Abstract
Purpose
This study aimed to assess the protective effects of silibinin on blue light-emitting diode (LED)-induced retinal ganglion cells (RGCs) damage.
Methods
Silibinin was applied in RGCs damage in vitro model to test its protective effects. Cell viability was assessed with the MTT method and cell apoptosis was evaluated by TUNEL and Annexin V/propidium iodide staining. The expressions of apoptosis related proteins and influenced signalling pathways were measured using western blotting and immunohistochemistry. Inflammatory factors induced by RGC damage were detected using ELISA method.
Results
It was found that silibinin in 50 and 100 μM treatment showed a significant protective effect in RGCs under blue light damage. Apoptosis assay showed that silibinin treatment could significantly improve the apoptotic status of RGCs. When the potentially affected signal pathway was considered, blue light would down-regulate the expression of MEK1/ERK/CREB. The levels of inflammatory factors (TNF-α, IL-1β, IL-6 and IL-10) were significantly regulated by silibinin treatment.
Conclusions
Silibinin pretreatment would demonstrate protective effect against blue light induced acute RGCs damage. Silibinin treatment has a direct suppression of apoptosis and inflammation through the activation of MEK/ERK/CREB pathway in vitro.
Introduction
Glaucoma is regarded as a group of disorders marked by progressive degeneration and it is associated with the death of retinal ganglion cells (RGCs) and axons within the optic nerve or optic neuropathy [Citation1]. Glaucoma is now one of the leading causes of blindness, with an increasing prevalence in the whole world [Citation2] and an estimated 60 million people have optic neuropathy due to glaucoma [Citation3]. The incidence of primary open-angle glaucoma (POAG) increases with age and elevated intra-ocular pressure (IOP) and other signs such as a change in retinal morphology or loss of visual function are sufficient for a glaucoma diagnosis without an elevated IOP [Citation3]. The presently available marketed anti-glaucoma drugs formulations have issues of either difficulty in crossing the blood-retinal barrier or lower systemic bioavailability [Citation4]. Hence, the drugs with lower therapeutic index were in urgent need, which would eventually lead to deposition of concentrated solutions at ocular site, producing toxic effects and cellular damage to the eye.
Nowadays, the pathophysiology of glaucoma remains unclear and it is often defined by the loss of RGCs. In contrast to the peripheral nervous system, the central nervous system loses its capability of regeneration after injury. It was reported that apoptosis and inflammation were involved in retinal degeneration and they were also related to the development of glaucoma [Citation5]. According to medical studies of glaucoma, high IOP or retinal ischemia leads to constant RGC apoptosis and anti-apoptosis would help in the management of glaucoma [Citation6]. Mounting evidence suggests that neuroinflammation is a key process in glaucoma, yet the precise roles of neuroinflammation in glaucoma are still unknown. Understanding these complex processes, which may also be a key in other common neurodegenerations will lead to targeted therapeutics for a disease that affects as many as 80 million people worldwide. A most recent study demonstrated that antagonists of adenosine A2A receptor control retinal inflammation and afford protection to rat retinal cells in glaucoma models [Citation7]. Thus, innovative neuro-protective and -regenerative therapies are urgently required for potential glaucoma treatment. Thus, the idea of the study was to look for significantly effective drugs in the RGCs degeneration, which could alter the survival and regeneration of RGCs in glaucoma.
Silibinin, which is a flavonoid plant extract from the dried fruits of Silybum marianum L., is mainly composed of four flavonoids [Citation8]. Silibinin is one of the widely used drugs in clinical practice, and its pharmacological action characteristics are recognized, including anti-free radical activity, anti-lipid peroxidation, anti-lipoxygenase action, anti-glutathione (GSH) emptying effect, anti-tumor function and hypolipidemic effect [Citation9]. In another study, it was also found that silibinin is a protective drug in retinal diseases. In a recent study, silibinin pretreatment of RPE cells up-regulated proline hydroxylase-2 expression, inhibited HIF-1α subunit accumulation, and inhibited VEGF secretion. Silibinin-induced HIF-1α and VEGF down-regulation required suppression of hypoxia-induced phosphatidylinositol 3-kinase/Akt/mammalian target of rapamycin (mTOR) pathway [Citation10]. And according to a recent study, silibinin might play a role in the onset of diabetic peripheral neuropathy [Citation11]. Therefore, based on the aforementioned information, we hypothesized that silibinin could regulate blue light induced apoptosis and inflammation of RGCs and then demonstrate a significant protective effect.
Materials and methods
Cell culture of RGC
RGCs were isolated from retinas of postnatal day 6 neonatal mice according to the two-step immunopanning method following a published protocol [Citation12]. Subsequently, collected tissues were washed with phosphate-buffered saline (PBS) containing 100 U/mL penicillin and 50 µg/mL streptomycin for four times. Tissues were then digested with 0.25% trypsin at 37 °C for 25 min. Isolated cells were seeded at 2500 cells/cm2 on a PDL (Poly-d-lysine) and laminin-coated dish and cultured in RGC medium [serum-free B27-supplemented Neurobasal® Medium, minus phenol red. The culture medium was replaced every 10 min, for a total of three times. The un-adherent cells were removed by washing with PBS for three times. As previously described, primary RGCs were induced with 50 mmol/L glucose for subsequent experiments. Animals were handled according to the institutional guidelines on animal care of the University of Inner Mongolia Medical University.
In addition, the cultured RGCs were incubated for 24 h in either darkness or blue light (530 nm with a peak of 470 nm, 12.08 W/m2). Blue light was delivered by LEDs (Electro DH SL, Barcelona, Spain) and the temperature maintained at 37 °C throughout. The intensity of light emitted from LEDs was checked by use of a spectrophotometer bought from Konica Minolta (CL-70F).
Cell viability detection
Cell viability was determined with 3-(4,5-dimethylthiazol-2-yl)-2,5-diphenyltetrazolium bromide (MTT) colorimetric assay (Sigma-Aldrich, St. Louis, MO, USA) to assess the cell viability of RGCs. Briefly, confluent monolayers of RGCs in different groups were used and the media were removed at the end of the treatment period. After that, RGCs monolayers were then exposed to 200 µl of MTT solution (0.5 mg/mL in PBS) for 2 h. After washing the cells with PBS, the formazan crystals were solubilized by DMSO (200 µl per well; Sigma-Aldrich Chimie, St. Louis, MO) before the plates were measured at 540 nm.
TUNEL assay
RGCs at 80% confluency were treated with blue light or blue light in combination with different concentrations of silibinin for 4 h, and then co-incubated with palmitate for another 24 h. DNA fragments were labelled using the In Situ Cell Death Detection TMR red kit (Roche Diagnostics Corp., Indianapolis, IN, USA) following the manufacturer’s instructions. Cells were mounted with anti-fade reagent containing 40,6-diamidino-2-phenylindole (DAPI; Vector Laboratories, Inc., Burlingame, CA, USA). Images were captured under an inverted fluorescence microscope (Olympus, Tokyo, Japan). The ratio of TUNEL-positive cells among all the RGCs was indicated as apoptotic status. This was evaluated at a 20× magnification with Image-Pro Plus 6.0 Imaging System (Silver Springs, MD).
Cell apoptosis
RGCs were treated with either blue light or blue light with 2 h before exposure to silibinin. After 24 h of incubation, the cells were subsequently washed with PBS and stained with the Annexin V-FITC Apoptosis Detection Kit Cell apoptosis was measured using an Annexin V-FITC Apoptosis Detection Kit (BD Pharmingen, Franklin Lakes, NJ, USA) according to the manufacturer’s instructions by flow cytometry.
Western blot analysis
To determine the expression of cleaved caspase-3, cleaved caspase-9, Bax, Bcl-2 and phosphorylation of MEK1, ERK1/2 and CREB, protein isolated from RGCs were subjected to WB analysis. Similarly, RGCs from different groups were lysed in buffer containing 10 mM Tris (Sigma-Aldrich Corp., St. Louis, MO), 1 mM EDTA (Sigma-Aldrich Corp., St. Louis, MO, USA), and 0.1% Triton X-100 (Sigma-Aldrich Corp, St. Louis, MO, USA) to yield total protein. Lysates were centrifuged at 13,000 g for 20 min at 4 °C. After quantitation of the total protein in all the groups, an equal amount of protein (20 µg) was loaded in each lane and electrophoresed together with molecular weight standards (BioRad, Hercules, CA, USA) in separate lanes on a 10% SDS polyacrylamide gel. After electrophoresis, the proteins were transferred onto PVDF membranes (Millipore, Billerica, MA). The membrane was blocked with 5% nonfat dry milk for 2 h and after that incubated overnight at 4 °C with primary antibody in 1:1000 dilution. Image-Pro Plus 6.0 Imaging System was used for analyzing the blot results.
Inflammatory factors detection
The supernatant of culture media was collected from cell culture media and stored in anticoagulant-free tubes. The levels of interleukin-1β (IL-1β), tumor necrosis factor-α (TNF-α), interleukin-6 (IL-6) and interleukin-10 (IL-10) were measured by enzyme-linked immunosorbent assay (ELISA) kits (Proteintech, Beijing, China) according to the manufacturer’s protocols.
Statistical analyses
Statistical analyses were performed using SPSS13.0 software (SPSS Inc., Chicago, IL, USA). Wilcoxon’s test was used to compare the mean value. The results were considered statistically significant when p values were less than .05.
Results
Effect of silibinin on cell viability of RGCs under blue light
Silibinin, which is a flavonoid plant extract from Silybum marianum L (), has been reported to demonstrate a significant effect in different disorders. First, we examined the effect of silibinin on cell viability in RGCs cultured under blue light with silibinin in different conferences for 24 h. The results of MTT assay were used for the detection of the cell viability. The silibinin at the concentration of 5, 25, 50 and 100 μM were chosen to detect its protective effect. In this study, it was found that silibinin less than in 50 μM did not protect the RGCs cellular viability under blue light, while silibinin in 50 and 100 μM demonstrated a significant protective effect under the blue light condition (). In the following experiment, 100 μM of silibinin would be used in the following experiments.
Figure 1. The chemical structure of silibinin and its effects on RGCs in blue light. (A) The chemical structure of silibinin. (B) The cell viability of RGCs in control, blue light and blue light in combination with different concentrations of silibinin. The cell viability was determined by the MTT assay. The bars indicate the SD; n = 6, in terms of independent wells; **p < .001.
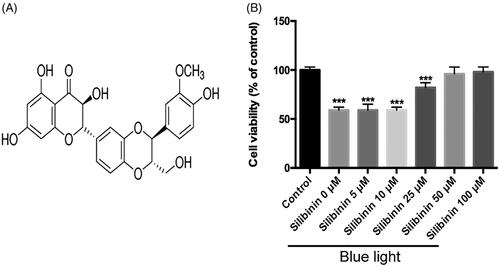
Silibinin regulates apoptosis-related protein expressions in RGCs
Caspase cascade and Bcl2/Bax pathways play important roles in intrinsic apoptotic pathways. When apoptosis is triggered, Bax moves to the mitochondrial outer membrane to promote the opening of the permeability transition (PT) pore. Sequentially, cytochrome C will be released to the cytoplasm and further activates the caspase cascade to induce apoptosis [Citation13]. Here through western blotting, it was demonstrated that silibinin treatment would reduce the expression of Bax, cleaved caspase-3 and cleaved caspase-9, while it up-regulated the expression of Bcl2 (. Since the ratio of Bcl-2 and Bax is important in the apoptosis process, it was found that Bcl2/Bax ratio increased after silibinin treatment. These data suggest that silibinin application would activate an anti-apoptosis effect in blue-light damage RGCs model.
Effect of silibinin on the apoptosis of RGCs induced by blue light damage
To explore the specific role of silibinin in the apoptosis of RGCs after blue light and blue light in combination with silibinin treatment, we detected the apoptotic rate by Annexin-VFITC/PI staining and TUNEL assay, respectively. TUNEL assay showed that less apoptotic RGCs were found in the silibinin treatment group than the blue light treated group (p < .05, ). Through Annexin-VFITC/PI staining, it was found that the apoptotic rate () in the silibinin group were remarkably decreased when compared with those of the blue light group (p < .05). Therefore, we believed that silibinin treatment could significantly decrease the apoptotic rate of RGCs induced by blue light damage.
Figure 3. The protective effect of silibinin on blue light induced apoptosis in RGCs. (A) Representative images of TUNEL-positive cells (red) and total cells (blue). (B) Quantification of TUNEL positive cells, presented as percentages of total cells. The bars indicate the SD; n = 6, in terms of independent wells; **p < .001.
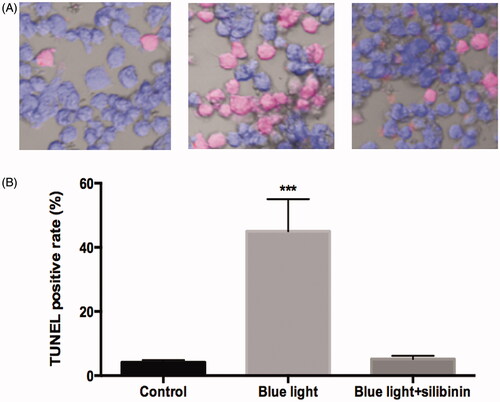
Figure 4. Effects of silibinin on apoptosis rate in blue light induced RGCs. (A) Flow cytometry analysis of apoptosis in cultured podocytes in different groups and quantitation of these results (n = 5). (B) Cell apoptosis detected using flow cytometry. The bars indicate the SD; n = 6, in terms of independent wells; **p < .001.
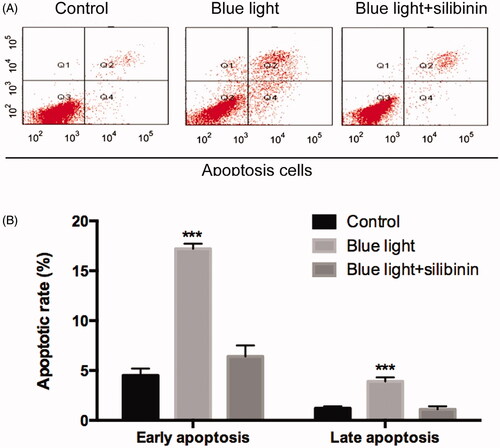
Analysis of the expression of inflammatory factors in RGCs treated with blue light with/without silibinin
There is a well-known association between inflammation and light damage [Citation14], which contributes to the injury and repair of RGCs during damaging conditions. In this study, we focused on the expression of some inflammatory factors in RGCs under blue light damage with/without silibinin. The cell culture medium in different groups was harvested and used for advanced studies. The levels of TNF-α (), IL-1β (), IL-6 () and IL-10 () were measured by ELISA. The level of all these inflammatory factors was significantly different with the control group, while no difference was detected in TNF-α, IL-1β, IL-6 and IL-10 expression level after treatment of silibinin comparing with the control group. The protective effects of silibinin in blue light damaged RGCs involved in different inflammatory factors.
Figure 5. Silibinin protect blue light induced inflammation in RGCs. (A). The expression of TNF-α in each group. (B). The expression of IL-1β in each group. (C). The expression of IL-6 in each group. (D). The expression of IL-10 in each group. The bars indicate the SD; n = 6. *: p < .05, ***: p < .001.
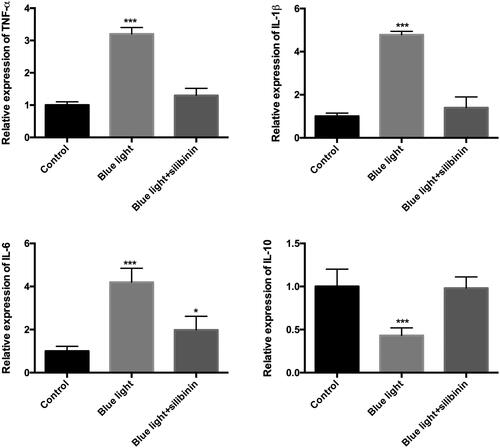
Treatment of silibinin on MEK1/ERK/CREB signalling on blue light damage of RGCs
To investigate whether silibinin protects inflammation and apoptosis through up-regulation of MEK1/ERK/CREB signalling, we detected the expression of pMEK1, ERK1/2 and pCREB in control, blue light and blue light in combination with silibinin groups. It was found that blue light would down-regulate the expression of MEK1/ERK/CREB. After the treatment with silibinin, the expression of pMEK1, ERK1/2 and pCREB were significantly upregulated (). Overall, these results suggest that silibinin protects against blue light induced oxidative damage by overexpression of MEK1/ERK/CREB pathway.
Discussion
Glaucoma is still one of the leading causes of blindness in the whole world. RGCs death is the most important pathologic change in the development of glaucoma. A convenient strategy that is commonly used at the first stage of research in the field of neuroprotection for different ocular pathologies, including glaucoma, is to develop an in-vitro model and it is related events that occur in-vivo. Related to this, the development of neuroprotective approaches capable of preserving the cells after the aggression and the damage generated by blue light is an important advance in the development of long-term treatments for glaucoma.
Apoptosis is an intracellular signalling cascade that causes programmed cell death. This process is necessary for normal growth and development; however, apoptosis also occurs in the major pathologic pathways of numerous diseases, including glaucoma. Apoptosis is triggered through both the intrinsic and extrinsic apoptotic pathways. As glaucoma is usually viewed as a neurodegenerative disease, the resistance against the apoptosis therein emerges as a pivotal strategy to save RGC in terms of glaucoma treatment [Citation15]. There exist two primary apoptotic pathways, the intrinsic pathway and the extrinsic pathway, both of which depend on the activation of the cascade of cysteine proteinases as caspases. In this investigation, to explore whether blue light damages RGCs via apoptosis, we used TUNEL and western-blot for apoptosis-related proteins. TUNEL detects DNA fragmentation by labelling the terminal end of nucleic acids, while the Bcl-2 family proteins regulate the intrinsic pathway of apoptosis by controlling mitochondrial outer membrane permeability. In this result of this study, it was found that blue light significantly increased the protein level of pro-apoptotic proteins (cleaved caspase-3, cleaved caspase-9 and Bax) and reduce the expression anti-apoptotic of Bcl-2. In advanced study, it was found that application of silibinin could significantly protect the RGCs from blue light damage. This result provides us conclusive evidence for the application of silibinin in the management of glaucoma.
Mounting evidence suggests neuroinflammation is a key process in glaucoma and this view has drawn the attentions groups of researchers in this field nowadays [Citation16]. NF-κB induced pro-inflammatory TNF-α, IL-6, and NOS2 expression was up-regulated in a glaucoma animal model and the ketogenic diet resolves energy demand and ameliorates the inflammation by inhibition of AMPK activation that inhibits NLRP3 inflammasome-mediated inflammation [Citation17]. In a recent review, it was reported that inflammation has been reported as common features in clinical and experimental glaucoma [Citation18]. Thus, therapies aimed at modulating the immune and inflammatory responses may represent a promising approach for limiting the optic nerve damage and the loss of retinal ganglion cells associated with glaucoma. In our study, we focus on the effect of silibinin on some pro-inflammatory and anti-inflammatory cytokines. After treatment with silibinin, it was found that the dysregulated expression of TNF-α, IL-1β, IL-6 and IL-10 induced by blue light reached a normal level comparing with control group. The protective effect of silibinin on the RGCs would help in the understanding of its neuroprotection effect and demonstrated its potential therapeutic effects in glaucoma.
Silibinin has been reported to function in different diseases, including cancers, non-alcoholic fatty liver and diabetic nephropathy [Citation19–22]. In the past several years, many signalling molecules related to apoptosis and inflammation have been reported to be neuroprotective and play an important survival effect in the management of glaucoma [Citation23]. In addition, some studies demonstrated that neuroprotective function increases the Bcl-2 level to an anti-apoptotic state and activates autophagy through the induction of silibinin through the Notch pathway [Citation24]. ERK and NF-кB pathways are known to alter the expression of pro-apoptotic and anti-apoptotic genes such as Bax and Bcl-2 by regulating the activity of transcription factors including CREB [Citation25,Citation26]. Therefore, MEK/ERK/CREB pathway is considered to play a critical role in apoptosis, but the regulation effect of silibinin on the MEK/ERK/CREB pathway remains unclear. We also conduct some experiments to detect the potential influenced pathways during the treatment with silibinin. In this study, we found that the MEK/ERK/CREB pathway regulated by silibinin and thus plays an important role in the regulation of apoptosis and inflammation of the RGCs.
Conclusion
In conclusion, the effects of silibinin pretreatment against blue light induced acute RGCs damage was demonstrated, and the underlying mechanism was investigated in this study. The data indicated that direct suppression of apoptosis and inflammation through the activation of MEK/ERK/CREB pathway in vitro. Our study supported that silibinin may be used as a potential candidate for glaucoma treatment.
Disclosure statement
No potential conflict of interest was reported by the authors.
References
- Conlon R, Saheb H, Ahmed II. Glaucoma treatment trends: a review. Can J Ophthalmol. 2017;52(1):114–124.
- Rukmini AV, Milea D, Gooley JJ. Chromatic pupillometry methods for assessing photoreceptor health in retinal and optic nerve diseases. Front Neurol. 2019;10:76.
- Yadav KS, Rajpurohit R, Sharma S. Glaucoma: current treatment and impact of advanced drug delivery systems. Life Sci. 2019;221:362–376.
- Lusthaus J, Goldberg I. Current management of glaucoma. Med J Aust. 2019;210(4):180–187.
- Wang C, Ren YL, Zhai J, et al. Down-regulated LAMA4 inhibits oxidative stress-induced apoptosis of retinal ganglion cells through the MAPK signaling pathway in rats with glaucoma. Cell Cycle. 2019;18(9):932.
- Peng H, Sun YB, Hao JL, et al. Neuroprotective effects of overexpressed microRNA-200a on activation of glaucoma-related retinal glial cells and apoptosis of ganglion cells via downregulating FGF7-mediated MAPK signaling pathway. Cell Signal. 2019;54:179–190.
- Aires ID, Boia R, Rodrigues-Neves AC, et al. Blockade of microglial adenosine A2A receptor suppresses elevated pressure-induced inflammation, oxidative stress, and cell death in retinal cells. Glia. 2019;67(5):896–914.
- Zhu XX, Ding YH, Wu Y, et al. Silibinin: a potential old drug for cancer therapy. Expert Rev Clin Pharmacol. 2016;1–8:1323–1330.
- Federico A, Dallio M, Loguercio C. Silymarin/silybin and chronic liver disease: a marriage of many years. Molecules. 2017;22(2):191.
- Lin CH, Li CH, Liao PL, et al. Silibinin inhibits VEGF secretion and age-related macular degeneration in a hypoxia-dependent manner through the PI-3 kinase/Akt/mTOR pathway. Br J Pharmacol. 2013;168(4):920–931.
- Donadoni ML, Gavezzotti R, Borella F, et al. Experimental diabetic neuropathy. Inhibition of protein mono-ADP-ribosylation prevents reduction of substance P axonal transport. J Pharmacol Exp Ther. 1995;274(1):570–576.
- Becker S, Eastlake K, Jayaram H, et al. Allogeneic transplantation of Muller-derived retinal Ganglion cells improves retinal function in a feline model of Ganglion cell depletion. Stem Cells Transl Med. 2016;5(2):192–205.
- Mallick DJ, Soderquist RS, Bates D, et al. Confounding off-target effects of BH3 mimetics at commonly used concentrations: MIM1, UMI-77, and A-1210477. Cell Death Dis. 2019;10(3):185.
- Li JY, Zhang K, Xu D, et al. Mitochondrial fission is required for blue light-induced apoptosis and mitophagy in retinal neuronal R28 cells. Front Mol Neurosci. 2018;11:432.
- Osborne A, Sanderson J, Martin KR. Neuroprotective effects of human mesenchymal stem cells and platelet-derived growth factor on human retinal Ganglion cells. Stem Cells. 2018;36(1):65–78.
- Williams PA, Marsh-Armstrong N, Howell GR. Neuroinflammation in glaucoma: a new opportunity. Exp Eye Res. 2017;157:20–27.
- Harun-Or-Rashid M, Inman DM. Reduced AMPK activation and increased HCAR activation drive anti-inflammatory response and neuroprotection in glaucoma. J Neuroinflammation. 2018;15(1):313.
- Adornetto A, Russo R, Parisi V. Neuroinflammation as a target for glaucoma therapy. Neural Regen Res. 2019;14(3):391–394.
- Bosch-Barrera J, Queralt B, Menendez JA. Targeting STAT3 with silibinin to improve cancer therapeutics. Cancer Treat Rev. 2017;58:61–69.
- Salomone F, Barbagallo I, Godos J, et al. Silibinin restores NAD(+) levels and induces the SIRT1/AMPK pathway in non-alcoholic fatty liver. Nutrients. 2017;9(10):1086.
- Byun HJ, Darvin P, Kang DY, et al. Silibinin downregulates MMP2 expression via Jak2/STAT3 pathway and inhibits the migration and invasive potential in MDA-MB-231 cells. Oncol Rep. 2017;37(6):3270–3278.
- Liu Y, Ye J, Cao Y, et al. Silibinin ameliorates diabetic nephropathy via improving diabetic condition in the mice. Eur J Pharmacol. 2019;845:24–31.
- Chi W, Chen H, Li F, et al. HMGB1 promotes the activation of NLRP3 and caspase-8 inflammasomes via NF-κB pathway in acute glaucoma. J Neuroinflammation. 2015;12:137.
- Zhang S, Yang Y, Liang Z, et al. Silybin-mediated inhibition of Notch signaling exerts antitumor activity in human hepatocellular carcinoma cells. PLOS One. 2013;8(12):e83699.
- Garcia S, Lopez E, Lopez-Colome AM. Glutamate accelerates RPE cell proliferation through ERK1/2 activation via distinct receptor-specific mechanisms. J Cell Biochem. 2008;104:377–390.
- Gomez M, Manzano A, Figueras A, et al. Sertoli-secreted FGF-2 induces PFKFB4 isozyme expression in mouse spermatogenic cells by activation of the MEK/ERK/CREB pathway. Am J Physiol Endocrinol Metab. 2012;303:E695–E707.