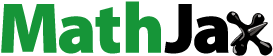
ABSTRACT
A type of nonstoichiometric piezoceramics, [(Na0.825K0.175)0.5Bi0.5]1+x TiO3 (BKNT-x, x = 0–0.009), was synthesized by the conventional sintering method. The effects of Bi3+, Na+ and K+ content on the structure and electrical properties of 0.825Bi0.5Na0.5TiO3-0.175Bi0.5K0.5TiO3 piezoceramics were investigated.The XRD patterns demonstrated that BKNT-x piezoceramics form a solid solution without a secondary phase. Meanwhile, SEM micrographs indicated that excess [(Na0.825K0.175)0.5Bi0.5]2+ suppresses grain growth and that BKNT-x ceramics become considerably denser. BKNT-0.002 ceramics offer the following enhanced properties: d33 = 154 pC/N, kp = 33%, εr = 1087, tanδ = 0.036, and Qm = 116. This improvement of properties is most likely related to the dense structure and the maintenance of morphotropic phase boundaries through the introduction of a proper A-site cation excess. For ceramics containing volatile elements, stoichiometric control is an efficacious method of enhancing performance.
1. Introduction
To date, lead-free piezoceramics have received a great amount of attention from researchers due to their environmental friendliness. Smolenski et al [Citation1]. reported Na0.5Bi0.5TiO3 (BNT) in 1960. At room temperature, rhombohedral BNT ceramic shows a high Curie point and relatively good electrical properties [Citation2,Citation3], which have made BNT ceramics become one of promising lead-free ceramics. BNT ceramics have a large coercive field Ec (73kv/cm), however, that makes ceramic poling difficult [Citation4,Citation5]. A great deal of modification work has been conducted since the early 1990s. Other approaches have been proved to be helpful in the poling of the ceramics, as well as, in improving their electrical properties, include the formation of new solid solutions, such as, Bi0.5(KxNa1-x-yLiy)0.5TiO3 [Citation6], BaTiO3(BT)-BNT [Citation3,Citation7], BNT-Na NbO3 [Citation8], K0.5Bi0.5TiO3(BKT)-BNT [Citation5], BT- BNT-BKT [Citation9], BNT-BaTiO3-NaNbO3 [Citation10], BKT-BNT-SrTiO3 [Citation11], Bi0.5(K0.20Na0.70Li0.10)0.5TiO3 [Citation12], Bi0.5(KxNa1-x-Agy)0.5 TiO3 [Citation13], and BNT-BT-(Na0.5K0.5)NbO3 [Citation14], and doping with metal oxides, such as, Dy2O3-doped (Na0.7K0.3)0.5Bi0.5TiO3 [Citation15], Gd2O3-doped (Na0.5 Bi0.5)0.94Ba0.06TiO3 [Citation16], Li2O-doped0.825BNT-0.175BKT [Citation17], La2O3-doped0.92BNT-0.08BT [Citation18]. Among these systems, BKT-BNT (BNKT) was observed by Sasaki et al [Citation5]. They found that it has relatively strong piezoelectric performance owing to the existence of morphotropic phase boundaries (MPB, x=0.16-0.2). However, properties of BNT-based piezoceramics are usually affected by the volatilization of A-site cations for the sintering process. Zuo R et al [Citation19]. revealed that the sintering behavior of (Na0.5Bi0.5)1+xTiO3 ceramics is sensitive to variants of A-site cations that contribute to enhancing its electrical properties(x=0.01, d33=83pC/N). Sung et al [Citation20,Citation21]. revealed that the effect of Bi nonstoichiometry on d33 and Td of BNT is different from the results of Na nonstoichiometry. Wu et al [Citation22]. found that a proper Na/K excess shows good electrical properties in 0.85 BNT-0.15BKT systems (Pr=13.6 μC/cm2, d33* = 56 pm/V). Prasertpalichat [Citation23] demonstrated that changes in Bi/Na stoichiometry have a significant influence on the electrical properties of (Bi0.5Na0.5)0.94Ba0.06TiO3 ceramics. Chu et al [Citation24]. also observed that changes in the A-site cations can effectively enhance the electrical properties of nonstoichiometric (Bi0.5Na0.5)0.92Ba0.08TiO3 systems (d33=149 pC/N, ε= 1230). Ni et al [Citation25]. reported that Bi0.505(K0.18Na0.82)0.485TiO3 ceramics show relatively good piezoelectric properties when tuned with A-site vacancies (d33=151pC/N, Pr=29.5μC/cm2). In order to clarify nonstoichiometry effects, therefore, it is significant to investigate the connection between the properties and the amount of A-site cations in BNT-based piezoceramics.
In this case, a type of lead-free [(Na0.825K0.175)0.5Bi0.5]1+xTiO3 piezoceramics was synthesized using a conventional mixed-oxide method. The influence of [(Na0.825K0.175)0.5Bi0.5]2+ nonstoichiometry on the microstructure, crystal phase and electrical properties of (Na0.825K0.175)0.5Bi0.5TiO3 (BKNT) piezoceramics was investigated in detail.
2. Experimental procedure
Piezoceramics [(Na0.825K0.175)0.5Bi0.5]1+xTiO3 (x = 0, 0.002, 0.004, 0.006, 0.009) were synthesized via a conventional solid state process. Bi2O3 (Chenguang Chemical Co., Ltd, Qishan, China, AR, 99.9%), K2CO3 (Xilong Chemical Co., Ltd, Shantou, China, AR, 99.8%), Na2CO3 (Xilong Chemical Co., Ltd, Shantou, China, AR, 99.9%), TiO2 (Zhongxing Electronic Materials Co., Ltd, Xiantao, China, AR, 99.5%) powders were used as raw materials with their water removed at 120 oC/24 h. According to the desired stoichiometry, all the powders were blended with anhydrous ethanol, and then ball-milled at a rate of 350 rotations/min for 7–8 h. When the powders become dry under 75 oC conditions, the mixture was calcined at 850 °C/2 h. The heating rate was 3 min/oC. Re-milled samples were blended with polyvinyl alcohol (5 wt%), and then pressed into discs measuring 10 mm in diameter and 1.2 mm in thickness under 20 Mpa pressure. The obtained samples were sintered at 1180 oC/2 h at a heating rate of 5 min/oC. In order to form electrodes, a coating of silver conducting paste (PC-Ag-8080, Xizhi Electronic Materials Co., Ltd, China) was applied to two sides of polished compositions, which were fired at 600 °C. In a silicone oil bath (80 oC), the annealed compositions (9 mm in diameter, 1 mm in thickness) were poled with a DC electric field (4.5 kV/mm) for 45 min.
The sintered compositions had been determined by XRD (Cu Kα radiation, Rigaku Dmax/RB). The lattice parameters of the BKNT-x ceramics were refined by the Rietveld refinement method [Citation26] and MAUD software (version 2.94) [Citation27–29]. The weighted profile residual factor (Rwp) was less than 15%, indicating good agreement of refinement. The density of the compositions was calculated by the Archimedes method. The microstructures of the samples were characterized by SEM (JSM-6510, Japan). The average grain size was obtained by measuring the number of grains at the intersection lines. The measurement of piezoelectric properties was carried out with a d33 meter (ZJ-3A, Institute of Acoustics, China). The dielectric properties of the BKNT-x piezoceramics were measured with an impedance analyzer (Agilent 4294A,1 KHz) at 25–500 °C. The kp and Qm were calculated by use of Onoe’s formula [Citation30], employing the resonance and antiresonance frequencies method as a basis.
3. Results and discussion
displays the XRD patterns of BKNT-x ceramics. All the compositions possess typical diffraction peaks displayed by an ABO3-type structure. There is no impurity phase in these solid solutions. Ni et al [Citation25]. observed that when x0.02, second phase Bi2Ti2O7 forms in (K0.18Na0.82)0.5–3xBi0.5+xTiO3 ceramics. Babu et al. [Citation31]
Figure 1. (a)XRD Patterns of various compositions in the BKNT-x ceramics; (b) and (c) The expanded XRD patterns of BKNT-x ceramics in the range of 39–47°
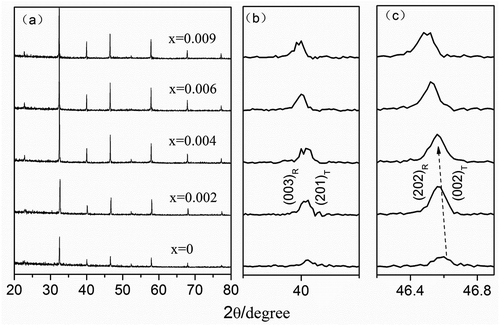
also demonstrated that excess K2CO3 (x ≤ 0.8 mol%) makes up for the volatility of K+ to maintain the phase purity of (Na0.8K0.2)0.5Bi0.5TiO3 piezoceramics. Seo et al [Citation32]. observed, moreover, that Ba0.06(Bi0.5Na0.5)0.94+xTiO3 (−0.01 ≤ x ≤ 0.02) piezoceramics possess a homogeneous structure with phase purity. Our results are consistent with previous reports, which showed that excess Bi3+, Na+, and K+(x ≤ 0.009) may keep (Na0.825K0.175)+/Bi3+ at 1:1 and maintain the phase purity of BKNT-x ceramics after the A-site cations of BKNT-x ceramics partly volatilize in the sintering process.
Sasaki et al [Citation5]. reported that (Na1-xKx)0.5Bi0.5TiO3 ceramics (BNKT) exhibit high performance due to the R-T MPB (0.16 ≤ x ≤ 0.2), which has a similar pseudocubic structure [Citation33]. To investigate the phase structure of BKNT-x ceramics, the magnified XRD patterns are given in the 2ɵ range of 39–47°, as shown in . According to the R3 c space group (COD ID: 2103295) and P4bm space group (COD ID: 2102068) [Citation34,Citation35], the structure could be designated by the following peaks: rhombohedral (003) and tetragonal (201) peaks at 40°, as well as rhombohedral(202) and tetragonal (002) peaks at 46°, repectively. The peak splitting reveals that BKNT-x ceramics are still in the rhombohedral(R)-tetragonal(T) phase transition range. In addition, the (002) peak moves toward lower angles with increases in x. The lattice parameters (aT, cT) and cell volume of the tetragonal phase of BKNT-x ceramics increase slightly with changes in the content of A-site cations, as shown in . In the sintering process, oxygen vacancies of BKNT ceramics are usually generated due to the volatilization of A-site cations. The addition of excess A-site cations (Bi3+, Na+, and K+, hereinafter BKN) balances the effect of BKN loss, which leads to a reduction in the number of oxygen vacancies. It is generally known that large numbers of oxygen vacancies can cause unit cells to shrink and lead to a shift of the diffraction peaks to high angles [Citation36–39]. Therefore, the low-angle movement of (002) peaks for BKNT-x ceramics indicates a decrease in the number of oxygen vacancies.
Table 1. Lattice Parameters and lattice volume of BKNT-x ceramics
displays surface micrographs of BKNT-x ceramics sintered at 1180 oC. The crystal grains of BKNT-x piezoceramics show a regular square shape. With increases in BKN, the average grain size decreases slightly. The mean crystallite sizes are about 1.28, 1.25, 0.98, 0.76 μm, respectively. This can probably be ascribed to the collection of excess BNK at the A-site lattice of ABO3 perovskite structure due to volatilization of A-site cations (Bi3+, Na+, and K+) at high sintering temperatures.This behavior can reduce oxygen vacancies, while the existence of the defects is conducive to mass transport, accelerating the grain growth [Citation40]. As a result, crystallite growth is restrained. This is similar to a previous report on (Bi0.5+xNa0.5)0.94Ba0.06TiO3 systems with A-site nonstoichiometry [Citation41]. It can be observed, from , that with the concentration of BKN, the relative densities of BKNT-x piezoceramics reach a maximum, and then descend, as was achieved by the theoretical density of (Na0.8K0.2)0.5Bi0.5TiO3 (5.889 g/cm3) [Citation42]. Appropriate BKN excesses are able to decrease the evaporation of volatile A-site cations, and contribute to an improvement in the density of BKNT-x ceramics [Citation43]. Bi2O3 with its melting point of 825°C usually decreases sintering temperatures by forming a liquid phase [Citation44]. With further increases in BKN(x > 0.004), the content of bismuth oxide goes up, which leads to lower sintering temperatures of BKNT-x piezoceramics. For this reason, the ceramics can’t be fully sintered at the same sintering temperature (1180 oC), resulting in decreased compactness. The relative densities of BKNT-x piezoceramics are more than 95% of the theoretical values when 0.002x
0.004. A dense microstructure is particularly advantageous to improvement of the electrical properties of BKNT-x ceramics.
Figure 2. SEM surface micrographs of BKNT-x ceramics with various compositions sintered at 1180 oC for 2 hours
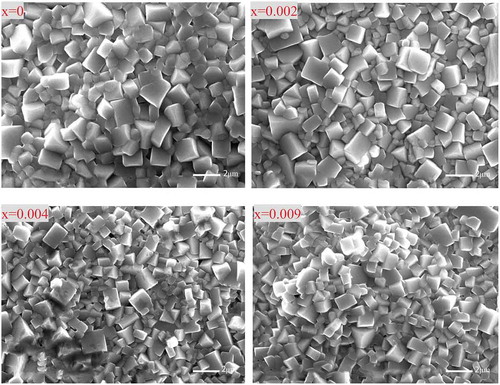
displays the temperature dependance of εr and tanδ for unpoled BKNT-x ceramics under different frequencies. BKNT-x ceramics have two dielectric anomalies: a relaxor antiferroelectric-antiferroelectric phase transformation and an antiferroelectric-paraelectric phase transformation corresponding to TRE and Tm, respectively, which are consistent with NBT-based piezoceramics [Citation5,Citation45–48]. A phenomenon of mergence is exhibited by the different εr-T curves of BKNT-x piezoceramics, where the temperature is described as TRE. Frequency has a great effect on εr when T< TRE. Ma et al. reported that nanodomains with a short-range order stimulate the relaxor behavior of BNT-based ceramics and suggested a new term, “relaxor antiferroelectric,” to describe the phenomenon of frequency dispersion [Citation46]. With further increments in temperature, the relative permittivity εr reaches a maximum value at the second dielectric anomaly, where the temperature is expressed as Tm. Ma et al. identified a long-range antiferroelectric(AFE) order presents in BNT-based ceramics at between TRE and Tm, and showed that the paraelectric phase is located at temperatures above Tm [Citation46]. As soon as the temperature rises above Tm, tanδ increases greatly due to the considerable leakage conduction [Citation49]. In addition, pure BKNT ceramics exhibit larger values for dielectric constants and loss tangents than BKNT-x ceramics at high temperatures (TTm) and low frequencies, which can be ascribed to the fact that A-site vacancies and oxygen vacancies form at preparation process and lead to the appearance of frequency dispersion for pure BKNT ceramics [Citation46].
Figure 4. Temperature dependence of relative permittivity and dielectric loss for BKNT-x ceramics (1180°C/2 h)
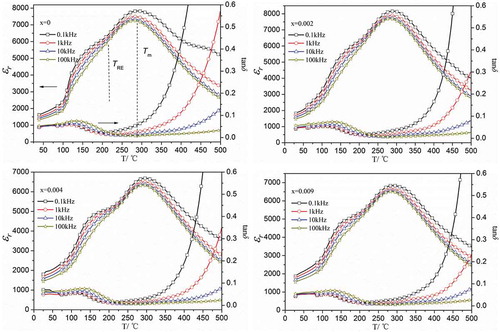
shows the electrical properties of BKNT-x piezoceramics. When the content of BKN is 0.2 mol%, the maximum values of the piezoelectric constant d33 and electromechanical coupling factor kp reach 154 pC/N and 33%, respectively. The comprehensive performance of BKNT-x piezoceramics is slightly superior to those of NBT-based ceramics with nonstoichiometry (see ). This can be ascribed to the following reasons: a proper BKN excess improves the compactability of BKNT-x piezoceramics which makes the polarization of ceramics more completely under a broad electrical field [Citation17]. Moreover, an excess amount of BKN can make up for the volatilization of A-site cations and maintain the R-T phase fractions of compositions around MPB, which improves the piezoelectricity of BKNT-x ceramics. Analogous to the close dependence of BKN content and d33, the maximum value of the relative dielectric constant εr (1087) occurs at x=0.002. Meanwhile, the dielectric loss tanδ initially decreases (x<0.002) and reaches a minimum value (0.0362), and then, finally increases with the concentration of BKN. Qm gradually decreases with the increases in BKN. This can be ascribed to the decrement of oxygen vacancies caused by the compensation of excess BKN for the loss of Bi3+, Na+ and K+.
Table 2. Comparison of the properties of different NBT-based ceramics with nonstoichiometry
The temperature dependence of the kp of BKNT-x ceramics is demonstrated in . The kp of BKNT-x ceramics shows a decreasing trend with increases in the annealing temperature. The kp changes significantly as the annealing temperature increases up to 134 oC (x=0.004, kp=0.03), and then decreases as the annealing temperature increases further. When the annealing temperature is 134-140 oC, the piezoelectric responses of BKNT-0.004 ceramics almost vanish. In other words, the depolarization temperature (Td) of BKNT-0.004 ceramics is about 134 oC, while pure BKNT ceramics have a high Td of around 140 oC, as reported by Sapper [Citation50]. As a result, the addition of excess BKN has a negative effect on Td. On the other hand, variations in Td can reflect changes in the oxygen vacancies. The depolarization temperature of ceramics increases at a high oxygen vacancy concentration owing to a strong coaction between the oxygen vacancies and the domains [Citation41]. Therefore, use of moderate amounts of BKN to compensate for the loss of Na+, Bi3+ and K+ during the sintering step contributes to a decrease in oxygen vacancies that causes Td to shift to a low temperature.
4. Conclusions
The influence of BKN on the structure, crystal phase and electrical properties of (Na0.825K0.175)0.5Bi0.5TiO3 piezoceramics, which were synthesized by conventional sintering, were systematically researched. All the BKNT-x piezoceramics have typical diffraction peaks with pure perovskite structures. Excess BKN compensates for the loss of the A-site cations, and leads to a decrease in oxygen vacancies which restrain the growth of crystallites. All the compositions are well sintered with dense microstructure at 1180 oC/2 h when 0.002x
0.004. BKNT-x piezoceramics with BKN compensation achieve high d33 (154 pC/N) and kp (33%). The electrical properties of BKNT-x ceramics have been enhanced by the introduction of excess BKN, which maintains the structural density and the R-T phase fractions of the samples at around MPB. As a result, in order to acquire the desirable electrical properties, it is essential to control the content of volatile A-site cations sufficiently.
Disclosure statement
No potential conflict of interest was reported by the authors.
Additional information
Funding
References
- Smolenskii GA, Isupov VA, Agranovskaya AI, et al. Ferroelectrics with diffuse phase transitions. Soviet Phys Solid State. 1961;2:2584.
- Takenaka T, Sakata K. Dielectric, piezoelectric and pyroelectric properties of (BiNa)1/2TiO3- based ceramics. Ferroelectrics. 1989;95(1):153–156.
- Tadashi T, Kei-ichi M, Koichiro S. (Bi1/2Na1/2)TiO3-BaTiO3 system for lead-free piezoelectric ceramics. Jpn J Appl Phys. 1991;30(9S):2236–2239.
- Hajime N. and Tadashi T. Lead-free piezoelectric ceramics of (NaBi)0.5TiO3-1/2(Bi2O3·Sc2O3) system. Jpn J Appl Phys. 1997;36(1):6055–6057.
- Sasaki A, Chiba T, Mamiya Y, et al. Dielectric and piezoelectric properties of (Bi0.5Na0.5)TiO3–(Bi0.5K0.5)TiO3 systems. Jpn J Appl Phys. 1999;38(9S):5564.
- Lin D, Xiao D, Zhu J, et al. Synthesis and piezoelectric properties of lead-free piezoelectric [Bi0.5(Na1−x−yKxLiy)0.5]TiO3 ceramics. Mater Lett. 2004;58(5):615–618.
- Zeng WD, Li QN, Zhou CR, et al. A new insight into structural complexity in ferroelectric ceramics. J Adv Ceram. 2017;6(3):262–268.
- Takenaka T, Okuda T, Takegahara K. Lead-free piezoelectric ceramics based on (Bi1/2Na1/2)TiO3-NaNbO3. Ferroelectrics. 1997;196(1–4):495–498.
- Hajime N, Masaki Y, Yoichi M, et al. Large piezoelectric constant and high curie temperature of lead-free piezoelectric ceramic ternary system based on bismuth sodium titanate-bismuth potassium titanate-barium titanate near the morphotropic phase boundary. Jpn J Appl Phys. 2003;42(12R):7401–7403.
- Xu Q, Lanagan MT, Luo W, et al. Electrical properties and relaxation behavior of Bi0.5Na0.5TiO3-BaTiO3 ceramics modified with NaNbO3. J Eur Ceram Soc. 2016;36(10):2469–2477. .
- Yoo J, Oh D, Jeong Y, et al. Dielectric and piezoelectric characteristics of lead-free Bi0.5(Na0.84K0.16)0.5TiO3 ceramics substituted with Sr. Mater Lett. 2004;58(29):3831–3835.
- Guo K, Sharifzadeh Mirshekarloo M, Lin M, et al. Microstructure and piezoelectric properties of thermal sprayed Bi0.5(Na0.70K0.20Li0.10)0.5TiO3 ceramic coatings. Ceram Int. 2019;45(3):3570–3573.
- Liao Y, Xiao D, Lin D, et al. Synthesis and properties of Bi0.5(Na1−x−yKxAgy)0.5TiO3 lead-free piezoelectric ceramics. Ceram Int. 2007;33(8):1445–1448.
- Shi X, Kumar N, Hoffman M. Electric field-temperature phase diagrams for (Bi1/2Na1/2)TiO3-BaTiO3-(K1/2Na1/2)NbO3 relaxor ceramics. J Mater Chem C. 2018;6(45):12224–12233.
- Muneeswaran M, Choi BC, Chang SH, et al. Effect of dysprosium doping on structural and vibrational properties of lead-free (Na0.7K0.3)0.5Bi0.5TiO3 ferroelectric ceramics. Ceram Int. 2017;43(16):13696–13701.
- Turki O, Slimani A, Seveyrat L, et al. Structural, dielectric, ferroelectric, and electrocaloric properties of 2% Gd2O3 doping (Na0.5Bi0.5)0.94Ba0.06TiO3 ceramics. J Appl Phys. 2016;120(5):054102.
- Chen X, Liao Y, Mao L, et al. Microstructure and piezoelectric properties of Li-doped Bi0.5(Na0.825K0.175)0.5TiO3 piezoelectric ceramics. Phys Status Solidi A. 2009;206(7):1616–1619.
- Liu L, Zhu M, Hou Y, et al. Abnormal piezoelectric and dielectric behavior of 0.92Na0.5Bi0.5TiO3-0.08BaTiO3 induced by La doping. J Mater Res. 2011;22(5):1188–1192.
- Zuo R, Su S, Wu Y, et al. Influence of A-site nonstoichiometry on sintering, microstructure and electrical properties of (Bi0.5Na0.5)TiO3 ceramics. Mater Chem Phys. 2008;110(2):311–315.
- Sung YS, Kim JM, Cho JH, et al. Effects of Bi nonstoichiometry in (Bi0.5+xNa)TiO3 ceramics. Appl Phys Lett. 2011;98(1):012902.
- Sung YS, Kim JM, Cho JH, et al. Effects of Na nonstoichiometry in (Bi0.5Na0.5+x)TiO3 ceramics. Appl Phys Lett. 2010;96(2):022901.
- Wu Y, Wang X, Zhong C, et al. Effect of Na/K excess on the electrical properties of Na0.5Bi0.5TiO3–K0.5Bi0.5TiO3 thin films prepared by sol–gel processing. Thin Solid Films. 2011;519(15):4798–4803.
- Prasertpalichat S, Schmidt W, Cann DP. Effects of A-site nonstoichiometry on oxide ion conduction in 0.94Bi0.5Na0.5TiO3-0.06BaTiO3 ceramics. J Adv Dielectr. 2016;6:2.
- Chu B-J, Chen D-R, Li G-R, et al. Electrical properties of Na1/2Bi1/2TiO3–BaTiO3 ceramics. J Eur Ceram Soc. 2002;22(13):2115–2121.
- Ni F, Luo L, Pan X, et al. Effects of A-site vacancy on the electrical properties in lead-free non-stoichiometric ceramics Bi0.5+x(Na0.82K0.18)0.5−3xTiO3 and Bi0.5+y(Na0.82K0.18)0.5TiO3. J Alloys Compd. 2012;541:150–156.
- Rietveld HM. A profile refinement method for nuclear and magnetic structures. J Appl Crystallogr. 1969;2(2):65–71.
- Lutterotti L, Bortolotti M. Object oriented programming and fast computation techniques in MAUD, a program for powder diffraction analysis written in java. IUCr Compcomm Newsletter. 2003;1:43–50.
- Lutterotti L, Matthies S, Wenk H. MAUD: a friendly Java program for material analysis using diffraction. IUCr: Newsletter CPD. 1999;21:14–15.
- Lutterotti L, Matthies S, Wenk H, MAUD (Material Analysis Using Diffraction): a user friendly Java program for Rietveld texture analysis and more. pp. 1599 In Proceeding of the Twelfth International Conference on Textures of Materials (ICOTOM-12). Vol. 1, Ottawa, Canada.
- Onoe M, Jumonji H. Useful formulas for piezoelectric ceramic resonators and their application to measurement of parameters. J Acoust Soc Am. 1967;41(4B):974–980.
- Veera Gajendra M, Babu SM, Abdul Kader M, et al. Enhanced piezoelectric constant and remnant polarisation in K-compensated sodium potassium bismuth titanate. Mater Lett. 2015;146:81–83.
- Seo I-T, Steiner S, Frömling T. The effect of A site non-stoichiometry on 0.94(NayBix)TiO3-0.06BaTiO3. J Eur Ceram Soc. 2017;37(4):1429–1436.
- Seifert KTP, Jo W, Rödel J. Temperature-insensitive large strain of (Bi1/2Na1/2)TiO3–(Bi1/2K1/2)TiO3–(K0.5Na0.5)NbO3 lead-free piezoceramics. J Am Ceram Soc. 2010;93(5):1392–1396.
- Jones GO, Thomas PA. Investigation of the structure and phase transitions in the novel A-site substituted distorted perovskite compound Na0.5Bi0.5TiO3. Acta Crystallographica Section B. 2002;58(2):168–178.
- Jones GO, Thomas PA. The tetragonal phase of Na0.5Bi0.5TiO3 - a new variant of the perovskite structure. Acta Crystallographica Section B. 2000;56(3):426–430.
- Lei N, Zhu M, Yang P, et al. Effect of lattice occupation behavior of Li+ cations on microstructure and electrical properties of (Bi1/2Na1/2)TiO3-based lead-free piezoceramics. J Appl Phys. 2011;109(5):054102.
- Shannon R. Revised effective ionic radii and systematic studies of interatomic distances in halides and chalcogenides. Acta Crystallogr A. 1976;32(5):751–767.
- Akram F, Ahmed Malik R, Hussain A, et al. Temperature stable dielectric properties of lead-free BiFeO3–BaTiO3 modified with LiTaO3 ceramics. Mater Lett. 2018;217:16–19.
- Wang B, Luo L, Ni F, et al. Piezoelectric and ferroelectric properties of (Bi1−xNa0.8K0.2Lax)0.5TiO3 lead-free ceramics. J Alloys Compd. 2012;526:79–84.
- Lee YC, Huang YL. Effects of CuO doping on the microstructural and dielectric properties of Ba0. 6Sr0. 4TiO3 ceramics. J Am Ceram Soc. 2009;92(11):2661–2667.
- Qiao X-S, Chen X-M, Lian H-L, et al. Microstructure and electrical properties of nonstoichiometric 0.94(Na0.5Bi0.5+x)TiO3–0.06BaTiO3 lead-free ceramics. J Am Ceram Soc. 2016;99(1):198–205.
- Jones GO, Kreisel J, Thomas PA. A structural study of the (Na1−xKx)0.5Bi0.5TiO3 perovskite series as a function of substitution (x) and temperature. Powder Diffr. 2012;17(4):301–319.
- Sung YS, Baik S, Lee JH, et al. Enhanced piezoelectric properties of (Na0.5+y+zK0.5−y)(Nb1−xTax)O3 ceramics. Appl Phys Lett. 2012;101(1):012902. .
- Zhao Y, Xu Z, Chu R, et al. Improved piezoelectricity and high strain response of (1 − x)(0.948K0.5Na0.5NbO3 − 0.052LiSbO3) − xBi2O3 ceramics. J Mater Sci Mater Electron. 2017;28(2):1211–1216.
- Kazushige Y, Yuji H, Hajime N, et al. Electrical properties and depolarization temperature of (Bi1/2Na1/2)TiO3 –(Bi1/2K1/2)TiO3 lead-free piezoelectric ceramics. Jpn J Appl Phys. 2006;45(5S):4493.
- Ma C, Tan X, Dul’kin E, et al. Domain structure-dielectric property relationship in lead-free (1−x)(Bi1/2Na1/2)TiO3-xBaTiO3 ceramics. J Appl Phys. 2010;108(10):104105.
- Akram F, Malik RA, Song TK, et al. Thermally-stable high dielectric properties of (1–x)(0.65Bi1.05FeO3–0.35BaTiO3)–xBiGaO3 piezoceramics. J Eur Ceram Soc. 2019;39(7):2304–2309.
- Akram F, Kim J, Khan SA, et al. Less temperature-dependent high dielectric and energy-storage properties of eco-friendly BiFeO3–BaTiO3-based ceramics. J Alloys Compd. 2020;818:152878.
- Sakata K, Takenaka T, Naitou Y. Phase-relations, dielectric and piezoelectric properties of ceramics in the system (Bi0.5Na0.5)TiO3-PbTiO3. Ferroelectrics. 1992;131(1–4):219–226.
- Sapper E, Schaab S, Jo W, et al. Influence of electric fields on the depolarization temperature of Mn-doped (1-x)Bi1/2Na1/2TiO3-xBaTiO3. J Appl Phys. 2012;111(1):014105.