Abstract
Brucellosis is one of the most common and important diseases between humans and animals. Herein, we developed a nanovaccine against Brucella melitensis based on oligopolysaccharide (OPS) antigen and PLGA nanoparticles. The conjugation of extracted OPS with poly lactic-co-glycolic acid was performed. The antigenicity evaluation was conducted in 4 groups of 5 female BALB/c mice including OPS–PLGA conjugate, OPS alone, PLGA alone and PBS as a control. The mice were vaccinated intra-peritoneal three times with two-week intervals. To determine the immune response and functional capacity of the antibodies, the enzyme linked immunosorbent, opsonophagocytosis and challenge tests were performed. For checking the immunization ability of the nanovaccine, the challenge test was performed. The results showed a significant increase in the total IgG and IgM antibody titres in the mice vaccinated with OPS–PLGA conjugate in comparison with other groups. The sera of animals immunized with OPS–PLGA conjugate promoted efficient opsonophagocytosis of Brucella bacteria. The results of challenge assay showed that the immunization with OPS–PLGA conjugate gave a high level of protection in comparison with other groups. These findings showed that the new nanovaccine can be considered as a candidate for immunization of animals and humans against the diseases caused by B. melitensis that needs further investigations.
Introduction
Brucella, coccobacilli, gram-negative and non-moving bacteria is a cause of fever of Malta, Mediterranean fever, or feverish fever in humans. The pathogenicity of Brucella melitensis is greater for humans than other strains of Brucella [Citation1]. International reports show that more than 500,000 new cases of brucellosis occur annually in humans [Citation2]. Antibiotic regimens for human brucellosis patients may last several months and are not always completely effective. In some patients, symptoms of acute brucellosis can endure over one year and eventually result in chronic persistence [Citation3]. On the other hand, The United States Centres for Disease Control and Prevention (CDC) has classified bioterrorism agents into three categories. In this classification, Brucellosis was also included in the second group in terms of ease of release and mortality [Citation4]. Brucella spp. is highly infectious through aerosol route, making it an attractive pathogen to be used as a potential agent for biological warfare [Citation2]. While there are no vaccines for humans, several licenced live Brucella vaccines are available for use in livestock, but existing animal vaccines may lead to illness and are considered inappropriate for use in humans [Citation5,Citation6]. The use of cellular components of bacteria for sterile immunity has been investigated in previous studies [Citation7]. But the problems of this method, weakness of soluble antigens, apart from some larger delivery vectors, are the less absorption by cells and consequently less stimulation of the immune system. This has led to the spread of vaccine carriers that have more ability to imitate the physical–chemical properties of natural pathogens and increase the delivery of antigen to the immune system [Citation8].
Recently, nanotechnology has been utilised through more powerful and complex adjuvants to meet the challenge of bio modelling. Although, initial adjuvants like aluminium hydroxide have been successful in stimulating the immune response, the advent of nanoparticles has accelerated the transmission of these simple chemical stimulants to the immune system. In a previous study, the difference in the effect of alum along with PLGA nanoparticles on the immune system has been investigated. The results showed that conjugated antigens with PLGA nanoparticles provide better immunity [Citation9]. In fact, adjuncts can stimulate direct interaction and responses of the immune system more closely to those used by pathogens [Citation10]. So far, nanovaccines have been developed for malaria, leishmaniasis, chlamydia, tuberculosis and other infections, some of which have been effective and others have failed to produce advanced immunity [Citation11].
In recent years, poly (lactic-co-glycolic acid) (PLGA) has attracted interest as a base material for drug delivery applications because of its: (a) compatibility with biological conditions and lack of toxic and harmful effects, (b) tailored biodegradation rate (depending on the molecular weight and copolymer ratio), (c) possibility for clinical use in humans and (d) high potential for better interaction with biological materials. Delivery based on antigen-presenting cells (APCs) particles is a promising technology due to the ability to targeting such as stimulating the absorption of dendritic genes anti-cells (DCs), delivering antigens and stimulant signals and producing strong and distinct immune responses. Each of these features may be adjusted, depending on the need, in addition to vaccines which formulated based on different physio-chemical characteristics of the particles [Citation8]. PLGA nanoparticles have been used to create prototypes of vaccines against bacterial antigens such as: Staphylococcal enterotoxin, Tetanus toxoid, Yersinia pestis, Bacillus anthracis and Brucella abortus [Citation12–16]. Manish et al. reported that encapsulation of Bacillus anthrax peptide in PLGA nanoparticles enhances the immunity 5 times more than the free peptides [Citation17]. Also, Murtada et al. reviewed the biodegradable nanoparticles of PLGA 85/15 as carriers for the delivery of recombinant peptides momp-187 on Chlamydia trachomatis, and showed encapsulating the momp-187 in PLGA, successfully. PLGA promotes the capacity of the peptide to induce Th1 to produce cytotoxins [Citation18]. Also, Alikhani, et al. worked on the effectiveness of a nanovaccine against Pseudomonas aeroginosa based on PLGA nanoparticles. Their results showed that the prepared nanovaccine has the ability to produce high antibody titres without any histopathological effects [Citation19].
A lot of researches have been carried out on the development of vaccines against brucellosis infections. But because of the inability to create sterile immunity, inability to produce long-term immune responses and the inability to stimulate the cellular and humoral immunity simultaneously led to the inability to produce the effective vaccines [Citation20]. Oligopolysaccharide (OPS) is the most foreign and most antigenic portion of the Brucella lipopolysaccharide (LPS) that is the most relevant antigen for vaccination against brucellosis [Citation21]. In this study, PLGA nanoparticles were used as a carrier for long term release of OPS antigen and simultaneous stimulation of cellular and humoral immunity.
Materials and methods
Materials
PLGA nanoparticles (lactide:glycolide (50:50), mol. wt. 30,000–60,000), Ethylene di methylcarbamate propyl hydrochloride (EDCA), N-hydroxyl succinimide (NHC), DMF (Dimethylformamide), CaCl2, KBr, DMSO, BrCN (Cyanogen bromide), Sodium bicarbonate, HRP-conjugated anti-mouse IgG, Tetramethylbenzidine were purchased from Sigma-Aldrich (St Louis, MO). The strain of B. melitensis M16 bacteria was prepared from Pasteur Institute of Iran (IPI). Brucella Broth and Brucella Agar were purchased from Merck (Darmstadt, Germany). 100 BALB/c mice (6–8 week-old, 25–30 g) were purchased from Royan Institute of Iran.
Bacteria cultivating and isolation of OPS
To prepare the seed cells, the B. melitensis bacteria were grown in Brucella agar culture medium at 37 °C for 72 h. Then, to prepare the cell mass of B. melitensis the fermentation process was performed. In process of fermentation, the bacteria were grown in 6 L Brucella broth medium at 37 °C, around 45 rpm and aeration of 8 L/min for 60 h [Citation22]. For OPS extraction, 6.75 mL of 90% phenols was added to the fermenter tank to inactivate the bacteria. After 45 m, the content of fermenter was evacuated and placed into a water bath at 80 °C for 90 min. The resulting biomass was centrifuged at 4 °C for 30 min at 2500 rpm. 25 g of wet weight of the bacteria was autoclaved at 200 mL of 2% acetic acid solution for 15 m at 121 °C. Then, the bacteria solution was centrifuged for 10 m at 2500 rpm. Then the centrifuged solution and supernatant were mixed with 50 g of trichloroacetic acid and the supernatant was dialysed against distilled water. Next, OPS was precipitated by adding ethanol up to three times of supernatant. After 24 h, the liquid phase was evacuated from OPS sedimentation and OPS sediment was collected and maintained at 4 °C [Citation23].
Conjugation of OPS with PLGA
0.5 mM adipic acid dihydrazide (ADH), 0.5 mM bicarbonate and 100 mL of distilled water were added to 500 mg OPS. The pH was adjusted by HCL 0.1 molar at 8.5 and maintained for 18 h in 4 °C. The ADH-OPS were dialysed three times against distilled water (dialysis bag with the cut-off 10,000 kDa) to extract extra ADH molecules and concentrated by ultrafiltration with a cut-off 10,000 kDa at 2500 rpm for 30 m. In the next step, ADH-OPS (1 mL) was reacted with PLGA (1 mM) in the presence of EDC (1 mM) and N-hydroxysulfo-succinamide (N-HS) (1 mM) for 24 h at 25 °C. shows a schematic of the conjugation procedure.
In order to isolate the non-conjugated molecules from OPS–PLGA conjugate, we used a hand-made gel filtration sephadex G-75 pillar (with a height of 24 cm × 2 cm) and a PBS diluent buffer was used with flow rate about 40 mL/h. Up to the time of liquid transplantation, it was continued equal to the volume of the bed from the column. It was then detected by reading the absorbance of related fractions to the OPS–PLGA conjugate at 210 nm wavelengths and kept for the next steps in the 4 °C [Citation24,Citation25].
Quality control
To ensure the accuracy of the OPS–PLGA conjugate formation, the Zeta Sizer (ZEN3600, Malvern, UK) device was used to measure the size and charge of OPS–PLGA conjugate and PLGA alone. 0.01 mg OPS–PLGA specimen was dissolved in 1 mL distilled water. Then, it was sonicated for 1 m, and the specimen was placed in the Zeta Sizer device. Next, the infrared spectroscopy (FTIR) was used to identify the functional groups and covalent bonds in the prepared conjugate and PLGA alone. First, 2 mg of the sample was prepared with KBr powder and prepared in a clear tablet form and placed in a special enclosure of FTIR device (Tensor27, Bruker, Germany) and measured by absorption device in the IR area [Citation26]. The atomic force microscopy (Entegra Afmnt, NT-MDT, Moscow, Russia) was used to confirm the adsorption of OPS by PLGA NPs. First, the samples were taken up to 10 μL and spread on a special slide and after drying the samples including OPS–PLGA conjugate and PLGA alone; they were studied using AFM [Citation27].
Immunization with prepared vaccine in mouse model
One-hundred BALB/c mice (6–8 week old, 25–30 g) were divided into 4 groups of 25 labelled for peritoneal injection to assess immunogenicity. The first group received OPS–PLGA conjugate. The second group received OPS antigen alone. The third group received PLGA NPs alone and the fourth group was injected with PBS (as a control group). Each injectable dose was included 10 µg of antigen in three times in a 2 weeks interval via the peritoneal. Blood samples were collected from the heart of the mice after the first vaccination course on days 14, 28 and 42 (antibody titration). To study opsonophagocytosis, blood collection from the heart of mice was performed 2 weeks after the last injection. The blood of the mice was centrifuged 10 m at 2500 rpm. The collected serums were stored at −70 °C until the next step [Citation28]. For performing the challenge test, 2 weeks after the last vaccination, the B. melitensis bacteria (5 × 105 CFU/mL) was injected to mice (4 groups of 5 each). In this research, all animal protection laws were considered.
Immunogenic analysis
In this study, the serum antibody titres including IgM, IgA, IgG, IgG1, IgG2a, IgG2b and IgG3 against oligopolysaccharide and OPS–PLGA conjugate were measured using ELISA method. An OPS antigen concentration of 100 μg/mL was prepared in a carbonate buffer of 0.05 M with a pH of 9.6. Then, 200 μL was added to the wells of the first column of the 96 wells plate. Next, 100 μL of PBS were added to other wells and they diluted up serially to eighth column using the antigens in the wells in the first column. In the eighth column, the buffer was added without antigen and the plate was placed at 4 °C for 24 h. Then, the plate was washed three times with Tris-Buffered Saline (TBS) containing 0.05% Tween 20 (TBS-Tween). Next, 300 µL PBS containing 2% BSA was added to each well as a blocking buffer for 1 h at room temperature.
The plate was washed again three times with TBS-Tween. Then, the serum of vaccinated mice was diluted (Dilution prepared 1.5) with block buffer (TBS-Tween 20 containing 1% BSA, 0.05% NaN3). 200 μL of diluted serum was added to the first column of the wells and 100 μL of diluent buffer (TBS containing 0.05% (1% BSA and Tween 20)) was added to the other wells. Then, the serial dilution was performed and the plate was incubated at room temperature for 2 h. Then, the plate was washed three times with TBS-Tween. Next, 100 µL HRP-conjugated anti-mouse antibody (IgM, IgA, IgG, IgG1, IgG2a, IgG2b and IgG3) diluted 1:1000 using PBS was added to each horizontal row of microplate wells as the secondary antibody and incubated for 1 h at room temperature. The plates were washed again three times with TBS-Tween. Then, tetramethylbenzidine was used (100 µL each well) as a substrate to develop the reaction in the dark for 15–20 m. Then, the reaction was stopped with 50 µL of H2SO4 in each well, and the results were read at the optical density of 450 nm by an ELISA reader (MR-96A, Mindray, Shenzhen, China). The anti-OPS antibody evaluation was performed in triplicate [Citation29–31].
Opsonophagocytic assay
The opsonophagocytic killing (OPK) assay was used as a correlate for protection to measure the functional capacity of vaccine-candidate-raised antibodies. Initially, 25 μL of the heat-inactivated mice serum recipient of OPS–PLGA conjugate, OPS alone, PLGA alone and negative control was shed in the first wells of a 96-well plate, vertically. Then, about 25 μL of hanks buffer was added to the other wells and then serial dilution was performed for each of the inactivated sera. Then, 20 μL of Hanks buffer containing agarose (0/1 mg/mL) was added to all the wells. In the next step, 10 μL of B. melitensis suspension was added to the wells (∼2000 CFU/well) and the plate was placed at 37 °C for 5 m. Next, 24 μL complement (baby rabbit serum (final concentration: 12.5%)) was added to each well, and the plate was placed on an automatic shaker for 30 m at 37 °C at 220 rpm. Then, the isolated mice polymorphonuclear (PMNs (were added at a 400:1 ratio to the bacterium (target)-complement-serum mixture (final volume, 160 μL/well). Then, the plate was placed on an auto shaker for 40 m at 37 °C at 250 rpm. In the next step, the solution in each well was diluted with an equal volume of 0.9% NaCl (160 μL/well). After 5 m, 10 μL from each well was harvested and cultured on Brucella agar culture medium. Then culture mediums was placed at 5% CO2 and 37 °C for 5 days. Then, the number of bacteria on culture mediums was counted [Citation32,Citation33].
Protection assay
Two weeks after the last vaccination, 5 × 105 CFU/mL of B. melitensis bacteria were injected intraperitoneally to the mice vaccinated with OPS–PLGA conjugate, OPS alone, PLGA alone and PBS (as a negative control). After 4 weeks, the mice were killed and the spleens were removed and weighed aseptically. Each spleen was homogenised in 10 mL of PBS and serial dilution was prepared. The cultivation was carried out on the Brucella agar medium. The culture medium was placed to 5% CO2 at 37 °C for 5 days. The CFU from the virulent challenging strain per spleen was calculated by subtraction of numbers obtained in non-selective and selective conditions and then logarithmically transformed. The results were expressed as the mean log CFU ± SD per group [Citation34–36].
Statistical methods
Study the significant statistical differences in immunogenic analysis, opsonophagocytosis and challenge tests using the SPSS version 16 software. The p-value of <.001 was considered as significant. All the experiments were repeated three times.
Results
Characterisation of OPS–PLGA conjugate
Zeta potential, size
The surface charge of the nanoparticles was determined by measuring the zeta potential. The charge of PLGA nanoparticles (black line) was −2.98 mv, which was increased to 0.599 mv after conjugation with OPS (blue line), that indicates the success of conjugation (. represents the size distribution. As you can see in this Figure, the diameter of the PLGA nanoparticles is 85 nm (black line) that increases to 126 nm in conjugation form with OPS (blue line).
FTIR spectroscopy
The are related to the FTIR spectra of the PLGA nanoparticles and the OPS–PLGA conjugate, respectively. In , peak spreading in the area of 3000–4000 cm−1 is very small and is related to the OH group of PLGA nanoparticles, but in , peak spreading in the FTIR spectrum of the OPS–PLGA conjugate in the 3000–4000 cm−1 region represents the presence of NH and OH groups. The increase of OH group in this region is related to the OPS antigen carbohydrate compounds. A small peak spreading in the FTIR spectrum of OPS–PLGA conjugate in region 2902 cm−1 represents the aliphatic C–H groups which are related to PLGA and OPS. The FTIR spectrum of OPS–PLGA conjugate represents a free carbonyl group in region 1784 cm−1 and a carbonyl involved in conjugation in region 1609 cm−1. The expansion of the FTIR spectrum of the conjugate in region 1080 cm−1 is related to C–H ether structure of the PLGA NPs (. The results of the study showed that the FTIR spectra added to the FTIR spectrum of the PLGA nanoparticles were related to the structure of the OPS antigen, and these spectra confirmed the binding of PLGA nanoparticles to the OPS antigens.
AFM
In , the AFM images of the PLGA NPs and OPS–PLGA conjugate were presented. These images depict PLGA and OPS–PLGA surface topography. The raw images were analysed by JPK software version 3.4.15 in order to investigate the changes in the topography of the surfaces. In , three-dimensional images were derived from the surface of the PLGA and OPS–PLGA specimen from different sections of the surface, respectively. The dimensions used for these images were exactly like the images of the PLGA samples, which makes it easier to compare the images. As shown in , the AFM images of the OPS–PLGA sample have a symmetrical surface topography compared to the PLGA sample. It can be argued that the connection of OPS to PLGA has led to more uniform surface topography.
Antibody titres
The titres of antibodies IgA, IgM, IgG, IgG1, IgG2a, IgG2b and IgG3 were measured in mice treated with OPS–PLGA conjugate, OPS alone, PLGA alone and PBS as a control to evaluate the humoral immune response (). Antibody titres in the mice treated with OPS–PLGA conjugate were significantly more than the mice treated with OPS alone and had a significant increase compared to the mice treated with PLGA alone or PBS. A significant increase in antibody titres was seen in the groups treated with OPS–PLGA conjugate and OPS alone between the first, second and the third injections. There was seen a significant difference in IgA and IgM titres in the main group in comparison with the OPS group, especially after the third vaccination. Meanwhile, the antibody titres of IgG and its sub-classes in the OPS–PLGA group were significantly higher than the OPS group after the third injection. As it can be seen the highest antibody titre is observed in immunoglobulins IgM, IgG, IgG1 and IgG3 and the least antibody titre is related to the immunoglobulin IgA. There were not seen any immunisation effect after PLGA or PBS injections, so their results were not shown in this Figure.
Figure 5. Distribution and kinetics of antibody titres. The IgA (a), IgM (b), IgG (c), IgG1 (d), IgG2a (e), IgG2b (f), IgG3 (g) show the results of serum antibody production against B. melitensis. The values are the average of three replicates (±SD). Different letters represent the meaningful of differences in level p < .001.
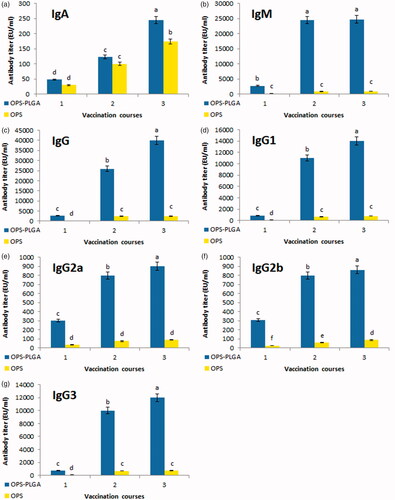
Opsonophagocytosis assay
We evaluated the amount of opsonophagocytosis of B. melitensis in mice receiving OPS–PLGA conjugate. The results showed that produced antibodies in mice which receiving conjugate OPS–PLGA had higher performance than the OPS group. The bacterial growth rate in OPS–PLGA and OPS recipient groups were 126 ± 14 and 239 ± 19, respectively and less than the control group. The amount of inhibition of bacterial growth in serum dilutions was shown in . The control wells, which contained only bacteria, were plated out without incubation (time zero) and were used as the CFU baseline reference. The control wells (after incubation) lacked the test serum but contained bacteria, complement, and effector cells. Upon plating after the opsonisation and phagocytic step incubations (75 min total), the wells had to yield counts on average 436 ± 48 CFU/well for assay admission. This observed number of CFU from the control wells after incubation then provided the basis for calculation reduction in CFU. The lowest titre of opsonophagocytic antibody that could be measured by our method was 9, based on the dilution of undiluted serum in the incubation well. The results of the phagocytosis epsilon colony counting were presented in . Thus, by comparing the values it can be found that the number of bacteria grown in serum of mice treated with OPS–PLGA conjugate represents a significant difference between the test group and the control group (p-value < .001).
Table 1. Opsonophagocytosis activity of test groups.
Protection assay
The vaccinated mice were challenged by B. melitensis M16 to examine the protective activity of the induced immunity. In this experiment, the protection was determined by calculating the reduction of spleen bacteria of vaccinated mice in comparison with the PBS recipient group. The bacteria counted in the OPS–PLGA group were 4.46 ± 0.09 and in the OPS group were 5.13 ± 0.08. As it was expected, the OPS–PLGA conjugate has caused much more protection in comparison with other trials (p-value < .001). As a result, the vaccinated mice with OPS–PLGA conjugate were more resistant against B. melitensis bacteria in comparison with the other groups. The protection level is shown in .
Table 2. Level of protection against B. melitensis infection induced by OPS–PLGA conjugate and OPS immunization.
Discussion
Initially in this study, PLGA nanoparticles were conjugated with OPS antigen of B. melitensis strain M16 using of carbodiimide system that mediates amide bonds between carboxylates and amines. Then, zeta potential test, FTIR spectroscopy, and AFM photography were performed to ensure the accuracy of conjugation. The immunogenic analysis showed the ability of the prepared nanovaccine for enhancing the serum antibody titre dramatically in particular in the production of antibodies on the class IgG and IgM. Interestingly, the results showed that class IgG antibodies have been increased much more in comparison with other antibodies. The titre of IgG antibodies produced after the first injection of the prepared nanovaccine was 2600 that increased to 40,000 after the third injection. The IgG antibodies are the most potent anti-toxin and have the highest half-life in comparison with the rest of the antibodies and at high concentrations they have a half-life for 15 to 20 days. These findings also demonstrated that the amount of antibody titre was heavily dependent on the loading of OPS on the PLGA NPs because OPS alone could not induce a high titre antibody in comparison with OPS–PLGA conjugate.
On the other hand, the new nanovaccine showed the production of high-performance antibodies in the process of opsonophagocytosis test. As shown in , the serum of the mice treated with the conjugate was able to diagnose the B. melitensis bacteria and destroying them by PMNs. The findings indicated that these mice had higher efficacy to destroy the bacteria compared to the mice treated with OPS alone that accordingly approves the higher antibody production in the main group. The calculation of the inhibitory level (percent of inhibition of OPK activity) showed that the mice treated with OPS–PLGA conjugate had the highest inhibition of bacterial growth up to 71% in comparison with the mice treated with OPS alone (45%) that indicates the higher effect of OPS–PLGA conjugate on the immune system for antibody production (). Then, the protection assay was performed to confirm the results of opsonophagocytosis test. Obviously, the findings confirmed the results of opsonophagocytosis test. In this test, the highest level of protection was seen in the mice treated with the OPS–PLGA conjugate (2.1 unit of protection) in comparison with the mice treated with OPS alone (1.43 unit of production). Overall, the results of this study showed that the designed nanovaccine is much more effective than OPS alone to produce high-performance antibodies with high titre levels and can be considered as a vaccine against B. melitensis in human beings that needs further investigations.
Conclusions
In the present study, it was shown that the OPS–PLGA conjugate is an effective immunogenic agent for inducing humoral immune response and antibody production in the mouse model and can be considered as a vaccine in human beings that needs further investigations. According to the results, PLGA nanoparticles can be considered as a suitable transfer system for OPS antigen to increase immunity against brucellosis.
Disclosure statement
No potential conflict of interest was reported by the authors.
References
- Aparicio ED. Epidemiology of brucellosis in domestic animals caused by Brucella melitensis, Brucella suis and Brucella abortus. Rev Sci Tech Off Int Epiz. 2013;32(1):53–60.
- Singh D, Goel D, Bhatnagar R. Recombinant L7/L12 protein entrapping PLGA (poly lactide-co-glycolide) micro particles protect BALB/c mice against the virulent B. abortus 544 infection. Vaccine. 2015;33(24):2786–2792.
- Alavi SM, Alavi L. Treatment of brucellosis: a systematic review of studies in recent twenty years. Caspian J Int Med. 2013;4(2):636.
- Fan Y, Moon JJ. Particulate delivery systems for vaccination against bioterrorism agents and emerging infectious pathogens. WIREs Nanomed Nanobiotechnol. 2017;9(1):e1403.
- Cutler SJ, Whatmore AM, Commander NJ. Brucellosis–new aspects of an old disease. J Appl Microbiol. 2005;98(6):1270–1281.
- Yang X, Skyberg JA, Cao L, et al. Progress in Brucella vaccine development. Front Biol. 2013;8(1):60–77.
- McCullers JA, Dunn JD. Advances in vaccine technology and their impact on managed care. P & T. 2008;33(1):35.
- Danhier F, Ansorena E, Silva JM, et al. PLGA-based nanoparticles: an overview of biomedical applications. J Contr Rel. 2012;161(2):505–522.
- Desai MP, Hilfinger JM, Amidon GL, et al. Immune response with biodegradable nanospheres and alum: studies in rabbits using staphylococcal enterotoxin B-toxoid. J Microencaps. 2000;17(2):215–225.
- Kim MG, Park JY, Shon Y, Kim G, et al. Nanotechnology and vaccine development. Asian J Pharma Sci. 2014;9(5):227–235.
- Karch CP, Burkhard P. Vaccine technologies: from whole organisms to rationally designed protein assemblies. Biochem Pharmacol. 2016;120:1–4.
- Chen L, Li S, Wang Z, et al. Protective effect of recombinant staphylococcal enterotoxin A entrapped in polylactic-co-glycolic acid microspheres against Staphylococcus aureus infection. Vet Res. 2012;43(1):20.
- Jiang W, Schwendeman SP. Stabilization of tetanus toxoid encapsulated in PLGA microspheres. Mol Pharma. 2008;5(5):808–817.
- Huang S-S, Li I-H, Hong P-D, et al. Development of Yersinia pestis F1 antigen-loaded microspheres vaccine against plague. Int J Nanomed. 2014; 9:813.
- Singh D, Somani VK, Aggarwal S, et al. PLGA (85:15) nanoparticle based delivery of rL7/L12 ribosomal protein in mice protects against Brucella abortus 544 infection: a promising alternate to traditional adjuvants. Mol Immunol. 2015;68(2):272–279.
- Zhu M, Wang R, Nie G. Applications of nanomaterials as vaccine adjuvants. Human Vaccines Immunother. 2014;10(9):2761–2774.
- Manish M, Rahi A, Kaur M, et al. A single-dose PLGA encapsulated protective antigen domain 4 nanoformulation protects mice against Bacillus anthracis spore challenge. PLoS one. 2013;8(4):e61885.
- Fairley SJ, Singh SR, Yilma AN, et al. Chlamydia trachomatis recombinant MOMP encapsulated in PLGA nanoparticles triggers primarily T helper 1 cellular and antibody immune responses in mice: a desirable candidate nanovaccine. Int J Nanomed. 2013;8:2085.
- Alikhani Z, Salouti M, Ardestani MS. Synthesis and immunological evaluation of a nanovaccine based on PLGA nanoparticles and alginate antigen against infections caused by Pseudomonas aeruginosa. Biomed Phys Eng Express. 2018;4(4):045016.
- Dorneles EM, Sriranganathan N, Lage AP. Recent advances in Brucella abortus vaccines. Vet Res. 2015;46(1):76.
- Bundle DR, McGiven J. Brucellosis: improved diagnostics and vaccine insights from synthetic glycans. Acc Chem Res. 2017;50(12):2958–2967.
- Cryz SJ, Fürer E, Que JU. Synthesis and characterization of a Pseudomonas aeruginosa alginate-toxin A conjugate vaccine. Infect Immun. 1991;59(1):45–50.
- Oñate AA, Vemulapalli R, Andrews E, et al. Vaccination with live Escherichia coli expressing Brucella abortus Cu/Zn superoxide dismutase protects mice against virulent B. abortus. Infect Immun. 1999;67(2):986–988.
- Farjah A, Owlia P, Siadat SD, et al. Immunological evaluation of an alginate‐based conjugate as a vaccine candidate against Pseudomonas aeruginosa. APMIS. 2015;123(2):175–183.
- Weissenböck A, Wirth M, Gabor F. WGA-grafted PLGA-nanospheres: preparation and association with Caco-2 single cells. J Contr Rel. 2004;99(3):383–392.
- Kumar R, Sahoo GC, Pandey K, et al. Development of PLGA–PEG encapsulated miltefosine based drug delivery system against visceral leishmaniasis. Mater Sci Eng: C. 2016;59:748–753.
- Sun SB, Liu P, Shao FM, et al. Formulation and evaluation of PLGA nanoparticles loaded capecitabine for prostate cancer. Int J Clin Exper Med. 2015;8(10):19670.
- Zaidi TS, Priebe GP, Pier GB. A live-attenuated Pseudomonas aeruginosa vaccine elicits outer membrane protein-specific active and passive protection against corneal infection. Infect Immun. 2006;74(2):975–983.
- Díaz AG, Quinteros DA, Llabot JM, et al. Spray dried microspheres based on chitosan: a promising new carrier for intranasal administration of polymeric antigen BLSOmp31 for prevention of ovine brucellosis. Mater Sci Eng: C. 2016;62:489–496.
- Verdolin BA, Ficker SM, Faria AM, et al. Stabilization of serum antibody responses triggered by initial mucosal contact with the antigen independently of oral tolerance induction. Braz J Med Biol Res. 2001;34(2):211–219.
- Kashef N, Behzadian-Nejad Q, Najar-Peerayeh S, et al. Synthesis and characterization of Pseudomonas aeruginosa alginate–tetanus toxoid conjugate. J Med Microbiol. 2006;55(10):1441–1446.
- Hufnagel M, Koch S, Kropec A, et al. Opsonophagocytic assay as a potentially useful tool for assessing safety of enterococcal preparations. Int J Food Microbiol. 2003;88(2–3):263–267.
- Hu BT, Yu X, Jones TR, et al. Approach to validating an opsonophagocytic assay for Streptococcus pneumoniae. Clin Diagn Lab Immunol. 2005;12(2):287–295.
- Al-Mariri A, Tibor A, Mertens P, et al. Protection of BALB/c mice against Brucella abortus 544 challenge by vaccination with bacterioferritin or P39 recombinant proteins with CpG oligodeoxynucleotides as adjuvant. Infect Immun. 2001;69(8):4816–4822.
- Delpino MV, Estein SM, Fossati CA, et al. Vaccination with Brucella recombinant DnaK and SurA proteins induces protection against Brucella abortus infection in BALB/c mice. Vaccine. 2007;25(37–38):6721–6729.
- Al-Mariri A. Protection of BALB/c mice against Brucella melitensis 16 M infection induced by vaccination with live Escherchia coli expression Brucella P39 protein. Vaccine. 2010;28(7):1766–1770.