ABSTRACT
Lead (Pb) is a neurotoxicant that still remains ahealth problem despite many efforts to minimize its levels in the environment. , The use of medicinal plants in the treatment of many diseases and different toxic agents has become popular due to their effectiveness and lower costs. The Adansonia digitata L. (AD) fruit is called a super fruit because of its exotic nature and rich nutrient profile with several medicinal and antioxidants - properties. This study was designed to explore the optional protectivity of Adansonia digitata L. fruit pulp aqueous extract against lead-induced memory impairment, oxidative stress and brain damage. Thirty adult male Wistar rats were distributed into five groups: control, Pb 30 mg/kg, AD 250 mg/kg plus Pb, AD 500 mg/kg plus Pb and Succimer 10 mg/kg plus Pb. Administrations were through oral gavage once daily for 42 days. Lead administration caused memory impairment, increased concentration of glutamate in brain and induction of oxidative stress. AD-treated groups protected memory impairment, reduced glutamate concentration, prevented oxidative stress and ameliorated histopathological changes in the brain. It was concluded that Adansonia digitata ameliorates lead-induced memory impairment in Wistar rats by improving the memory index, controlling glutamate concentration, preventing oxidative stress.
Graphical abstract
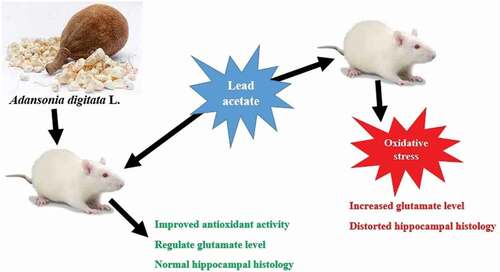
Introduction
An increase in the number of industries as a result of development has led to heavy metals accumulation on land, air and water; the heavy metals include lead, mercury and nickel [Citation1]. Lead (Pb) is one of the heavy metals that constitutes numerous environmental and health hazards despite efforts to minimize its environmental level [Citation2]. The brain is a vital organ mostly affected by lead; the adult brain is less susceptible to lead toxicity compared to children [Citation3–5]. However, some reports showed that lead is a neurodevelopmental toxicant that also affect adults with more burden in pregnant women [Citation6]. Reports from previous studies suggest that exposure to lead disrupts the glutathione system [Citation7,Citation8], causing oxidative stress [Citation9,Citation10] and inducing neuronal cell death [Citation11,Citation12].
Because of its strong affinity to protein kinase C (PKC), Pb displaces Ca2+, binds to PKC and activates it under normal physiologic conditions; the binding affects the normal functions of PKC thereby affecting neurotransmitters like glutamate and other second-messenger systems, leading to changes in gene expression as well as protein synthesis [Citation13].
Glutamate is the most abundant excitatory neurotransmitter; it activates more than 50% of the brain synapses and is responsible for learning and memory [Citation14]. Previous studies report that Pb exposure can influence glutamate signaling that plays an important role in neuronal degeneration and cognition [Citation15,Citation16]. In the brain, astrocytes carry glutamate through excitatory amino acid transporter-2 (EAAT-2) and glutamate transporter-1 (GLUT-1) in humans and rodents, respectively [Citation17,Citation18]. Glutamate acts on ionotrophic receptors to enhance ion entry into cells that eventually trigger intracellular signaling. N-methyl-d-aspartate (NMDA), an ionotrophic receptor, plays an important role in the progress of neurodegenerative diseases. NMDA binds to glutamate and prompts ion influx at post-synaptic membrane, thereby linking pre-synaptic ad post-synaptic activation [Citation19,Citation20]. Lead was reported to affect glutamate by selective blockage of the N-methyl-D-aspartate (NMDA) receptor, responsible for neuronal plasticity and development [Citation13].
The Adansonia digitata L. (baobab) fruit is called a super fruit because of its exotic nature and rich nutrient profile including vitamin C, pectin, potassium, calcium, flavonoids, sterols/triterpens, tannins, saponins, coumarins, glycosides, reducing sugar, lignin, carbohydrates and antioxidants [Citation21,Citation22]. The vitamin C content of baobab fruit pulp is about 10 times the values found in oranges, and this confers the antioxidant properties of baobab fruit enabling it to prevent oxidative stress [Citation23,Citation24]. Antioxidant prevents oxidative stress related diseases such as inflammation, cancer, neurodegenerative and cardiovascular diseases [Citation25,Citation26]. The purpose of the present study was to evaluate the role of Adansonia digitata L. fruit pulp on lead-induced memory impairment by assessing the memory index, determining glutamate concentration and oxidative stress in rats. In addition, the study also evaluated the histology and histochemistry of rats’ brain through H&E and Bielschowsky stains, respectively.
Material and methods
Plant extraction
Adansonia digitata fruit pulp was ground, soaked in distilled water and filtered. The filtrate was oven dried at 40°C. Percentage yield of 5.5% was obtained and was calculated using the formula: % yield = (weight of AD fruit pulp/weight of extract) x 100%
Animals treatment
Thirty (30) Wistar rats (120–160 g) were obtained from the Faculty of Pharmacy, Ahmadu Bello University (ABU), Zaria, Nigeria. The rats were acclimatized to the Department of Human Anatomy animal house for 14 days. They had free access to feed (Grower mash, Grand Cereal, Nigeria) and water. The research was approved by the ABU Zaria Research and Ethical Committee (ABUCAUC/2018/064).
Experimental design
The rats were divided into five groups (n = 6). Group I (control rats) received distilled water at 1 ml/kg. Group II received lead acetate at 30 mg/kg. Group III received AD extract at 250 mg/kg) + lead acetate (30 mg/kg). Group IV received AD extract at 500 mg/kg + lead acetate (30 mg/kg). Group V received succimer at 10 mg/kg [Citation27] + lead acetate (30 mg/kg). Note that 250 mg/kg and 500 mg/kg represent 5 and 10% LD50 of the extract, respectively [Citation28]. All administrations were done through oral gavage daily for 6 weeks.
Behavioral test
The Morris water maze (MWM) test for spatial memory and learning was carried out as described by [Citation29,Citation30]. Briefly, during the training phase, rats were placed on a platform for 20 sec and then lowered into the pool. The time taken for each rat to locate the platform (escape latency) was recorded. Any rat that could not locate the platform after 60 sec was guided to the platform and 60 sec was allocated to the rat. During the test phase, the pool was filled with water 1 inch above the platform and rats were lowered into the water at one quadrant. The test finishes when the rat finds the platform or after 60 sec. Rats that could not locate the platform within 60 sec were placed on the platform for 15 sec and then removed from the tank. The rats were than tested for three trials per day and the latency of each animal was recorded. Rats were tested every two weeks, escape latency on last day of training (day 5) before treatment was considered as an index of learning.
The probe test was also carried out on the same groups of rats 24 hours after the escape latency test. The test was performed 3 consecutive times the average computed. Briefly, the platform was removed, and the time spent by the rat in the target quadrant, where the platform was previously placed, was recorded. The average time spent by each rat in the target quadrant searching for the hidden platform was considered as an index of memory [Citation31].
Histological examination
At the end of the study, all the rats were euthanized with ketamine injection, and the brain of each rat was divided into two halves. One half was fixed in 10% neutral buffered formalin, processed for light microscopy and stained with H&E for general histology and Bielschowsky stain for neuronal processes [Citation32,Citation33].
Glutamate analysis
The other halves of the brain were weighed and homogenized in phosphate buffer (pH 7.4) at 1 g:5 ml. The homogenate was centrifuged at 3000 rpm for 20 min, and the supernatant was used for glutamate analysis and biochemical analysis. Glutamate concentration was measured using Rat Glutamate Elisa Kit: CK-bio-20422 (Biotech Limited, Shanghai, China) according to manufacturer’s instruction.
Biochemical analysis
Malondialdehyde (MDA) was determined by quantifying the lipid peroxidation (LPO) level from the supernatant using the protocols of [Citation34]. The reaction contained tissue homogenates, 5% (w/v) butylated hydroxytoluene (BHT), 10% TCA and 0.75% TBA in 0.1 mol/L of HCl. Values are expressed in μmol/mg of tissue. Catalase (CAT) activity was analyzed using the method of [Citation31] in a solution containing tissue homogenates, 50 mM phosphate buffer and 19 mM hydrogen peroxide. Glutathione peroxidase (GPx) activity was assayed according to the method described by [Citation35] in a solution containing tissue homogenates, sodium phosphate buffer, 10 mM sodium azide, 4 mM GSH and 2.5 mM H2O2. Superoxide dismutase (SOD) activity was determined using the protocol of [Citation36, Citation37]. The solution includes tissue homogenates, 0.05 carbonate buffer and 0.3 mM epinephrine. Values are expressed in IU/mg of tissue
Statistical analysis
Data were analyzed using GraphPad Prism 7. One-way analysis of variance (ANOVA) followed by the Bonnferoni post-hoc test was used. The results were presented as mean ± standard error of mean (SEM), and p < 0.05 was considered statistically significant.
Results
Cognitive ability
Lead treated rats showed significant increase in latency when compared to the control (P < 0.05) with respect to the pre-treatment period. Rats treated with AD extract plus lead and succimer plus lead showed significant reduction in latency when compared to the lead-treated group at P < 0.05 (). For the probe test, lead-treated rats showed significant decrease in memory index when compared to the control group (P < 0.05). Groups treated with AD extract plus lead and succimer plus lead showed significant increase in memory index when compared to the lead-treated group at P < 0.05 ().
Histopathological studies
Hippocampal architecture CA1 and CA3 areas of the control showed closely packed, linearly arranged pyramidal cells with a well-defined shape (). By contrast, architectural distortion especially in the pyramidal layer and cell loss with cellular disarray were observed in the Pb-treated group; the group also showed cytoplasmic features suggestive of cell death (). Distortions were markedly reduced in the AD250mg + Pb, AD500mg + Pb, and DMSA + Pb groups compared to the control group. These groups showed a nearly similar arrangement of cells with an intact nucleus and well-defined boundaries with occasional areas having few randomly arranged cells exhibiting mild reactive neurodegenarative changes such as necrosis and some levels of neuronal losses ().
Figure 2. Composite photomicrograph of CA1 and CA3 hippocampal subfields of the brain of rats treated with Pb, AD + Pb and DSMA + Pb showing normal pyramidal cells in A and F, mild distortion in C, E and H and necrosis and neuronal loss in B, D, G, I and J. H and E × 400
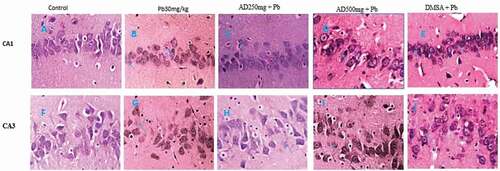
Bielschowsky stain showed normal neuronal organization and well-organized neuronal processes in the control group (). By contrast, architectural distortion and neuronal processes degeneration were observed in Pb-treated rats (). Mild distortion and loss of the neuronal processes were noticed in the CA1 region of AD250mg + Pb, AD500mg + Pb and DMSA + Pb-treated rats () when compared with the control group. Degeneration and severe neuronal loss were observed in the CA3 region of Pb, AD250mg + Pb, AD500mg + Pb and DMSA + Pb treated ().
Glutamate concentration
Glutamate concentration increased significantly in lead-treated rats when compared with the control (p < 0.05) group. There was a significant decrease in the glutamate concentration of rats treated with AD250 + Pb, AD500 + Pb and DMSA10 + Pb when compared with that of lead-treated rats at p < 0.05 ().
Malondialdehyde level
MDA levels increased significantly in all the treated groups when compared to the control group (P < 0.05). A significant decrease in the MDA was observed in rats treated with AD + Pb, and DMSA + Pb when compared with rats treated with lead only ().
Antioxidant enzymes
Catalase activity
CAT activity decreased significantly (P < 0.05) in all the treated groups when compared to the control group. A significant increase in tissue CAT activity was observed in AD + Pb and DMSA + Pb treated groups when compared with that of lead only group at P < 0.05 ().
Glutathione peroxidase activity
GPx activity decreased significantly in all the treated groups when compared to the control (P < 0.05). More so, there was also a significant (P < 0.05) increase in tissue GPx activity in AD + Pb and DMSA + Pb treated groups when compared with that of the lead only group ().
Superoxide dismutase activity
SOD activity decreased significantly in all the treated groups when compared to the control group (P < 0.05). There was a significant increase in SOD activity in AD + Pb and DMSA + Pb treated groups when compared with the lead only group at P < 0.05 ().
Discussion
The present study reported memory impairment, oxidative stress and increased glutamate concentration in brain of lead treated rats. Lead was reported as a poisonous heavy metal that can cross the blood–brain barrier and induces several neurological damages because of its ability to displace calcium ions [Citation2]. The present study suggests that Adansonia digitata L. fruit pulp aqueous extract ameliorates memory impairment caused by lead and prevents oxidative stress due to its antioxidant properties. Previous studies have shown that Adansonia digitata contains several bioactive constituents with strong antioxidant capabilities [Citation22,Citation24]. Increased oxidative stress due to Pb toxicity observed in this study supports the other finding that reported memory impairment and oxidative stress as a result of lead toxicity [Citation38]. The beneficial effect of Adansonia digitata on memory improvement is believed to be mediated through its action as a potent antioxidant that results from its direct scavenging activity of free radicals, thereby preventing oxidative stress [Citation23]. Previous work has shown the use of arbutin extract as an antioxidant in stabilizing the antioxidant system in the cells and was crucial for the protection of the brain from chemical-induced damage as such enhancing learning and memory performance in MWM [Citation39]. Numerous studies in animals and humans have confirmed the involvement of oxidative damage resulting in the production of reactive oxygen species (ROS), leading to peroxidation of cell membrane lipids, disrupting the integrity and function of the cell membrane and causing cell death due to lead-induced neurotoxicity [Citation40]. Some studies have supported the ROS-mediated damage to the cell membrane and the depletion of antioxidant reserves as the possible mechanism of lead-induced oxidative stress, and other studies believed that direct formation of ROS including singlet oxygen, H2O2, and hydroperoxides is another possible mechanism for lead-induced oxidative damage [Citation41].
Our study showed that groups treated with AD significantly reduced the level of MDA, but increased the activities of SOD, CAT and GPx in the brain and was similar to the DMSA-treated group when compared with the Pb-treated group. This antioxidant property effect could be attributed to the presence of some bioactive compounds such as flavonoids, phenols and ascorbic acid. Research has shown that flavonoids and ascorbic acid have antioxidant properties such as scavenging free radicals, preventing lipid peroxidation and neuroprotective role [Citation42].
Glutamate is the most abundant excitatory neurotransmitter in the CNS involved in neuronal transmission, development, differentiation and plasticity [Citation43]. However, excess accumulation of glutamate leads to abnormal depolarization of neurons, resulting in excitotoxicity and neuronal cell death [Citation44]. The significant increase in glutamate concentration of Pb-treated rats was attributed to the fact that Pb can inhibit glutamate uptake by astrocyte at the synaptic cleft. Lead toxicity was reported to affect astrocytes functions by depolarizing the cell membrane, resulting in calcium in entry into post-synaptic cell and affecting normal neurotransmission [Citation45,Citation46]. Notably, our study has showed that AD and DMSA modulated glutamate concentration in lead-treated rats. Adansonia digitata L. modulates glutamate concentration by increasing cellular uptake to maintain low extracellular glutamate. Low levels of extracellular glutamate increases neurotransmission, and glutamate has no extracellular metabolism. Therefore, a low level of glutamate can be achieved by improving cellular uptake [Citation47]. Adansonia digitata L. protects neurons by preventing cell membrane depolarization while the antioxidant role chelate Pb and prevents its excess accumulation in the brain to allow astrocytes function effectively. The vitamin C content of AD might also prevent cell membrane depolarization. Similarly, some plants with antioxidant potentials like Lion’s Mane were reported to control glutamate concentration and prevent excitotoxicity [Citation48]. It is evident that lead toxicity distorts the histological architecture of brain, resulting in several neurodegenerative changes and hence affecting its function [Citation2]. The ameliorative role of AD in brain architecture could be linked to its antioxidant property and the presence of phenolic compounds. Plants with antioxidant properties have been shown to protect the brain structure against lead toxicity [Citation38].
Conclusion
Adansonia digitata L. fruit pulp at 250 mg/kg and 500 mg/kg was shown to ameliorate lead-induced brain damage by preventing neuronal cell membrane depolarization to maintain low extracellular glutamate and chelating Pb. These promote neurotransmission, thereby improving the memory index and preventing neurodegeneration. Therefore, Adansonia digitata L. fruit pulp could be used in the treatment and prevention of neurodegenerative diseases.
Ethics declaration
The research was approved by ABU Zaria Research and Ethical Committee (ABUCAUC/2018/064).
Disclosure statement
No potential conflict of interest was reported by the author(s).
Additional information
Funding
References
- Omamuyovwi MI, Stephen OO, Olayemi KI, et al. Sub-acute nickel exposure impairs behavior, alters neuronal microarchitecture, and induces oxidative stress in rats’ brain. Drug Chem Toxicol. 2018. DOI:10.1080/01480545.2018.1437173
- Pramod KS, Manish KS, Rajesh SY, et al. Omega-3 fatty acid attenuates oxidative stress in cerebral cortex, cerebellum, and hippocampus tissue and improves neurobehavioral activity in chronic lead-induced neurotoxicity. Nutr Neurosci. 2017. DOI:10.1080/1028415X.2017.1354542.
- Mahmoud YI, Sayed SS. Effects of L-cysteine on lead acetate induced neurotoxicity in albino mice. Biotech Histochem. 2016;91(5):327–332.
- Liu J, Lewis G. Environmental toxicity and poor cognitive outcomes in children and adults. J Environ Health. 2014;76(6):130–138.
- Lidia S, Małgorzata C, Grzegorz S. The role of astroglia in Pb-exposed adult rat brain with respect to glutamate toxicity. Toxicology. 2005;212(2–3):185–194.
- Hamid AS, Gamal SA, Hesham NM, et al. Thymoquinone ameliorates oxidative damage and histopathological changes of developing brain neurotoxicity. J Histotechnol. 2019. DOI:10.1080/01478885.2019.1619654.
- Bokara KK, Blaylock L, Denise SB, et al. Influence of lead acetate on glutathione and its related enzymes in different regions of rat brain. J Appl Toxicol. 2009;29(5):452–458.
- Omobowale TO, Oyagbeml AA, Akinrinde AS, et al. Failure of recovery from lead induced hepatotoxicity and disruption of erythrocyte antioxidant defense system in Wistar rats. Environ Toxicol Pharmacol. 2014;37(3):1202–1211.
- Bokara KK, Brown E, Mc Cormick R, et al. Lead induced increase in antioxidant enzymes and lipid peroxidation products in developing rat brain. Biometals. 2008;21:9–16.
- Lee JC, Son YO, Pratheeshkumar P, et al. Oxidative stress and metal carcinogenesis. Free Radic Biol Med. 2012;53(4):742–757.
- Mousa AM, Al-Fadhli AS, Rao MS, et al. Gestational lead exposure induces developmental abnormalities and up regulates apoptosis of fetal cerebellar cells in rats. Drug Chem Toxicol. 2015;38(1):73–83.
- Mujaibel LM, Kilarkaje N. Mitogen activated protein kinase signaling and its association with oxidative stress and apoptosis in lead exposed hepatocytes. Environ Toxicol. 2015;30(5):513–529.
- Djebli N, Slimani M, Aoues A. Effect of lead exposure on dopaminergic transmission in the rat brain. Toxicology. 2005;28207(3):363–368.
- Garcia-Arenaz G, Ramirez-Amaya V, Balderas I, et al. Cognitive deficits in adult rats by lead intoxication are related with regional specific inhibition of cNOS. Behav Brain Res. 2004;149:49–59.
- Sharifi AM, Baniasadi S, Jorjani M, et al. Investigation of acute lead poisoning on apoptosis in rat hippocampus in vivo. Neurosci Lett. 2002;329(1):45–48.
- Vazquez A, Pena de Ortiz S. Lead (Pb2+) impairs long-term memory and blocks learning-induced increases in hippocampal protein kinase C activity. Toxicol Appl Pharmacol. 2004;200:27–39.
- Melone M, Bellesi M, Conti F. Synaptic localization of GLT-1a in the rat somatic sensory cortex. Glia. 2009;57(1):108–117.
- Guerriero RM, Giza CC, Rotenberg A. Glutamate and GABA imbalance following traumatic brain injury. Curr Neurol Neurosci Rep. 2015;15(5):27.
- Paula-Lima AC, Brito-Moreira J, Ferreira ST. Deregulation of excitatory neurotransmission underlying synapse failure in Alzheimer’s disease. J Neurochem. 2013;126(2):191–202.
- Featherstone RE, Shin R, Kogan JH, et al. Mice with subtle reduction of NMDA NR1 receptor subunit expression have a selective decrease in mismatch negativity: implications for schizophrenia prodromal population. Neurobiol Dis Elsevier B.V 2015;73:289–295.
- Jitin R, Manish KJ, Shishu PS, et al. Adansonia digitata L. (baobab): a review of traditional information and taxonomic description. Asian Pac J Tropical Biomedicine. 2015;5(1):79–84.
- Ayat AA, Shimaa AA, Sahar HE, et al. The effect of blending of extracts of Sudanese Adansonia digitata and Tamarindus indica on their antioxidant, anti-inflammatory and antimicrobial activities. J Pharmacogn Phytother. 2019;11(2):28–34.
- Mona AG, Amal IH, Manal GM, et al. Protective effect of Adansonia digitata against isoproterenol-induced myocardial injury in rats. Anim Biotechnol. 2016;27(2):84–95.
- Keyla R, Margarida M, Ana P, et al. Effect of Adansonia digitata L.fruit on postprandial glycaemia in nondiabetic adults. Ann Med. 2019;51(1):159.
- Monica HC, Bente LH, Kari H, et al. The total antioxidant content of more than 3100 foods, beverages, spices, herbs and supplements used worldwide. Nutr J. 2010;9(3):16.
- Kaur C, Kapoor HC. Antioxidants in fruits and vegetables-the Millennium’s health. Int J Food Sci Technol. 2001;36:703–725.
- Salisu MH, Raji I, Musa A, et al. Amelioration of lead toxicity in selected organs and tissue of wistar rats using resveratrol. Dutse J Pure Appl Sci. 2020;6:1.
- Muhammad IU, Jarumi IK, Alhassan AJ, et al. Acute toxicity and hypoglycemic activity of aqueous fruit pulp extract of Adansonia digitata L. (Afpead) on Alloxan induced diabetic rats. Journal of Adv Med Pharm Sci. 2016;6(3):1–6.
- Drapeau E, Mayo W, Aurousseau C, et al. Spatial memory performances of aged rats in the water maze predict levels of hippocampal neurogenesis. Proc Nat Acadamy Sci: Elodie Drapeau. 2003;100(24):14385–14390.
- Maryam B, Iran G, Kataneh A, et al. Effect of postnatal chronic lead exposure on spatial learning and memory in male rat. Zahedan J Res Med Sci. 2015;17(9):1050.
- Nunez J. Morris water maze experiment. JoVE. 19. 2008 Accessed20 05 2020. http://www.jove.com/index/Details.stp?ID=897
- Suvarna SK, Layton C, Bancroft JD. Bancroft’s theory and practice of histological techniques. 7th ed. Nottingham: Churchill Livingstone; 2013.
- Yan L, Jianli Y, Yaowen C, et al. Hippocampal neurochemical changes in senescent mice induced with chronic injection of D-Galactose and NaNO2: an in vitro high-resolution NMR spectroscopy. PLoS ONE. 2014;9(2):e88562.
- Farombi EO, Tahnteng JG, Agboola AO. Chemoprevention of 2-acetylaminofluoreneinduced hepatotoxicity and lipid peroxidation in rats by kolaviron - a Garcinia kola seed extract. Food Chem Toxicol. 2000;38(6):535–541.
- Clairborne A. Catalase activity. In: Greewald AR, editor. Handbook of methods for oxygen radical research. Boca Raton (Florida): CRC; 1995. p. 237–242.
- Rotruck JT, Pope AL, Ganther HE, et al. Selenium: biochemical role as a component of glutathione peroxidase. Science. 1973;179(4073):588–590.
- Misra HP, Fridovich I. The role of superoxide anion in the auto-oxidation of epinephrine and a simple assay for superoxide dismutase. J Biol Chem. 1972;247(10):3170–3175 Accessed04 03 2021.
- Sheharbano B, Syed -Adnan AS, Touqeer A, et al. Neuroprotective effects of Foeniculum vulgare seeds extract on lead-induced neurotoxicity in mice brain. Drug Chem Toxicol. 2018. DOI:10.1080/01480545.2018.1459669
- Zohreh D, Mahdi P, Maryam GK, et al. Arbutin reduces cognitive deficit and oxidative stress in animal model of Alzheimer’s disease. Int J Neurosci. 2019. DOI:10.1080/00207454.2019.1638376.
- Al Omar SY, Alkhuriji AF, Ahmed EA, et al. The neuroprotective role of Coenzyme Q10 against lead acetate-induced neurotoxicity is mediated by antioxidant, anti-inflammatory and anti-apoptotic activities. Int J Environ Res Public Health. 2019;16:2895.
- Lopes AC, Peixe TS, Mesas AE, et al. Lead exposure and oxidative stress: a systematic review. Rev Environ Contam Toxicol. 2016;236:193–238.
- Valencia F, Dilip S, Kiran K, et al. Neuroprotective effects of Silibinin: an in-silico and in-vitro study. Int J Neurosci. 2018. DOI:10.1080/00207454.2018.1443926
- Mi Hye K, Ju-Sik M, Joon YL, et al. Oleuropein isolated from Fraxinus rhynchophylla inhibits glutamate-induced neuronal cell death by attenuating mitochondrial dysfunction. Nutr Neurosci. 2017. DOI:10.1080/1028415X.2017.1317449.
- Kritis AA, Stamoula EG, Paniskaki KA, et al. Researching glutamate-induced cytotoxicity in different cell lines: a comparative/collective analysis/study. Front Cell Neurosci. 2015;9:91.
- Arne S, Susanna S, Lasse KB, et al. Glutamate metabolism in the brain focusing on astrocytes. Adv Neurobiol. 2014;11:13–30.
- Kandel E. Principles of Neural Science. Vol. 5. Kandel ER, Schwartz JH, and Jessell TM, et al. (Editors), McGraw Hill Professional. 2013:210–306 Accessed04 03 2021.
- Zhou Y, Danbolt NC. GABA and glutamate transporters in brain. Front Endocrinol (Lausanne). 2013;4(165):1–14.
- Phan CW, David P, Naidu M, et al. Therapeutic potential of culinary-medicinal mushrooms for the management of neurodegenerative diseases: diversity, metabolite, and mechanism. Crit Rev Biotechnol. 2015;35(3):355–368.