Abstract
Propofol, an intravenous anaesthetic agent, has been found to exhibit antitumour effects in various kinds of cancer cells. However, the potential roles and regulatory mechanisms of propofol in oral squamous cell carcinoma (OSCC) remain unknown. Herein, we found that propofol inhibits OSCC cell growth and promotes cell apoptosis in a dose- and time-dependent manner. Further mechanistic studies revealed that the long noncoding RNA GAS5 is induced by propofol in OSCC cells. Elevated GAS5 acts as a competing endogenous RNA for miR-1297 and attenuates its inhibitory effect on GSK3β, leading to GSK3β increase and Mcl1 decrease. Additionally, we found that FoxO1 binds to the promoter of GAS5, facilitating its transcription in response to propofol treatment. Thus, these results suggest that propofol exhibits antitumour effects in OSCC cells and that the FoxO1-GAS5-miR-1297-GSK3β axis plays an important role in propofol-induced OSCC cell apoptosis.
Introduction
Oral squamous cell carcinoma (OSCC) is one of the most frequent head and neck tumours and accounts for more than 90% of all oral malignancies [Citation1,Citation2]. Although multiple therapeutic strategies can be administered clinically to treat OSCC, the overall 5-year survival rate after diagnosis remains less than 50%, mainly owing to its metastasis or resistance to conventional therapeutic drugs [Citation3]. Thus, in order to improve the prognosis of patients with OSCC, it is important to identify effective therapeutic drugs.
Propofol (2,6-diisopropylphenol) is extensively used as an intravenous anaesthetic agent due to its rapid induction and fast recovery from anaesthesia [Citation4]. Accumulating evidence has indicated that propofol exerts a number of non-anaesthetic effects, including antitumour activity [Citation5]. Recently, propofol was reported to trigger apoptosis and suppress proliferation, motility and invasion in multiple human cancers, including breast cancer, pancreatic cancer, ovarian cancer, gastric cancer, lung cancer and hepatocellular carcinoma [Citation6–10]. However, the effect of propofol on OSCC cells remains unknown.
Long non-coding RNAs (lncRNAs) are a class of transcripts longer than 200 nucleotides with no protein-coding capacity [Citation11]. LncRNAs, initially thought to be non-functional, were recently shown to play important regulatory roles in many human diseases, especially in malignancies [Citation12]. Growth arrest-specific 5 (GAS5) can encode ncRNAs of various sizes, including lncRNAs [Citation13]. Emerging evidence has proven that lncRNA GAS5 is an important tumour suppressor that is downregulated in many types of cancers and involved in many cellular processes, such as cell proliferation, apoptosis and invasion [Citation14]. Although GAS5 has been indicated to play an important role in cancer, the functions of GAS5 in response to propofol treatment are not well studied.
Here, we found that GAS5 is increased under propofol treatment in OSCC cells. Elevated GAS5 enhances propofol-induced OSCC cell apoptosis and inhibits cell proliferation. Subsequent mechanistic studies indicated that GAS5 acts as a competing endogenous RNA for miR-1297 and attenuates its inhibitory effect on GSK3β, which in turn facilitates the degradation of Mcl1 and promotes the antitumour activity of propofol. Additionally, we discovered that FoxO1 directly binds to the promoter of GAS5, facilitating its transcription in response to propofol treatment. Thus, these data indicate that the FoxO1-GAS5-miR-1297-GSK3β signalling axis plays an important role in propofol-induced OSCC cell apoptosis.
Materials and methods
Cell culture and reagents
The human OSCC cell line UM-SCC6 was provided by Dr. Thomas E. Cary (University of Michigan, Ann Arbor, MI). SCC-090 cells were obtained from American Type Culture Collection (ATCC). SCC-090 cells were cultured with MEM containing 10% foetal bovine serum (FBS, Gibco-BRL). The medium was renewed every day, and cells were passaged before reaching confluence. The following antibodies were used: GAPDH (Santa Cruz Biotechnology, Dallas, TX; SC-25778), PARP1 (Santa Cruz Biotechnology, SC-8007), Mcl1 (Cell Signalling Tech, 94296), phospho-Mcl1 (ser159/thr163) (Cell Signalling Tech, 4579), GSK3β (Cell Signalling Tech, 12456), GSK3α (Cell Signalling Tech, 4818), and FoxO1 (Cell Signalling Tech, 2880), Bcl2 (Cell Signalling Tech, 2872), pGSK3β(ser9) (Cell Signalling Tech, 5558), pGSK3α (ser21) (Cell Signalling Tech, 8452).
Real-time RT-PCR and RT-PCR
Total RNA was isolated using Trizol (Invitrogen, Waltham, MA). One microgram of total RNA was used to synthesize cDNA using PrimeScriptTM RT reagent kit (Takara, RR047A) according to the manufacturer’s instructions. The primers for miR-1297 were purchased from MyBioSource (MBS825976). The primers for GAS5 and Actin were as follows: GAS5: F: 5′- ACAGGCATTAGACAGAAAGC-3′ and R: 5′-TACCCAAGCAAGTCATCCA-3′; Actin: F: 5′-GACCTGACTGACTACCTCATGAAGAT-3′ and R: 5′-GTCACACTTCATGATGGAGTTGAAGG-3′.
RNA interference and virus infection
RNA interference was performed as previously described [Citation15]. The shRNA was purchased from Sigma, and the sequence targeting FoxO1 was 5′-TTAGACTGTGACATGGAAT-3′. GAS5 siRNAs were purchased from GenePharma Company (Shanghai, People’s Republic of China), and the siRNA sequences of GAS5 were as follows: (1) 5'-GGACCAGCTTAATGGTTCT-3′ and (2) 5′-GCAAGCCTAACTCAAGCCA-3'.
Introduction of microRNA mimics and inhibitors
Mimics and inhibitors of miRNA-1297 were synthesized by the GenePharma Company (Shanghai, People’s Republic of China). For each transfection in a six-well plate, 100 nM miRNA mimics, scramble or inhibitor, or scramble was used. The transfection of melanoma cells by Oligofectamine (Invitrogen) was performed according to the manufacturer’s instructions.
Dual-luciferase reporter assay
The assay was performed as previously described [Citation15–17].
ChIP assay
Cells were crosslinked with 1% formaldehyde for 10 min at room temperature. The ChIP assay was completed according to the manufacturer’s instructions using FoxO1 antibody and the ChIP assay kit (Millipore, Merck KGaA, Darmstadt, Germany). Anti-rabbit IgG was used as a control. The bound DNA fragments were eluted and amplified by PCR. The PCR products were separated on a 2% agarose gel by gel electrophoresis.
Colony formation and EdU assays
UM-SCC and SCC090 cells with knockdown of GAS5 were harvested and thoroughly pipetted to generate single-cell suspensions in complete culture media at a given concentration (such as 1 × 106/ml). The single-cell suspension was diluted to 500 or 1000 cells per well of a 6 well-plate and incubated at 37 °C with 5% CO2 for 2 weeks. Then, the colonies were stained with 0.04% crystal violet-2% ethanol in PBS. Photographs of the stained colonies were then taken.
The EdU assay was performed with an EdU Assay Kit (Guangzhou RIBOBIO, Guangzhou, China) according to the manufacturer's instructions. Briefly, the cells were incubated with DMEM medium containing 50 μM EdU for 2 h. The nuclei were also stained with Hoechst 33342 (Sigma, St. Louis, MO), and the images were acquired with an Olympus DP71X microscope (Olympus, Tokyo, Japan).
Caspase-3 activity assay
Caspase-3 activity was analyzed using the Caspase-3 Activity Assay Kit (Roche) according to the manufacturer’s instructions. Briefly, the tissue lysate was incubated with 100 μL of caspase-3 reagent at 37 °C for 1–2 h, and the fluorescence intensity (at 370–425 nm excitation and 490–530 nm emission wavelengths) was then measured using a Spectra MAX M5 spectrophotometer (Molecular Devices, Sunnyvale, CA).
Statistics and data analyses
The data are expressed as the means ± SD, and the statistical evaluation was performed using one-way analysis of variance (ANOVA). Values of p < .05 were considered statistically significant.
Results
Propofol triggers OSCC cell apoptosis and inhibits cell proliferation
To investigate the effects of propofol on OSCC cells, we first examined the influence of propofol on cell apoptosis. We treated the OSCC cell lines UM-SCC6 and SCC090 with propofol at different doses. After 48 h of treatment, cell apoptosis was analyzed. As shown in , PARP1 cleavage and Caspase 3 activity were elevated following treatment with increasing concentrations of propofol. Subsequently, UM-SCC6 and SCC090 were treated with 20 μg/ml propofol for different times, and cell apoptosis was detected. Similarly, we found that cleaved PARP1 and the activity of Caspase 3 were increased following longer propofol treatment durations ().
Figure 1. Propofol inhibits OSCC cell growth and promotes cell apoptosis. (A–D) UM-SCC6 and SCC090 cells were treated with propofol at the indicated concentrations, and cleaved PARP1 and Caspase 3 activity was detected. (E–H) UM-SCC6 and SCC090 cells were treated with 20 μg/ml propofol for the indicated times, and cleaved PARP1 and Caspase 3 activity was detected. (I–M) UM-SCC6 and SCC090 were treated with propofol as indicated. Cell proliferation was analyzed by colony formation and EdU assays. For (B), (D), (F), (H), (J), (K) and (M), the results are representative of three independent experiments, and the data are shown as the means ± s.d. Student’s t-test, *p < .05, **p < .01, ***p < .001.
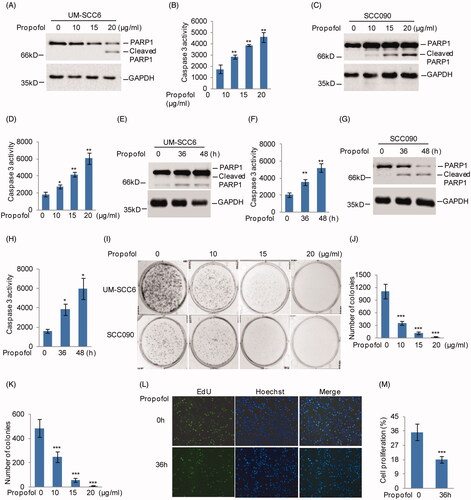
In addition, the effects of propofol on cell proliferation were also analyzed. Colony formation and EdU assays revealed that propofol treatment significantly reduced the proliferation of UM-SCC6 and SCC090 cells (). Therefore, our data suggest that propofol exerts suppressive effects on OSCC cells in a dose- and time-dependent manner.
LncRNA GAS5 induced by propofol inhibits cell growth and promotes cell apoptosis in OSCC cells
To explore the underlying mechanism of the suppressive effects of propofol on OSCC cells, UM-SCC6 cells were treated with 20 μg/ml propofol for the indicated times, and lncRNA expression profiles were then obtained by RNA sequencing analysis. The sequencing results revealed more than 51 upregulated and 83 downregulated genes in UM-SCC6 cells upon propofol treatment compared with control cells (. Among the altered lncRNAs, we found that GAS5 was significantly increased in response to propofol treatment (. To confirm this, UM-SCC6 and SCC090 cells were treated with propofol at different doses and times. Consistent with the RNA sequencing analysis, GAS5 was dramatically upregulated upon propofol treatment in OSCC cells ().
Figure 2. Propofol enhances GAS5 expression in OSCC cells. (A, B) UM-SCC6 cells were treated with 20 μg/ml propofol as the indicated times, and the altered lncRNAs were analyzed by RNA sequencing analysis. (C) The upregulated lncRNAs (fold change >2) are listed. (D–G) UM-SCC6 and SCC090 cells were treated with propofol as indicated. GAS5 expression levels were analyzed by q-RT-PCR. (H–M) GAS5 was knocked down in UM-SCC6 and SCC090 cells, and the cells were then treated with propofol as indicated. Cell proliferation was analyzed by colony formation assay, and cell apoptosis was analyzed by western blot. For (D–G), (I), and (K), the results are representative of three independent experiments, and the data are shown as the means ± s.d. Student’s t-test, *p < .05, **p < .01, ***p < .001.
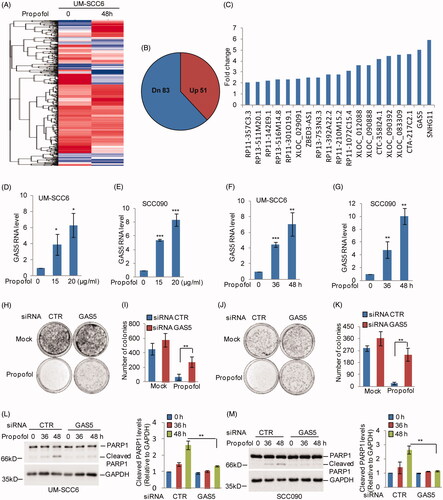
Next, to investigate the role of GAS5 in propofol-induced cell decrease in proliferation and increase in cell apoptosis, we first knocked down GAS5 expression using siRNAs in UM-SCC6 and SCC090 cells. The cells were then treated with propofol as indicated. Cell proliferation and cell apoptosis were determined by western blotting and colony formation assays. As shown in , knockdown of GAS5 partially attenuated the increased apoptosis and reduced colony formation caused by propofol treatment. Thus, these results reveal that GAS5 is an important regulator of propofol-induced OSCC cell apoptosis increase and cell proliferation decrease.
GAS5 acts as a ceRNA of miR-1297 and downregulates miR-1297 expression upon propofol treatment
GAS5 can act as a competing endogenous RNA (ceRNA) to regulate tumourigenesis [Citation14]. A recent study indicated that GAS5 sponges miR-205 and suppresses miR-205 expression [Citation18]. Thus, we asked whether GAS5 promotes propofol-induced OSCC cell apoptosis by binding microRNAs. Based on the RNA sequencing data, we found that miR-1297 was dramatically decreased in response to propofol treatment (. The bioinformatics analysis also suggested that GAS5 contains a potential binding region of miR-1297 (. To confirm this, we first examined the expression of miR-1297 in response to propofol treatment and found that propofol significantly reduced miR-1297 expression in OSCC cells (. However, the propofol-induced downregulation of miR-1297 was recovered by GAS5 knockdown in OSCC cells (. Then, RIP was performed on UM-SCC6 cell extracts using an antibody against Ago2 to test whether GAS5 could function as a miR-1297 sponge. The q-RT-PCR results showed that GAS5 and miR-1297 were preferentially enriched in the anti-Ago2 immunoprecipitates compared with the IgG immunoprecipitates (. We next inserted the wild-type GAS5 or the mutated version downstream of the luciferase gene in the reporter plasmid for the luciferase assay (. Compared with the control group, the luciferase activity of the vector with wild-type GAS5 was notably inhibited by miR-1297, and the reduction was abolished when the binding site was mutated (. In contrast, the luciferase activity of the vector with GAS5 was notably increased in response to propofol treatment; however, the increase disappeared when miR-1297 was overexpressed (. Thus, these results indicate that GAS5 can act as a ceRNA of miR-1297 and inhibits its expression.
Figure 3. GAS5 acts as a ceRNA of miR-1297. (A) The RNA level of miR-1297 is listed. (B) The binding site between GAS5 and miR-1297 was predicated from starBase. (C) UM-SCC6 and SCC090 were treated with propofol as indicated. The RNA levels of miR-1297 were analyzed by q-RT-PCR. (D, E) UM-SCC6 and SCC090 cells with or without GAS5 knockdown were treated with propofol for the indicated times. The expression levels of miR-1297 were analyzed. (F, G) RNA-binding protein immunoprecipitation was performed using anti-Ago2 antibody in UM-SCC6 cells, and IgG was used as the negative control. Q-RT-PCR was performed to detect the pull-down of GAS5 and miR-1297. (H) Schematic diagrams of luciferase reporters containing the wild-type binding site of miR-1297 on GAS5, named psiCHECK2-GAS5 wild type (WT), and the mutant binding site, named psiCHECK2-GAS5 Mut. (I) psiCHECK2-GAS5 WT or psiCHECK2-GAS5 Mut was co-transfected with miR-1297 or the negative control into UM-SCC6 cells, and luciferase activity was measured. (J) psiCHECK2-GAS5 WT together with miR-1297 or the negative control was transfected into UM-SCC6 cells. The cells were then treated with propofol for the indicated times, and luciferase activity was measured. For B-F, H, and I, the results are representative of three independent experiments, and the data are shown as the means ± s.d. Student’s t-test, *p < .05, **p < .01, ***p < .001.
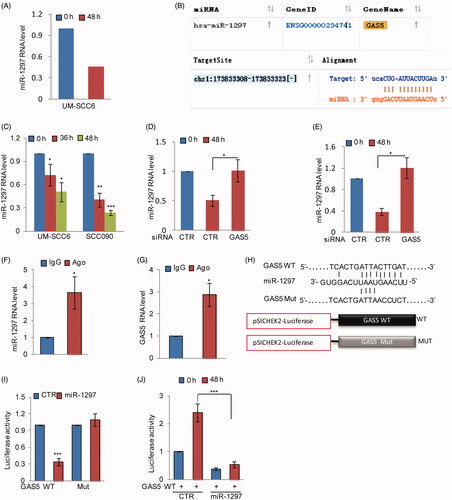
MiR-1297 suppresses GSK3β in human OSCC cells
Having identified that GAS5 promotes propofol-induced apoptosis via binding miR-1297, we next sought to investigate the downstream targets of miR-1297. Previous studies have indicated that propofol induces cell apoptosis by downregulating Bcl2 expression [Citation19]. Thus, we asked whether the Bcl2 is also involved in propofol-induced OSCC cell apoptosis. To this end, we first detected the expression levels of Bcl2 family proteins Bcl2 and Mcl1 in response to propofol treatment. Interestingly, we found that Mcl1, but not Bcl2, was significantly decreased in UM-SCC6 cells upon propofol treatment. Additionally, phosphorylation of Mcl1 at ser 159 was increased in response to propofol treatment (. Increasing evidence has indicated that phosphorylation of Mcl1 at ser 159 plays an important role in Mcl1 stability [Citation20,Citation21]. To further validate that propofol affects Mcl1 protein stability, we treated the indicated cells with the protein synthesis inhibitor cycloheximide (CHX) together with or without propofol treatment. Notably, propofol decreased Mcl1 stability (.
Figure 4. miR-1297 suppresses GSK3β in human OSCC cells. (A) UM-SCC6 cells were treated with propofol for the indicated times. The expression levels of p-Mcl1 and Mcl1 were analyzed by western blot. (B, C) UM-SCC6 cells with or without knockdown of GAS5 were treated with 20 μg/ml propofol for 36 h, and the cells were incubated with 10 μM CHX for the indicated periods. Cell lysates from the indicated time points were subjected to western blot analysis with anti-Mcl1 antibody. (D, E) UM-SCC6 and SCC090 cells were treated with 20 μg/ml propofol for the indicated times. The expression levels of GSK3α and GSK3β were analyzed by western blot. (F) Schematic diagrams of luciferase reporters containing the wild-type or mutational binding site of miR-1297 on GSK3β 3'UTR, named GSK3β-3 UTR wild type (WT) or Mut. (G–I) GSK3β-3 UTR WT or Mut together with miR-1297 were transfected into UM-SCC6 and SCC090 cells. Luciferase activity was measured by luciferase assay, and the protein levels of GSK3β were analyzed by western blot. (J–L) GSK3β-3 UTR WT or Mut together with the miR-1297 inhibitor were transfected into UM-SCC6 and SCC090 cells. Luciferase activity was measured by luciferase assay, and the protein levels of GSK3β were analyzed by western blot. (M) UM-SCC6 cells with or without GAS5 knockdown were treated with 20 μg/ml propofol for the indicated times, and cell lysates were analyzed using the indicated antibodies. (N) miR-1297 inhibitor or the negative control was transfected into UM-SCC6 cells with or without GAS5 knockdown. The cells were then treated with 20 μg/ml propofol, and cell lysates were analyzed using the indicated antibodies. (O) Flag-GSK3β or the empty vector was transfected into UM-SCC6 cells with or without GAS5 knockdown, and the cells were then treated with 20 μg/ml propofol. Cell lysates were analyzed using the indicated antibodies. For G, H, J, and K, the results are representative of three independent experiments, and the data are shown as the means ± s.d. Student’s t-test, *p < .05, **p < .01, ***p < .001.
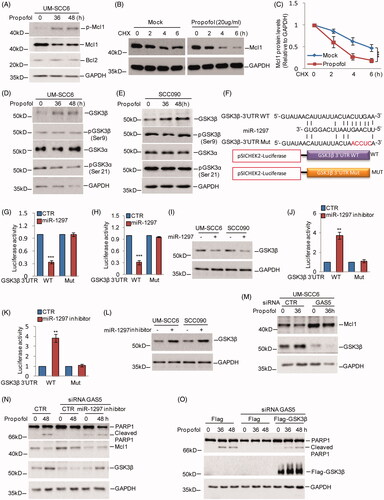
Glycogen synthase kinase 3 (GSK3) is reported to phosphorylate Mcl1 at Ser159 and trigger the degradation of Mcl1 [Citation21]. To determine whether the decrease in Mcl1 by propofol was GSK3-dependent, we investigated the expression levels of GSK3 upon propofol treatment in OSCC cells. As shown in , the expression levels of GSK3β, not the GSK3α or pGSK3β (ser 9) and pGSK3α (ser21), were induced by propofol.
Based on the data that miR-1297 is downregulated by propofol, we thus hypothesized that GSK3β is a potential target of miR-1297. To test this hypothesis, we first searched the TargetScan database for potential targets of miR-1297. Among the candidates identified, the 3′UTR of GSK3β contains a putative region (nucleotides 4636–4643) that matched perfectly to the miR-1297 “seed” region (.
To examine whether GSK3β was indeed inhibited by miR-1297, we introduced luciferase reporter plasmids containing the wild-type 3′UTR (WT) or the matched mutant (MUT) of GSK3β into UM-SCC6 and SCC090 cells, and the cells were then treated with or without the miR-1297 mimics. We found that overexpression of miR-1297 markedly suppressed the luciferase activity of the wild-type 3′UTR of GSK3β and led to a decrease in endogenous GSK3β (). Furthermore, treatment with the miR-1297 inhibitor resulted in the luciferase activity of wild-type 3′UTR elevation and increased GSK3β expression ().
To further determine whether GAS5 enhances propofol-induced OSCC cell apoptosis by regulating the miR-1297-GSK3β pathway, we first assessed the effect of GAS5 on GSK3β and Mcl1 upon propofol treatment. We found that knockdown of GAS5 suppressed the propofol-induced GSK3β increase and Mcl1 decrease, which was then reversed by the miR-1297 inhibitor in OSCC cells (. Additionally, cell apoptosis was detected by western blotting. We found that the decreased cell apoptosis by GAS5 knockdown was also reversed by the miR-1297 inhibitor or GSK3β overexpression upon propofol treatment (.
FoxO1 upregulates GAS5 expression upon propofol treatment in OSCC cells
Forkhead box protein O1 (FoxO1), an important transcription factor, has been reported to be upregulated in response to propofol treatment [Citation22]. A recent study indicated that FoxO1 transcriptionally upregulates GAS5 in human glioma stem cells [Citation23]. Thus, we asked whether FoxO1 contributes to the propofol-induced increase in GAS5 in OSCC cells. To address this, we first assessed FoxO1 expression under propofol treatment in OSCC cells and found that the expression levels of FoxO1 were increased (. Subsequently, we knocked down FoxO1 expression using shRNAs in UM-SCC6 and SCC090 cells, and the expression levels of GAS5 were then analyzed. As shown in , we found that knockdown of FoxO1 suppressed propofol-induced GAS5 expression, which then led to miR-1297 and Mcl1 increase and GSK3β decrease. In addition, we found four potential binding sites of FoxO1 on the GAS5 promoter, named PCR 1, 2, 3. Subsequent chromatin immunoprecipitation (ChIP) showed that the chromatin fragments corresponding to the putative FoxO1-binding sites (PCR 3) were specifically present in anti-FoxO1 immunoprecipitates from OSCC cells, and the binding increased upon propofol treatment (. Taken together, these data indicate that FoxO1 can bind to the promoter of GAS5, leading to its increase in response to propofol treatment.
Figure 5. FoxO1 promotes GAS5 expression in response to propofol treatment. (A) UM-SCC6 and SCC090 cells were treated with 20 μg/ml propofol for the indicated times, and the protein levels of FoxO1 were analyzed by western blot. (B–E) UM-SCC6 and SCC090 cells with or without FoxO1 knockdown were treated with 20 μg/ml propofol for the indicated times. The protein levels of FoxO1, GSK3β and Mcl1 were analyzed by western blot. The RNA levels of GAS5 and miR-1297 were analyzed by q-RT-PCR. (F) ChIP analysis shows the binding of FoxO1 to the GAS5 promoter. For C and E, the results are representative of three independent experiments, and the data are shown as the means ± s.d. Student’s t-test, *p < .05, **p < .01, ***p < .001.
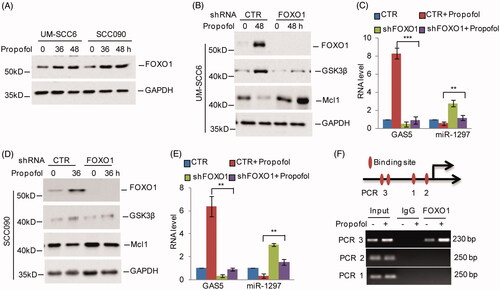
Discussion
Propofol is an intravenous sedative-hypnotic agent administered to induce and maintain anaesthesia [Citation4]. Additionally, many studies have reported that propofol exerts antitumour properties in numerous cancers [Citation5]. However, its role in OSCC remains unknown. In this study, we found that propofol suppresses OSCC cell proliferation and induces cell apoptosis via upregulating GAS5 expression. Elevated GAS5 can bind to miR-1297, leading to decreased miR-1297 in response to propofol treatment. Subsequently, we found that GSK3β is a downstream target gene of miR-1297. Propofol promotes GSK3β expression via miR-1297 downregulation. Increased GSK3β enhances Mcl1 degradation and propofol-induced cell apoptosis. Our data also revealed that FoxO1 directly binds to the promoter of GAS5, facilitating its transcription in response to propofol treatment. Taken together, these results suggest that propofol exhibits antitumour effects in OSCC.
GAS5 is a tumour suppressor gene located at chromosome 1q25 and was first discovered in 1988 by screening highly expressed genes in growth-arrested cells [Citation24]. Downregulation of GAS5 expression is observed in many cancers, including colorectal cancer, breast cancer, renal cell carcinoma, and lung cancer [Citation14]. Many studies have indicated that GAS5 is induced in response to various stimulants, such as ER stress and chemotherapeutic drugs [Citation25,Citation26]. Consistently, our study revealed that GAS5 is increased in response to propofol treatment in OSCC cells, and elevated GAS5 enhances propofol-induced cell apoptosis. Previous studies have suggested that GAS5 can function as a competing endogenous RNA (ceRNA) to regulate signalling pathways and biological functions [Citation27]. Bioinformatics analysis of complementary regions of GAS5 to microRNAs identified 690 candidates, among which 234 microRNAs showed statistically significant binding [Citation28]. In the context of cancer, GAS5 has been reported to bind to miR-21, miR-221 and miR-222 [Citation29,Citation30]. Similarly, we found that increased GAS5 by propofol can bind to miR-1297 and inhibit its expression in OSCC cells.
Accumulating studies have revealed that miR-1297 plays an important role in cancer. MiR-1297 was reported to suppress tumourigenesis in most cancers, including lung cancer, colorectal cancer, and prostate cancer [Citation31–33]. However, we found that GSK3β is a novel target gene of miR-1297. GSK3β and GSK3α are two isoforms of GSK3, which are encoded by separate genes. GSK3 is a serine/threonine kinase, and thus transfers a phosphate group to either the serine or threonine residues of its substrates [Citation34]. Phosphorylation of GSK3β at ser9 and GSK3α at ser 21 render their inactive [Citation35,Citation36]. Interestingly, we found that phosphorylation of GSK3β at ser9 and GSK3α ser 21 were not altered in response to propofol treatment. Only GSK3β was increased under propofol treatment in OSCC cells, which may own to the miR-1297 decrease by propofol. Thus, our data indicated that downregulation of miR-1297 by propofol enhances GSK3β expression and decreases Mcl1 protein levels.
In addition, we found that the transcription factor FoxO1 promotes GAS5 expression upon propofol treatment in OSCC cells [Citation23]. Previous studies have indicated that the protein levels of FoxO1 were increased in response to propofol treatment [Citation9]. FoxO1 was also reported to regulate GAS5 expression in glioma cells. Consistently, our data indicated that FoxO1 directly binds to the GAS5 promoter and facilitates its transcription upon propofol treatment. Collectively, our study suggests that FoxO1-GAS5-miR-1297-GSK3β plays an important role in propofol-induced OSCC cell apoptosis.
Disclosure statement
The authors declare no competing interests.
Additional information
Funding
References
- Seethala RR, Stenman G. Update from the 4th edition of the World Health Organization classification of head and neck tumours: tumors of the salivary gland. Head and Neck Pathol. 2017;11(1):55–67.
- Torre LA, Bray F, Siegel RL, et al. Global cancer statistics, 2012. CA Cancer J Clin. 2015;65(2):87–108.
- Warnakulasuriya S. Global epidemiology of oral and oropharyngeal cancer. Oral Oncol. 2009;45(4–5):309–316.
- Vasileiou I, Xanthos T, Koudouna E, et al. Propofol: a review of its non-anaesthetic effects. Eur J Pharmacol. 2009;605(1–3):1–8.
- Kim R. Effects of surgery and anesthetic choice on immunosuppression and cancer recurrence. J Transl Med. 2018;16(8):1–13.
- Meng C, Song L, Wang J, et al. Propofol induces proliferation partially via downregulation of p53 protein and promotes migration via activation of the Nrf2 pathway in human breast cancer cell line MDA-MB-231. Oncol Rep. 2017;37(2):841–848.
- Du QH, Xu YB, Zhang MY, et al. Propofol induces apoptosis and increases gemcitabine sensitivity in pancreatic cancer cells in vitro by inhibition of nuclear factor-kappaB activity. WJG. 2013;19(33):5485–5492.
- Wang P, Chen J, Mu LH, et al. Propofol inhibits invasion and enhances paclitaxel- induced apoptosis in ovarian cancer cells through the suppression of the transcription factor slug. Eur Rev Med Pharmacol Sci. 2013;17(13):1722–1729.
- Yang N, Liang Y, Yang P, et al. Propofol inhibits lung cancer cell viability and induces cell apoptosis by upregulating microRNA-486 expression. Braz J Med Biol Res. 2017;50(1):e5794.
- Gong T, Ning X, Deng Z, et al. Propofol-induced miR-219-5p inhibits growth and invasion of hepatocellular carcinoma through suppression of GPC3-mediated Wnt/beta-catenin signalling activation. J Cell Biochem. 2019;120(10):16934–16945.
- Ponting CP, Oliver PL, Reik W. Evolution and functions of long noncoding RNAs. Cell. 2009;136(4):629–641.
- Lalevee S, Feil R. Long noncoding RNAs in human disease: emerging mechanisms and therapeutic strategies. Epigenomics. 2015;7(6):877–879.
- Sun D, Yu Z, Fang X, et al. LncRNA GAS5 inhibits microglial M2 polarization and exacerbates demyelination. EMBO Rep. 2017;18(10):1801–1816.
- Ji J, Dai X, Yeung SJ, et al. The role of long non-coding RNA GAS5 in cancers. Cancer Manag Res. 2019;11:2729–2737.
- Ma B, Zhang L, Zou Y, et al. Reciprocal regulation of integrin beta4 and KLF4 promotes gliomagenesis through maintaining cancer stem cell traits. J Exp Clin Cancer Res. 2019;38(1):23.
- Yang X, Zhang D, Liu S, et al. KLF4 suppresses the migration of hepatocellular carcinoma by transcriptionally upregulating monoglyceride lipase. Am J Cancer Res. 2018;8(6):1019–1029.
- Lin J, Zhang D, Fan Y, et al. Regulation of cancer stem cell self-renewal by HOXB9 antagonizes endoplasmic reticulum stress-induced melanoma cell apoptosis via the miR-765-FOXA2 axis. J Invest Dermatol. 2018;138(7):1609–1619.
- Dong L, Li G, Li Y, et al. Upregulation of long noncoding RNA GAS5 inhibits lung cancer cell proliferation and metastasis via miR-205/PTEN axis. Med Sci Monit. 2019;25:2311–2319.
- He W, Yuan QH, Zhou Q. Histamine H3 receptor antagonist clobenpropit protects propofol-induced apoptosis of hippocampal neurons through PI3K/AKT pathway. Eur Rev Med Pharmacol Sci. 2018;22(22):8013–8020.
- Thomas LW, Lam C, Edwards SW. Mcl-1; the molecular regulation of protein function. FEBS Lett. 2010;584(14):2981–2989.
- Maurer U, Charvet C, Wagman AS, et al. Glycogen synthase kinase-3 regulates mitochondrial outer membrane permeabilization and apoptosis by destabilization of MCL-1. Mol Cell. 2006;21(6):749–760.
- Zhou D, Zhuang J, Wang Y, et al. Propofol alleviates DNA damage induced by oxygen glucose deprivation and reperfusion via FoxO1 nuclear translocation in H9c2 cells. Front Physiol. 2019;10:223.
- Zhao X, Liu Y, Zheng J, et al. GAS5 suppresses malignancy of human glioma stem cells via a miR-196a-5p/FOXO1 feedback loop. Biochim Biophys Acta Mol Cell Res. 2017;1864(10):1605–1617.
- Schneider C, King RM, Philipson L. Genes specifically expressed at growth arrest of mammalian cells. Cell. 1988;54(6):787–793.
- Zhang L, Wang Y, Zhang L, et al. ZBTB7A, a miR-663a target gene, protects osteosarcoma from endoplasmic reticulum stress-induced apoptosis by suppressing LncRNA GAS5 expression. Cancer Lett. 2019;448:105–116.
- Gao ZQ, Wang JF, Chen DH, et al. Long non-coding RNA GAS5 antagonizes the chemoresistance of pancreatic cancer cells through down-regulation of miR-181c-5p. Biomed Pharmacother. 2018;97:809–817.
- Xu C, Zhang Y, Wang Q, et al. Long non-coding RNA GAS5 controls human embryonic stem cell self-renewal by maintaining NODAL signalling. Nat Commun. 2016;7(1):13287.
- Wang J, Ma R, Ma W, et al. LncDisease: a sequence based bioinformatics tool for predicting lncRNA-disease associations. Nucleic Acids Res. 2016;44(9):e90.
- Zhang Z, Zhu Z, Watabe K, et al. Negative regulation of lncRNA GAS5 by miR-21. Cell Death Differ. 2013;20(11):1558–1568.
- Zhao X, Wang P, Liu J, et al. Gas5 exerts tumor-suppressive functions in human glioma cells by targeting miR-222. Mol Ther. 2015;23(12):1899–1911.
- Chen Z, Ma Y, Pan Y, et al. MiR-1297 suppresses pancreatic cancer cell proliferation and metastasis by targeting MTDH. Mol Cell Probes. 2018;40:19–26.
- Bu W, Luo T. miR-1297 promotes cell proliferation of non-small cell lung cancer cells: involving in PTEN/Akt/Skp2 signaling pathway. DNA Cell Biol. 2017;36(11):976–982.
- Wang Y, Xue J, Kuang H, et al. microRNA-1297 inhibits the growth and metastasis of colorectal cancer by suppressing cyclin D2 expression. DNA Cell Biol. 2017;36(11):991–999.
- Doble BW, Woodgett JR. GSK-3: tricks of the trade for a multi-tasking kinase. J Cell Sci. 2003;116(7):1175–1186.
- ter Haar E, Coll JT, Austen DA, et al. Structure of GSK3beta reveals a primed phosphorylation mechanism. Nat Struct Biol. 2001;8(7):593–596.
- Woodgett JR. Judging a protein by more than its name: GSK-3. Sci STKE. 2001;2001(100):re122001.