ABSTRACT
Selenium one of the most important trace elements exhibits diverse biological functions. Particularly in fishes, it plays a protective role and enhances growth performances. The green synthesized selenium nanoparticles have added benefits including ease of availability, economic, safe, etc. Furthermore, the development of multidrug-resistant pattern among pathogens in general and aquatic pathogens in particular has raised the demand for a potential substitute for regularly used antibiotics . Hence, this study was intended to synthesize selenium nanoparticles in a green route method from sodium selenite salt by using the plant Blumea axillaris and to evaluate their antibacterial activity against aquatic pathogens. The findings of this study indicated that the stem and root of B. axillaris were capable of synthesizing selenium nanoparticles with an optimised salt concentration of 0.1 M. Further both the stem and root extracts synthesized selenium nanoparticles were found to inhibit pathogens. While aquatic pathogens exhibited multidrug resistance pattern against many commonly used drugs, the green synthesized selenium nanoparticles were found to effectively control these pathogens. However, the detailed study on the mechanism of inhibition is going on which in turn can be a very cheap, safe, and economical way of enhancing aquaculture industry.
Introduction
Nanotechnological interventions are emerging as the new science and technology platform [Citation1,Citation2]. Particularly, with dimensions ranging in nanometer scale (1–100 nanometers, i.e. 1 × 10−9M) and presence of certain unique features like high surface area, small size, surface charge, surface chemistry, solubility, etc. [Citation3–5], nanoparticles have tremendous potential for next generation of development and transformation of agri-food systems [Citation1,Citation2]. Above all, in aquaculture sector, nanotechnology has a very wide range of application including water treatment, sterilization of ponds, detection and control of pathogens, efficient delivery of drugs, better nutrition, etc [Citation5,Citation6].
The current intensive aquaculture practices often cause crowding stress followed by increased risk of diseases [Citation7]. On the other hand, the use and overuse of antibiotics in aquaculture results in various adverse consequences such as residual effect, development of resistant bacteria, etc [Citation8–11]. Therefore, this issue raises concern regarding the prophylactic and therapeutic use of antimicrobials employed to prevent stock losses resulting from disease conditions [Citation8]. Further due to several advantages including tissue specific-targeting, dose reduction, and increased bioavailability of drug [Citation12,Citation13], nanoparticles are currently being explored as efficient antimicrobial agents. Besides, the development of resistance pattern among the pathogens has led to the exploration of nanotechnology as a potential substitute for routinely used antibiotics in aquaculture as well [Citation14]. Earlier, metal and/or metal oxide based nanoparticles belonging to copper, zinc, titanium, silver etc have already been demonstrated to be effective against different pathogens [Citation15]. However, toxicity is the most important issue associated with the use of such nanoparticles [Citation16–20]. Conversely, the antimicrobial potency of safe elements like selenium could be advantageous [Citation21].
Selenium being a micronutrient has been demonstrated to execute beneficial effects in fish in both elemental and nanoform [Citation15,Citation22,Citation23]. Further it’s antimicrobial effect as well as role in drug delivery has also been observed [Citation4,Citation21,Citation24–32]. Therefore, nowadays much emphasis is given to use selenium especially in nanoform in the health management strategy of fish. Selenium nanoparticles (Se NPs) are mostly prepared by chemical reduction methods using different reducing agents and stabilizers; radiolytic reduction, sonochemical methods, etc [Citation33,Citation34]. But such preparation methods have certain disadvantages due to use of hazardous chemicals, ultrasound, radiation, etc and the toxicity and biological interference ability of certain stabilizers often restricts their applications in biological system [Citation35]. Therefore, a simple alternative, low cost and eco-friendly biosynthesis method of stable Se NPs preparation with biomedical applications is still a challenge [Citation35,Citation36]. In recent years, the synthesis of nanoparticles using plant extracts has been a possible alternative to chemical and physical methods [Citation37]. Despite, limited attempts have been made not only to synthesize Se NPS in green route method using certain plants but also to explore their antimicrobial potential against pathogens in general and aquatic pathogens in particular.
Looking into the fact that diseases are the major stumbling block in sustainable aquaculture practices due to significant increase in both onset of infectious diseases and emergence of multidrug resistance among the aquatic pathogens, the present study was intended to evaluate the antibacterial activity of green synthesized Se NPs against bacterial pathogens. While Asteraceae family is known for their wide range of bioactive potency, Blumea axillaris (Lam.) an annual herb of this family with anti-inflammatory, antioxidant, anticancer, antimicrobial, antiparasitic activities, etc and Southeast Asia, south of Sahara and South America distribution [Citation38–40] has not been explored earlier to synthesize Se NPs. Therefore, the present study was conducted to synthesize Se NPs from the aqueous extracts of stem and roots of B. axillaris and to evaluate their antibacterial activity against certain multidrug resistant bacterial pathogens associated with disease conditions of fish.
Materials and Methods
Pathogens used
Different primary and secondary pathogens viz., Aeromonas hydrophila (Virulent), Aeromonas hydrophila (Moderately virulent), Pseudomonas aeruginosa, E. coli, Aeromonas, Enterococcus, Salmonella, and Shigella species isolated from disease affected fish and maintained at Department of Biotechnology, MSCB University, Baripada, Odisha, India were used in this study.
Preparation of stem and root extracts of Blumea axillaris
B. axillaris plant was collected from the Similipal Biosphere Reserve (Located 21°10°–22°12° N latitude and 85°58°–86°42° E longitude), Mayurbhanj, Odisha, India. After collection the whole plants were brought to the laboratory. The stem and root of the plant were separated in the laboratory and washed gently with running tap water and then with distilled water followed by air drying. After complete drying, the dried individual parts (stem and root) were mixed with equal amount of water to make a 1:1 solution and were separately crushed using a mortar and pestle. The paste was then centrifuged at 2,500 × g for 10 min and the supernatant was collected in sterile containers to synthesize nanoparticles.
Synthesis of selenium nanoparticles (Se NPs)
The Se NPs were synthesized as per the following procedure using sodium selenite as precursor. Briefly, 10 ml of sodium selenite (0.1 M, 0.01 M, and 0.001 M) was maintained separately at 50°C on a hot plate magnetic stirrer. This preheated salt solution was separately mixed with the extracts of different parts of the plant of varying amounts ranging from 1 ml to 5 ml while stirring. Stirring was continued till the change in color was observed. Further the solutions were kept on an orbital shaker and observed at an interval of 24 h.
Characterization of Se NPs
The green synthesized Se NPs were characterized by measuring the UV-visible spectra of the synthesized solutions within 300–700 nm range. The morphological features of the B. axillaris root and stem extracts synthesized Se NPs were further characterized by scanning electron microscope (SEM). First of all, the synthesized Se NPs were sonicated with distilled water and then one drop from each sample was placed on clean glass slide. After drying, the sample was sputter coated with a thin layer of 60% gold and 40% platinum for 30 sec with 45 mA current. The morphological features of the synthesized Se NPs were observed on SEM (JEOL JSM T220A MA).
Similarly. Dynamic Light Scattering (DLS) was done to measure the hydrodynamic effective diameters of synthesized Se NPs. A Dynamic Light Scattering Particle size analyzer (Malvern instruments, USA) was used to obtain the exact values of the mean particle size, Zeta potential and Polydispersity index (PDI) for the synthesized Se NPs.
Antibiotic sensitivity assay
The antibiotic sensitivity assay of bacterial pathogens against different antibiotics {Ampicillin (10 mcg); Bacitracin (10 units); Cefotaxime (30 mcg); Chloramphenicol (30 mcg); Co-trimoxazole, (25 mcg); Erythromycin (15mcg); Oxytetracycline (30 mcg); Norfloxacin (10 mcg); Nitrofurantoin (300 mcg); Novobiocin (30 mcg); Penicillin (10 units); Tetracycline (30 mcg); Vancomycin (30 mcg) (Hi-Media, India)} was performed as per standard microbiological method [Citation41]. Briefly, the overnight grown individual pathogen was separately lawn cultured on Muller Hinton agar plates and then antibiotic discs were firmly placed into the seeded agar plates. Finally plates were incubated at 30°C for 24 h and after incubation, the zone of inhibition was measured and interpreted as sensitive, resistant, and intermediate sensitive.
Evaluation of antibacterial activity
Antimicrobial activity of Se NPs was determined by disc diffusion technique as per standard protocol [Citation41]. Briefly overnight grown bacterial culture in nutrient broth (OD540 = 0.5) was lawn cultured on nutrient agar plates. 20 µl of sample containing 40 µg of the synthesized Se NPs was loaded in discs of the culture and then the plates were incubated at 30°C for 12 h after which, the zone of inhibition was recorded. Based on the findings of inhibition zone after incubation (conducted in triplicate), the results were interpreted either as positive or negative.
Results
Organoleptic characterization of Se NPs
The synthesis of the Se NPs was confirmed by a change in color to brick-red. Solutions containing stem and root extracts of B. axillaris plant indicated the synthesis of Se NPs after keeping them on the orbital shaker for a period of 24–36 h. Further three different salt (sodium selenite) concentrations were used, i.e. 0.1 M, 0.01 M, and 0.001 M. Both stem and root extracts of B. axillaris synthesized Se NPs from 0.1 M sodium selenite . On the contrary, salts with 0.01 M as well as 0.001 M had no and/or poor synthesis of Se NPs. In case of 0.001 M sodium selenite, none of the plant parts exhibited synthesis of Se NPs as no significant color change was observed. Similarly at higher concentration of sodium selenite, i.e. 0.1 M, there was a very poor development of color for the root extract indicating very less synthesis of Se NPs. In case of the stem extract the color change was very slow and though the final color became reddish, it was not much prominent.
Characterization of green synthesized Se NPs
Preliminary characterization of stem and root extracts synthesized Se NPs was done by UV-visible spectrophotometry. Se NPs synthesized from both the extracts exhibited a peak at 420 nm (). The SEM analysis of the B. axillaris root and stem extracts synthesized Se NPs revealed dense, roughly spherical and aggregated forms. While the diameters in the size range of B. axillaris stem extract synthesized Se NPs appeared to be little more with around 400 nm, it was homogenous and less sized (≈ 300 nm) in case of B. axillaris root extract synthesized Se NPs ( & ). Similarly DLS analysis of Se NPs synthesized from root extract of B. axillaris showed heterogenous particle formation with least percentage of particles (39%) having a particle size of 248.1 nm. On the other hand, B. axillaris stem extract synthesized Se NPs were homogenous with 96.5% of the particles having a size of 346.6 nm ( & ).
Figure 1. UV-VIS spectra of selenium nanoparticles synthesized from stem and root extracts of Blumea axillaris.
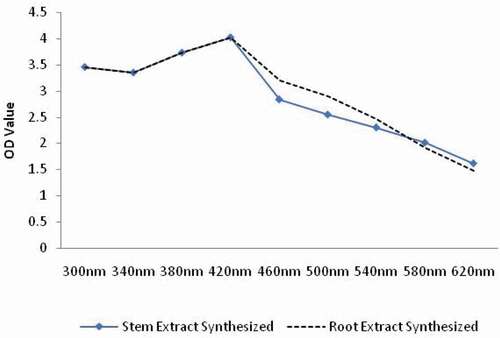
Antibiotic sensitivity assay
Antibiotic sensitivity assay study revealed the multidrug resistance pattern among the primary and secondary aquatic pathogens. All the pathogens were found to be resistant to a minimum of five antibiotics and the resistance pattern among the pathogens is shown in . All the bacteria were found to be resistant toward ampicillin, erythromycin and novobiocin. Apart from these antibiotics, both P. aeruginosa and E. coli isolates were resistant toward tetracyclin, oxytetracycline and bacitracin. Similarly all bacteria, except Aeromonas species, P. aeruginosa, and E. coli isolates showed resistance toward co-trimoxazole and tetracycline. On the other hand both the virulent and moderately virulent A. hydrophila pathogens were resistant to ampicillin, erythromycin, novobiocin, vancomycin, and co-trimoxazole. Aeromonas species also showed a similar type of resistance pattern against ampicillin, erythromycin, novobiocin, vancomycin, and bacitracin.
Table 1. The bacterial pathogens associated with fish diseases showing resistance pattern toward commonly used antibiotics
Antibacterial activity of Se NPs
The green synthesized Se NPs from both the stem and root extracts of B. axillaris plant exhibited antibacterial activity against all the tested bacteria. On the other hand, both the root and stem extracts were found to be noninhibitory against any of the isolates. Both stem and root extracts synthesized Se NPs inhibited Aeromonas species with an inhibitory zone size of 1.8 cm and 2.6 cm, respectively. Similarly, B. axillaris stem and root extracts synthesized Se NPs inhibited A. hydrophila (moderate), with inhibitory zone size of 2.8 cm and 3.0 cm, respectively, while it was 1.0 cm and 1.5 cm against A. hydrophila (virulent) by stem and root extracts synthesized Se NPs, respectively ().
Figure 4. Zone of inhibition (in cm) of green synthesized selenium nanoparticles from stem and root extracts of B. axillaris against the pathogenic bacteria of fish.
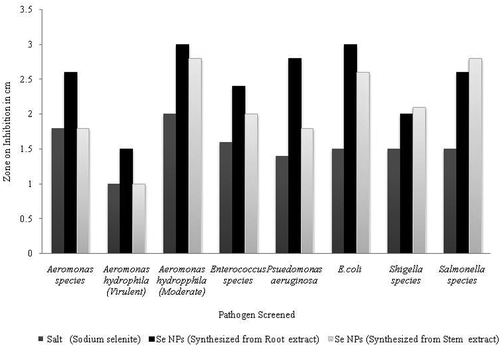
Se NPs synthesized from stem extract of B. axillaris showed a zone size of 2.0 cm and those synthesized from root extract showed a zone size of 2.4 cm against Enterococcus species. Further, stem extract synthesized Se NPs showed a zone size of 1.8 cm and the root extract synthesized Se NPs showed a zone size of 2.8 cm against P. aeruginosa. Similarly stem and root extracts synthesized Se NPs exhibited a zone size of 3 cm and 2.6 cm, respectively against E. coli. Similarly in case of Salmonella species the salt solution had a zone size of 1.8 cm. The root and stem extracts synthesized Se NPs exhibited a zone size of 2.6 cm and 2.8 cm against Salmonella species, respectively. Likewise, the stem extract and root extracts synthesized Se NPs exhibited a zone size of 2.1 cm and 2 cm against Shigella species, respectively.
Discussion
Nowadays, the emergence of multidrug resistance phenomena is a serious issue and possesses potential threats not only to aquatic but also to terrestrial animals including human beings [Citation42]. In aquaculture, indiscriminate use of antibiotics has led to the emergence of multidrug resistance phenomena among several bacterial pathogens [Citation43,Citation44]. However, recent nanotechnological interventions with metal and nonmetal nanoparticles have drawn a remarkable attention as antimicrobial agents to replace antibiotics use. Amongst, Se NPs have gained wide attraction due to their specific medicinal, chemical, biological and pharmaceutical properties. Hence this study was focused on the green synthesis of Se NPs from B. axillaris and assessment of its antibacterial activity against certain selected primary and secondary pathogenic bacteria. Earlier few attempts have been made to green synthesize Se NPs using plants like Aloe vera, Vitis vinifera, Allium sativum etc [Citation45–47]. Herein in this study, Se NPs from B. axillaris were successfully synthesized in a green synthesis manner. With respect to synthesis of Se NPs the stem of B. axillaris was more efficient than root. The particles distribution was not uniform along with their size.
The green synthesized Se NPs using this plant were found to exhibit antibacterial activity against some of the selected bacteria of aquatic origin. Earlier, studies have shown the multidrug resistance pattern among the aquatic bacteria. Bacteria from the Enterobacteriaceae as well as the Gram positives have also been reported to exhibit multidrug resistance pattern [Citation48]. Aeromonas species are pathogenic to several aquatic organisms in general and particularly in case of fishes, are known as important fish opportunistic pathogens [Citation49,Citation50]. Many fish species like carp, tilapia, rainbow trout, brown trout, eel, perch, catfish, goldfish, and salmon pose a great threat from Aeromonas species since it is responsible for disease and mortality of fishes [Citation51–54]. A. hydrophila, which is a pathogenic bacterium, has been implicated in fish and animals including human beings [Citation55–57]. The multidrug resistance pattern has already been reported earlier from tilapia (Oreochromis niloticus) isolates [Citation58]. Multidrug resistance pattern has also been found in Aeromonas -species against antibiotics like ampicillin, clindamycin and penicillin [Citation59] and particularly in A. hydrophila, multidrug resistance pattern has been found against ampicillin and streptomycin [Citation60]. Herein in this study, both the pathogenic isolates of A. hydrophila were found to be resistant to ampicillin, erythromycin, novobiocin, vancomycin, and co-trimoxazole. Aeromonas species also showed a similar type of resistance pattern against ampicillin, erythromycin, novobiocin, vancomycin, and bacitracin. Other isolates like E. coli, Salmonella, Shigella, Enterococcus species also exhibited multidrug resistance pattern. Earlier studies on these bacteria have also demonstrated such type of multidrug resistance pattern [Citation61–64].
Selenium was known to be a very essential element within living systems for the last 40 years. However it is important biological and biochemical features has emerged only during past few decades [Citation65]. Further selenium exerts diverse biological roles within living systems. Furthermore, it also exhibits significant role in fishes by improving their immunity [Citation66], growth [Citation67], flesh color and texture [Citation68], etc. However, toxicity could be an issue [Citation69] but the green synthesized Se NPs when injected to Indian major carp, rohu (Labeo rohita) fingerlings at 40 µg per fish did not show any abnormality and mortality (Unpublished Data). Earlier antibacterial activity of Se NPs against various aquatic pathogens has been reported [Citation15,Citation23]. It was observed that among the green synthesized Se NPs , those from the root and stem are effective against all the tested pathogens. Further this approach of synthesizing Se NPs proves to be a simple, clean and environment friendly approach as stated in various literatures. Hence this plant can be used to synthesize antimicrobial Se NPs . But as it is evident from the above data, there is certain heterogeneity in the size of the synthesized nanoparticles formed and therefore the green synthesis of Se NPs from B. axillaris requires further standardization.Though the exact mechanism of it’s working against the microbes is not well known, it may be able to create some osmotic imbalance or may be capable of degrading some important biochemical bonds in the microbes [Citation69]. However, more in-depth studies are going on to establish the detailed mode of action.
Acknowledgments
Authors are thankful to the Head, Department of Biotechnology, Maharaja Sriram Chandra Bhanja Deo University (Erstwhile North Orissa University), Baripada, Odisha, India for providing necessary permission and facilities to carry out the research work.
Disclosure statement
No potential conflict of interest was reported by the author(s).
Additional information
Funding
References
- Kumari A, Yadav SK. Nanotechnology in agri-food sector. Crit Rev Food Sci Nutr. 2014;54(8):975–984.
- Rodrigues SM, Demokritou P, Dokoozlian N, et al. Nanotechnology for sustainable food production: promising opportunities and scientific challenges. Environ Sci Nano. 2017;4:767–781.
- Shah B, Mraz J. Advances in nanotechnology for sustainable aquaculture and fisheries. Rev Aquac. 2019 12 ;925–942.
- Khurana A, Tekula S, Saifi MA, et al. Therapeutic applications of selenium nanoparticles. Biomed Pharmacother. 2019;111:802–812.
- Bhattacharya A, Janardana Reddy S, Mahbub Hasan M, et al. Nanotechnology: a unique future technology in aquaculture for food security. Int J Bioassays. 2015;04: 4115–4426.
- Huang S, Wang L, Liu L, et al. Nanotechnology in agriculture, livestock, and aquaculture in China: A review. Agron Sustain Dev. 2015;35(2):369–400.
- ECDC (European Centre for Disease Prevention and Control), EFSA (European Food Safety Authority), and EMA (European Medicines Agency) ECDC/EFSA/EMA second joint report on the integrated analysis of the consumption of antimicrobial agents and occurrence of antimicrobial resistance in bacteria from humans and food-producing animals – Joint Interagency Antimicrobial Consumption and Resistance Analysis (JIACRA) Report. EFSA J. 2017;15(7):e04872.
- Cabello F, Godfrey H, Tomova A, et al. Antimicrobial use in aquaculture re examined: it’s relevance to antimicrobial resistance and to animal and human health. Environ Microbiol. 2013 15 1917–1942 .
- Cabello FC. Heavy use of prophylactic antibiotics in aquaculture: a growing problem for human and animal health and for the environment. Environ Microbiol. 2006;8(7):1137–1144.
- Ryu SH, Park SG, Choi SM, et al. Antimicrobial resistance and resistant genes in Escherichia coli strains isolated from commercial fish and sea food. Int J Food Microbiol. 2012;152(1–2):14–18.
- Done HY, Venkatesan AK, Halden RU., et al. Does the recent growth of aquaculture create antibiotic resistance threats different from those associated with land animal production in agriculture? AAPS J. 2015;17(3):513–524.
- Toyokawa H, Nakao A, Bailey RJ, et al. Relative contribution of direct and indirect allorecognition in developing tolerance after liver transplantation. Liver Transpl. 2008;14(3):346–357.
- Xu W, Huang K, Jin W, et al. Catalytic and anti-bacterial properties of biosynthesized silver nanoparticles using native inulin. RSC Adv. 2018a;8(50):28746–28752.
- Zhang L, Pornpattananangkul D, Hu CMJ, et al. Development of nanoparticles for antimicrobial drug delivery. Curr Med Chem. 2010;17(6):585–594.
- Swain P, Das R, Das A, et al. Effects of dietary zinc oxide and selenium nanoparticles on growth performance, immune responses and enzyme activity in rohu, labeo rohita (Hamilton). Aquac Nutr. 2018 25 ;486–494.
- Malhotra N, Ger TR, Uapipatanakul B, et al. Review of copper and copper nanoparticle toxicity in fish. Nanomaterials. 2020 10 :1126.
- Wang T, Long XH, Cheng Y, et al. The potential toxicity of copper nanoparticles and copper sulphate on juvenile Epinephelus coioides. Aquat Toxicol. 2014;152:96–104.
- Buerki-Thurnherr T, Xiao L, Diener L, et al. In vitro mechanistic study towards a better understanding of ZnO nanoparticle toxicity. Nanotoxicology. 2013;7(4):402–416.
- Blinova I, Ivask A, Heinlaan M, et al. Ecotoxicity of nanoparticles of CuO and ZnO in natural water. Environ Pollut. 2010;158(1):41–47.
- Franklin NM, Rogers NJ, Apte SC, et al. Comparative toxicity of nanoparticulate ZnO, bulk ZnO, and ZnCl2 to a fresh water micro alga (Pseudokirchneriella subcapitata): the importance of particle solubility. Environ Sci Technol. 2007;41(24):8484–8490.
- Tran PA, Webster TJ. Selenium nanoparticles inhibit staphylococcus aureus growth. Int J Nanomedicine. 2011;6:1553–1558.
- Khan KU, Zuberi A, Nazir S, et al. Effects of dietary selenium nanoparticles on physiological and biochemical aspects of juvenile tor putitora. Turk J Zool. 2016; 40: 704–712.
- Anf N-A, Mahmoud EA, Hakim YAE., et al. Efficacy of dietary nano-selenium on growth, immune response, antioxidant, transcriptomic profile and resistance of Nile tilapia, Oreochromis niloticus against streptococcus iniae infection. Fish Shellfish Immunol. 2019;94:280–287.
- Wang CL, Lovell RT, Klesius PH., et al. Response to edwardsiella ictaluri challenge by channel catfish fed organic and inorganic sources of Selenium. J Aquat Anim Health. 1997;9(3):72–179.
- Zheng W, Cao C, Liu Y, et al. Multifunctional polyamidoamine-modified selenium nanoparticles dual-delivering siRNA and cisplatin to A549/DDP cells for reversal multidrug resistance. Acta Biomater. 2015;11:368–380.
- Mary TA, Shanthi K, Vimala K, et al. PEG functionalized selenium nanoparticles as a carrier of crocin to achieve anticancer synergism. RSC Adv. 2016;6(27):22936–22949.
- Zhao S, Yu Q, Pan J, et al. Redox-responsive mesoporous selenium delivery of doxorubicin targets MCF-7 cells and synergistically enhances its anti-tumor activity. Acta Biomater. 2017;54:294–306.
- Nugroho RA, Fotedar R. Dietary organic selenium improves growth, survival and resistance to vibrio mimicus in cultured marron, Cherax cainii (Austin, 2002). Fish Shellfish Immunol. 2013;35(1):79–85.
- Le KT, Fotedar R. Immune responses to vibrio anguillarum in yellowtail kingfish, Seriola lalandi, fed Selenium supplementation. J World Aquacult Soc. 2014;5:138148.
- Baidya S, Murthy HS. Effect of organic selenium on growth, immune response and resistance of labeo rohita to Aeromonas hydrophila infection. Int J Fish Aquat Stud. 2017;5(5):243–246.
- Chiu ST, Hsieh SL, Yeh SP, et al. The increase of immunity and disease resistance of the giant freshwater prawn, Macrobrachium rosenbergii by feeding with selenium enriched-diet. Fish Shellfish Immunol. 2010;29(4):623–629.
- Wang Q, Webster TJ. Short communication: inhibiting biofilm formation on paper towels through the use of selenium nanoparticles coatings. Int J Nanomedicine. 2013;8:407–411.
- Li X, Li Y, Li S, et al. Single crystalline trigonal selenium nanotubes and nanowires synthesized by sonochemical process. Cryst Growth Des. 2005;5(3):911–916.
- Sauvaire Y, Baissac Y, Leconte O, et al. Steroid saponins from fenugreek and some of their biological properties. Adv Exp Med Biol. 1996;405:37–46.
- Sowndarya P, Ramkumar G, Shivakumar MS., et al. Green synthesis of selenium nanoparticles conjugated clausena dentata plant leaf extract and their insecticidal potential against mosquito vectors. Artif Cells Nanomed Biotechnol. 2017;45(8):1490–1495.
- Ramamurthy CH, Sampath KS, Arunkumar P, et al. Green synthesis and characterization of selenium nanoparticles and its augmented cytotoxicity with doxorubicin on cancer cells. Bioprocess Biosyst Eng. 2012;36(8):1131–1139.
- Cui D, Liang T, Sun L, et al. Green synthesis of selenium nanoparticles with extract of hawthorn fruit induced HepG2 cells apoptosis. Pharm Biol. 2018;56(1):528–534.
- Senthilkumar A, Kannathasan K, Venkatesalu V., et al. Chemical constituents and larvicidal properties of the essential oil of blumea mollis (D. Don) Merr. against culex quinquefasciatus. Parasitol Res. 2008;103(4):959–962.
- Sreelekha KP, Ajeesh Krishna TP, Adarsh Krishna TP, et al. Pharmaco-chemical characterization of leaves of blumea mollis (D. Don) merr.- from Western Ghats of Wayanad region of Kerala, India. J Pharmacogn Phytochem. 2017;6(4): 319–323.
- Sivanandhan S, Ganesan P, Jackson A, et al. Activity of some medicinal plants against phytopathogenic fungi. Int Res J Biol Sci. 2018;5(5):124–137.
- Bauer AW, Kirby WMM, Sherris J, et al. Antibiotic susceptibility testing by a standardized single disc method. Am J Clin Pathol. 1966;45(4_ts):493–496.
- Da Costa PM, Loureir L, Augusto J, et al. Transfer of multidrug-resistant bacteria between intermingled ecological niches: the interface between humans, animals and the environment. Int J Env Res Pub Health. 2013;10(1):278–294.
- Nikaido, H Multidrug Resistance in Bacteria Ann Rev Biochem. 2009;78(1):119–146.
- Ryu SH, Park SG, Choi SM, et al. Antimicrobial resistance and resistance genes in Escherichia coli strains isolated from commercial fish and sea food. Int J Food Microbiol. 2011;152(1–2):14–18.
- Sharma G, Sharma AR, Bhavesh R, et al. Biomolecule-mediated synthesis of selenium nanoparticles using dried Vitis vinifera (raisin) extract. Molecules. 2014;19(3):2761–2770.
- Anu K, Singaravelu G, Murugan K, et al. Green-synthesis of selenium nanoparticles using garlic cloves (Allium sativum): biophysical characterization and cytotoxicity on Vero cells. J Cluster Sci. 2017;28(1):551–563.
- Fardsadegh B, Jafarizadeh-Malmiri H. Aloe vera leaf extract mediated green synthesis of selenium nanoparticles and assessment of their in vitro antimicrobial activity against spoilage fungi and pathogenic bacteria strains. Green Pro Syn. 2019;8(1):399–407.
- Resende JA, Silva VL, Fontes CO, et al. Multidrug-resistance and toxic metal tolerance of medically important bacteria isolated from an aquaculture system. Microbes Environ. 2012;27(4):449–455.
- Kozińska A, Pękala A. Serotyping of Aeromonas species isolated from polish fish farms in relation to species and virulence phenotype of the bacteria. Bull Vet Inst Pulawy. 2010;54:315–320.
- Hu M, Wang N, Pan ZH, et al. Identity and virulence properties of Aeromonas isolates from diseased fish, healthy controls and water environment in China. Lett Appl Microbiol. 2012;55(3):223–224.
- Orozova P, Chikova V, Najdenski H., et al. Antibiotic resistance of pathogenic for fish isolates of Aeromonas spp. Bulg J Agric Sci. 2010;16:376–386.
- Hossain MJ, Sun D, McGarey DJ, et al. An Asian origin of virulent Aeromonas hydrophila responsible for disease epidemics in United States-farmed catfish. mBio. 2014;(3). DOI:10.1128/mBio.00848-14.
- Stratev D, Daskalov H, Vashin I., et al. Characterisation and determination of antimicrobial resistance of β-haemolytic Aeromonas spp. isolated from common carp (Cyprinus carpio L.). Revue Méd Vét. 2015;166:54–61.
- Mulyani Y, Aryantha NP, Suhandono S, et al. Intestinal bacteria common carp (Cyprinus carpio L) as a biological control agent for Aeromonas. J Pure Appl Microbiol. 2018;12(2):601–610.
- Chan FK, Ching JY, Ling TK, et al. Aeromonas infection in acute suppurative cholangitis: review of 30 cases. J Infect. 2000;40(1):69–73.
- Chim H, Song C. Aeromonas infection in critically ill burn patients. Burns. 2007;33(6):756–759.
- Okumura K, Shoji F, Yoshida M, et al. Severe sepsis caused by Aeromonas hydrophila in a patient using tocilizumab: a case report. J Med Case Rep. 2011;5(1):499.
- Castillo CS, Hikima JI, Jang HB, et al. Comparative sequence analysis of a multidrug-resistant plasmid from Aeromonas hydrophila. Antimicrob Agents Chemother. 2013;57(1):120–129.
- Zdanowicz M, Mudryk ZJ, Perliński P., et al. Abundance and antibiotic resistance of Aeromonas isolated from the water of three carp ponds. Vet Res Commun. 2020;44(1):9–18.
- Son R, Rusul G, Sahilah AM, et al. Antibiotic resistance and plasmid profile of Aeromonas hydrophila isolates from cultured fish, Tilapia (Tilapia mossambica). Lett Appl Microbiol. 1997;24(6):479–482.
- Adenaike O, Olonitola OS, Ameh JB, et al. Multidrug resistance and multiple antibiotic resistance index of Escherichia coli strains isolated from retailed smoked fish. J Nat Sci Res. 2016 6 :2225–2921.
- Nair Divek VT, Kumar V, Anup K., et al. Antibiotic-resistant salmonella in the food supply and the potential role of antibiotic alternatives for control. Foods. 2018;7(10):167.
- Shohreh F, Reza R, Mojtaba A, et al. Emergence of multi drug resistant strains of Escherichia coli isolated from urinary tract infection Open Conf Proc J. 2010. Vol. 1, p. 192–196.
- Sekhar GSS, Sv R, Shylaja M, et al. The detection and antimicrobial susceptibility profile of shigella isolates in and around Hyderabad, Telangana. Pharm Innov. 2018;7(2):84–88.
- Taylor D, Dalton C, Hall A, et al. Recent developments in selenium research. Br J Biomed Sci. 2009;66(2):107–116.
- Lin YH, Shiau SY. The effects of dietary selenium on the oxidative stress of grouper, Epinephelus malabaricus, fed high copper. Aquaculture. 2007;(1–4). DOI: 10.1016/j.aquaculture.2006.12.015.
- Nazari K, Shamsaie M, Eila N, et al. The effects of different dietary levels of organic and inorganic selenium on some growth performance and proximate composition of juvenile rainbow trout (Oncorhynchus mykiss). Iran J Fish Sci. 2016;16(1):238–251.
- Lyons MSD. Organic selenium as a supplement for Atlantic salmon: effects on meat quality. In: Lyons TP, and Jacques KA, editors. Biotechnology in the feed industry. Proceedings of Alltech’s 14th Annual Symposium: passport to the year 2000 Nottingham University Press, Nottingham, UK; p. 505–508.
- Sarkar B, Bhattacharjee S, Daware A, et al. Selenium nanoparticles for stress-resilient fish and livestock. Nanoscale Res Lett. 2015;10(1):371.