Abstract
Spinal cord injury (SCI) can lead to varying degrees of sensory and motor dysfunction. Salvianolic acid B (Sal-B) is the dominating bioactive constituent of Danshen, which has been reported to alleviate liver fibrosis and exert neuroprotective effects. But, the influence of Sal-B in SCI remains mysterious. The research planned to delve the protective function of Sal-B in hydrogen peroxide (H2O2)-caused PC-12 cell injury. H2O2-caused PC-12 cells injury model was built, CCK-8, Transwell and flow cytometry experiments were enforced to assess cell proliferation, migration and apoptosis. The microRNA (miR)-26a plasmid and the matching control were transfected into PC-12 cells, subsequently, the influence of miR-26a inhibition in H2O2-corrupted PC-12 cells was evaluated. The cell growth-correlated factors and PI3K/AKT and MEK/ERK pathways were assayed through western blot assay. Results corroborated that Sal-B eased H2O2-evoked injury in PC-12 cells. Ascended miR-26a was monitored in Sal-B and H2O2-exposed cells. MiR-26a inhibition annulled the protective action of Sal-B in H2O2-corrupted cells. The protective function of Sal-B was enabled through activating PI3K/AKT and MEK/ERK pathways. These findings delineated that Sal-B protected PC-12 cells against H2O2-caused injury through ascending miR-26a via initiating PI3K/AKT and MEK/ERK pathways.
H2O2 causes PC-12 cell injury;
Sal-B eases H2O2-caused PC-12 cell injury;
Sal-B protects PC-12 cells against H2O2-caused injury via elevating miR-26a;
Sal-B activates AKT and MEK/ERK pathways via modulating miR-26a.
Highlights
Introduction
Spinal cord injury (SCI) is characterized by an extensive series of functional deficits, which can lead to varying degrees of sensory and motor dysfunction [Citation1]. The predominant causes of SCI comprise motor vehicle accidents, falls, sports-correlated accidents, shot or knife injuries [Citation2,Citation3]. SCI injury can be split into three phases: the acute, secondary and chronic injury processes [Citation4]. Currently, drug and surgical treatments are the main therapeutic strategies for SCI [Citation5], nevertheless, the side effects of these treatments are inevitable. In various researches, local oxidative damage has been reported to occur after SCI [Citation6,Citation7]. It has been discovered that accelerations of reactive oxygen species (ROS) and oxidative stress are deemed to the paramount events in SCI [Citation8]. Moreover, a substantial amount of studies suggested that ROS could destroy lipid membranes and proteins, and ultimately lead to the injury or death of neuronal cells [Citation9,Citation10]. Therefore, to delve into the molecular mechanism of oxidative damage in SCI has aroused our extensive interest.
Danshen (Salvia miltiorrhiza Bunge) is a well-known traditional Chinese medicine (TCM), which has been diffusely applied for curing cerebrovascular and cardiovascular illnesses [Citation11,Citation12]. Salvianolic acid B (Sal-B) is the dominating bioactive component of Danshen, which has the paramount pharmacological activities, encompassing anti-tumour, anti-oxidant, anti-apoptosis and cardiovascular and cerebrovascular protection [Citation13–15]. Accumulating evidence from in vivo experiments has testified the protective function of Sal B in SCI [Citation16,Citation17]. One study from Tang and Zhang uncovered the protective activity of Sal-B in amyloid beta (Aβ) peptide-evoked impairment in PC-12 cells [Citation18]. Nonetheless, whether Sal-B can protect PC-12 cells against hydrogen peroxide (H2O2)-caused cell injury has not been fully delved.
Herein, we planned to delve the protective activity of Sal-B in H2O2-evoked PC-12 cell impairments. The abilities of PC-12 cells viability, migration and apoptosis were evaluated in the actual study. Additionally, we also probed the regulatory functions of microRNA-26a (miR-26a) in H2O2-corrupted PC-12 cells. The latent mechanism was uncovered by PI3K/AKT and MEK/ERK pathways. The research might emerge a unique avenue for remedying SCI.
Materials and methods
Cell culture and management
PC-12 cells (ATCC® CRL-1721™) were achieved from American Type Culture Collection (ATCC, Rockville, MD). The RPMI-1640 medium with 10% horse serum and 5% foetal bovine serum (FBS) was applied for cell cultivation. PC-12 cells were subsequently transferred into an incubator with constant 37 °C comprising 5% CO2 and 95% air. For H2O2 manipulation, PC-12 cells were exposed to fresh medium with 200 µM H2O2 for 24 h to establish cell injury model. Sal-B was purchased from Paipai Limited Technology Corporation (Guangzhou, China). To treat cells with Sal-B, PC-12 cells were managed with diverse doses of Sal-B (0, 1, 5 and 10 μL) for 1–7 d in the next correlated experiments.
Quantitative reverse transcription-PCR (qRT-PCR)
Total RNA was elicited from PC-12 cells with H2O2 and Sal-B manipulations through the application of Trizol reagent (Life Technologies, Frederick, MD, USA). The Taqman miR Reverse Transcription Kit and Taqman Universal Master Mix II (ABI, Foster City, CAUSA) were implemented for evaluation of miR-26a expression in PC-12 cells. U6 was elected for standardization of miR-26a expression and the interrelated data were reckoned through exploiting the 2−ΔΔCT method.
Transfection
The miRNA expression plasmids of miR-26a inhibitor and the correlated control (NC) were compounded by Life Technologies Corporation (Frederick, MD, USA). The above-involved expression plasmids were utilized for PC-12 cells transfection through exploiting Lipofectamine 3000 (Invitrogen, Carlsbad, CA, USA) conforming to its explanatory memorandum. We collected the transfected cells after 48 h transfection and utilized these cells in the follow-up experiments.
Cell viability assay
After manipulations with H2O2 and Sal-B, PC-12 cell viability was carried out through the application of Cell Counting Kit-8 (CCK-8; Sigma, St Louis, MO, USA) assay. The stimulated cells were gathered and were fostered in an incubator covering 5% CO2 for overnight at 37 °C. Afterward, the CCK-8 solution (10 μL) was employed to co-foster with above cells for supererogatory 1 h at 37 °C. Microplate Reader apparatus (Bio-Rad, Hercules, CA, USA) was implemented for the assessment of the absorbance at 450 nm. For cell number kinetics in one week, 1 × 105 PC-12 cells were inoculated in 60 mm dishes. After manipulations, above-managed cells were rinsed and live cell numbers were evaluated through employing trypan blue exclusion.
Apoptosis analysis by flow cytometry
PC-12 cells were gathered and washed in PBS. After washing, the managed cells were re-suspended in 400 μL 1× binding buffer. Afterward, 5 μL PI/FITC-Annexin V along with 50 μg/mL RNase A (Sigma, St Louis, MO, USA) was utilized to stain PC-12 cells for 1 h, at an indoor temperature in the darkness. In the end, the flow cytometry (FACSAria II; BD Biosciences, San Jose, CA, USA) was implemented for cell apoptosis determination.
Migration assay
Transwell assay was carried for the determination the activity of cell migration in PC-12 cells after H2O2 and Sal-B manipulations. The managed PC-12 cells were suspended in 200 μL serum-free medium and were replenished into the upper chamber. In the meantime, 600 μL complete medium was replenished into the lower compartment. After cultivation in a CO2 incubator, the non-migrated cells on the upper surface of the filter were cleaned out utilizing a drippy cotton swab. After this, the migrated cells were fastened with methyl alcohol and dyed with 0.5% crystal violet (Sigma, St Louis, MO, USA) for 20 min, and then were counted adopting inverted microscope (Olympus, Tokyo, Japan).
Western blot assay
For the extraction of total protein from PC-12 cells after H2O2 and Sal-B manipulations, RIPA lysis buffer (Beyotime, Shanghai, China) with protease inhibitor (Roche, San Francisco, CA, USA) was adopted. The commonly-utilized BCA™ Protein Assay Kit (Beyotime, Shanghai, China) was carried out for determination of the extracted protein concentrations.
Subsequently, the proteins were subjected to sodium dodecyl sulphate-polyacrylamide gel electrophoresis (SDS-PAGE) for electrophoretic separation and shifted to the nitrocellulose membranes (Millipore, Billerica, MA, USA) via implementing the semi-dry blotter method. The nitrocellulose membranes were sealed with 5% bovine serum albumin (BSA) and were co-fostered with the correlated primary antibodies all night at 37 °C. Subsequently, the suitable secondary antibody was exploited to co-cultivate with the nitrocellulose membranes for extra 1 h, at indoor temperature. The enhanced chemiluminescence (ECL) reagent (Pierce Biotechnology, Rockford, IL, USA) was executed for visualization of the bands and the signals were scanned via adopting the Image Lab™ Software (Bio-Rad, Hercules, CA, USA).
Statistical analysis
The outcomes in the current research were exhibited as the mean ± SD. For analysis, the statistical data, SPSS version 19.0 statistical software (IBM, Armonk, NY, USA) was carried out. The p values in two or multiple groups were computed by Student t-test and ANOVA with Tukey’s multiple comparisons test. The p values less than .05 was deemed to be a significant difference consequence.
Results
H2O2 caused PC-12 cell injury
After manipulation with H2O2, the abilities of PC-12 cell viability, migration and apoptosis were detected. In , the prohibitions of cell viability (p < .05) and migration (p < .01) of PC-12 triggered by H2O2 were emerged. However, the acceleration of apoptosis of PC-12 (p < .05) evoked by H2O2 was presented in . Further, the pro-apoptotic factor (Bax), cleaved-caspase-3 and cleaved-caspase-9 were exalted by H2O2 manipulation in PC-12 cells (p < .001, . But, the anti-apoptotic factor (Bcl-2) was restrained by H2O2 manipulation in PC-12 cells (p < .05, . Data hinted that H2O2 could cause PC-12 cell impairments.
Figure 1. H2O2 caused PC-12 cell injury. (a) Roles of H2O2 in cell viability by CCK-8 assay. (b) Roles of H2O2 in cell migration by Transwell assay. (c) Roles of H2O2 in cell apoptosis by flow cytometry assay. (d) Roles of H2O2 in Bcl-2, Bax and pro/cleaved-caspase-3/-9 by Western blot assay. Results were acquired from three independent experiments and were emerged as the means ± SD. *p<.05, **p<.01, ***p<.001.
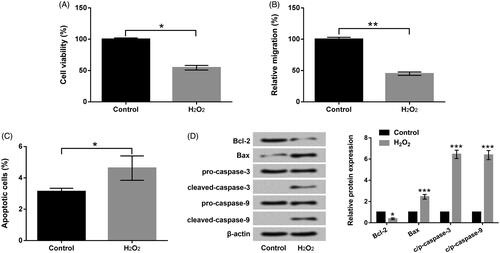
Sal-B protected PC-12 cells against H2O2-caused injury
The consequences in exhibited that Sal-B accelerated PC-12 cell viability, migration and restrained cell apoptosis in a concentration-dependent manner under H2O2 manipulation (p < .05 or .01). Moreover, the protected action of Sal-B enhanced along with the increase of concentrations. The potency of 5 and 10 μM Sal-B was better than the group of 1 μM Sal-B, which were close to control. Thus, we elected 5 μM Sal-B to deal with PC-12 cells in the follow-up experiments. Western blot assay uncovered the declinations of PCNA, Cyclin A, Cyclin E1, CDK2, Cyclin D1 and CDK4 (p < .001) in H2O2-managed PC-12 cells (. Nonetheless, the repressive function of H2O2 in these proteins was obviously annulled by Sal-B manipulation (p < .001, . Besides, influences of H2O2 in Bcl-2, Bax, and cleaved-caspase-3/-9 were also inverted by Sal-B (p < .05 or .001, . The above discoveries delineated that Sal-B could protected PC-12 cells against H2O2-evoked impairment.
Figure 2. Sal-B protected PC-12 cells resisting H2O2-triggered injury. (a,b) Roles of Sal-B in cell viability by CCK-8 assay. (c) Roles of Sal-B in cell migration by Transwell assay. (d) Roles of Sal-B in cell apoptosis by flow cytometry assay. (e) Roles of Sal-B in Cyclin A, Cyclin E1, Cyclin D1, CDK2 and CDK4 by Western blot assay. (f) Roles of Sal-B in Bcl-2, Bax and pro/cleaved-caspase-3/-9 by Western blot assay. Results were acquired from three independent experiments and were emerged as the means ± SD. *p < .05, **p < .01, ***p < .001.
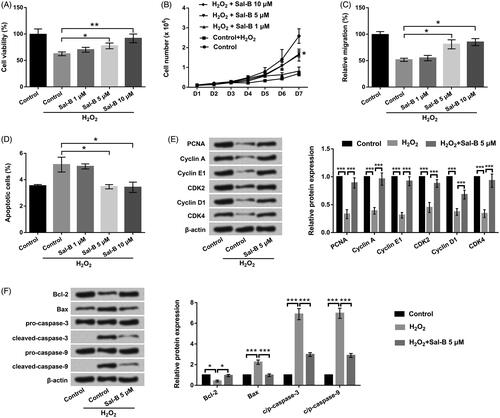
Sal-B ascended miR-26a expression in H2O2-corrupted PC-12 cells
The influence of Sal-B in miR-26a expression was appraised through the application of qRT-PCR. displayed that repressed miR-26a (p < .05) was discovered in the manipulation group of H2O2. But, after managing cells with Sal-B, miR-26a expression was evidently enhanced in a concentration-dependent manner (p < .05). These outcomes testified that miR-26a expression was elevated by Sal-B under H2O2 manipulation, illustrating that miR-26a might join in adjusting H2O2-caused impairment in PC-12 cells.
Sal-B eased H2O2-caused PC-12 cell injury through enhancement of miR-26a
The transfection efficiency revealed in , results displayed that miR-26a expression was distinctly dropped off after miR-26a inhibitor transfection (p < .01). The observations from CCK-8, Transwell and flow cytometry assays uncovered that repressed miR-26a attenuated the accelerative functions of Sal-B in cell viability (p < .01) and migration (p < .05), as well as the suppressive functions of Sal-B in cell apoptosis (p < .05) in H2O2-stimulated PC-12 cells (). Further, the impacts of Sal-B on cell proliferation-associated factors (PCNA, Cyclin A, Cyclin E1, CDK2, Cyclin D1 and CDK4) and apoptosis-associated factors (Bcl-2, Bax and cleaved-caspase-3/-9) were both notably eliminated by suppressed miR-26a (p < .001, . These outcomes manifested that Sal-B flattened H2O2-triggered impairment in PC-12 cells through elevation of miR-26a.
Figure 4. Sal-B protected PC-12 cells resisting H2O2-triggered injury by elevation of miR-26a. (a) The expression of miR-26a in miR-26a inhibitor and NC-transfected PC-12 cells. (b) Roles of miR-26a inhibition in cell viability in Sal-B and H2O2 co-managed PC-12 cells by CCK-8 assay. (c) Roles of miR-26a inhibition in cell migration in Sal-B and H2O2 co-managed PC-12 cells by Transwell assay. (d) Roles of miR-26a inhibition in cell apoptosis in Sal-B and H2O2 co-managed PC-12 cells by flow cytometry assay. (e) Roles of miR-26a inhibition in Cyclin A, Cyclin E1, Cyclin D1, CDK2 and CDK4 in Sal-B and H2O2 co-managed PC-12 cells by Western blot assay. (f) Roles of miR-26a inhibition in Bax, Bcl-2 and pro/cleaved-caspase-3/-9 in Sal-B and H2O2 co-managed PC-12 cells by Western blot assay. Results were acquired from three independent experiments and were emerged as the means ± SD. *p < .05, **p < .01, ***p < .001.
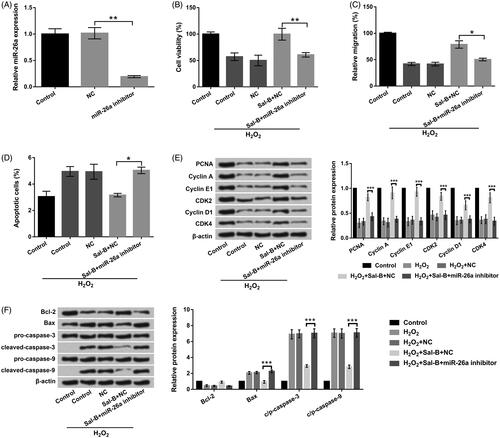
Sal-B triggered PI3K/AKT and MEK/ERK activations through ascending miR-26a
Finally, western blot experiment was executed to assess the roles of Sal-B in the dominating components of PI3K/AKT and MEK/ERK pathways. Results emerged that Sal-B ascended the phosphorylation of PI3K, AKT and GSK3β expression (p < .001) in H2O2-managed PC-12 cells (. Similarly, Sal-B also enhanced the phosphorylation of ERK and MEK expression (p < .001) in H2O2-corrupted PC-12 cells (. Nonetheless, the accelerative functions of Sal-B in PI3K/AKT and MEK/ERK pathways were all annulled by declination of miR-26a in H2O2-corrupted PC-12 cells (p < .001, . These data announced that Sal-B evoked the activations of PI3K/AKT and MEK/ERK pathways through adjusting miR-26a.
Figure 5. Sal-B evoked the activations of PI3K/AKT and MEK/ERK pathways by enhancing miR-26a. (a) Roles of Sal-B and miR-26a inhibition in PI3K/AKT pathway in H2O2-managed PC-12 cells by Western blot assay. (b) Roles of Sal-B and miR-26a inhibition in MEK/ERK pathway in H2O2-managed PC-12 cells by Western blot assay. Results were acquired from three independent experiments and were emerged as the means ± SD. ***p < .001.
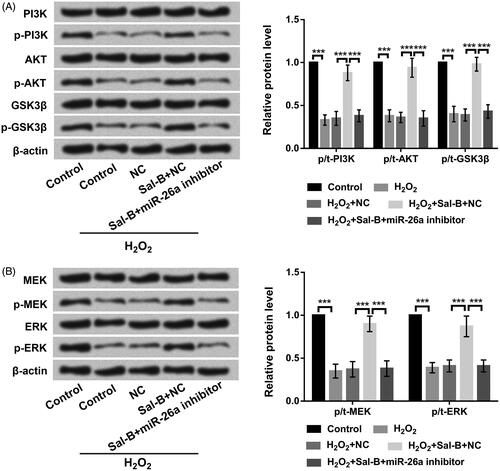
Discussion
Increasing evidence discovered that oxidative stress can provoke the injury of neurocytes under acute pathological conditions [Citation19]. H2O2 is a representative ROS, which can induce oxidative stress, and has been diffusely exploited to construct cell injury model in a large number of studies [Citation20,Citation21]. PC-12 cells are extensively utilized to study neurogenic diseases [Citation22,Citation23]. In our study, we constructed an H2O2-triggered cell injury model and delved the protective functions of Sal-B in H2O2-corrupted PC-12 cells. We observed in the actual research that Sal-B protected PC-12 cells withstand H2O2-triggered cell injury through adjusting cell proliferation, migration and apoptosis. Additionally, the enhancement of miR-26a was discovered in Sal-B-managed PC-12 cells, and the protective activity of Sal-B in H2O2-corrupted PC-12 cells was annulled by miR-26a suppression. In the end, we found that Sal-B evoked PI3K/AKT and MEK/ERK activations through modulating miR-26a in H2O2-corrupted PC-12 cells.
With the advantages of lower toxicity and higher safety, natural anti-oxidants have been diffusely utilized to protect cells resisting oxidative stress injury [Citation24]. Sal-B is a hydrophilic caffeic acid derivative, which has been proven to exert anti-oxidative properties [Citation25,Citation26]. Evidence from Liu et al. testified that Sal-B emerged the neuroprotective functions in PC-12 cells resisting H2O2 toxicity [Citation27]. Herein, CCK-8 and Transwell assays revealed that Sal-B accelerated PC-12 cell viability and migration in H2O2-corrupted PC-12 cells. Simultaneously, flow cytometry illustrated that Sal-B impeded cell apoptosis in H2O2-corrupted PC-12 cells. The correlative data corroborated that Sal-B protected PC-12 cells withstanding H2O2-triggered injury in PC-12 cells. Partly of these explorations were consistent with the research from Liu et al. [Citation27].
Dysregulated miRNAs have been announced to pertinent to multitudinous ailments [Citation28,Citation29]. Previous study corroborated that miRNAs play critical roles in various ailments which are linked to oxidative damage [Citation30]. MiR-26a is a crucial miRNA, which has been reported in multitudinous cancers [Citation31,Citation32]. Study from Peng et al. disclosed the protective action of miR-26a in vascular smooth muscle cells resisting H2O2-triggered impairment via adjusting PTEN/AKT/mTOR pathway [Citation33]. Nonetheless, whether miR-26a takes part in adjusting the protective action of Sal-B in H2O2-corrupted PC-12 cells is still unannounced. Our consequences manifested that H2O2 manipulation cut down miR-26a expression, while Sal-B manipulation ascended miR-26a expression in H2O2-corrupted PC-12 cells. To further delve the functions of miR-26a, miR-26a inhibitor plasmid was exploited for PC-12 cell transfection. The results suggested that repression of miR-26a eliminated the protective action of Sal-B in PC-12 cells resisting the impairment triggered by H2O2. The investigations implied that miR-26a might be a paramount regulator in modulating the protective activity of Sal-B in H2O2-corrupted PC-12 cells.
The mitochondria-dependent apoptotic pathway has been disclosed to join in H2O2-triggered cytotoxicity in PC-12 cells [Citation34]. It has been testified that PI3K/AKT and MEK/ERK pathways are the dominating pro-survival pathways within the cells [Citation35]. Study from Ma et al. corroborated that erythropoietin protected PC-12 cells from β-amyloid 25–35-evoked apoptosis through adjusting PI3K/AKT pathway [Citation36]. Furthermore, ERK/MEK signalling has been declared to evoke neuritogenesis in PC-12 cells [Citation37]. Herein, we studied the influence of Sal-B in PI3K/AKT and MEK/ERK pathways under H2O2 manipulation. We discovered that Sal-B triggered the activations of PI3K/AKT and ERK/MEK in H2O2-corrupted PC-12 cells by adjusting miR-26a expression. The observations hinted the involvements of PI3K/AKT and ERK/MEK pathways in regulating the protective action of Sal-B in H2O2-corrupted PC-12 cells.
Conclusions
This research delineated that Sal-B weakened H2O2-triggered injury in PC-12 cells by adjusting miR-26a via evoking PI3K/AKT and ERK/MEK activations. These explorations suggested that Sal-B might be applied as a neoteric strategy for the remedy of SCI. Nevertheless, the in vivo evidence is scarce in this research for further confirmation the influence of Sal-B in SCI. Further, clinical trials are difficult to conduct due to practical limitations. The above limitations of this research will be probed in the future.
Author contributions
Mingfu Fan conceived and designed the experiments, Niansheng Liu performed the experiments and analyzed the data, and Niansheng Liu and Mingfu Fan wrote the manuscript.
Acknowledgements
This research received no specific grant from any funding agency in the public, commercial or not-for-profit sectors.
Disclosure statement
The authors declare no conflict of interest.
References
- Hulsebosch CE. Recent advances in pathophysiology and treatment of spinal cord injury. Adv Physiol Educ. 2002;26(4):238–255.
- Dietrich WD, Cappuccino A, Cappuccino H. Systemic hypothermia for the treatment of acute cervical spinal cord injury in sports. Curr Sports Med Rep. 2011;10(1):50–54.
- Ambrozaitis KV, Kontautas E, Spakauskas B, et al. Pathophysiology of acute spinal cord injury. Medicina (Kaunas). 2006;42(3):255–261.
- Hachem LD, Ahuja CS, Fehlings MG. Assessment and management of acute spinal cord injury: from point of injury to rehabilitation. J Spinal Cord Med. 2017;40(6):665–675.
- Song Z, Wang Z, Shen J, et al. Nerve growth factor delivery by ultrasound-mediated nanobubble destruction as a treatment for acute spinal cord injury in rats. Int J Nanomedicine. 2017;12:1717–1729.
- Yoon MY, Hwang JH, Park JH, et al. Neuroprotective effects of SG-168 against oxidative stress-induced apoptosis in PC12 cells. J Med Food. 2011;14(1–2):120–127.
- Guo Y, Niu S. MiR-25 protects PC-12 cells from H2O2 mediated oxidative damage via WNT/beta-catenin pathway. J Spinal Cord Med. 2018;41(4):416–425.
- Li R, Yin F, Guo YY, et al. Knockdown of ANRIL aggravates H2O2-induced injury in PC-12 cells by targeting microRNA-125a. Biomed Pharmacother. 2017;92:952–961.
- Wee HY, Lim SW, Chio CC, et al. Hyperbaric oxygen effects on neuronal apoptosis associations in a traumatic brain injury rat model. J Surg Res. 2015;197(2):382–389.
- Chaturvedi RK, Flint Beal M. Mitochondrial diseases of the brain. Free Radic Biol Med. 2013;63:1–29.
- Cheng TO. Danshen: what every cardiologist should know about this Chinese herbal drug. Int J Cardiol. 2006;110(3):411–412.
- Fish JM, Welchons DR, Kim YS, et al. Dimethyl lithospermate B, an extract of Danshen, suppresses arrhythmogenesis associated with the Brugada syndrome. Circulation. 2006;113(11):1393–1400.
- Lin YH, Liu AH, Wu HL, et al. Salvianolic acid B, an antioxidant from Salvia miltiorrhiza, prevents Abeta(25-35)-induced reduction in BPRP in PC12 cells. Biochem Biophys Res Commun. 2006;348(2):593–599.
- Joe Y, Zheng M, Kim HJ, et al. Salvianolic acid B exerts vasoprotective effects through the modulation of heme oxygenase-1 and arginase activities. J Pharmacol Exp Ther. 2012;341(3):850–858.
- Watzke A, O’Malley SJ, Bergman RG, et al. Reassignment of the configuration of salvianolic acid B and establishment of its identity with lithospermic acid B. J Nat Prod. 2006;69(8):1231–1233.
- Fan ZK, Lv G, Wang YF, et al. The protective effect of salvianolic acid B on blood-spinal cord barrier after compression spinal cord injury in rats. J Mol Neurosci. 2013;51(3):986–993.
- Xun C, Hu Y, Lu M, et al. Study of effect of salvianolic acid B on motor function recovery in rats with spinal cord injury. BioMed Res Int. 2014;2014:1–6.
- Tang M, Zhang J. Prostate apoptosis response-4 involved in the protective effect of salvianolic acid B against amyloid beta peptide-induced damage in PC12 cells. Jpn J Pharmacol. 2002;88(4):422–427.
- Thanan R, Oikawa S, Hiraku Y, et al. Oxidative stress and its significant roles in neurodegenerative diseases and cancer. Int J Mol Sci. 2014;16(1):193–217.
- Jin MM, Zhang L, Yu HX, et al. Protective effect of whey protein hydrolysates on H(2)O(2)-induced PC12 cells oxidative stress via a mitochondria-mediated pathway. Food Chem. 2013;141(2):847–852.
- Incani A, Deiana M, Corona G, et al. Involvement of ERK, Akt and JNK signalling in H2O2-induced cell injury and protection by hydroxytyrosol and its metabolite homovanillic alcohol. Mol Nutr Food Res. 2010;54(6):788–796.
- Hirose M, Takatori M, Kuroda Y, et al. Effect of synthetic cell-penetrating peptides on TrkA activity in PC12 cells. J Pharmacol Sci. 2008;106(1):107–113.
- Koh SH, Kwon H, Park KH, et al. Protective effect of diallyl disulfide on oxidative stress-injured neuronally differentiated PC12 cells. Brain Res Mol Brain Res. 2005;133(2):176–186.
- Kannan K, Jain SK. Oxidative stress and apoptosis. Pathophysiology. 2000;7(3):153–163.
- Zhao DH, Wu YJ, Liu ST, et al. Salvianolic acid B attenuates lipopolysaccharide-induced acute lung injury in rats through inhibition of apoptosis, oxidative stress and inflammation. Exp Ther Med. 2017;14(1):759–764.
- Zhu Z, Ding L, Qiu WF, et al. Salvianolic acid B protects the myelin sheath around injured spinal cord axons. Neural Regen Res. 2016;11(3):487–492.
- Liu CS, Chen NH, Zhang JT. Protection of PC12 cells from hydrogen peroxide-induced cytotoxicity by salvianolic acid B, a new compound isolated from Radix Salviae miltiorrhizae. Phytomedicine. 2007;14(7–8):492–497.
- Honardoost MA, Naghavian R, Ahmadinejad F, et al. Integrative computational mRNA-miRNA interaction analyses of the autoimmune-deregulated miRNAs and well-known Th17 differentiation regulators: an attempt to discover new potential miRNAs involved in Th17 differentiation. Gene. 2015;572(2):153–162.
- Xie JX, Fan X, Drummond CA, et al. MicroRNA profiling in kidney disease: plasma versus plasma-derived exosomes. Gene. 2017;627:1–8.
- Cardin R, Bortolami M, Piciocchi M, et al. Hepatocellular carcinoma (HCC) and hepatitis viruses: oxidative damage correlate with cell immortalization and miRNA expression. Digest Liver Dis. 2009;41(5):A1–A1.
- Kota J, Chivukula RR, O’Donnell KA, et al. Therapeutic microRNA delivery suppresses tumorigenesis in a murine liver cancer model. Cell. 2009;137(6):1005–1017.
- Lu J, He ML, Wang L, et al. MiR-26a inhibits cell growth and tumorigenesis of nasopharyngeal carcinoma through repression of EZH2. Cancer Res. 2011;71(1):225–233.
- Peng J, He X, Zhang L, et al. MicroRNA-26a protects vascular smooth muscle cells against H2O2-induced injury through activation of the PTEN/AKT/mTOR pathway. Int J Mol Med. 2018;42(3):1367–1378.
- Chen B, Yue R, Yang Y, et al. Protective effects of (E)-2-(1-hydroxyl-4-oxocyclohexyl) ethyl caffeine against hydrogen peroxide-induced injury in PC12 cells. Neurochem Res. 2015;40(3):531–541.
- Maddika S, Ande SR, Panigrahi S, et al. Cell survival, cell death and cell cycle pathways are interconnected: implications for cancer therapy. Drug Resist Updates. 2007;10(1–2):13–29.
- Ma R, Xiong N, Huang C, et al. Erythropoietin protects PC12 cells from beta-amyloid(25-35)-induced apoptosis via PI3K/Akt signaling pathway. Neuropharmacology. 2009;56(6–7):1027–1034.
- Kudo TA, Kanetaka H, Shimizu Y, et al. Induction of neuritogenesis in PC12 cells by a pulsed electromagnetic field via MEK-ERK1/2 signaling. Cell Struct Funct. 2013;38(1):15–20.