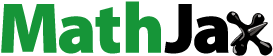
Abstract
This paper aims to demonstrate the efficacy of the immobilisation of the chemotherapy drug doxorubicin on nanodiamond platforms as a potential cancer therapy. This effective drug is experimentally fed into a human breast adenocarcinoma cell lines. Drug loading activity and cell viability are detected by spectrometer, microscopy, and MTT assay in this study at Biomedical Physics Research Unit, Department of Physics, Faculty of Science, King Mongkut’s Institute of Technology Ladkrabang, Bangkok 10520, Thailand between 1 Oct 2018 and 10 Jun 2019. Experimental results show that in the basic environment (pH = 8.0), the nanodiamond carboxylic group cooperated with the doxorubicin amino group to form a stable and non-covalent bond on nanodiamond surfaces served as a simple physical adsorption. In an acidic environment suitable to targeting the cancer cells, the nanodiamond carboxylic group ionised so that doxorubicin is effectively released. Doxorubicin therefore affirmatively absorbed into the cytoplasm and later into the nucleus. The significant finding of the study is that IC-50 equivalent to 0.40 mg/mL and viable nanodiamond–doxorubicin is a good candidate material for drug delivery.
Keywords:
Introduction
Since breast cancer is the second deadliest cancer among women in the United States as of 2017, the main task in breast cancer treatment research is to maximize chemotherapeutic effects [Citation1]. Although the standard treatment including chemotherapy is often effective to kill the breast cancer cells, only a little progress has been accomplished in clinical applications [Citation2]. So far, the use of nanoparticle-based drug carriers is rapidly progressing to overcome the troubles of traditional chemotherapy. Several particulate drug nanocarriers are being widely studied especially for tumour-targeting therapy [Citation3,Citation4]. Among these materials, nanodiamonds have the potential to gain prominence in clinical use as drug delivery systems due to the following considerations [Citation5,Citation6]. First, nanodiamonds have an extraordinarily large surface-area-to-volume ratio, which promises to increase performance in drug loading criterion. Second, nanodiamonds offer low toxicity for healthy breast cells. Finally, nanodiamonds are biocompatible at the genetic level. However, a few researchers have investigated the cooperation of nanodiamonds for the tumour-targeting activity. These nanodiamonds are expected to form a cluster structure with water-insoluble drugs in addition to water-soluble drugs. Appropriately functionalized nanodiamonds promise not only to serve as the drug storage mechanism, but also to implement a long distribution time in breast cancer cells.
In the interim, improved drug refusal in breast cancer is a general constraint with doxorubicin-based chemotherapy [Citation7]. Such chemoresistance occurs due to the following reasons: (1) the hydrophilic molecule from doxorubicin restricts transportation over the cellular membrane, resulting in minimal drug internalization; (2) circulation inside the breast cancer tissues suffers in the absence of tumour-targeting ability from doxorubicin; (3) doxorubicin causes severe and undesirable responses like myelosuppression and cardiotoxicity [Citation8,Citation9]; (4) overexpression from membrane transporters actively pumps doxorubicin out of the cell. Hence, doxorubicin coupled with a nanoparticle-based drug delivery agent might eliminate this mode of efflux when they are taken up by the cells efficiently via endocytosis. For instance, nanodiamonds’ incorporation with doxorubicin might be beaten by chemoresistance, hence shortening the demand for larger doses and thus shortening their ill effects against healthy cells. An extensive group of studies supposes that tumours are acidic in nature. Kato et al. [Citation10] have shown that the extracellular pH of cancer cells is acidic partly because of a generation of lactic acid, although they mostly preserve a normal intracellular pH [Citation10]. Som et al. [Citation11] have reported that cancer cells boost the acidic environment intracellularly. Doxorubicin-loaded nanoparticles are also possible to target cancer cells under intracellular acidic conditions [Citation11].
The studies about nanodiamond as a chemo drug carrier platform have been reported by many groups in the past decade. For instance, Li et al. [Citation12] informed that nanodiamond–doxorubicin system has been studied for delivering the drug inside HeLa cells via endocytosis, whereas the uptake of doxorubicin occurred through a passive diffusion confirmed by fluorescence microscopy [Citation12]. The diameter of nanodiamond was about 140 nm. The efficacy of the cell death of doxorubicin release behaviour of nanodiamond–doxorubicin was about four times as compared to doxorubicin alone. Salaam et al. [Citation13] reported that nanodiamond–DGEA peptide–doxorubicin system has been studied for delivering the drug inside prostate cancer confirmed by a transmission electron microscopy [Citation13]. The efficacy of the cell death of nanodiamond–DGEA peptide–doxorubicin releases was about four times as compared to doxorubicin alone. The diameter of nanodiamond was about 35 nm. However, the cellular mechanisms have not been confirmed. Man et al. revealed that doxorubicin-nanodiamond system has been studied for delivering the drug inside liver and mammary cancers confirmed by fluorescence microscopy [Citation14].
The novelty of the current reported studies as compared to the previously reported works is that there is no report a test on breast cancer inhibition based on doxorubicin–nanodiamond complex for delivering the drug inside. The diameter of nanodiamond in the present study is about 5–10 nm. Characterization, including the fluorescence polarisation technique, is applied to confirm a molecular interaction between the chemo drug and nanodiamond in place of TEM and FTIR methods. The innovative strategy presented in the current study is the following: nanodiamonds are engineered to control release and binding between drug and nanodiamond by means of electrostatic interactions between nanodiamond surfaces and doxorubicin molecules, which are superior to covalent linkages. It is, therefore, possible to bypass drug efflux mechanisms to increase intracellular drug concentration and consequently enhance drug sensitivity. Doxorubicin interrupts DNA within cell nuclei to eliminate tumours. Doxorubicin is very effective as a chemotherapy treatment since it can be absorbed and released from original nanodiamonds. Doxorubicin-loaded nanodiamonds are investigated via the Uv-Vis spectroscopy; we determine drug loading capacity and efficacy as well as fluorescence polarisation. To evaluate the efficacy of drug release, doxorubicin-loaded nanodiamonds tests are carried out in solution under acidic environment identical to endosomes and lysosomes in the cells. Furthermore, 3-[4,5-dimethylthiazol-2-yl]-2,5-diphenyltetrazolium bromide (MTT) assay implemented on human breast adenocarcinoma cell line reveals that doxorubicin-loaded nanodiamonds result in built-up cytotoxicity. Microscopic methods (i.e. optical microscopy and fluorescence microscopy) are also used to confirm the drug release behaviour. Therefore, doxorubicin-loaded nanodiamonds can provide a promising approach to immigrate and discharge doxorubicin precisely in the cancer cells.
Methods
Material
A human breast adenocarcinoma cell lines (MCF-7 cells) was purchased from ATCC (USA) and maintained in Dulbecco’s Modified Eagle Medium (DMEM) complemented with 10% foetal bovine serum (FBS). The cells were kept in an incubator at a humidified atmosphere, 37 °C and 5% carbon dioxide. Nanodiamonds (10 + 0.5 nm in diameter corresponding to the ideal size used for drug delivery purpose) and doxorubicin were purchased from Sigma-Aldrich (USA). Drug loading activity and cell viability were detected by spectrometer and MTT assay, respectively, in this study at Biomedical Physics Research Unit, Department of Physics, Faculty of Science, King Mongkut’s Institute of Technology Ladkrabang, Bangkok 10520, Thailand between 1 Oct 2018 and 10 Jun 2019. Note that ethics approval and consent to participate is not applicable. Doxorubicin-loaded nanodiamonds are investigated via the Uv-Vis spectroscopy and microscopic methods (i.e. optical microscopy and fluorescence microscopy) as well as MTT colorimetric assay.
Doxorubicin loading onto nanodiamonds
Nanodiamond platforms were pH-adjusted to 8.0 to promote physical adsorption and were subsequently stirred with cationic doxorubicin at a w/w mixing ratio of 5:1. The weight ratio of nanodiamond:doxorubicin was maintained at 5:1 in the preliminary stage of test-tube experiments. After incubation (5 min, 25 °C), the resultant solution was centrifuged (15 min, 5000 rpm) to pellet-bound nanodiamond–doxorubicin complexes. Data about unbound doxorubicin kept in supernatant was consequently collected to calculate the loading capacity and efficacy of doxorubicin bound to nanodiamonds. Since we used the optical absorption wavelength of 495 nm for the determination of doxorubicin in a physiological buffer, determining the standard curve from absorbance measurements at 495 nm wavelength was carried out by serial dilution of doxorubicin at a range of 0–350 μg/mL to obtain the linear fit. The loading capacity and efficacy of doxorubicin were calculated by the following equations:
(1)
(1)
(2)
(2)
Doxorubicin releasing from nanodiamonds
To carry out a feasibility study investigating the potential application of doxorubicin-loaded nanodiamonds in tumour targeting, the pelleted nanodiamond–doxorubicin complexes were resuspended in a physiological buffer by sonication. As shown in , a combination of treatments was applied to different wells of a 24-well plate (2.05 × 105 cells/mL) under pH 6.0 and the wells were incubated at a physiological condition of 37 °C for 24 h. After exposure of doxorubicin-loaded nanodiamonds for 24 h, cell viability was determined by MTT colorimetric assay for a wide range of treatment concentrations, as shown in . The effect of nanodiamond–doxorubicin complexes on MCF-7 cells was determined by calculating IC-50 values over triplicate runs. IC-50 value, also known as half maximal inhibitory concentration, indicates the performance of an antagonistic drug in suppressing biological action. In our case, IC-50 represents the amount of treatment concentration required to create 50% cancer cell death. The cell viability was calculated by the following equation:
(3)
(3)
Table 1. Detailed preparation of the drug delivery system under various treatment conditions.
Results
The binding ratio of 5:1 (w/w) was used for loading activity according to . shows the unique characteristic of nanodiamond–doxorubicin complexes. The solution was found to turn a deep purple after 0.1 mM of sodium hydroxide was filled, conforming to a pH of 8.0. After pelleting nanodiamond–doxorubicin complexes through centrifugation, the supernatant was found to be a light purple, showing the small amount of unbound doxorubicin.
Figure 1. Photos showing the drug delivery scheme from representative samples 1–5. (a) Nanodiamond–doxorubicin solution after the binding condition is adjusted to pH = 8.0. (b) Pelleting of nanodiamond–doxorubicin complexes after the centrifugation. (c) The resuspended solution after supernatant from part (b) is separated and replaced by an identical volume of a physiological buffer (5 min, 5000 rpm). The final colour is orange.

The releasing profile of doxorubicin from nanodiamond surface is confirmed by UV-Vis spectrometer as shown in . Consequently, nanodiamond–doxorubicin complexes are possible to enhance the therapeutic efficacy of doxorubicin in treating MCF-7.
Figure 2. Representative absorbance of 1.0 mg/mL doxorubicin (DOX) before and after adsorption. About 0.2 mg/mL of the added 1.0 mg/mL DOX is remained in the supernatant after facilitating adsorption to nanodiamond–doxorubicin complexes. The absorbance peak of nanodiamond–doxorubicin is found at 495 nm.
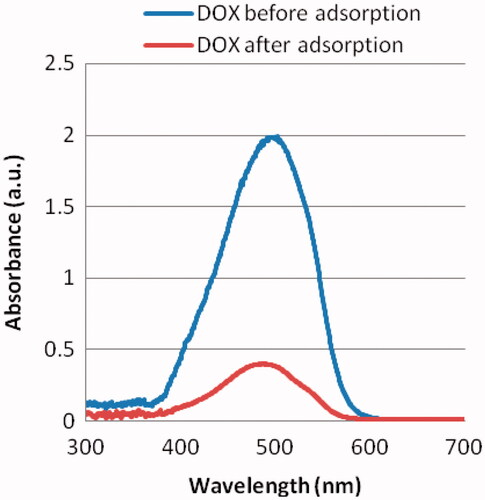
Characterization of the nanodiamond before and after doxorubicin adsorption to nanodiamond–doxorubicin are detected using the fluorescence polarization technique in order to show the uptake mechanism of nanodiamond–doxorubicin complexes. This approach is based on a degree of polarization of a molecule is related to its rotation arising from Brownian dynamics. Using the fluorescence data as shown in , fluorescence polarisation results show that a large fluorescent molecule (i.e. nanodiamond–doxorubicin) excited by a polarised light rotates slower and emits a large degree of polarisation, whereas a small fluorescent molecule (i.e. doxorubicin) applied at the same manner rotates faster and emit a small degree of polarisation as shown in .
Figure 3. Representative fluorescence intensity of 1.0 mg/mL doxorubicin (DOX) before and after adsorption. The fluorescence peak of nanodiamond–doxorubicin is found at 600 nm.
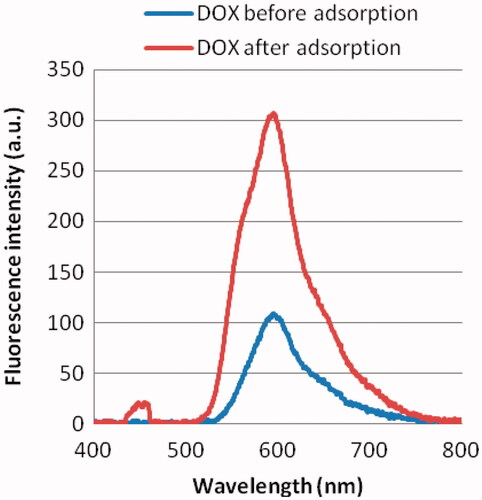
shows the improved loading capacity and efficacy of doxorubicin under various treatment conditions. Nanodiamonds have a unique characteristic surface charge in aqueous solution, with both positively- and negatively-charged facets that promote substantial polar interactions with other highly-charged groups such as water molecules and other nanodiamond particles. Furthermore, nanodiamond surfaces are highly charged and heavily functionalized by carboxylic acid groups. Under the basic condition of this study (pH = 8.0), we found that these groups deprotonate and cooperate with the protonated amine functional group of doxorubicin molecules via electrostatic interactions and hydrogen bonds. H-bond binding energies is about 10–30 kcal/mol [Citation15]. The adsorption mechanism of loading doxorubicin is, therefore, a rapid and uncomplicated approach that results in the reversible nanodiamond–doxorubicin complexes. Individual nanodiamonds are finally covered by additional layers of bound doxorubicin. Note that doxorubicin-loaded nanodiamonds tend to aggregate in MCF-7 cells preferentially through passive diffusion, as the pore of the cellular membrane is 220 + 80 nm in diameter [Citation16].
Discussion
It is imperative not only that fruitful drug delivery vehicles load diversity of drugs, but also they are able to release the treatments in the appropriate biological setting. Acidic circumstances inside endosome and lysosome compartments are suggested to trigger the fast release of doxorubicin from doxorubicin-loaded nanodiamonds by remarkably increasing the cell cytotoxicity [Citation17]. As shown in , most doxorubicin molecules are released from nanodiamond surfaces after 24-h incubation under pH of 6.0. This helps to shorten toxicity of doxorubicin to healthy cells because pH of body fluid is kept about pH 7.4 [Citation18]. Upon entering cells, this doxorubicin is cleaved from nanodiamonds; it subsequently diffuses into cell cytoplasm and enters into the nucleus. As the nuclear pores are sufficiently large to take up smaller particles under 10 nm, they mediate cell death by entering the nucleus and intercalating DNA strands [Citation19]. The small nanodiamonds (less than 10 nm in diameter) are safely moved by the kidney via renal excretion. Optimum efficacy of drug delivery enhanced uptake efficacy under this study’s conditions is expected to be better than that of larger nanodiamonds (i.e. diameter 50–100 nm) because doxorubicin-loaded nanodiamonds are internalized by cancer cells via passive diffusion through the cell membrane. Using fluorescence microscopy, it is confirmed that doxorubicin is cleaved from nanodiamond and passively diffuses into the cell cytoplasm and enters into the nucleus as shown in . Significant toxic effect of nanodiamond–doxorubicin with inhibitory concentration of 50% in MCF-7 is selective to cancer cells because there is a specific cancer-cell targeting motifs functionalized onto the nanodiamond.
Figure 6. Representative fluorescent microscopy images of MCF-7 cells after 12 h exposure (a) and after 24 h exposure (b) to 3.0 mg/mL nanodiamond–doxorubicin conjugates. Green identifies the fluorescence due to Ethidium Bromide staining. All images are shown with 40× magnification.
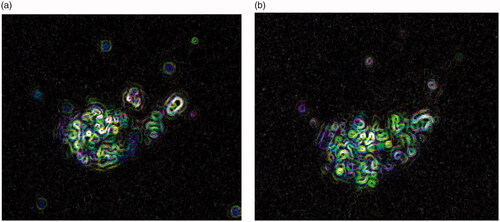
We further evaluate the cytotoxicity of doxorubicin-loaded nanodiamonds in an MCF-7 cell line. presents a cytotoxicity response curve for a wide range of treatment concentrations. The finding is supported with suitable data as a comparison is made with doxorubicin alone and with a normal cell line. The effect is selective to cancer cells. Besides, the cytotoxicity of blank nanodiamonds is examined and we determine that the cell-killing effect is not due to the toxic effect of nanodiamonds, using an optical microscope. The cell-killing effect by nanodiamond alone to the normal cell lines shows its less toxic effect as shown in . In contrast, high toxicity by doxorubicin alone is confirmed in the normal cell lines as shown in . Thus, doxorubicin-loaded nanodiamonds demonstrate increased cytotoxicity. This result may be due to the powerful transport of doxorubicin by nanodiamonds via the passive diffusion mechanism (similar to passive diffusion of free doxorubicin into cancer cells). We found that nanodiamond–doxorubicin complexes are able to introduce a decrease in IC-50 of nanodiamond–doxorubicin treatment. In this case, IC-50 is equivalent to 0.40 mg/mL (see the dashed blue line in . This determination of antiproliferative effects of drugs is considered as a promising material for drug delivery. Nanodiamond–doxorubicin and related complexes show potential for clinical settings. In future work, nanodiamond–doxorubicin complexes should be compared with the drug administered alone and characterized for the loading efficacy.
Figure 7. Cytotoxicity of MCF-7 cells after exposure to a range of treatment concentrations. NDX represents nanodiamond–doxorubicin complexes. Dash line denotes IC-50 value.
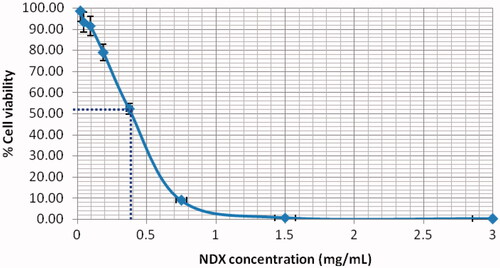
Figure 8. Representative bright-field microscopy images of Vero as the normal cells after 24 h exposure with 5.0 mg/mL nanodiamond alone (a) and 1.0 mg/mL doxorubicin alone (b). The interaction of doxorubicin with Vero was much higher than that of nanodiamond. All images are shown with 40× magnification.
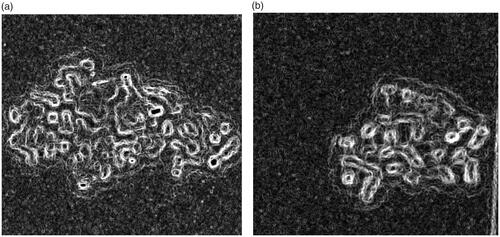
Conclusions
Nanodiamond platforms demonstrate powerful binding characteristics. They are able to load doxorubicin as a chemotherapy treatment against breast cancer cells. The loading of doxorubicin onto nanodiamonds can be properly prepared by varying pH environments and drug loading ratio. In this study, nanodiamond–doxorubicin complexes were extensively exposed to a human breast adenocarcinoma cell lines. The majority of doxorubicin-loaded nanodiamonds are aimed to permeate through the cells and function very well. Efficient uptake of doxorubicin-loaded nanodiamonds and feedback at intracellular acidic pH makes them a representative drug delivery system. In this study, IC-50 amounting to 0.40 mg/mL indicates a small dose for antiproliferative effects. With the data reported, the potential of nanodiamond–doxorubicin and related complexes is promising and is a candidate to enter stages of clinical testing.
Acknowledgements
Author thanks Dr. Suttijit Srivatcharakul, Pattarakorn Kanchitchai and Munthana Changsuph from the Department of Biology, King Mongkut’s Institute of Technology Ladkrabang for their helpful discussion.
Disclosure statement
No potential conflict of interest was reported by the authors.
Data availability
All data used to support the findings of this study are included in the article.
Additional information
Funding
References
- American Cancer Society. Cancer facts & figures. New York (NY): American Cancer Society; 2017.
- DeVita VT, Chu E. A history of cancer chemotherapy. Cancer Res. 2008;68(21):8643–8653.
- Kumari P, Ghosh B, Biswas S. Nanocarriers for cancer-targeted drug delivery. J Drug Targeting. 2015;24(3):1–13.
- Peng X, Rahman MA, Mao H, et al. Nanomaterials facilitate tumor targeting and drug delivery. Austin J Cancer & Clinical Res. 2016;3(2):1072.
- Zhang T, Cui H, Fang CY, et al. Targeted nanodiamonds as phenotype specific photoacoustic contrast agents for breast cancer. Nanomedicine. 2015;10(4):573–587.
- Ho D. Nanodiamonds: applications in biology and nanoscale medicine. Brussel (Belgium): Springer; 2009.
- Poklepovic A, Qu Y, Dickinson M, et al. Randomized study of doxorubicin-based chemotherapy regimens, with and without sildenafil, with analysis of intermediate cardiac markers. Cardio-Oncology. 2018;4(1):7.
- Chen T, Shen HM, Deng ZY, et al. A herbal formula, SYKT, reverses doxorubicin-induced myelosuppression and cardiotoxicity by inhibiting ROS-mediated apoptosis. Mol Med Rep. 2017;15(4):2057–2066.
- Chatterjee K, Zhang J, Honbo N, et al. Doxorubicin cardiomyopathy. Cardiology. 2010;115(2):155–162.
- Kato Y, Ozawa S, Miyamoto C, et al. Acidic extracellular microenvironment and cancer. Cancer Cell Int. 2013;13(1):89.
- Som A, Bloch S, Ippolito JE, et al. Acidic extracellular pH of tumors induces octamer-binding transcription factor 4 expression in murine fibroblasts in vitro and in vivo. Sci Rep. 2016;6(1):27803.
- Li Y, Zhou X, Wang D, et al. Nanodiamond mediated delivery of chemotherapeutic drugs. J Mater Chem. 2011;21(41):16406–16412.
- Salaam AD, Hwang P, McIntosh R, et al. Nanodiamond-DGEA peptide conjugates for enhanced delivery of doxorubicin to prostate cancer. Beilstein J Nanotechnol. 2014;5:937–945.
- Man HB, Ho D. Nanodiamonds as platforms for biology and medicine. J Lab Autom. 2013;18(1):12–18.
- Shimkuna RA, Robinson E, Lam R, et al. Nanodiamond–insulin complexes as pH-dependent protein delivery vehicles. Biomaterials. 2009;30:5720–5728.
- Zhou Y, Kumon RE, Cui J, et al. The size of sonoporation pores on the cell membrane. Ultrasound Med Biol. 2009;35(10):1756–1760.
- Liu Y, Wang W, Yang J, et al. pH-sensitive polymeric micelles triggered drug release for extracellular and intracellular drug targeting delivery. Asian J Pharma Sci. 2013;8(3):159–167.
- Marunaka Y. Roles of interstitial fluid pH in diabetes mellitus: glycolysis and mitochondrial function. World J Diabetes. 2015;6(1):125–135.
- Naim B, Brumfeld V, Kapon R, et al. Passive and facilitated transport in nuclear pore complexes is largely uncoupled. J Biol Chem. 2007;282(6):3881–3888.