Abstract
Cervical cancer (CC) is a primary gynecological malignancy worldwide. Cancer stem cells (CSCs) possess enhanced tumor-initiating and self-renewing abilities. MicroRNAs (miRNAs) play essential roles in CSCs’ tumorigenesis. This study investigated the effects of miR-146a on CSCs’ tumorigenesis and invasion. Tumorsphere cells (TCs) were enriched from the HeLa cell line. Real-time PCR, Western blots, ALDH assays, colony formation and invasion assays, luciferase reporter assays and the Xenograft mouse model were used to determine the underlying mechanism of CC. The results showed that TCs displayed higher ALDH activity and miR-146a was upregulated in differentiated TCs. Moreover, miR-146a inhibitor increased colony formation and cell invasion in TCs while miR-146a mimics displayed the opposite roles. An inverse relationship between miR-146a and VEGF expression was found in TCs and the luciferase reporter assay revealed that VEGF was a direct target of miR-146a. Inhibition of VEGF reversed the effects of miR-146a inhibitor on TCs. The activated CDC42/PAK1 signaling was associated with TCs’ tumorigenesis and invasion. Furthermore, miR-146a inhibitor-treated TCs promoted tumor growth in nude mice. Altogether, the results suggest that miR-146a modulated TCs’ tumor formation and invasion and was associated with VEGF/CDC42/PAK1 signaling. This study provided insight into developing new therapeutic strategies for CC.
Introduction
Cervical cancer (CC) is the second highest cause of cancer death in females with an estimating 530,000 new cases and 270,000 deaths each year [Citation1]. The majority of CC cases result from carcinogenic human papillomavirus (HPV) infection [Citation2]. In recent years, widespread Papnaikolaou and HPV screening programs have dramatically increased diagnostic accuracy and survival rates in many countries [Citation3], however, the high fatality of CC is still a public health and socioeconomic issue, especially in developing countries [Citation4]. Therefore, there are imminent demands to explore novel and more effective ways to diagnose and treat CC.
Cancer stem cells (CSCs) have been intensively studied in the past decades. They were first discovered in lymphomas and leukemia [Citation5] and then found in various solid tumors, such as the colon [Citation6] and the breast [Citation7]. Having great capability to differentiate into progenies of malignant cells [Citation8], CSCs are also less sensitive to regular therapies, such as radiotherapy and chemotherapy [Citation9]. It is well established that CSCs are the “seed” for tumor cell metastasis and relapse [Citation10]. A high proportion of CSCs is related to poor diagnosis and low survival rates in various cancer types [Citation11]. Thus, studying CSCs’ properties is essential for exploring the mechanisms underlying cancer metastasis. In addition, cervical CSCs (CCSCs) were first isolated using the spheroid method [Citation12], and are characterized as highly tumorigenesis and radioresistance [Citation13], and can self-renew [Citation14].
MicroRNAs (miRNAs), 19–25 nucleotides in length, are small conserved RNA molecules that play an essential role in various biological processes [Citation15]. Meanwhile, growing evidence shows that miRNAs help regulate CCSCs. For example, the upregulation of miR-145 is associated with a good prognosis, reduced tumor invasion, and colony formation in CCSCs [Citation16]. MiR-23b has low expression levels in CCSCs while the elevation of miR-23b alters the sensitivity of CCSCs to cisplatin treatment and the capability of tumorsphere formation [Citation17]. Thus, miRNAs may be an important regulator of CCSCs.
It has been demonstrated that tumors express a high level of antigenic factors to initiate neovascularization [Citation18], in which many cytokines or growth factors collectively modulate tumor growth, metastasis, and invasion [Citation19]. Among these angiogenic factors, vascular endothelial growth factor (VEGF), plays important roles in vascular remodeling, angiogenesis, and vascular permeability [Citation20], and has been well-documented [Citation19]. Furthermore, miRNAs and VEGF have been reported to collectively regulate tumor cell properties, such as metastasis and invasion. MiR-26b inhibits the metastasis of lung cancer cells through the NF-κB/MMP-9/VEGF signaling pathway [Citation21]. In addition, miR-140-5p suppresses breast cancer metastasis and angiogenesis via targeting VEGF.
Accumulating reports reveal that miR-146a plays dual functions in multiple cancer types. For example, low expression levels of miR-146a are regarded as a tumor initiator in hepatocellular carcinoma [Citation22] and breast cancer [Citation23]. On the other hand, upregulated miR-146a acts as an oncogene in colorectal cancer [Citation24] and oral carcinoma [Citation25]. However, very little attention is focused on the effect of miR-146a on CCSCs. Therefore, the aims of this study were to investigate the function of miR-146a in CCSCs’ tumorigenesis and invasion. Therefore, this study would offer a novel insight into understanding the mechanism of CC and the development of new treatments.
Materials and methods
Ethics statement
All experiments for this study were approved by the ethics committee of Xinjiang Medical University. All procedures using nude mice in this study complied with the guidelines of the Animal Ethics Committee for the care and use of Xinjiang Medical University.
HeLa cell culture
HeLa cervix carcinoma cells were obtained from the Cell Bank of Type Culture Collection of Chinese Academy of Sciences (Shanghai, China). HeLa cells were cultured in Dulbecco's Modified Eagle's Medium (DMEM; Gibco, Grand Island, USA) and supplemented with 100 mg/L streptomycin and 1 × 105 U/L penicillin (EMD Millipore, Darmstadt, Germany) at 37 °C in a humidified atmosphere with 5% CO2.
Tumor stem cell and cell differentiation
HeLa cells (1200 cells per well) were placed in 6-well ultra-low attachment plates (Corning Inc., NY, USA) and incubated in serum-free stem cell DMEM (Gibco, Grand Island, USA) containing 10 U/ml leukemia inhibitory factor, 10 ng/ml basic fibroblast growth factor (bFGF), 100 mg/L streptomycin, and 1 × 105 U/L penicillin (EMD Millipore, Darmstadt, Germany) for 7 days. Cell clones (>50) were regarded as tumorspheres. Tumorsphere cells (TCs) were harvested by dissociating tumorspheres in the non-enzymatic cell dissociation solution (Sigma-Aldrich, St. Louis, MO, USA) every 7 days for 2 min. Differentiated tumorsphere cells (DTCs) were obtained by cultivating TCs in stem cell medium without bFGF for 8 days.
Aldehyde dehydrogenase (ALDH) assay
The ALDH activity was analyzed following the manufacturer’s instructions for the ALDEFLUOR kit (StemCell Technologies, Cambridge, MA, USA). In brief, TCs and DTCs were treated with ALDEFLUOR assay buffer containing substrate bodipy-aminoacetaldehyde (1 µmol/L). Cells treated with a specific ALDH inhibitor diethylaminobenzaldehyde (DEAB) were used as a negative control. Flow cytometry (FACS) assay was conducted to evaluate ALDH-positive cells (BD FACSCalibur, Coppell, TX, USA).
Real-time PCR
Total RNAs from cells were extracted using the TRIzol reagent (Aidlab, Beijing, China). After purification, first-strand cDNAs were synthesized using the M-MLV Reverse Transcriptase (RNase H) kit (GeneCopoeia, Rockville, MD, USA). Real-time PCR was carried out on an ABI7900/illumina eco (Applied Biosystems, Beverly, MA, USA). MiR-146a expression was detected using the TaqMan MicroRNA Assay (Life Technologies, NY, USA). The primers were used as followed: miR-146a: 5′- GGCCTTCAGAGTTTGTTCCA-3′ (Forward) and 5′-GGCCTCATCTGGAGAGTCTG-3′ (Reverse), Nanog: 5′-AATGGTGTGACGCAGAAG-3′ (Forward) and 5′-AGATTCCTCTCCACAGTTATAG-3′(Reverse), Oct4: 5′- AGCTGGAGAAGGAGAAGC-3′ (Forward) and 5′-AAAGCGGCAGATGGTCGT-3′ (Reverse), Sox: 5′-CAATAGCATGGCGAGCGG-3′ (Forward) and 5′-GTCGTAGCGGTGCATGGG-3′ (Reverse), and GAPDH: 5′-AAGGCTGAGAATGGGAAAC-3′ (Forward) and 5’-TTCAGGGACTTGTCATACTTC-3′(Reverse). Real-time PCR data were analyzed using the ΔΔCT method [Citation26], and GAPDH mRNA was used as the reference gene.
Western blots
Total protein was isolated by using the cell lysis buffer (Beyotime Institute of Biotechnology, Shanghai, China). Protein extracts were isolated by 10% SDS-PAGE electrophoresis and electrically transferred to polyvinylidene difluoride membranes that were blocked with 5% nonfat dry milk in PBS-0.05% Tween 20 for 1 h. Then, the primary antibodies for VEFG, Nanog, Oct4, Sox, CDC42, PAK1 and GAPDH (Santa Cruz Biotechnology, Santa Cruz, CA, USA) were incubated at 4 °C overnight. The membranes were incubated with their corresponding secondary antibodies for 1 h at 37 °C. The results were visualized by enhanced chemiluminescence assays (Thermo Fisher Scientific, Rockford, IL, USA).
Cell transfection
MiR-146a inhibitor (5′-AACCCAUGGAAUUCAGUUCUCA-3′) and mimics (5′-UGAGAACUGAAUUCCAUGGGUU-3′; 5′-CCCAUGGAAUUCAGUUCUCAUU-3′) (Qiagen, Hilden, Germany) and small interfering RNA for VEGF (siVEGF, 5′-GAUUAUGCGGAUCAAACCUtt-3′) (RiboBio Life Science Co., Guangzhou, China) were transferred to cells in fresh culture medium for 18 h at 37 °C in a humidified atmosphere containing 5% CO2. The detailed protocol was conducted as previously described [Citation27]. The transfection efficacy was evaluated by PCR.
Colony formation assay
The TCs and DTCs were cultured in DMEM containing fetal calf serum (10%), glutamine (2 mmol/L), penicillin (1 × 105 U/L), and streptomycin (100 mg/l). Cells were cultivated (clonal densities of 200/cm2) at 37 °C in 5% CO2 on 2% gelatin-coated tissue culture dishes (BD Biosciences, San Jose, CA, USA) for 28 days. Cell colonies were monitored daily, and the medium was replaced every 2–3 days. Clones (>50 cells) were regarded as tumorspheres. The colony formation efficiency was defined as [(number of colonies)/(number of cells seeded)] × 100.
Cell invasion assay
Cells were transfected with either miR-146a inhibitor or siVEGF and were subjected to the Transwell assay. The detailed protocol was conducted as previously reported [Citation28].
MiRNA target gene prediction and luciferase reporter assay
The target genes of miR-146a were predicted by using online prediction tools, such as PicTar (http://pictar.mdc-berlin.de/), TargetScan (http://www.targetscan.org/index.html) and miRanda-mirSVR (http://www.microrna.org/microrna/home.do). VEFG was identified to have a potential binding site of miR-146a. The luciferase reporter assay (Promega, Fitchburg, WI, USA) was performed to identify whether VEFG 3′-UTR was the binding site of miR-146a. The detailed protocol was conducted as previously reported [Citation29].
Injection of modified TCs into nude mice
The TCs that were transfected with miR-146a inhibitor were suspended in serum-free DMEM and subcutaneously injected (1 × 105 cells) into the mid-abdominal area of nude female C57BL/6 mice (8 weeks, BW: 19.5 ± 2.3 g; Jackson Laboratory, Bar Harbor, ME, USA). Mice were subjected to necropsy 28 days after injection and tumor size was measured.
Statistical analyses
Data were analyzed by SPSS 19.0 software (SPSS, Chicago, IL, USA) and were expressed as means ± standard error of the mean. Each independent experiment had a sample size of 3–5 in each treatment group. The two-group comparison was analyzed by two-tailed t-tests and multiple comparisons analysis was analyzed using the F-test ANOVA and the post-hoc Tukey test. In this study, differences were regarded to be significant at p < .05.
Results
TCs enrichment and identification
HeLa cell-derived CSCs were enriched by the spheroid culture method [Citation30] and the representative images of the stem cell spheres were taken (. HeLa cells were cultured on ultra-low attachment plates in serum-free medium containing EGF and bFGF, which allows the stem cells to proliferate and form spheres exclusively [Citation30]. To assess cell identification, ALDH activity [Citation31], one of the hallmarks of CSCs phenotype, was evaluated in TCs that were harvested by dissociating tumorspheres. The results revealed that the ALDH activity was significantly enhanced in TCs relative to DTCs and HeLa cells (. Furthermore, stem cell transcription factors (SCTFs), such as Nanog, Oct4, and Sox2, positively express in CSCs and are regarded as CSC markers [Citation32]. To further identify stem cell properties, SCTFs expression levels were detected using real-time PCR and Western blots. The results revealed that the expressions of Nanog, Oct4, and Sox2 elevated in TCs compared with DTCs ().
Figure 1. Cervical cancer tumorsphere cells (TCs) enrichment. (A) Representative images of TCs and differentiated TCs (DTCs). Original magnification × 100. (B) ALDH activity in the HeLa cell line TCs and DTCs. (C) Expressions of stem cell transcription factors (SCTFs): Nanog, Oct4, and Sox2 in TCs and DTCs. (*) denotes differences from the control group (p < .05). Values are means ± standard error of the mean. Three to five samples were available for the analysis in each treatment.
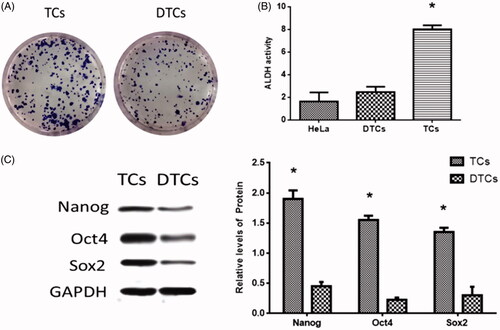
Effects of miR-146a on TCs colony formation and invasion
Real-time PCR results revealed that the expression of miR-146a was higher in DTCs relative to TCs (), suggesting that miR-146a may be associated with TCs differentiation. After transfection with miR-146a inhibitor and mimics, the expression of miR-146a was downregulated and upregulated, respectively (. The TCs transfected with miR-146a inhibitor increased colony formation and cell invasion compared to control TCs () whereas miR-146a mimics transfection resulted in opposite effects in TCs (), suggesting that miR-146a plays an important role in TCs tumorigenesis and invasion.
Figure 2. Effects of miR-146a on tumorsphere cells (TCs) colony formation and invasion. (A) MiR-146a expression in TCs and differentiated TCs (DTCs). (B) MiR-146a expression in TCs transfected with miR-146a inhibitor or mimics. (C, D). Effects of miR-146a inhibitor on TCs colony formation and invasion. (E, F). Effects of miR-146a mimics on TCs colony formation and invasion. (*) denotes difference from the control group (p < .05). Values are means ± standard error of the mean. Three to five samples were available for the analysis in each treatment.
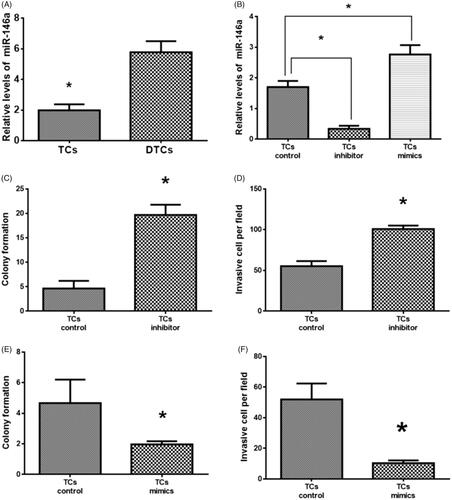
MiR-146a regulated TCs tumorigenesis by targeting VEGF
To further explore the target genes of miR-146a in TCs tumorigenesis and invasion, the target genes of miR-146a were first predicted using online prediction tools, such as PicTar (http://pictar.mdc-berlin.de/), TargetScan (http://www.targetscan.org/index.html) and miRanda-mirSVR (http://www.microrna.org/microrna/home.do). A binding sequence of miR-146a was found in the 3′UTR of VEGF (. There was an inverse relationship between miR-146a and VEGF expression (. The luciferase reporter assay results revealed that the luciferase activity was reduced in miR-146a mimics-transfected TCs with wild-type 3′UTR reporter whereas the luciferase activity did not show a significant change in TCs with the mutant 3′UTR reporter (. These results suggest that VEGF may be a direct target of miR-146a in TCs.
Figure 3. MiR-146a targets vascular endothelial growth factor (VEGF). (A). Prediction of miR-146a binding sequence on the 3’-UTR of VEGF. (B). VEGF expression in tumorsphere cells (TCs) transfected with miR-146a inhibitor or mimics. (C). Relative luciferase activity. (*) denotes difference from the control group (p < 0.05). Values are means ± standard error of the mean. 3–5 samples were available for the analysis in each treatment.
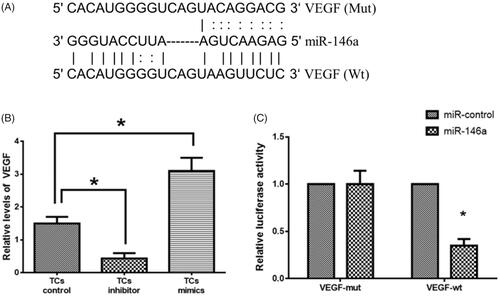
Downregulation of VEGF reversed effects of miR-146a inhibitor in TCs
Since reduced expression of miR-146a led to increased colony formation and cell invasion, siVEGF were applied to TCs transfected with the miR-146a inhibitor. There was decreased VEGF expression in TCs transfected with the miR-146a inhibitor (. The TCs transfected with miR-146a inhibitor displayed decreased colony formation and cell invasion compared to control TCs without transfection of siVEGF (). These results suggested that VEGF was not only the direct target of miR-146a in TCs but also may participate in the regulation of tumorigenesis and invasion in TCs.
Figure 4. Effects of vascular endothelial growth factor (VEGF) on tumorsphere cells (TCs) colony formation and invasion. (A) VEGF expression in TCs transfected with miR-146a inhibitor and siVEGF, respectively. (B, C) Colony formation and invasion in TCs transfected with miR-146a inhibitor and siVEGF, respectively. (*) denotes difference from the control group (p<.05). Values are means ± standard error of the mean. Three to five samples were available for the analysis in each treatment.
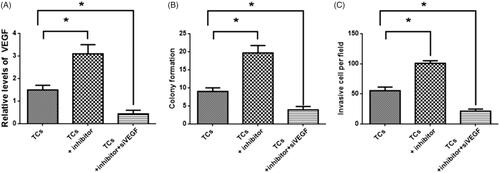
MiR-146a regulated TCs tumorigenesis and invasion via CDC42/PAK1 signaling
To further investigate the downstream signaling mediating effects of miR-146a on TCs tumorigenesis and invasion, Western blot results revealed that in miR-146a inhibitor-treated TCs, protein expressions of both CDC42 and PAK1 were decreased (), while in TCs transfected with siVEGF, CDC42 and PAK1 expressions were increased (. The PAK1 inhibitor, IPA3 [Citation33], significantly reversed the effects of miRNA-146a inhibitor on TCs (). Collectively, the results demonstrated that CDC42/PAK1 signaling may mediate miR-146a-associated effects on tumorigenesis and invasion in TCs.
Figure 5. CDC42/PAK1 signaling was involved in tumorsphere cells (TCs) colony formation and invasion. (A) CDC42 and PAK1 expressions in TCs transfected with miR-146a inhibitor. (B) CDC42 and PAK1 expressions in TCs transfected with siVEGF. (C, D) Effects of PAK1 inhibitor, IPA3, in TCs colony formation and invasion. (*) denotes difference from the control group (p < .05). Values are means ± standard error of the mean. Three to five samples were available for the analysis in each treatment.
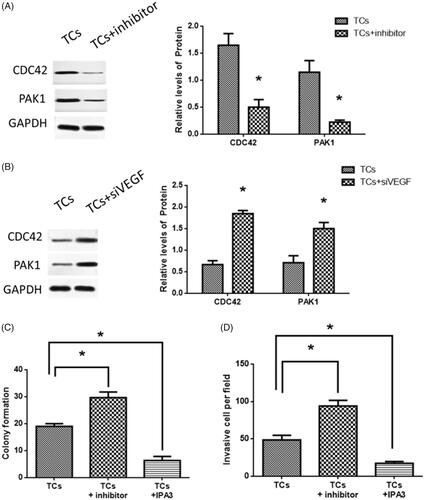
MiR-146a inhibitor promoted tumor growth in vivo
Tumors from mice injected with TCs treated with miR-146a inhibitor were larger than those from control mice (. Protein expressions of VEGF, CDC42 and PAK1 were reduced in tumors of miR-146a inhibitor-treated mice ().
Figure 6. Effects of miR-146a inhibitor in tumor growth in a mouse model. (A) Tumor size. (B) Tumor formation in nude mice. (C) VEGF, CDC42 and PAK1 expressions in a mouse model. (*) denotes difference from the control group (p<.05). Values are means ± standard error of the mean. Three to five samples were available for the analysis in each treatment.
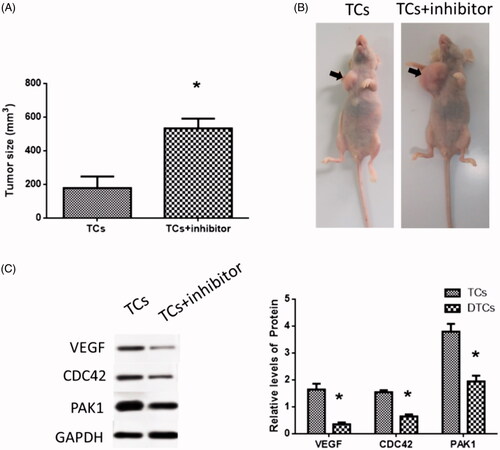
Discussion
As the second leading cause of mortality among females [Citation34], CC represents the fourth highest malignancy in women worldwide [Citation35]. Among these cases, majority of the deaths take place in developing areas and present at advanced stages [Citation36]. To date, there are many reports of CC patients developing recurrent diseases and metastasis. Since CSCs are regarded as the origin of tumor cell metastasis, the function of miR-146a in TCs tumorigenesis and invasion was elucidated. In TCs, there was a low expression of miR-146a and high expression of SCTFs compared with those in DTCs. Upregulation and downregulation of miR-146a in TCs led to decreased and increased colony formation and invasion, respectively. Furthermore, the effects of miR-146a may be mediated by VEGF through the CDC42/PAK1 signaling pathway.
As a distinct population of cells in tumors, CSCs are capable of initiating and inducing tumor growth and invasion [Citation37]. The properties of CSCs are similar to somatic stem cells due to their ability to self-renew and differentiate to non-CSCs [Citation38]. In addition, due to the heterogeneity of CSCs in various cancers, the stem cell markers have been intensively studied. These markers are used to assess the identification of CSCs, particularly in CC, in which the stem cell-enriched areas are essential for HPV infection and carcinogenesis [Citation39]. In the present study, CCSCs were enriched from the HeLa cell line using the spheroid method and applied to several SCTFs, which were recognized as CSC markers to assess the identification of CCSCs. In TCs, Sox2, Oct4, and Nanog were upregulated, while in DTCs, they were downregulated, suggesting that these SCTFs were critical for CCSCs maintenance and tumorigenesis. Moreover, the enriched TCs in the present study were bearing characteristics of CSCs. These results are consistent with previous studies that SCTFs play a significant role in maintaining the self-renewal and tumorigenicity in CCSCs [Citation40].
As a multi-functional regulator, miR-146a has been widely studied in different types of cancers. It has been reported that miR-146a is downregulated in the lung [Citation41] and gastric cancers [Citation42]. In addition, the upregulation of miR-146a is associated with reduced cancer cell proliferation [Citation42]. However, other reports suggest that increased miR-146a promotes tumorigenesis in CC [Citation43] and melanoma [Citation44]. Collectively, miR-146a plays a wide variety of roles in various cancers based on the regulation of genes and pathways. However, the roles of miR-146a in CSCs has received less attention. In this study, miR-146a expression at low levels was associated with enhanced colony formation and invasion in TCs while upregulation of miR-146a displayed the opposite effect. Furthermore, the expressions of SCTFs were inversely related to those of miR-146a. The downregulation of miR-146a was related to increased tumor size in vivo. Altogether, the results suggest that miR-146a may be an important regulator in tumorigenesis and invasion in CC, where miR-146a may modulate the activities of SCTFs.
To further elucidate the signaling underlying the effects of miR-146a in TCs, VEGF was identified as a direct target gene of miR-146a and was found to play a key role in the regulation of tumorigenesis and invasion in TCs. This suggests that VEGF, a tumor proangiogenic factor, is activated and silenced by miR-146a in TCs differentiation and tumorigenesis, respectively. The functions of VEGF in tumor angiogenesis has been intensively studied in the past decades [Citation45]. It is well noted that tumor vessels are dilated and tortuous and the vasculature is disorganized compared with normal vessels [Citation46]. This may result from the aberrant expression of angiogenic factors, such as VEGF [Citation46]. In addition, abnormal tumor angiogenesis is regarded as a major cause for lower tumor therapeutic effectiveness and the anti-VEGF regulator has increasingly become a potential strategy for cancer therapies [Citation47]. Altogether, the low-expression of miR-146a may contribute to angiogenesis in CC and miR-146a may be used as a promising anti-angiogenesis regulator in cancer treatments.
In the present study, the CDC42/PAK1 signaling pathway was activated in TCs’ tumorigenesis and invasion. In addition, CDC42 is an important GTP-binding protein that is associated with various signal transductions [Citation48]. The aberrant expression of CDC42 is involved in tumor genesis and progression, in which p21-activated kinase (PAK) 1 kinase, an effector of the CDC42, can facilitate this process by phosphorylating specific substrates including cyclinD1, cofilin and BAD [Citation49]. Thus, CDC42/PAK1 signaling is an essential modulatory pathway for tumor development [Citation49]. In this study, activated CDC42/PAK1 was associated with down-regulated miR-146a and upregulated VEGF, suggesting miR-146/VEGF/CDC42/PAK1 may play an important role in TCs tumorigenesis and invasion.
In conclusion, the results suggest that miR-146a may modulate TCs tumor formation and invasion in CC and the underlying mechanism may be associated with activated VEGF/CDC42/PAK1 signaling. This provides novel insight into the molecular basis and may be used as a promising therapy for CC.
Disclosure statement
The authors report no conflict of interest.
References
- Ferlay J, Soerjomataram I, Dikshit R, et al. Cancer incidence and mortality worldwide: sources, methods and major patterns in GLOBOCAN 2012. Int J Cancer. 2015;136(5):E359–86.
- Schiffman M, Wentzensen N, Wacholder S, et al. Human papillomavirus testing in the prevention of cervical cancer. JNCI. 2011;103(5):368–383.
- Kodama J, Seki N, Masahiro S, et al. Prognostic factors in stage IB-IIB cervical adenocarcinoma patients treated with radical hysterectomy and pelvic lymphadenectomy. J Surg Oncol. 2010;101(5):413–417.
- Ferlay J, Colombet M, Soerjomataram I, et al. Estimating the global cancer incidence and mortality in 2018: GLOBOCAN sources and methods. Int J Cancer. 2019;144(8):1941–1953.
- Bonnet D, Dick JE. Human acute myeloid leukemia is organized as a hierarchy that originates from a primitive hematopoietic cell. Nat Med. 1997;3(7):730–737.
- O'Brien CA, Pollett A, Gallinger S, et al. A human colon cancer cell capable of initiating tumour growth in immunodeficient mice. Nature. 2007;445(7123):106–110.
- Al-Hajj M, Wicha MS, Benito-Hernandez A, et al. Prospective identification of tumorigenic breast cancer cells. Proc Natl Acad Sci USA. 2003;100(7):3983–3988.
- Clarke MF, Dick JE, Dirks PB, et al. Cancer stem cells–perspectives on current status and future directions: AACR workshop on cancer stem cells. Cancer Res. 2006;66(19):9339–9344.
- Park CY, Tseng D, Weissman IL. Cancer stem cell-directed therapies: recent data from the laboratory and clinic. Mol Ther. 2009;17(2):219–230.
- Baccelli I, Trumpp A. The evolving concept of cancer and metastasis stem cells. J Cell Biol. 2012;198(3):281–293.
- Liu R, Wang X, Chen GY, et al. The prognostic role of a gene signature from tumorigenic breast-cancer cells. The. N Engl J Med. 2007;356(3):217–226.
- Bortolomai I, Canevari S, Facetti I, et al. Tumor initiating cells: development and critical characterization of a model derived from the A431 carcinoma cell line forming spheres in suspension. Cell Cycle. 2010;9(6):1194–1206.
- Wang K, Zeng J, Luo L, et al. Identification of a cancer stem cell-like side population in the HeLa human cervical carcinoma cell line. Oncol Lett. 2013;6(6):1673–1680.
- Liu SY, Zheng PS. High aldehyde dehydrogenase activity identifies cancer stem cells in human cervical cancer. Oncotarget. 2013;4(12):2462–2475.
- Engels BM, Hutvagner G. Principles and effects of microRNA-mediated post-transcriptional gene regulation. Oncogene. 2006;25(46):6163–6169.
- Zhou X, Yue Y, Wang R, et al. MicroRNA-145 inhibits tumorigenesis and invasion of cervical cancer stem cells. Int J Oncol. 2017;50(3):853–862.
- Wang W, Li Y, Liu N, et al. MiR-23b controls ALDH1A1 expression in cervical cancer stem cells. BMC Cancer. 2017;17(1):292.
- Jubb AM, Pham TQ, Hanby AM, et al. Expression of vascular endothelial growth factor, hypoxia inducible factor 1 alpha and carbonic anhydrase IX in human tumours. J Clin Pathol. 2004;57(5):504–512.
- Cao Y, Liu Q. Therapeutic targets of multiple angiogenic factors for the treatment of cancer and metastasis. Adv Cancer Res. 2007;97:203–224.
- Yang Y, Zhang Y, Iwamoto H, et al. Discontinuation of anti-VEGF cancer therapy promotes metastasis through a liver revascularization mechanism. Nat Commun. 2016;7(1):12680.
- Li D, Wei Y, Wang D, et al. MicroRNA-26b suppresses the metastasis of non-small cell lung cancer by targeting MIEN1 via NF-κB/MMP-9/VEGF pathways. Biochem Biophys Res Commun. 2016;472(3):465–470.
- Zu Y, Yang Y, Zhu J, et al. MiR-146a suppresses hepatocellular carcinoma by downregulating TRAF6. Am J Cancer Res. 2016;6(11):2502–2513.
- Liu Q, Wang W, Yang X, et al. MicroRNA-146a inhibits cell migration and invasion by targeting RhoA in breast cancer. Oncol Rep. 2016;36(1):189–196.
- Lu D, Yao Q, Zhan C, et al. MicroRNA-146a promote cell migration and invasion in human colorectal cancer via carboxypeptidase M/src-FAK pathway. Oncotarget. 2017;8(14):22674–22684.
- Hung PS, Liu CJ, Chou CS, et al. miR-146a enhances the oncogenicity of oral carcinoma by concomitant targeting of the IRAK1, TRAF6 and NUMB genes. PLOS One. 2013;8(11):e79926.
- Livak KJ, Schmittgen TD. Analysis of relative gene expression data using real-time quantitative PCR and the 2(-Delta Delta C(T)) Method. Methods. 2001;25(4):402–408.
- Lian C, Wu Z, Gao B, et al. Melatonin reversed tumor necrosis factor-alpha-inhibited osteogenesis of human mesenchymal stem cells by stabilizing SMAD1 protein. J Pineal Res. 2016;61(3):317–327.
- Pulito C, Mori F, Sacconi A, et al. Metformin-induced ablation of microRNA 21-5p releases Sestrin-1 and CAB39L antitumoral activities. Cell Discov. 2017;3(1):17022.
- Liu W, Xia P, Feng J, et al. MicroRNA-132 upregulation promotes matrix degradation in intervertebral disc degeneration. Exp Cell Res. 2017;359(1):39–49.
- Jung P, Sato T, Merlos-Suarez A, et al. Isolation and in vitro expansion of human colonic stem cells. Nat Med. 2011;17(10):1225–1227.
- Liu P, Brown S, Goktug T, et al. Cytotoxic effect of disulfiram/copper on human glioblastoma cell lines and ALDH-positive cancer-stem-like cells. Br J Cancer. 2012;107(9):1488–1497.
- Liu A, Yu X, Liu S. Pluripotency transcription factors and cancer stem cells: small genes make a big difference. Chin J Cancer. 2013;32(9):483–487.
- Wang Z, Oh E, Clapp DW, et al. Inhibition or ablation of p21-activated kinase (PAK1) disrupts glucose homeostatic mechanisms in vivo. J Biol Chem. 2011;286(48):41359–41367.
- Siegel RL, Miller KD, Jemal A. Cancer statistics, 2016. CA Cancer J Clin. 2016;66(1):7–30.
- Jemal A, Bray F, Center MM, et al. Global cancer statistics. CA Cancer J Clin. 2011;61(2):69–90.
- Shepherd JH. Cervical cancer. Best Pract Res Clin Obstet Gynaecol.. 2012;26(3):293–309.
- Lobo NA, Shimono Y, Qian D, et al. The biology of cancer stem cells. Annu Rev Cell Dev Biol. 2007;23:675–699.
- Dalerba P, Cho RW, Clarke MF. Cancer stem cells: models and concepts. Annu Rev Med. 2007;58:267–284.
- Chhabra R. Cervical cancer stem cells: opportunities and challenges. J Cancer Res Clin Oncol. 2015;141(11):1889–1897.
- Ji J, Zheng PS. Expression of Sox2 in human cervical carcinogenesis. Hum Pathol. 2010;41(10):1438–1447.
- Chen G, Umelo IA, Lv S, et al. miR-146a inhibits cell growth, cell migration and induces apoptosis in non-small cell lung cancer cells. PLOS One. 2013;8(3):e60317.
- Yao Q, Cao Z, Tu C, et al. MicroRNA-146a acts as a metastasis suppressor in gastric cancer by targeting WASF2. Cancer Lett. 2013;335(1):219–224.
- Wang X, Tang S, Le SY, et al. Aberrant expression of oncogenic and tumor-suppressive microRNAs in cervical cancer is required for cancer cell growth. PLOS One. 2008;3(7):e2557.
- Philippidou D, Schmitt M, Moser D, et al. Signatures of microRNAs and selected microRNA target genes in human melanoma. Cancer Res. 2010;70(10):4163–4173.
- Sullivan LA, Brekken RA. The VEGF family in cancer and antibody-based strategies for their inhibition. mAbs. 2010;2(2):165–175.
- Carmeliet P, Jain RK. Angiogenesis in cancer and other diseases. Nature. 2000;407(6801):249–257.
- Carmeliet P. VEGF as a key mediator of angiogenesis in cancer. Oncology. 2005;69(Suppl 3):4–10.
- Parrini MC, Camonis J, Matsuda M, et al. Dissecting activation of the PAK1 kinase at protrusions in living cells. J Biol Chem. 2009;284(36):24133–24143.
- Cheng Z, Liu F, Wang G, et al. miR-133 is a key negative regulator of CDC42-PAK pathway in gastric cancer. Cellular Signal. 2014;26(12):2667–2673.