Abstract
Background
miRNAs have been involved in neural development, degeneration, and regeneration. MiR-463-3p is expressed in reproductive and nervous systems. In this study, the role of miR-463-3p in tibial nerve injury and regeneration was explored.
Materials and methods
A model of tibial nerve injury was established with the crush method, and the levels of miR-463-3p were detected at days 0, 3, 7, 12, 18 and 24 post-injury. Then, primary tibial nerve cells were isolated from newborn mice, and miR-463-3p was respectively overexpressed and knocked down in cultured cells. Behaviors of tibial nerve cells were detected. Furthermore, bioinformatics technology was used to investigate the underlying mechanism.
Results
The expression miR-463-3p was robustly increased in the injured tibial nerve in vivo and in tibial nerve cells treated with oxygen-glucose deprivation. The data on gain- and loss-of-function demonstrated that miR-463-3p negatively regulated including neurite length, percentage of cells with neurites, and cell branching in tibial nerve cells. Small proline-rich repeat protein 1 A (SPRR1A), an identified nerve regeneration associated genes, was identified as a target gene of miR-463-3p.
Conclusion
Inhibition of miR-463-3p could increase SPRR1A expression in the tibial nerve tissue and improve regeneration of the tibial nerve post-injury in vivo.
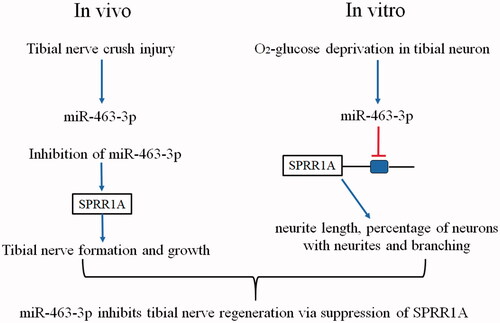
Introduction
Nerve injury may lead to a severe disability, which greatly influences the individual’s activity and quality of life of patients. Unlike the central nervous system, peripheral nerves have the capacity to regenerate and which depends on the nature and severity of the lesions [Citation1,Citation2]. After peripheral nerve injury, the degenerative debris and axon discontinuity at the injury site may block the regeneration of axons and result in the death of axotomized neurons [Citation3]. Several studies have shown that the development of microsurgery instrumentation and the new suture techniques have provided a complete morphological regeneration in peripheral nerves repair patients [Citation4], but there is still have no any medical treatment could overcome the limitations in axonal regeneration functions after nerve injury [Citation5]. Thus, a new therapeutic strategy for peripheral nerve regeneration is urgent.
MicroRNAs (MiRNAs) are endogenously encoded small RNAs, which are widely regarded as a novel class of important molecular switches playing a major role in posttranscriptional gene regulation by acting on the 3’UTRs of target genes [Citation6]. Many studies have indicated a correlation between neurological diseases and the alteration of miRNAs biogenesis [Citation7]. Recently, the therapeutic potential of miRNAs has also been explored in disorders of neural development, degeneration, and regeneration [Citation8,Citation9]. Such as miR-338 and miR-21 co-transfection has been reported could treatment of rat sciatic nerve injury [Citation10]. MiR-145 inhibited neurite growth of dorsal root ganglia (DRG) neuron through Slit-Robo-srGAP signaling pathway [Citation11]. In rats with sciatic nerve crush injury, dysregulation of the miR-340 expression in the injury location affected the cell debris removal and axonal regrowth [Citation12]. From this, miRNAs are closely involved in nerve regeneration. Although the importance of miRNAs in neural development and neurodegeneration has been recognized [Citation13], however, the role of many miRNAs in nerve injury and repair is completely unrevealed. Here, we investigated the effect of miR-463-3p, a poorly characterized miRNA, on the functional regeneration in tibial nerve injury mice in vivo and in vitro. This experimental evidence demonstrated that miR-463-3p suppressed the tibial nerve regeneration through targeted and negatively regulated expression of Small proline-rich repeat protein 1 A (SPRR1A), which is an identified nerve regeneration associated genes.
Materials and methods
Animals and surgery
A total of ninety-six male C57BL/6 mice were used in this experiment and purchased from Fisher Scientific (Fair Lawn, NJ, USA). Animals were maintained at a constant temperature (2 2∼24 °C) and 60% humidity with access to food and water libitum. All procedures were carried out in accordance with the Declaration of The National Institutes of Health Guide for Care and were approved by the Ethics Committee of Huaihe Hospital of Henan University (Kaifeng, China). Mice were randomly assigned to three groups, after anesthesia, the model group animals (n = 24) received a standardized crush injury to the right tibial nerve [Citation14]. Briefly, the tibial nerve was exposed and isolated from the ipsilateral sciatic, peroneal, and sural nerves without any epineural tunneling using aseptic technique. Next, the sham group mice (n = 24) were repaired immediately with micro sutures, but the model group animals received a crush injury with a pair of No. 5 jeweler’s forceps for exactly in the tibial nerve before repairing. Meanwhile, the control group (n = 24) without any procedure. After that, mice were anesthetized again for the harvest of tibial nerve at post-injury 0, 3, 7, 12, 18 or 24 days after crush injury using medetomidine HCl (0.2 mg/kg) and ketamine (30 mg/kg). A 15-mm section of tibial nerve in injury area was collected for further study.
Isolation of tibial nerve cells from newborn mice
The tibial nerve was dissected out from newborn mice, removing the epithelial covering, stored in serum-free neurobasal medium (NBM) and then minced using forceps and a razor blade. Minced tibial nerve segments were dissociated in serum-free NBM and 0.25% trypsin-EDTA. Dissociation suspensions were strained through a 100 μm cell strainer (Fisher Scientific, Pittsburgh, PA, USA), spun at 1500 r/min for 10 min, and re-suspended in NBM.
Cell transfection
Tibial nerve cells were seeded in 24-well plates at the concentration of 105/ml. The logarithmic growth phase cells were collected and transfected respectively with the 25 nM miR-463-3p mimics, the mimics-negative control (mimic-NC), the antagonist of miR-463-3p used to inhibit endogenous miR-463-3p expression (anta-463-3p) and the antagomir-negative control (anta-NC) by using LipofectamineTM3000 (Invitrogen Life Technologies, CA) according to the manufacturer’ s instructions after 80–90% cell confluence. All the transfection reagents were purchased from Genepharma Co., Ltd (Shanghai, China). The effect of transfection was determined by RT-PCR.
The neurite outgrowth assay
Assays for neurite outgrowth was determined according to the previously described methods with slight modifications [Citation15]. Briefly, post-transfection, the primary tibial nerve cells were cultured in fresh medium in chamber slides coated with laminin and polylysine (20 μg/mL each) for 2 h overnight at 37 °C in a humidified atmosphere. The chemically defined media was added in wells after rinsing. Cells were then fixed with 4% paraformaldehyde and immunolabeled with antibodies against neuron-specific β-tubulin III. After that, the primary tibial nerve cells were cultured, the percentage of cells with neurites, length of neurites per cells and the number of branches per cells were determined by using NIH Image J software as described [Citation16].
Luciferase reporter assay
HEK-293T cell line was purchased from the American Type Culture Collection. The pGL3 control vector (Promega) carrying the SPRR1A 3’-UTR was amplified with specific primers and was then cloned into a pMiR-Report vector system (Ambion Inc., Austin, Texas, USA). A mutated 3′-UTR of SPRR1A was introduced into the potential miR-463-3p binding site by using a two-step PCR approach. HEK-293T cells were co-transfected with the reporter vectors containing the wild type or mutant of SPRR1A 3′-UTR and miR-463-3p mimic or anta-463-3p. After 48 h, luciferase activity was measured using a dual-luciferase reporter assay system (Promega, WI, USA).
Quantitative RT-PCR analysis
Total RNA was extracted using TRIzol reagent (Invitrogen) and miRNeasy kits (Qiagen, Hilden, Germany) according to the manufacturer’s protocol. The miRNA was converted to cDNA by using a PrimeScript RT reagent kit (TaKaRa, Tokyo, Japan) according to the manufacturer’s protocol. MiR-463-3p and SPRR1A were investigated using a miRVana real-time PCR miRNA Detection Kit and real-time PCR Primer Sets. The primer pairs were designed and synthesized by Ribobio Inc. (Guangzhou, China). The reaction was performed under the following conditions: one cycle of 95 °C for 10 min, then 40 cycles of 95 °C for 15 s, 60 °C for 60 s and 72 °C for 45 s.
Histomorphology
To explore the role of anta-463-3p in tibial nerve injury, mice received subcutaneous injections of anta-463-3p or anta-NC in the injury locations after crush injury to the right tibial nerve. Morphological analysis of axon profiles was detected to reflect the crush injury of nerve fibers. Briefly, the tibial nerve tissues of mice were isolated at 3, 7, and 12 days after anta-463-3p or anta-NC subcutaneous injection. The tibial nerve was cut into 3 mm of sections and fixed with 4% paraformaldehyde and 2% glutaraldehyde in 0.1 M PBS at 4 °C overnight. Next, nerves were stained with osmium tetroxide and toluidine blue according to the previously described [Citation16]. Number of myelinated fiber was acquired at the corresponding time point. In addition, the total myelin sheath area was also analyzed by using the NIH Image J software.
Statistical analysis
All statistical values were represented as the mean ± SD, and were analyzed with one-way repeated measures analysis of variance (ANOVA) and followed by Student’s t-test. All statistical parameters were calculated using SPSS 19.0 software. Differences with p < .05 were considered statistically significant.
Results
MiR-463-3p was up-regulated after early tibial nerve injury
To determine the potential roles of miR-463-3p in tibial nerve injury, mice received a standardized crush injury to the right tibial nerve to establish the tibial nerve injury model animals. After anesthesia, the tibial nerve tissues in mice were harvest and the level of miR-463-3p was measured by using an RT-PCR assay. As shown in , the levels of miR-463-3p model group were significantly increased at post-injury 0 and 3 days, and which then decreased at post-injury 7, 12, 18 or 24 days gradually. These results suggest that miR-463-3p was up-regulated after early tibial nerve injury. To further investigate these results, the tibial nerve cells were isolated and primary cultured. After ODG for cells, the levels of miR-144-3p were detected again. Similar with the results of RT-PCR analysis in tibial nerve tissues in an animal model, compared to the control group, a distinctly decrease miR-463-3p expression and followed by increase miR-463-3p expression was observed in OGD group (. These results demonstrate that miR-463-3p was up-regulated after early tibial nerve injury.
Figure 1. MiR-463-3p was first increased and then decreased after tibial nerve injury. The tibial nerve tissues were isolated and acquired in mice from lesions at post-injury 0, 3, 7, 12, 18 or 24 days, respectively. The tibial nerve cells from mice were primary cultured and received the OGD treatment. (A) The expression of miR-463-3p was analyzed by qRT-PCR in tibial nerve tissues. (B) The level of miR-463-3p was detected in tibial nerve cells at 0, 0.5, 1.0, 2.0 h under OGD. Data are shown as mean ± SD (N = 4). *p < .05 and #p < .05 vs. day 7 or 0.5 h.
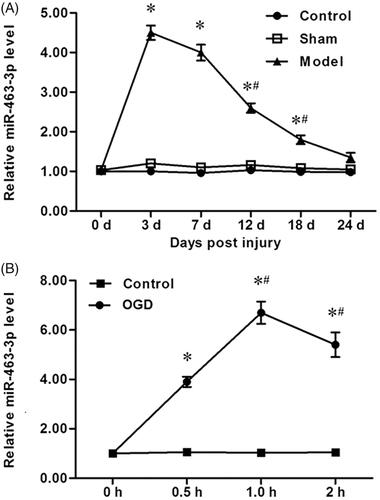
MiR-463-3p mimic inhibited neurite elongation and branching in vitro
Based on these results, we then explored the effect of miR-463-3p on neurite elongation and branching in vitro. The tibial nerve cells were collected and transfected with miR-463-3p mimics or mimic-NC. In miR-463-3p mimics group, we found that miR-463-3p expression was higher than the control and mimic-NC group (. Subsequently, the percentage of cells with neurites, length of neurites per cell and the number of branches in cells were determined in tibial nerve cells after transfection. We surprisingly found that miR-463-3p mimic not only decreased the percentage of cells with neurites () but also inhibited the length of neurites per cell () and the number of branches in cells (. These results demonstrated that miR-463-3p mimic inhibited neurite elongation and branching in vitro successfully.
Figure 2. MiR-463-3p mimic inhibits neurite elongation and branching in vitro. The tibial nerve cells were transfected with miR-463-3p mimic or mimic-NC. (A) The level of miR-463-3p among groups was measured with qRT-PCR. (B) The length of neurites per cell was increased after miR-463-3p mimic transfection. (C) At 48 h, the percentage of cells with neurites was decreased in miR-463-3p mimic group relative to control group. (D) The number of branches per cell was increased in miR-463-3p mimic group. N = 4, *p<.05.
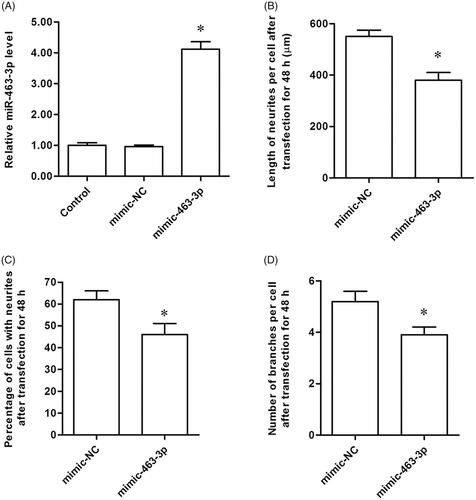
MiR-463-3p antagonist enhanced neurite elongation and branching in vitro
To further confirm the effect of miR-463-3p on neurite elongation and branching, anta-miR-463-3p or anta-NC was transfected into tibial nerve cells to knockdown of endogenous miR-463-3p. In anta-miR-463-3p group, miR-463-3p was down-regulated significantly (. We then detected that the percentage of cells with neurites, length of neurites per cell and the number of branches in cells among groups as the above methods. As we expected, anta-miR-463-3p not only significantly increased the percentage of cells with neurites (), but also induced the length of neurites in cells () and the number of branches in cells (. These data indicated that knockdown of endogenous miR-463-3p enhanced neurite elongation and branching in vitro.
Figure 3. MiR-463-3p antagonist enhances neurite elongation and branching in vitro. The tibial nerve cells were transfected with miR-463-3p antagonist or anta-NC, respectively. (A) The level of miR-463-3p among groups. (B) The length of neurites per cell after transfection. (C) The percentage of cells with neurites among groups. (D) The number of branches per cell among groups. N = 4, *p<.05.
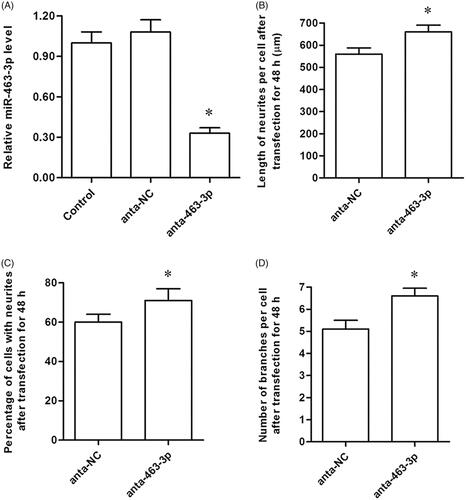
MiR-463-3p directly bound to SPRR1A and suppressed its expression
SPRR1A, which is closely associated with nerve regeneration, was found to be a potential target of miR-463-3p (. Subsequently, wild type (WT) and mutant (MUT) SPRR1A were constructed and cloned into downstream of a luciferase reporter gene (. To confirm whether miR-463-3p targeted SPRR1A 3′-UTR, a luciferase activity assay was carried out. As shown in , our results found that the relative luciferase activity was significantly decreased in wild type SPRR1A 3′-UTR group after transfected with miR-463-3p mimic, but the luciferase intensity had no difference from that in the SPRR1A 3′UTR-mut groups.
Figure 4. MiR-463-3p is direct target of SPRR1A. (A) Prediction result of potential binding sites between miR-463-3p and 3′UTR of SPRR1A by using the TargetScan. (B) Sequence of the wild-type and the mutant form 3′UTR of SPRR1A mRNA in mice contains a putative microRNA-463-3p-binding sites. (C) Relative luciferase activity in HEK-293T cells after transfection with miR-463-3p mimic and SPRR1A-3′UTR-WT or SPRR1A-3′UTR-MUT. Data are shown as mean ± SD (N = 4). *p<.05.
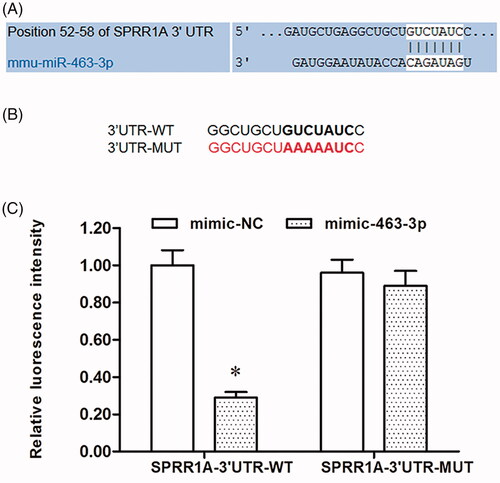
MiR-463-3p antagonist caused tibial nerve regeneration via promotion of Sprr1A in vivo
To explore the role of miR-463-3p antagonist in tibial nerve regeneration in mice, we then established the tibial nerve injury model and performed the miR-463-3p antagonist subcutaneous injection. Afterward, the tibial nerve lesions tissues were prepared for further study. The levels of SPRR1A and miR-463-3p among groups were measured again. Our results showed that SPRR1A was up-regulated in anta-miR-463-3p group compared with the anta-NC group (. Correspondingly, the miR-463-3p was down-regulated in anta-miR-463-3p group (. These results indicated that SPRR1A was indeed regulated by miR-463-3p in vivo. Besides, histomorphological analysis of cross-sections of crushed nerves in mice was made to assess the myelinated fiber number and the area of myelination. Our data indicated that the total number of myelinated fibers () and the area of myelination () were higher in anta-miR-463-3p group than in anta-NC group. These results demonstrate that miR-463-3p suppresses the tibial nerve regeneration by regulating SPRR1A negatively in vivo.
Figure 5. MiR-463-3p antagonist causes tibial nerve regeneration and promotes SPRR1A expression in vivo. The tibial nerve injury model of mice was established, miR-463-3p antagonist or anta-NC was subcutaneously injected into mice. The tibial nerve lesions tissues were isolated from mice at post-injury 3, 7 or 12 days, respectively. (A) The SPRR1A expression among groups were measured by using qRT-PCR. (B) The level of miR-463-3p was detected by qRT-PCR. Mice tibial nerve tissues were stained with toluidine blue to tibial nerve regeneration. (C) The total number of myelinated fibers was increased in anta-miR-463-3p group. (D) The area of myelination was increased after anta-miR-463-3p transfection. Data are shown as mean ± SD (N = 4). *p<.05.
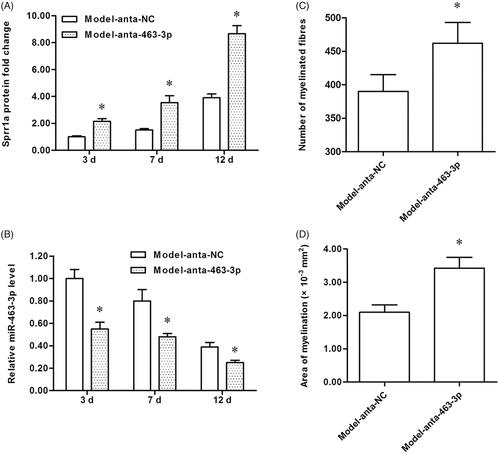
Discussion
MiR-463-3p was discovered by computational analyses as a differentially expressed miRNA during prepubertal testis differentiation in 2005 [Citation17]. From then on, a couple of studies suggested that miR-463-3p was associated with the development of reproductive organs and imbalance of glucose metabolism [Citation18,Citation19]. Recently, it was shown that miR-463-3p was enriched in neuronal hinting a possible regulatory role in development and function maintenance of the nervous system [Citation20]. In this study, we found that expression miR-463-3p was robustly increased in the injured tibial nerve in vivo and in tibial nerve cells treated with oxygen-glucose deprivation in vitro. Then, our data on gain- and loss-of-function demonstrated that miR-463-3p negatively regulated behaviors of tibial nerve cells, including neurite length, percentage of cells with neurites, and cell branching. Moreover, we confirmed that inhibition of miR-463-3p in vivo could improve SPRR1A-mediated regeneration of the tibial nerve post-injury.
Small proline-rich repeat protein 1 A (SPRR1A), also known as cornifin A, belongs to the multigene SPRR family of keratinocyte differentiation markers [Citation21]. The discovery of SPRR1A has provided important advances in nerve regeneration as regeneration-associated genes [Citation22]. SPRR1A expression in neuronal was firstly verified by microarray analysis during sciatic nerve regeneration in the mouse [Citation22]. Previous studies showed that SPRR1A protects nigrostriatal axons from degeneration in the 6-Hydroxydopamine lesion rat model of Parkinson's disease [Citation23]. Some studies also suggest that SPRR1A is expressed during development and constitutively by some cells in the adult. Recently, miR-155 deletion in mice overcomes neuron-intrinsic and neuron-extrinsic barriers to spinal cord repair via targeting SPRR1A [Citation24]. Thus, SPRR1A was identified as a promoter of nerve regeneration. At the present work, we found that SPRR1A is one of the potential targets of miR-463-3p predicted by TargetScan. Our results from luciferase activity assay and cell transfection confirmed that miR-463-3p directly targeted SPRR1A and suppressed its expression. Furthermore, we observed that upregulation of SPRR1A protein by inhibition of miR-463-3p significantly improved tibial nerve function and facilitated the regeneration of the tibial nerve post-injury in vivo.
Conclusion
In conclusion, these findings demonstrated that miR-463-3p was robustly increased in the injured tibial nerve in vivo and in tibial nerve cells treated with oxygen-glucose deprivation in vitro. MiR-463-3p inhibits tibial nerve regeneration via post-transcriptional suppression of SPRR1A.
Disclosure statement
No potential conflict of interest was reported by the authors.
References
- Daly W, Yao L, Zeugolis D, et al. A biomaterials approach to peripheral nerve regeneration: bridging the peripheral nerve gap and enhancing functional recovery. J R Soc Interface. 2012;9:202–221.
- Li S, Wang X, Gu Y, et al. Let-7 microRNAs regenerate peripheral nerve regeneration by targeting nerve growth factor. Mol Ther. 2015;23:423–433.
- Schüning J, Scherens A, Haussleiter IS, et al. Sensory changes and loss of intraepidermal nerve fibers in painful unilateral nerve injury. Clin J Pain. 2009;25:683–690.
- Taha MO, Rosseto M, Fraga MM, et al. Effect of retinoic acid on tibial nerve regeneration after anastomosis in rats: histological and functional analyses. Transplant Proc. 2004;36:404–408.
- Navarro X, Vivó M, Valero-Cabré A. Neural plasticity after peripheral nerve injury and regeneration. Prog Neurobiol. 2007;82:163–201.
- Bushati N, Cohen SM. MicroRNA functions – annual review of cell and developmental biology. Annu Rev Cell Dev Biol. 2007;23:175.
- Di W, Murashov AK. Molecular mechanisms of peripheral nerve regeneration: emerging roles of microRNAs. Front Physiol. 2013;4:55.
- Fineberg SK, Kosik KS, Davidson BL. MicroRNAs potentiate neural development. Neuron. 2009;64:303–309.
- Eacker SM, Dawson TM, Dawson VL. Understanding microRNAs in neurodegeneration. Nat Rev Neurosci. 2009;10:837–841.
- Wang J, Muheremu A, Zhang M, et al. MicroRNA-338 and microRNA-21 co-transfection for the treatment of rat sciatic nerve injury. Neurol Sci. 2016;37:883–890.
- Zhang HY, Zheng SJ, Zhao JH, et al. MicroRNAs 144, 145, and 214 are down-regulated in primary neurons responding to sciatic nerve transection. Brain Res. 2011;1383:62.
- Li S, Zhang R, Yuan Y, et al. MiR-340 regulates fibrinolysis and axon regrowth following sciatic nerve injury. Mol Neurobiol. 2016;54:1–11.
- Yu B, Zhou S, Wang Y, et al. Profile of microRNAs following rat sciatic nerve injury by deep sequencing: implication for mechanisms of nerve regeneration. PLOS One. 2011;6:e24612.
- George LT, Myckatyn TM, Jensen JN, et al. Functional recovery and histomorphometric assessment following tibial nerve injury in the mouse. J Reconstr Microsurg. 2003;19:041–048.
- Huang DW, Mckerracher L, Braun PE, et al. A therapeutic vaccine approach to stimulate axon regeneration in the adult mammalian spinal cord. Neuron. 1999; 24:639–647.
- Jing X, Wang T, Huang S, et al. The transcription factor Sox11 promotes nerve regeneration through activation of the regeneration-associated gene Sprr1a. Exp Neurol. 2012;233:221–232.
- Yu Z, Raabe T, Hecht NB. MicroRNA Mirn122a reduces expression of the posttranscriptionally regulated germ cell transition protein 2 (Tnp2) messenger RNA (mRNA) by mRNA cleavage. Biol Reprod. 2005;73:427–433.
- Hou X, Wu W, Yin B, et al. MicroRNA-463-3p/ABCG4: a new axis in glucose-stimulated insulin secretion. Obesity (Silver Spring). 2016;24:2368.
- Ahn HW, Morin RD, Zhao H, et al. MicroRNA transcriptome in the newborn mouse ovaries determined by massive parallel sequencing. Mol Hum Reprod. 2010;16:463.
- Landgraf P, Rusu M, Sheridan R, et al. A Mammalian microRNA expression atlas based on small RNA library sequencing. Cell. 2007;129:1401–1414.
- Gibbs S, Fijneman R, Wiegant J, et al. Molecular characterization and evolution of the SPRR family of keratinocyte differentiation markers encoding small proline-rich proteins. Genomics. 1993;16:630.
- Bonilla IE, Tanabe K, Strittmatter SM. Small proline-rich repeat protein 1A is expressed by axotomized neurons and promotes axonal outgrowth. J Neurosci. 2002;22:1303–1315.
- Kanaan NM, Grabinski T, Combs B, et al. Small proline-rich repeat 1A protein protects nigrostriatal axons from degeneration in the 6-hydroxydopamine lesion rat model of Parkinson's disease. Cell Transpl. 2015;24:761.
- Gaudet AD, Mandrekar-Colucci S, Hall JC, et al. miR-155 Deletion in mice overcomes neuron-intrinsic and neuron-extrinsic barriers to spinal cord repair. J Neurosci. 2016;36:8516.