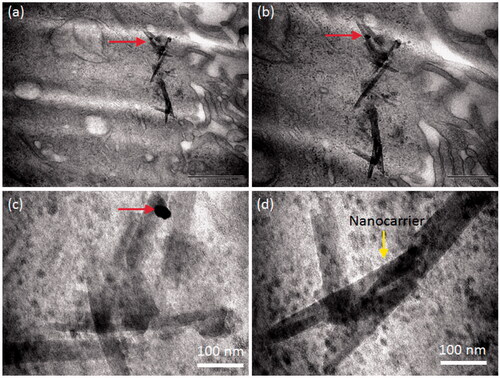
Conclusions
The application of Fe/S-16 nanoformulations as targeted multifunctional drug delivery vehicle has been demonstrated with cisplatin drug. 10 wt.% SPIONs impregnation over S-16 showed the presence of SPIONs as nanostructured magnetite (17 ± 1.83 nm) at the cubic pore walls of SBA-16 with magnetization value of 2.39 emu/g. Fe functionalized with amine and pH-sensitive polymer exhibited the highest cisplatin adsorption (90%) and release ability (60%). in vitro results indicate that Fe/S-16-A-Cp (Group-IV) based nanoformulation is effective against breast cancer. Fe/S-16-A-Cp enters MCF-7 cell cytoplasm and nucleus resulting in cell wall damage, nuclear fragmentation and DNA condensation. In addition, MCF-7 is more sensitive towards Fe/S-16-AP-Cp than HFF-1, with observed nuclear fragmentation and DNA condensation in confocal microscopy. These data indicate the high release of cisplatin of Fe/S-16-A-Cp in cells compared to Fe/S-16-AP-Cp, which showed higher release in MCF-7 than HFF-1. The study indicates the suitability of designed nanoformulation for breast cancer disease and can be further validated in in vivo experiment to provide new insight about targeted drug therapeutics.
Author contributions
B. Rabindran Jermy designed and optimized the nanoformulations in drug delivery study. M. Alomari performed data analysis and discussed about the MTT assay to assess viability of MCF-7 and HFF-1 cell line after treatment with different conditions, also Alomari wrote and reviewed the draft and added comments. V. Ravinayagam concept designed in vitro cancer cell line study, MTT data analysis, DAPI staining and scientific discussion. Sultan Aktar performed TEM experiments, analysis and wrote TEM part as well as reviewed the manuscript thoroughly. S. A. Almofty performed Hoechst staining and involved in experimental discussion. Hiba Bahmdan helped in MTT and confocal work and analysis. J. F. Borgio and Suriya Rehman reviewed the draft and provided valuable comments. S. Azeez reviewed the final draft and contributed in scientific writing.
1supplimentary-file.docx
Download ()Acknowledgement
V. Ravinayagam likes to acknowledge Deanship of Scientific Research grant [2017–111-DSR], Imam Abdulrahman Bin Faisal University. B. Rabindran Jermy like to acknowledge Institute for Research and Medical Consultations department funding for the year 2017.
Disclosure statement
The authors declare no competing interests.
Additional information
Funding
References
- Prager GW, Braga S, Bystricky B, et al. Global cancer control: responding to the growing burden, rising costs and inequalities in access. ESMO Open. 2018;3:e000285.
- Zhou Y, Quan G, Wu Q, et al. Mesoporous silica nanoparticles for drug and gene delivery. Acta Pharmaceutica Sinica B. 2018; 8: 165–177.
- Hasan A, Morshed M, Memic A, et al. Nanoparticles in tissue engineering: applications, challenges and prospects. IJN. 2018;13:5637–5655.
- Patra JK, Das G, Fraceto LF, et al. Nano based drug delivery systems: recent developments and future prospects. J Nanobiotechnol. 2018;16:71.
- Vallabani NVS, Singh S. Recent advances and future prospects of iron oxide nanoparticles in biomedicine and diagnostics. 3 Biotech. 2018;8:279.
- Abbasi Aval N, Pirayesh Islamian J, Hatamian M, et al. Doxorubicin loaded large-pore mesoporous hydroxyapatite coated superparamagnetic Fe3O4 nanoparticles for cancer treatment. Int J Pharm. 2016;509:159–167.
- Egodawatte S, Dominguez S, Larsen SC. Solvent effects in the development of a drug delivery system for 5-fluorouracil using magnetic mesoporous silica nanoparticles. Micropor Mesopor Mater. 2017;237:108–116.
- Zeleňák V, Halamová D, Zeleňáková A, et al. Periodic 3D nanoporous silica modified by amine or SPION nanoparticles as NSAID delivery system. J Porous Mater. 2016;23:1633–1645.
- Jermy BR, Ravinayagam V, Akhtar S, et al. Magnetic mesocellular foam functionalized by curcumin for potential multifunctional therapeutics. J Supercond Nov Magn. 2019; 32:2077–2090.
- Jermy BR, Acharya S, Ravinayagam V, et al. Hierarchical mesosilicalite nanoformulation integrated with cisplatin exhibits target-specific efficient anticancer activity. Appl Nanosci. 2018;8:1205–1220.
- Hayat MA. Principles and techniques electron microscopy biological applications. Biological imaging. 4th ed. Cambridge, UK: Cambridge University Press; 2000.
- Kuo J, editor. Electron microscopy: methods and protocols. 3rd ed. Totowa, NJ: Humana Press; 2014.
- Cuello NI, Elías VR, Mendieta SN, et al. Drug release profiles of modified MCM-41 with superparamagnetic behavior correlated with the employed synthesis method. Mater Sci Eng C. 2017;78:674–681.
- Morse DL, Gray H, Payne CM, et al. Docetaxel induces cell death through mitotic catastrophe in human breast cancer cells. Mol Cancer Ther. 2005;4:1495–1504.
- Zhang L, Cai Q, Lin J, et al. Chloroform fraction of Scutellaria barbata D. Don promotes apoptosis and suppresses proliferation in human colon cancer cells. Mol Med Rep. 2014;9:701–706.