Abstract
Objective
Antibiotics have saved hundreds of millions of patient’s lives, but there are also many side effects. This paper mainly studies the regulation of Chinese yam on antibiotic-induced gut dysbiosis in rats.
Methods
Male Wistar rats were divided into three groups, normal control group, antibiotic group, and Chinese yam group. In the antibiotic group and the yam group, a rat model of intestinal dysfunction was established by intragastric administration of imipenem/cilastatin sodium (antibiotic) for 21 days. The yam group was also given yam decoction for intervention. The fecal samples of each group of rats were analyzed using an ultra-performance liquid chromatography quadrupole/time-of-flight mass spectrometry (UPLC-Q-TOF/MS) method to find potential biomarkers. The DNA in the feces was extracted, and analyzed using 16S rDNA method to find the differential flora. Spearman correlation analysis was performed on differential flora and differential metabolites to determine the main metabolic pathways of the host after intestinal flora dysregulation.
Results
The administration of antibiotics can lead to disorders of intestinal flora, decreased microbial diversity, and changes in host metabolic phenotype. Chinese yam can significantly improve the intestinal flora, making the host's metabolic phenotype tend toward normal.
Conclusion
Long-term use of Imipenem/cilastatin sodium can cause disturbance of intestinal flora in normal rats. Chinese yam may regulate intestinal flora disorder and have potential prebiotic effects.
Introduction
The discovery, large-scale production, and use of antibiotics are tremendous advances in the history of human medicine. Antibiotics have saved hundreds of millions of patient’s lives, but there are also many side effects, such as Clostridium difficile infection, antibiotic resistance and change in intestinal flora [Citation1]. Studies have shown that oral antibiotics in healthy adults could cause the decline of intestinal flora diversity and also drastic changes in its composition and structure; for example, excessive growth of Streptococcus and Lactobacillus, as well as reduction of bacteria with important metabolic functions (such as butyric acid producing bacteria) [Citation2–5]. Changes on the structure and composition of the flora caused by antibiotics can be restored to a level close to the pre-antibiotic intervention after 1.5 months, but it is difficult to fully recover [Citation6]. Moreover, 7 days of continuous antibiotic use will lead to drug resistance [Citation7]. The disturbance of antibiotics to the intestinal flora in mice increased the incidence of obesity, insulin resistance and liver diseases associated with high-fat diets [Citation8]. Therefore, finding and discovering drugs that alleviate the flora disorder caused by antibiotics is essential for relieving antibiotic side effects.
Chinese yam is the dried rhizome of Dioscorea opposita Thunb., commonly known as Chinese yam. It was first recorded in the Shen Nong Ben Cao Jing. It is classified as high-grade (has tonifying effects with little to no toxins), with a sweet flavor, and neutral in nature. It mainly manifests its therapeutic actions in the Spleen, Lung and Kidney meridians. In TCM theory, it has the effect of invigorating Spleen, nourishing Stomach and invigorating Lung and Kidney. Studies have shown that Chinese yam extract could prevent the damages of the liver and kidney [Citation9], and allantoin (a major metabolic intermediate produced from uric acid) can help prevent skeletal muscle dysfunction by stimulating energy metabolism [Citation10], as well as allantoin may play an important role on antioxidative and antitumor capacity in yam peel [Citation11]. Diosgenin (extracted from the tubers of Dioscorea wild yam) has protective effects on fructose-induced renal damage by regulating SIRT3-mediated oxidative stress, renal fibrosis, lipid metabolism and inflammation [Citation12]. Chinese Yam polysaccharide can improve the survival rate of sperm and protect the integrity of sperm DNA [Citation13]; and can also improve experimental colitis induced by 2,4,6-trinitrobenzene sulfonic acid (TNBS) in rats by regulating the composition and functional characteristics of intestinal flora [Citation14].
In this study, we used an antibiotic-induced intestinal dysbiosis rat model to investigate the effects of microbial imbalance on the composition of fecal metabolites. The 16S rDNA gene sequencing and UPLC-Q-TOF/MS-based metabolomics method was used to comprehensively investigate the protective effect of Chinese yam on the metabolic phenotype in the intestinal dysbiosis rat model.
Materials and methods
Materials
Experimental instruments
Maxis HD Q-TOF (Quadrupole Time-of-Flight) Mass Spectrometry (Bruker, Germany); Diane Ultimate 3000 Ultra-High Performance Liquid Chromatography (equipped with quaternary pump, online degasser, automatic sampler, UV detector, Chromeleon workstation; Dionex, USA); Advantage A10 Ultra-Pure Water Meter (Sartorius, Germany); 5810R High-speed refrigerated centrifuge (Eppendorf, Germany); SK-1 Rapid Mixer (Changzhou Guoyu Instrument Manufacturing Co., Ltd., China); BCD-206TAS Low-temperature refrigerator (Haier Company, China); Pipette (Gilson, France); Analytical balance (Metler -Toledo Instruments; AB204-N); IVC Independent Ventilation System (Suzhou Fengshi Laboratory Animal Equipment Co., Ltd, China); Normal-temperature centrifuge (Eppendorf Centrifuge 5424, Germany); WH-861 Vortex Shaker (Taicang Hualida Experimental Equipment Co., Ltd., China); DK-8D Three Temperatures Three Controls Constant Temperature Water Bath (Shanghai Boxun Industrial Co., Ltd., China); A200 Gene Amplifier (Hangzhou Lange Scientific Instruments Co., Ltd., China); EPS 300 Electrophoresis Instrument (Tanon Science & Technology Co., Ltd. China); Tanon-2500 Gel Imager (Tanon Science & Technology Co., Ltd. China).
Experimental reagent
Pusion Hot start flex 2X Master Mix (M0536L, Shanghai Yitao Biological Instruments Co., Ltd., China); DL2000 DNA Maker (3427A, Takara, Japan); Gene color (GBY-1, Beijing Jinboyi Biotechnology Co., Ltd., China); Qubit dsDNA HS Assay Kit (Q32854, Invitrogen, Life Technologies, USA); Biowest Agarose G-10 (111860, Biowest, France); 50× TAE Buffer (B548101-500, Biotechnology Bioengineering (Shanghai) Co., Ltd., China); AxyPrep PCR Cleanup Kit (35314KB1, Axygen, Life Science Research, USA); Stool DNA Kit (D4015-02, Omega Bio-tek, USA); 95% ethanol (Xinxiang Sanwei Disinfectant Co., Ltd., China); methanol, acetonitrile, formic acid (Fisher Science World Company, USA); rat gastrin(Gas) ELISA Kit, rat motilin(MTL) ELISA Kit (Bioswamp Biotechnology Co., Ltd., China); activated charcoal (Tianjin Zhiyuan Chemical Reagent Co., Ltd., China).
Animal
The study was conducted in accordance with the Regulations of Experimental Animal Administration issued by the State Committee of Science and Technology of the People’s Republic of China. Twenty-four SPF-grade male Wistar rats weighing 100 ± 10 g were purchased from Beijing Weitonglihua Laboratory Animal Technology Co., Ltd. under the license number: SCXK (Beijing) 2016-0011. The animals were fed adaptively for 1 week at 18–22 °C in a clean animal laboratory, with access to food and water 24 h a day.
Experimental medication
Chinese yam is the dried rhizome of Dioscotea opposita Thunb.. As well as it were collected from Jiaozuo City, China, and identified by Professor Chen Suiqing, Henan University of Chinese Medicine. A voucher specimen (No. 20160715A) was deposited in the Research Department of Natural Medicine. The extraction method of yam extract is as shown in the literature [Citation15,Citation16], Briefly: 1 kg of Chinese yam, add 10 L of water and heated to boiling three times, each time for half an hour, After each 30 min boiling cycle, the filtrate was filtered out and collected in a common container. The total volume of the three filtrations was then concentrated under reduced pressure, freeze–drying to obtain the complete extract of Chinese yam. The total yield was 24.31%.
Imipenem/cilastatin sodium, Hangzhou Merck Pharmaceutical Co., Ltd., batch number: National Drug Standard J20130123.
Animal grouping and intervention administration
After a week of adaptive feeding, Wistar rats were divided into three groups according to the principle of weight uniformity: normal control group (NC), antibiotic group (A), and Chinese yam group (ADR), with six rats in each group. Group A was treated with 50 mg/kg of Imipenem/cilastatin sodium aqueous solution [Citation17,Citation18]. In the ADR group, 50 mg/kg of Imipenem/cilastatin sodium aqueous solution and 3.4 g/kg Chinese yam extract were given. The dosage volume was 1 mL/100 g of body weight. The NC group was given saline of equal volume. The intervention was administered by gastric lavage once daily for 21 consecutive days.
Measurement of intestinal transit
Rat were administered activated charcoal (0.5 g per 10 mL in 0.5% methylcellulose; 0.1 mL per 10 g body weight by oral gavage). After 20 min, the rats were anesthetized with 10% chloral hydrate and the distance travelled by charcoal was measured.
Collection and treatment of feces
24 h after the last administration, fecal samples of rats from each group were collected by abdominal pressing and stored in liquid nitrogen without enzymes. Immediately after collection was completed, the stool samples were transferred to a low-temperature refrigerator for storage at –80 °C.
UPLC-Q-TOF/MS analysis
Fecal samples of 100 mg were ground on ice and added 500 μL of ice water, shaken in a vortex, and centrifuged at 12,000 r/min for 15 min; Then the supernatant was collected by pipette. Secondly, 500 μL of cold methanol was added to the precipitate, shaken in a vortex, centrifuged at 12,000 r/min for 15 min, and the supernatant was collected. The two supernatant volumes were combined and centrifuged at 12,000 r/min for 15 min. The final supernatant was collected in a vial for analysis by UHPLC-Q-TOF/MS. A 50 μL sample was extracted from each fecal specimen and mixed to make quality control (QC) samples. The QC samples were tested once every six specimens to monitor and analyze the stability of the system.
Chromatographic analysis was performed using the Diane Ultra 3000 UPLC system. Fecal samples were analyzed by RSLC 120 C18 column (2.1 × 100 mm, 2.2 μm) with a column temperature of 40 °C and a flow rate of 0.45 mL/min. The mobile phase consisted of solvent A (95% H2O/5% acetonitrile + 0.1% formic acid containing 2 mM ammonium formate) and B (95% acetonitrile/5% H2O + 0.1% formic acid containing 2 mM ammonium). The gradient scheme is optimized as follows: 0–0.5 min, 1% B, 0.5–5 min, 1%–30% B, 5–13 min, 30%–50% B, 13–17 min, 50%–100% B, 17–19 min, 100% B washing, 19–20 min, 1% B balance. The column eluent is directed to the mass spectrometer without separation.
Maxis HD Q-TOF mass spectrometry was used for mass spectrometry analysis in positive ion mode using electrospray ionization ion source technique. Capillary voltage was 3.0 kV; The sampling and extraction cone-hole voltages were 40 V and 4.0 V, respectively; The eluent gas flow rate and temperature were 800 L/h and 400 °C; The flow rate of conical hole gas was 40 L/h; The ion source temperature was 100 °C; The scan time and inter-scan delay were 0.15 and 0.02 s, respectively. In all analyses ([M + H]+ = 556.7277), leucine-enkephalin was calibrated at a concentration of 0.5 μg/mL and a flow rate of 5 μL/min. Data of mass-to-charge ratios (m/z) between 50 and 1500 were collected by centroid model.
16S rDNA microbial community analysis
DNA extractions
DNA from different samples was extracted using the E.Z.N.A.® Stool DNA Kit (D4015, Omega, Inc., USA) according to manufacturer’s instructions. The reagent, which was designed to uncover DNA from trace amounts of sample, has been shown to be effective for the DNA preparation of most bacteria. Nuclear-free water was used for the blank sample. The total DNA was eluted in 50 µL of elution buffer and stored at –80 °C until measurement in the PCR by LC-Bio Technology Co., Ltd, Hang Zhou, Zhejiang Province, China.
PCR amplification and 16S rDNA sequencing
The V3-V4 region of the prokaryotic (bacterial and archaeal) small sub-unit (16S) rRNA gene was amplified with slightly modified versions of primers 338 F (5′-ACTCCTACGGGAGGCAGCAG-3′) and 806 R (5′-GGACTACHVGGGTWTCTAAT-3′) [Citation19]. The 5′ ends of the primers were tagged with specific barcodes for sample and sequencing universal primers. PCR amplification was performed in a total volume of 25 µL reaction mixture containing 25 ng of template DNA, 12.5 µL PCR premix, 2.5 µL of each primer, and PCR-grade water to adjust the volume. The PCR conditions to amplify the prokaryotic 16S fragments consisted of an initial denaturation at 98 °C for 30 s; 35cycles of denaturation at 98 °C for 10 s, annealing at 54 °C for 30 s, and extension at 72 °C for 45 s; and then final extension at 72 °C for 10 min. The PCR products were confirmed with 2% agarose gel electrophoresis. Throughout the DNA extraction process, ultrapure water, instead of a sample solution, was used to exclude the possibility of false-positive PCR results as a negative control. The PCR products were purified by AMPure XT beads (Beckman Coulter Genomics; Danvers, MA, USA) and quantified by Qubit (Invitrogen, USA). The amplicon pools were prepared for sequencing and the size and quantity of the amplicon libraries were assessed on the Agilent 2100 Bioanalyzer (Agilent, USA) and with the Library Quantification Kit for Illumina (Kapa Biosciences; Woburn, MA, USA), respectively. PhiX Control library (v3) (Illumina) was combined with the amplicon library (expected at 30%). The libraries were mostly sequenced on 300PE MiSeq runs with one library being sequenced with both protocols using the standard Illumina sequencing primers, eliminating the need for a third (or fourth) index read.
Statistical analysis
The original data collected by UHPLC-Q-TOF/MS was imported into Profile Analysis software for peak matching, noise reduction, normalization, correction of missing values and other data preprocessing to obtain a Bucket table. The tabular data was imported into the SIMCA-P13.0 software for unsupervised principal component analysis (PCA) and supervised orthogonal partial least squares discriminant analysis (OPLS-DA), and the corresponding score map was obtained. The differences between NC and A groups were compared by score plots, and the different metabolites (VIP > 3.5, p < .05) were screened. Potential biomarkers were confirmed and identified using online databases such as METLIN (https://metlin.scripps.edu/) and Human Metabolome Database (HMDB) (http://www.hmdb.ca/spectra/ms/search). Semi-quantitative analysis (heat map) and cluster analysis of potential biomarkers by Mev (version 4.8.0) software were performed. Finally, the metabolic pathway databases such as KEGG (http://www.genome.jp/kegg/) and MetaboAnalyst (http://www.metaboanalyst.ca/) were used to perform enrichment analysis and network construction of related metabolic pathways.
Samples were sequenced on an Illumina MiSeq platform, according to the manufacturer's recommendations, provided by LC-Bio. Paired-end reads was assigned to samples based on their unique barcode and truncated by cutting off the barcode and primer sequence. Paired-end reads were merged using FLASH. Quality filtering on the raw tags were performed under specific filtering conditions to obtain the high-quality, clean tags according to the FastQC (V 0.10.1). Chimeric sequences were filtered using Verseach software (v2.3.4). Sequences with ≥97% similarity were assigned to the same operational taxonomic units (OTUs) by Verseach (v2.3.4) [Citation20]. Representative sequences were chosen for each OTU, and taxonomic data were then assigned to each representative sequence using the RDP (Ribosomal Database Project) classifier. The differences of the dominant species in different groups, multiple sequence alignment were conducted using the PyNAST software to study phylogenetic relationship of different OTUs. OTUs abundance information was normalized using a standard of sequence number corresponding to the sample with the least sequences. Alpha diversity is applied in analyzing the complexity of species diversity for a sample through 4 indices, including Chao1, Shannon, Simpson, and Observed species (Supplementary material). All these indices in our samples were calculated with QIIME (Version 1.8.0). Beta diversity analysis was used to evaluate the differences in species complexity of samples. Beta diversity was calculated by principle co-ordinates analysis (PCoA) and cluster analysis by QIIME software (Version 1.8.0)
Spearman correlation analysis was carried out on the second metabolite screened by metabonomics and 16S sequencing analysis of the significant difference generic level bacterial flora, and the relationship between the difference bacterial flora, metabolites, metabolites and the difference bacterial flora was obtained. Based on the calculation results, the appropriate screening conditions were selected to obtain the final correlation and a network map between the final differential flora and metabolites.
One-way ANOVA was performed using the Social Science Statistics Software Package (SPSS 16.0, Chicago, USA). The significance threshold of this experiment was p < .05. After the end of the data analysis, use the Graphpad prism7.0 software to map.
Results
Effect of Chinese yam on body weight, urine volume and small intestinal propulsion (SIP) function of rats with antibiotic-induced intestinal dysbiosis
From the general condition of rats, we know that: Compared with the NC group, rats in group A showed significant diarrhea, slow weight gain, and decreased SIP function; Compared with the A group, the body weight, urine volume and SIP function of the ADR group were significantly improved. The result is shown in .
Figure 1. The effects of Chinese yam on body weight, urine volume, and SIP function in rats with antibiotic-induced intestinal dysbiosis. (a) Body weight: Compared with the NC group, the weight gain of the rats in group A was slow and the body weight was light (p < .01); Compared with group A, the weight of rats in the ADR group increased steadily and the body weight increased significantly (p < .05). (b) Urine volume: Diarrhea occurred in rats after administration of antibiotics, and urine volume decreased significantly (p < .05); After the administration of antibiotics and Chinese yam, the incidence of diarrhea in rats was decreased and the urine output returned to normal (p < .05). (c) SIP function: Compared with NC group, the SIP function of group A was weakened (p < .01); Compared with group A, the SIP function of ADR group was improved (p < .01).
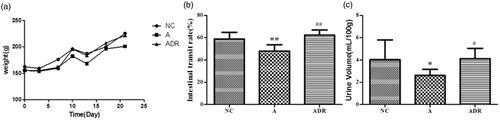
Effect of Chinese yam on antibiotic-induced intestinal dysbiosis
The composition of fecal microbiota was analyzed by 16S rDNA gene sequencing. Sequences with ≥97% similarity were assigned to the same OTUs, representing a species, and the similarities and differences between different samples were counted at the OTU level, and the common or unique OTUs among different samples are shown by the Venn diagram (). Compared with the NC group (2774), the diversity of the microbiome in the A group was decreased (1498); The diversity of the microbiome in the ADR group tends to return to normal.
Figure 2. Effect of Chinese yam on antibiotic-induced intestinal dysbiosis in rats. (a) Veen diagram: The number of OTUs in this area is represented by the number of OTU. The NC group is red, the A group is yellow, and the ADR group is green. (b) PCoA: After the administration of antibiotics, the A and NC groups were each clustered together and separated, while the ADR group clearly deviated from the A group and tended to return to normal. (c, d) Species classification histogram: After administration of antibiotics, the intestinal flora of the A group showed significant changes; while that of the ADR group tended to return to normal. The Bray-Curtis distance clustering of species abundance showed that the NC and ADR groups were grouped together and deviated from the A group.
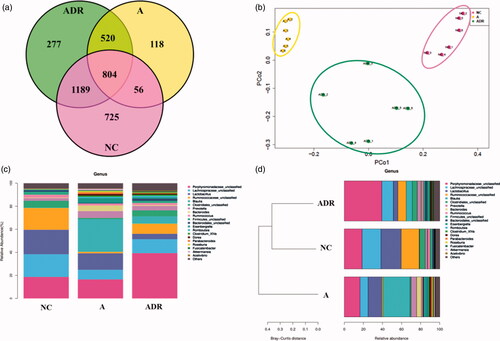
Principal coordinate analysis (PCoA) was used to compare the colonial differences (). By comparing the histogram of species classification, we found that there were significant differences in the flora between A group and NC group at the generic level (). Bray-Curtis distance clustering on top 20 species abundance was used to observe the relationship between species classification and sample distance (). From the figure we can draw: the species composition also tends to normal.
Effect of Chinese yam on the fecal metabolomics in rats with antibiotic- induced intestinal dysbiosis
UPLC-Q-TOF/MS was used to detect the metabolic characteristics of fecal samples from rats with antibiotic-induced intestinal dysbiosis. The PCA model was established to evaluate the metabolic patterns of antibiotic-induced intestinal dysbiosis in rats (). The metabolic profile of the ADR group was significantly different from that of the A group, but similar to the NC group, indicating that the bias induced by antibiotics was significantly improved after ADR intervention. The OPLS-DA and S-Plot diagrams based on the fecal metabolic patterns of rats in NC and A groups can be found in . Using an online database for screening, 23 potential biomarkers involved in intestinal dysibiosis were screened. These metabolites were: L-Urobilin (M1); 3-Methyldioxyindole (M2); 6-Hydroxyhexanoic acid (M3); N-acetylmuramate (M4); Xanthine (M5); DL-Glutamate (M6); 9, 10, 13-TriHOME (M7); Cortolone (M8); Gluconic acid (M9); Sulfate (M10); 9, 10-DHOME (M11); (S)-2-Acetolactate (M12); Cholic acid (M13); L-Leucine (M14); L-Valine (M15); Acetylcholine (M16); Succinic acid (M17); N-Acetylputrescine (M18); N1-Methyl-2-pyridone-5-carboxamide (M19); Beta-D-Glucose/Alpha-D-Glucose (M20); Phosphodimethylethanolamine (M21); 5-Phosphoribosylamine (M22); and L-Tyrosine (M23). The heat maps of potential biomarkers were performed using Mev (version 4.8.0) software (.
Figure 3. Effect of Chinese yam on the fecal metabolomics of antibiotic-induced intestinal dysbiosis in rats. (a, b) PCA: (a), Positive mode (R2X = 0.752, Q2 = 0.468); (b), Negative mode (R2X = 0.952, Q2 = 0.874). The metabolic profile of the ADR group was significantly different from that of the A group, but similar to the NC group, indicating that the bias induced by antibiotics was significantly improved after ADR intervention. (c, d) OPLS-DA: (c) positive mode (R2X = 0.69, R2Y = 0.981, Q2 = 0.884); (d), negative mode (R2X = 0.944, R2Y = 1, Q2 = 0.978). The sample points of the NC and A groups were obviously separated in the scoring chart, which indicates that the antibiotics have an obvious effect on the metabolism of the NC group rats, which has analytical value. (e, f) S-Plot: (e), positive mode; (f), negative mode. In the S-plot load graph, metabolite points that are significantly far from the origin (VIP > 1; p < .05) were selected as potential markers and then screened and identified by the online database.
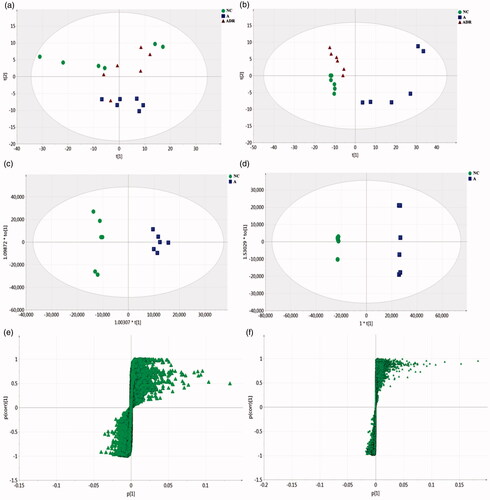
Correlation analysis of fecal metabolites related to intestinal dysbiosis and changes in intestinal microbiota
Spearman correlation analysis (r > 0.5) was used to analyze the significant difference between secondary metabolites screened from metabolomics and genus-level flora with significant differences obtained by 16S sequencing analysis. It was found that there was a clear correlation between the disturbed microbiome and fecal metabolites, as shown in . DL-Glutamate (M6) was positively correlated with the genus Erysipelotrichaceae_incertae and Akkermansia; It was negatively correlated with Intestinibacter, Porphyromonadaceae and Clostridiales; 3-Methyldioxyindole (M2) is positively correlated with the genus Erysipelotrichaceae_incertae_sedis and Christensenella, and negatively correlated with the Porphyromonadaceae, (S) -2-Acetolactate (M12) is positively correlated with Phocea and Akkermansia, negatively correlated with Erysipelotrichaceae and Clostridia Succinic acid (M17) is negatively correlated with Porphyromonadaceae and Barnesiella. L-Leucine (M14) is positively correlated with Bittarella and Akkermansia. L-Valine (M15) is negatively correlated with Bittarella. In conclusion, the administration of antibiotics can have a significant interference with the microbiome, and the metabolites and metabolic pathways of different microorganisms demonstrate that the administration of antibiotics significantly alters the microbiome’s metabolic phenotype.
Figure 5. Heat map of correlation between different intestinal flora and different fecal metabolites in NC and A groups. Heat maps were used to represent the relationship between different flora and fecal metabolites in the NC and A groups, with r > 0.5. (a) The correlation between negative ion metabolites; (b) The correlation between positive ion metabolites. Red indicates a positive correlation and blue indicates a negative correlation. The darker the color the stronger the correlation.
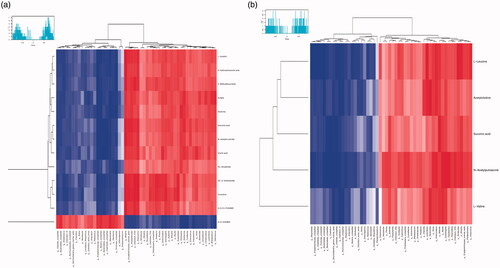
Validation of key metabolic pathways related to intestinal dysbiosis
To further verify that the key metabolic pathway identified by correlation analysis is the most relevant pathway for intestinal dysbiosis, a complete metabolic network was constructed by integrating all potential biomarkers found in this study. The importance of these pathways to the development of intestinal dysbiosis was assessed by the response value of MetPA analysis (. The results indicate that there were five metabolic pathways most relevant to antibiotic-induced intestinal dysbiosis (response value > 0.2), namely: Valine, leucine and isoleucine biosynthesis; Sulfur metabolism; Phylalanine, tyrosine and tryptophan biosynthesis; Alanine, aspartate and glutamate metabolism; D-Glutamine and D-glutamate metabolism. Among them, three metabolic pathways were identified by statistical correlation analysis and MetPA analysis: Valine, leucine and isoleucine biosynthesis; Phenylalanine, tyrosine and tryptophan biosynthesis; and Alanine, aspartate and glutamate metabolism. These three metabolic pathways are the key metabolic pathways for antibiotic-induced intestinal dysbiosis.
Discussion
Lmipenem/cilastatin sodium as a mixture antibiotic which could prevent and treat some serious disease caused by aerobic/anaerobic bacteria for its more safety and less adverse reaction [Citation21]. But current research shows that imipenem can cause kidney toxicity and even urolithiasis [Citation22]. As well as it could induced an oxidative stress-status and histopathological changes in the testis and altered spermatogenesis in particular at both 50 and 80 mg/kg dose-levels [Citation23]. A study on the analysis of urine samples collected from cynomolgus monkey dosed with imipenem revealed a significant increase of β-hydroxybutyrate and ketone bodies, indicating a disruption of energy metabolism resulting from the suppression of gut microflora [Citation24]. Research shows: normal Wistar rats were exposed to a broad spectrum β-lactam antibiotic imipenem/cilastatin sodium, at 50 mg/kg/daily for 4 days, then 14 days after recovery, the flora and metabolites began to recover, but still not exactly the same as the normal group [Citation25]. Imipenem has an extremely wide range of activities against aerobic and anaerobic gram-positive bacteria as well as gram-negative bacteria. Therefore, in our study, imipenem was selected to rapidly and completely remove microorganisms from the gastrointestinal tract.
Clinically, Chinese yam is used to treat spleen deficiency and diarrhea. It can adjust gastrointestinal dysfunction, and restores the tension balance of gastrointestinal smooth muscle movement, thereby improving the symptoms of spleen deficiency to achieve spleen and stomach [Citation26]. In this study, we found that Chinese yam can increase the body weight of rats, enhance the gastrointestinal motility of rats, and improve the gastrointestinal function of rats. Research shows: Saponin of Dioscorea may reduce the reabsorption of uric acid by inhibiting the high expression of rat renal URAT1, has an obvious effect of kidney protection [Citation27]. In this research, we also found that Chinese yam can promote the discharge of urine.
The results of Venn diagram show: the diversity of the microbiome in the A group was decreased(1498), about 860 OTUs shared with the normal group, and species similarity is 57.41%; The diversity of the microbiome in the ADR group(2790) tends to return to normal, and about 1193 OTUs shared with the normal group, accounting for 71.43% of the total OTUs. Principal coordinate analysis (PCoA) was used to compare the colonial differences. The main coordinate analysis (PCoA) showed that the A group and the NC group were clustered into two categories, indicating that the intestinal flora disorder model was successfully established. After administration Chinese yam, the ADR group was obviously called back to the NC group. By comparing the histogram of species classification, we found that there were significant differences in the flora between A group and NC group at the generic level. Compared with the NC group, the level of Lachnospiraceae, Ruminococcaceae, Clostridiales, Firmicutes are significantly reduced and the level of Blautia, Prevotella, Eisenbergiella are significantly increased in group A. After administration of Chinese yam, the proportion of each species was significantly restored. Bray-Curtis distance clustering was performed using the abundance of the top 20 species. The result showed that the NC and ADR groups were grouped together and deviated from the A group, and we can conclude that the microbial species composition in the feces of the ADR group also tends to be normal.
UPLC-Q-TOF/MS was used to detect the metabolic characteristics of fecal samples from rats with antibiotic-induced intestinal dysbiosis. The metabolic profile of the ADR group was significantly different from that of the A group, but similar to the NC group, indicating that the bias induced by antibiotics was significantly improved after ADR intervention. The sample points of the NC and A groups were obviously separated in the scoring chart, which indicates that the antibiotics have an obvious effect on the metabolism of the NC group rats, and the model was successfully established. Using an online database for screening, 23 potential biomarkers involved in intestinal dysibiosis were screened. It can be seen from the PCA score chart: The level of M11 is significantly reduced, and the level of M1, M2, M3, M4, M5, M6, M7, M8, M9, M12, M13, M14, M17, M18, M20 are significantly increased in group A. After administration of Chinese yam, metabolites level returns to normal.
Given the above analysis we conclude that: M6 was positively correlated with the genus Erysipelotrichaceae_incertae and Akkermansia; It was negatively correlated with Intestinibacter, Porphyromonadaceae and Clostridiales; M6 is involved in the body’s alanine, aspartate and glutamate metabolism. 3-Methyldioxyindole (M2) is positively correlated with the genus Erysipelotrichaceae_incertae_sedis and Christensenella, and negatively correlated with the Porphyromonadaceae, participating in the body’s tryptophan metabolism. (S) -2-Acetolactate (M12) is positively correlated with Phocea and Akkermansia, negatively correlated with Erysipelotrichaceae and Clostridia and participates in the body’s valine, leucine and isoleucine biosynthesis. Succinic acid (M17) is negatively correlated with Porphyromonadaceae and Barnesiella, and participates in alanine, aspartate and glutamate metabolism, tyrosine metabolism, phenylalanine metabolism. l-Leucine (M14) is positively correlated with Bittarella and Akkermansia. l-Valine (M15) is negatively correlated with Bittarella and participates in valine, leucine and isoleucine degradation. In conclusion, the administration of antibiotics can have a significant interference with the microbiome, and the metabolites and metabolic pathways of different microorganisms demonstrate that the administration of antibiotics significantly alters the microbiome’s metabolic phenotype.
Valine, leucine and isoleucine biosynthesis. The collective term of branched chain amino acids (BCAA) for leucine, valine and isoleucine. BCAA (isoleucine, leucine and valine) regulate many key signaling pathways, the most classic of which is the activation of the m TOR signaling pathway [Citation28]. These BCAA metabolites are catabolized by a series of enzyme reactions to final-products (acetyl-CoA, succinyl-CoA), which enter the tricarboxylic acid cycle (TCA) cycle. BCAA can be utilized by bacteria in the lumen of the gut [Citation29]. Participate in bacterial metabolism indicating they might participate in the regulation of intestinal microbial species and diversity. Phenylalanine is one of the essential amino acids in the body. Under normal circumstances, phenylalanine can be used as an amino acid to synthesize or affect various proteins in body tissues, and more importantly, it can be metabolized into tyrosine in body tissues, and then specific hormones and neurotransmitters (dopamine, adrenaline, norepinephrine, etc.) can be synthesized in brain tissue. In addition to being used to synthesize proteins, the tryptophan ingested by the human body is mainly metabolized in liver, kidney, brain and other tissues and organs to produce a variety of biologically active molecules with physiological functions, such as kynurenine, serotonin (5-HT), quinolinic acid, and so on. 5-HT is an important messenger and neurotransmitter regulator in the body, which inhibits the reabsorption of water by the small intestine and causes diarrhea. As an excitatory amino acid, aspartic acid can promote bacterial chemotaxis [Citation30], which may be a coexisting transmitter that acts as a neuromodulator [Citation31]. It can also participate in the process of cell metabolism, and participate in the TCA as a metabolic substrate of nerve cells [Citation32,Citation33]. Glutamate is currently recognized as a neurotransmitter in the central nervous system excitatory pathway in almost all mammals. Three metabolic pathways were identified by statistical correlation analysis and MetPA analysis: valine, leucine and isoleucine biosynthesis; phenylalanine, tyrosine and tryptophan biosynthesis; and Alanine, aspartate and glutamate metabolism. These three metabolic pathways are the key metabolic pathways for antibiotic-induced intestinal dysbiosis.
Conclusions
In this study, 16S rDNA gene sequencing and fecal metabolomics were used to study the protective effect of Chinese yam on antibiotic-induced intestinal dysbiosis in rats. Statistical analysis showed that after oral administration of antibiotics in normal rats, the microbiota was imbalance and fecal metabolites altered, which was clearly different from the NC group, while the microbiota diversity in the ADR group was improved and the metabolic spectrum was also close to the NC group. In addition, correlation analysis and MetPA analysis of different metabolites and differential intestinal flora revealed that (1) valine, leucine and isoleucine biosynthesis, (2) phenylalanine, tyrosine and tryptophan biosynthesis, as well as (3) alanine, aspartate and glutamate metabolism are three key metabolic pathways related to antibiotic-induced microbiota disorders.
Through the above studies, it can be concluded that the TCM herb Shanyao can be used as a probiotic to regulate the structure and metabolic phenotype of the intestinal microbiota in the host, and further serve as a new source of leading drugs for the treatment of intestinal microbial-related diseases.
Supplemental Material
Download ()Supplemental Material
Download ()Supplemental Material
Download ()Annex_II.xlsx
Download ()Disclosure statement
No potential conflict of interest was reported by the authors.
Additional information
Funding
References
- Tamma PD, Miller MA, Cosgrove SE. Rethinking how antibiotics are prescribed: incorporating the 4 moments of antibiotic decision making into clinical practice. JAMA. 2019;321:139–140.
- Bajaj JS, Kakiyama G, Savidge T, et al. Antibiotic-associated disruption of microbiota composition and function in cirrhosis is restored by fecal transplant. Hepatology. 2018;68:1549–1558.
- Block JP, Bailey LC, Gillman MW, et al. Early antibiotic exposure and weight outcomes in young children. Pediatrics. 2018;142:e20180290.
- Boutin RCT, Dwyer Z, Farmer K, et al. Perinatal antibiotic exposure alters composition of murine gut microbiota and may influence later responses to peanut antigen. Allergy Asthma Clin Immunol. 2018;14:42.
- Haak BW, Lankelma JM, Hugenholtz F, et al. Long-term impact of oral vancomycin, ciprofloxacin and metronidazole on the gut microbiota in healthy humans. J Antimicrob Chemother. 2019;74:782–786.
- Palleja A, Mikkelsen KH, Forslund SK, et al. Recovery of gut microbiota of healthy adults following antibiotic exposure. Nat Microbiol. 2018;3:1255–1265.
- Raymond F, Ouameur AA, Déraspe M, et al. The initial state of the human gut microbiome determines its reshaping by antibiotics. ISME J. 2016;10:707–720.
- Mahana D, Trent CM, Kurtz ZD, et al. Antibiotic perturbation of the murine gut microbiome enhances the adiposity, insulin resistance, and liver disease associated with high-fat diet. Genome Med. 2016;8:48.
- Lee SC, Tsai CC, Chen JC, et al. Effects of “Chinese yam” on hepato-nephrotoxicity of acetaminophen in rats. Acta Pharmacol Sin. 2002;23:503–508.
- Ma JK, Meng X, Kang AN, et al. Effects of rhizome extract of Dioscorea batatas and its active compound, allantoin, on the regulation of myoblast differentiation and mitochondrial biogenesis in C2C12 myotubes. Molecules. 2018;23:2023.
- Liu Y, Li H, Fan Y, et al. Antioxidant and antitumor activities of the extracts from Chinese Yam (Dioscorea opposite Thunb.) flesh and peel and the effective compounds. J Food Sci. 2016;81:H1553–H1564.
- Qiao Y, Xu L, Tao X, et al. Protective effects of dioscin against fructose-induced renal damage via adjusting Sirt3-mediated oxidative stress, fibrosis, lipid metabolism and inflammation. Toxicol Lett. 2018;284:37–45.
- Zhang MH, Fang ZY, Li AN, et al. Yam polysaccharide improves sperm viability and protects sperm DNA integrity in vitro. Nat J Androl. 2017;13:1020–1024.
- Cai Y, Liu W, Lin Y, et al. Compound polysaccharides ameliorate experimental colitis by modulating gut microbiota composition and function. J Gastroenterol Hepatol. 2018. DOI:10.1111/jgh.14583
- Zeng M, Zhang L, Li M, et al. Estrogenic effects of the extracts from the Chinese yam (Dioscorea opposite Thunb.) and its effective compounds in vitro and in vivo. Molecules. 2018;23:11.
- Zeng M, Zhang L, Zhang B, Li B, et al. Chinese yam extract and adenosine attenuated LPS-induced cardiac dysfunction by inhibiting RAS and apoptosis via the ER-mediated activation of SHC/Ras/Raf1 pathway. Phytomedicine. 2019;61:152857.
- Zheng XJ, Xie GX, Zhao AH, et al. The footprints of gut microbial-mammalian co-metabolism. J Proteome Res. 2011;10:5512–5522.
- Yu M, Jia HM, Zhou C, et al. Urinary and fecal metabonomics study of the protective effect of Chaihu-Shu-Gan-San on antibiotic-induced gut microbiota dysbiosis in rats. Sci Rep. 2017;7:46551.
- Fadrosh DW, Ma B, Gajer P, et al. An improved dual-indexing approach for multiplexed 16S rRNA gene sequencing on the Illumina MiSeq platform. Microbiome. 2014;2:6.
- Li S, Li J, Mao G, Wu T, et al. Fucosylated chondroitin sulfate from Isostichopus badionotus alleviates metabolic syndromes and gut microbiota dysbiosis induced by high-fat and high-fructose diet. Int J Biol Macromol. 2019;124:377–388.
- Yang J, Zhang S, Wu J, et al. Imipenem and normal saline with cyclophosphamide have positive effects on the intestinal barrier in rats with sepsis. Biomed Pap Med Fac Univ Palacky Olomouc Czech Repub. 2018;162:90–98.
- Rababa HA, Hijjawi TB, Alzoubi KH, et al. The nephroprotective effect of N-acetyl-l-cysteine and atorvastatin against imipenem induced nephrotoxicity. Curr Mol Pharmacol. 2018;10:155–161.
- Tahri A, Lahyani A, Kallel R, et al. Exposure to imipenem/cilastatin causes nephrotoxicity and even urolithiasis in Wistar rats. Toxicology. 2018;404–405:59–67.
- Tahri A, Ksouda K, Kallel R, et al. A carbapenem antibiotic imipenem/cilastatin induces an oxidative stress-status and gonadotoxic effects in « wistar » rats. Biomed Pharmacother. 2017;95:308–316.
- Harrison MP, Jones DV, Pickford RJ, et al. β-hydroxybutyrate: a urinary marker of imipenem induced nephrotoxicity in the cynomolgus monkey detected by high field 1 H NMR spectroscopy. Biochem Pharmacol. 1991;41:2045–2049.
- Li SYC, Miao LJ, Liang YJ, et al. Study on the effect of yam and spleen and stomach. Chin Med Pharmacol Clin. 1994;1:19–21.
- Chen GL, Zhu LR, Sha NA, et al. Effect of total saponin of Dioscorea on chronic hyperuricemia and expression of URAT1 in rats. Zhongguo Zhong Yao Za Zhi. 2013;38:2348–2353.
- Shihai Z, Xiangfang Z, Xiangbing S, et al. Novel metabolic and physiological functions of branched chain amino acids: a review. J Anim Sci Biotechnol. 2017;8:10.
- Dai ZL, Zhang J, Wu G, et al. Utilization of amino acids by bacteria from the pig small intestine. Amino Acids. 2010;39:1201–1215.
- Falke JJ, Bass RB, Butler SL, et al. The two-component signaling pathway of bacterial chemotaxis: a molecular view of signal transduction by receptors, kinases, and adaptation enzymes. Annu Rev Cell Dev Biol. 1997;13:457.
- Bradford SE, Nadler JV. Aspartate release from rat hippocampal synaptosomes. Neuroscience. 2004;128:751–765.
- Bakken IJ, Aasly J, Unsg RG, et al. [U-13C] aspartate metabolism in cultured cortical astrocytes and cerebellar granule neurons studied by NMR spectroscopy. Glia. 2015;23:271–277.
- Bakken IJ, White LR, Aasly J, et al. Lactate formation from [U-13C] aspartate in cultured astrocytes: compartmentation of pyruvate metabolism. Neurosci Lett. 1997;237:117–120.