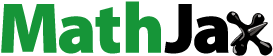
ABSTRACT
The word order that is easiest to understand in a language generally coincides with the word order most frequently used in that language. In Kaqchikel, however, there is a discrepancy between the two: the syntactically basic VOS incurs the least cognitive load, whereas SVO is most frequently employed. This suggests that processing load is primarily determined by grammatical processes, whereas word order selection is affected by additional conceptual factors. Thus, the agent could be conceptually more salient than other elements even for Kaqchikel speakers. This hypothesis leads us to the following expectations: (1) utterance latency should be shorter for SVO sentences than for VOS sentences; (2) Kaqchikel speakers should pay more attention to agents than to other elements during sentence production; and (3) despite these, the cognitive load during sentence production should be higher for SVO than for VOS. A Kaqchikel sentence production experiment confirmed all three expectations.
1. Introduction
Languages differ in terms of the order in which the subject (S), the object (O), and the verb (V) are aligned in sentences. In English, for example, most transitive declarative sentences follow the SVO order, and the other orders are either quite rare or impossible. In other languages, word order is more flexible with multiple possibilities being allowed. Japanese employs SOV and OSV; all the six logically possible orders are actually used in Finnish; and so on. It has been observed, in many flexible as well as rigid word order languages, that the syntactically basic word order is easier to process than the other grammatically possible word orders (derived word orders) (Bader & Meng, Citation1999 for German, Kaiser & Trueswell, Citation2004 for Finnish, Kim, Citation2012 for Korean, Mazuka, Itoh, & Kondo, Citation2002 and Tamaoka et al., Citation2005 for Japanese, Sekerina, Citation1997 for Russian, Tamaoka, Kanduboda, & Sakai, Citation2011 for Sinhalese).Footnote1 In Japanese, for example, sentences with the syntactically basic SOV order are processed faster than comparable OSV sentences according to various psycholinguistic studies using sentence plausibility judgment tasks (Chujo, Citation1983; Tamaoka et al., Citation2005), self-paced reading (Koizumi & Imamura, Citation2017; Shibata et al., Citation2006), and eye tracking (Mazuka et al., Citation2002; Tamaoka et al., Citation2005). Neurolinguistic studies have also shown a similar processing advantage of the syntactically basic word order. Functional magnetic resonance imaging (fMRI) studies have found a higher activation of the left inferior frontal gyrus (IFG) while processing derived word orders when compared to basic word orders (Grewe et al., Citation2007 for German, Kim et al., Citation2009 and Kinno, Kawamura, Shioda, & Sakai, Citation2008 for Japanese). Furthermore, studies with event-related potentials (ERPs) have revealed that relative to syntactically basic word orders, derived word orders elicit a late positivity effect called P600 and/or (sustained) anterior negativity (Erdocia, Laka, Mestres-Missé, & Rodriguez-Fornells, Citation2009 for Basque, Rösler, Pechmann, Streb, Roeder, & Hennighausen, Citation1998 for German, Ueno & Kluender, Citation2003 and Hagiwara, Soshi, Ishihara, & Imanaka, Citation2007 for Japanese).
This basic word order advantage has also been observed in sentence production, as syntactically basic word orders are more frequently used than are derived word orders. For instance, Imamura and Koizumi (Citation2011) reported that among Japanese transitive sentences with a nominal subject and a nominal object in the corpus of novels they studied, more than 97% followed the SOV word order. This shows that although Japanese is said to be “a free word order language” or “a flexible word order language”, the SOV order is strongly preferred to the OSV order in sentence production. Higher rates of basic word order utterances have been reported in many other flexible languages as well (Slobin & Bever, Citation1982 for Turkish and Serbo-Croatian, Hakulinen & Karlsson, Citation1980 for Finnish, Bates, Citation1976 for Italian).
As articulated by Tamaoka and Koizumi (Citation2006), one possible interpretation of this correlation between the syntactically basic word order, the word order easiest to understand, and the word order most frequently employed has to do with syntactic complexity. The syntactically basic word order in a language, by definition, is associated with simpler syntactic structures than the other grammatically possible orders in that language. It is, therefore, less demanding in terms of working-memory load, and is hence easier to process (cf. Gibson, Citation2000; Hawkins, Citation2004; Marantz, Citation2005; O’Grady, Citation1997; Pritchett & Whitman, Citation1995; Tamaoka et al., Citation2005). That is, syntactic complexity affects the processing load in the sense that the former partially determines the latter. Processing load, in turn, affects production frequency because, all other things being equal, a structure that is easier to process is more likely to be used than one which is more difficult to process. As speakers of the language are more proficient in sentence structures and words that are used frequently, they are more likely to process these with greater speed and accuracy, and hence, production frequency also affects processing load. This model is schematically shown in .
Figure 1. Relationship among syntax, processing, and frequency. (Adopted from Tamaoka & Koizumi, Citation2006).
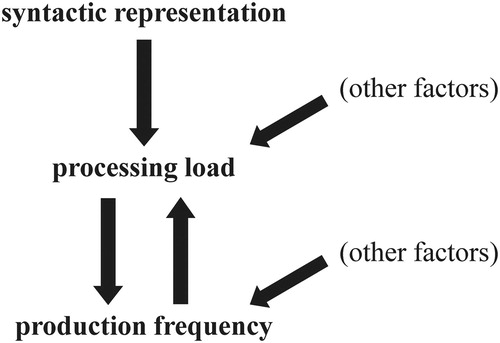
In some languages, however, there is a discrepancy between these three kinds of word orders (Brody, Citation1984; Koizumi et al., Citation2014). Kaqchikel, a Mayan language spoken in Guatemala, is a case in point. It is a head-marking and morphologically ergative language in which subjects and objects are not overtly case-marked for grammatical relations. Rather, grammatical relations are obligatorily marked on predicates, for example, a verb with two sets of agreement morphemes, one set for a transitive subject and another for a transitive object and an intransitive subject. The word order of Kaqchikel is relatively flexible, and all the logically possible six word orders are grammatically allowed. Among these, VOS is considered the basic word order of Kaqchikel by many Mayan language researchers (Ajsivinac Sian, García Matzar, Cutzal, & Alonzo Guaján, Citation2004; Tichoc et al., Citation2000; García Matzar & Rodríguez Guaján, Citation1997; Rodríguez Guaján, Citation1994). SVO is another commonly used word order. For the purpose of the present study, we assume the schematic syntactic structures shown in (1) below, in which VOS is structurally simpler than the other orders.
Order Schematic syntactic structure
VOS [VOS]
SVO [Si [VO gapi]]
VSO [[V gapi S] Oi]
OVS [Oi [V gapi S]]
Sentence comprehension studies with behavioural indices (Kiyama, Tamaoka, Kim, & Koizumi, Citation2013; Koizumi et al., Citation2014), fMRI (Koizumi & Kim, Citation2016), and ERPs (Yano, Yasunaga, & Koizumi, Citation2017; Yasunaga, Yano, Yasugi, & Koizumi, Citation2015) have all observed that the syntactically basic VOS is easier to process than SVO and other derived word orders in Kaqchikel. Despite the fact that VOS is the syntactically basic word order and the easiest to understand among grammatically possible word orders in Kaqchikel, it is the SVO order that is most frequently used in this language (England, Citation1991; Kubo, Ono, Tanaka, Koizumi, & Sakai, Citation2015; Maxwell & Little, Citation2006; Rodríguez Guaján, Citation1994). According to Kubo et al. (Citation2015), for instance, of all the sentences with a transitive verb and nominal subject and object produced in their sentence-production experiment with a picture-description task, sentences with the SVO, VOS, and VSO orders constituted 74.4%, 24.2%, and 1.4%, respectively. In fact, not just in Kaqchikel but in many other Mayan languages as well, word orders in which subjects are preposed appear more frequently than the syntactically determined basic word order. The question is why this should be the case at all.
Koizumi et al. (Citation2014) suggested that three conceivable factors combined together contribute toward the higher production ratio of SVO sentences.Footnote2 The first has to do with the head-marking nature of Kaqchikel. As mentioned earlier, Kaqchikel is a head-marking language that exhibits subject and object agreement markers on the verb. The verbal complex of a transitive sentence contains information on the person and the number of the subject and object. Therefore, in Kaqchikel, having a verbal complex in the sentence-initial position may be advantageous in that it helps develop predictions of the upcoming subject and object, rendering the processing of the subsequent portion of the sentence easier. In case of sentence production, in contrast, verb-initial word orders in Kaqchikel may be more disadvantageous than nominal-initial word orders such as SVO. This is because in order to initiate a sentence with a verbal complex, conceptual and grammatical information on the subject and object must have been activated and processed to a certain degree, prior to the beginning of the utterance (). For this reason, therefore, subject-initial sentences may be easier to initiate than verb-initial sentences for Kaqchikel speakers, and hence, SVO is produced more frequently than VOS and VSO.
A second possible factor for the preference of SVO in sentence production is concerned with the “saliency of subjects.” There are two interrelated but different ways in which relative conceptual saliencies affect word order selection in sentence production. First, conceptual factors may directly affect word order selection (De Smedt, Citation1990; Kempen & Hoenkamp, Citation1987; Myachykov & Tomlin, Citation2008; Tanaka, Branigan, McLean, & Pickering, Citation2011). The order of word retrieval from the mental lexicon at the stage of grammatical processing may be determined by the availability of individual concepts at the stage of conceptual processing, and the structure of the sentence being generated is accordingly constrained by whichever word is retrieved first. In other words, conceptually more accessible entities may claim early word order positions irrespective of grammatical function. In this respect, SVO is preferred over VOS in Kaqchikel partly because of the relatively higher conceptual saliency of the agent directly influencing the word order of utterances in Kaqchikel. Second, conceptual factors may also influence the way in which grammatical functions are assigned, which, in turn, indirectly affects word order choice through the mediation of the grammar of a particular language (Bock & Warren, Citation1985; Lee, Brown-Schmidt, & Watson, Citation2013; McDonald, Bock, & Kelly, Citation1993). It has been observed in many languages that subjects tend to become topics of conversation more easily than other immediate sentence constituents, and topics tend to appear at the beginning of sentences. Indeed, in Mayan languages, constituents that appear before verbs are often interpreted as the topic of the utterance, and the observation that there is a syntactically designated position for topic before verbs is widely supported (Aissen, Citation1992; England, Citation1991; García Matzar & Rodríguez Guaján, Citation1997). This means that although VOS is syntactically the basic word order and induces a lower processing load, SVO is used more frequently in conversation because the agent, being conceptually salient, is likely to be assigned to the grammatical roles of subject and topic, and a topicalized subject, in turn, is placed in the preverbal position according to the grammar of Kaqchikel (Tichoc et al., Citation2000; Ajsivinac Sian et al., Citation2004).
Finally, the “similarity-based competition” may contribute to the frequency of SVO. Gennari, Mirković, and MacDonald (Citation2012) argued that when there is a temporal overlap in the planning of two conceptually similar nouns, the similarity leads to interference between the semantic information of the nouns. As a result, when the concept of one noun is activated, the concept of the other noun is inhibited, and the latter is mentioned away from the initially activated noun, or simply omitted in the sentence. Moreover, the effect of conceptual similarity interacts with language-specific grammatical constraints, and the actual instantiation may vary across languages. Kubo et al. (Citation2015) examined how similarity-based competition influences speakers’ choices of sentence patterns in Kaqchikel. The production of VOS sentences is interesting because the most accessible element, an animate agent noun usually realised as the subject, must be retained in memory until the end of the sentence, and hence, it potentially competes with other elements. If similarity-based competition arises between the subject and object in Kaqchikel, one of them must be realised away from the other. Since the object usually follows the verb in Kaqchikel, the increase in competition would lead to the decrease of VOS word order. Kubo et al. (Citation2015) conducted two picture description experiments to verify this prediction. In these experiments, the animacy of the patient noun was manipulated (human, animal, inanimate object) such that similarity between the agent (human) and patient varied among conditions. The results showed that VOS sentences were produced more often with an inanimate patient than with an animal or human patient, as predicted by similarity-based competition. Speakers of Kaqchikel, therefore, seem to be sensitive to the competition caused by the similarity of noun concepts involved in an event described in the sentence. They select the sentence pattern in order to resolve competition between nouns with similar concepts.
The above considerations taken together lead us to the following expectations. First, if subject-initial sentences are easier to initiate than verb-initial sentences in Kaqchikel because of its head-marking nature and the conceptual saliency of the agent, then utterance latency should be shorter for SVO than for VOS in Kaqchikel. Second, if the agent is conceptually more salient than other entities even for speakers of VOS languages, Kaqchikel speakers should pay more attention to agents than to other elements during sentence production. Third, despite these, the cognitive load during sentence production should be higher for SVO sentences than for VOS sentences because the production of a sentence surely includes, as its central part, the construction of linguistic representations, and the grammatical processes involved in this are presumably similar to those involved in the comprehension of a parallel sentence, although there may be some differences (Momma & Phillips, Citation2018). Additionally, it is interesting to observe how the animacy of the patient may or may not influence time course and cognitive load in Kaqchikel sentence production. In particular, the similarity-based competition in the sense of Gennari et al. (Citation2012) would incur some cognitive load in VOS sentences compared to SVO sentences. This factor should impede the production processes of VOS compared to SVO especially when the subject and object are similar to each other (e.g. both are human). If this is so, we would expect the interaction of the effect of syntactic complexity and that of similarity-based competition: the difference between SVO and VOS, in cognitive load, should be smaller when the event is reversible (i.e. the human agent is acting on the human patient) than when the event is non-reversible (i.e. the human agent acting on the inanimate patient).
In this study, we report a sentence production experiment with a picture description task conducted to test these predictions by measuring the utterance latencies, eye movements, and cortical activations of Kaqchikel speakers. The results confirmed all but the last expectation, as reported in detail below.
Before presenting the details of the current Kaqchikel production study, we will briefly discuss, as a basis for comparison, a parallel study we had conducted previously with Japanese speakers (Takeshima et al., Citation2014), and another relevant study on a Mayan language, Tzeltal, by Norcliffe, Konopka, Brown, and Levinson (Citation2015).
In the experiment reported in Takeshima et al. (Citation2014), participants (native speakers of Japanese) described pictures of simple transitive events involving familiar characters and actions while their gaze, speech, and brain activation were recorded. The results revealed that the utterance latency was shorter in the SOV condition (i.e. SOV sentences) than in the OSV condition (OSV sentences). The brain activities for the SOV order were lower compared with that for the OSV order at the left inferior frontal gyrus (IFG). In both conditions, the relative fixation times were higher for the agent than for the patient during the first 700 ms after the onset of the picture presentation (see ). The period of the first several hundred milliseconds (until around 400–600 ms) after picture onset arguably corresponded to event apprehension at the stage of conceptual processing (Griffin & Bock, Citation2000; Norcliffe et al., Citation2015, ; but see also Gleitman, January, Nappa, & Trueswell, Citation2007). Therefore, this pattern of eye movement confirmed the saliency and early processing of agent at the stage of conceptual processing in Japanese sentence production. To summarise, all the three indices converged to indicate the processing advantage of the syntactically basic word order in Japanese.
Figure 3. Relative fixation time during the picture presentation for (a) canonical SOV utterances and (b) scrambled OSV utterances in Japanese. The black vertical lines represent speech onset. (Adopted from Takeshima et al., Citation2014).
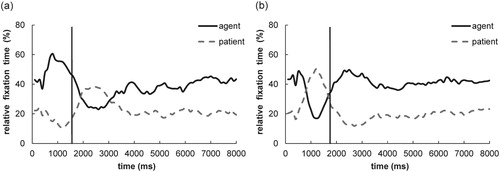
Norcliffe et al. (Citation2015) conducted a similar eye-tracked picture description experiment with native speakers of Tzeltal. They found an animacy effect on word order preference parallel to the findings in Kubo et al. (Citation2015): verb-initial word order was more frequent when the event was non-reversible compared to when the event was reversible. While producing SVO sentences Tzeltal speakers fixated preferentially on the first-mentioned character (i.e. the agent) before speech onset and fixated preferentially on the second-mentioned character (i.e. the patient) after speech onset. However, in the formulation of VOS orders, speakers’ attention and gaze were more evenly distributed across the two characters, with a smaller advantage of agent than while producing SVO orders.
We now turn to the Kaqchikel sentence production experiment.
2. Materials and methods
2.1. Participants
Eighteen native speakers of Kaqchikel (9 females and 9 males) participated in this experiment. Five participants, whose accuracy of word order was below 60%, were excluded from the final analysis. We, thus, eventually analyzed 13 participants (8 females, 5 males; mean age ± SD = 31.5 years ± 11.2). All of the 13 participants were Kaqchikel-Spanish bilinguals (age of acquisition of Spanish: 5.6 years ± 3.5), and spoke Kaqchikel as the primary language at home. All of them were classified as right-handed based on the Edinburgh Handedness Inventory (Oldfield, Citation1971), and had normal or corrected-to-normal vision. The present study was approved by the Ethics Committee of the Graduate School of Arts and Letters, Tohoku University. All participants provided written informed consent prior to their participation.
2.2. Apparatus
The experimental methods used were the same as those in the Japanese experiment reviewed above (Takeshima et al., Citation2014). During the experiment, the participants sat in front of an LCD display (Tobii; Tobii TX300 screen unit; resolution: 1024 × 768 pixels; refresh rate: 60 Hz). The viewing distance was approximately 60 cm. The experiment was controlled by E-Prime 2.0 with E-Prime Extensions for Tobii (Psychology Software Tools, Inc.) and a PC (Dell: Precision T7500). We used a voice recorder (Olympus: Voice Trek DS-850), an eye tracker (Tobii; Tobii TX300; sampling rate: 300 Hz), and a multi-channel NIRS system (Shimadzu: FOIRE-3000) to record the participants’ utterances, eye movements, and the relative concentration changes in oxygenated, deoxygenated, and total hemoglobin (oxy-Hb, deoxy-Hb, and total-Hb, respectively) in the blood. The NIRS instrument exploits the optimal properties of oxy-Hb and deoxy-Hb forms with different absorption spectra in the near infrared (NIR) wavelength region. By using two NIR wavelengths (780 and 830 nm) and applying data analyses based on the modified Lambert–Beer law, this instrument measures the above indices (Homae, Watanabe, Nakano, & Taga, Citation2007). A whole-head probe cap was used. The probes were placed over the prefrontal cortex of each hemisphere, each consisting of a 4 × 3 array with 6 emitters and 6 detectors, constituting 17 channels per hemisphere (see below). We arranged these probes in reference to the international 10–20 system: the locations of the 5th receiver and 15th emitter were arranged at T8 and T7, respectively. The sampling rate of each channel was 10 Hz.
2.3. Stimuli
We prepared 20 pictures (1024 × 724 pixels) depicting a two-character transitive event. Half the pictures showed human agents acting on human patients, and the other half showed human agents acting on inanimate patients. The agent was depicted on the left side and the patient on the right side. In order to counterbalance the locations, we also prepared 20 pictures that were mirror images of the originals. These 40 pictures were the same as those used in the previous Japanese study (Takeshima et al., Citation2014).
2.4. Procedure
The participants were given instructions in Kaqchikel by an experimenter who is a native speaker of Kaqchikel. Before the experiment, the participants observed the pictures provided as stimuli in a random order and described the events depicted in the pictures using transitive sentences in Kaqchikel. If the participants could not utter the content or if they interpreted the content incorrectly, the experimenter explained the picture. After this session, the participants were fitted with NIRS probes and the nine-point eye tracking calibration was performed.
Each trial began with the presentation of a fixation cross for 8000 ms, followed by an instructional display of word order for 5000 ms (). A short beeping sound was presented for 500 ms simultaneously with the onset of this instructional display to use in utterance latency analysis. The Kaqchikel word B’anöl “subject” or B’anoj “verb” was shown in the instructional display. After the instructional display, a picture was presented for 8000 ms. The participants were instructed to describe the event in the picture as concisely as possible in Kaqchikel, starting with the subject after the B’anöl display or the verb after the B’anoj display. Thus, it was expected that the participants would produce Kaqchikel sentences in the SVO and VOS orders after the B’anöl and B’anoj displays, respectively. The fixation cross appeared again after the picture display. The participants performed a total of 40 trials, with 20 pictures per word order. The agent was depicted on the left side in 10 pictures, and on the right side in the other 10 pictures.
2.5. Analysis
We excluded data from trials in which participants could not produce utterances of the instructed type. This led to the removal of 30.19% of all data.Footnote3
We calculated the utterance latency for each condition using Sound Engine (http://soundengine.jp/). The latency was defined as the interval between the onset of the picture presentation and the starting point of the participant’s utterance. Interjections, such as “Let’s see,” were not included in the utterance.
The gaze data were aggregated into 50 ms time bins. For each bin, we then calculated the probability of looks at the agent and patient during the time allotted to each picture (8 s) using area of interest (AOI) analysis. There was no significant difference in size between the AOI for agent and the AOI for patient (agent AOI = 168289.8 ± 39.55 pixels, patient AOI = 168281.2 ± 40.94 pixels, p = .54) ().
Figure 6. An example of AOI setting. The left AOI covers the agent area, and the right AOI covers the patient area, with the sizes of the two AOIs being comparable.
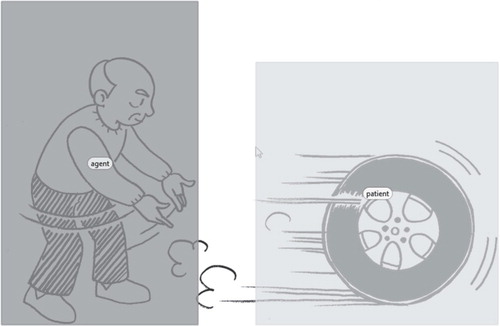
For the statistical analysis, we used a target advantage score (Kronmüller & Barr, Citation2015), which is typically defined as the probability of looks at the target object minus the probability of looks at the non-target object (see, for instance, Symeonidou, Dumontheil, Chow, & Breheny, Citation2016). Here, we designated the agent entity on a picture as the “target” and the patient entity as the “non-target,” so that a higher score indicated that the participant looked more at the agent entity than the patient.
We focused on oxy-Hb as an index of neural activation because it is more sensitive to changes in the regional cerebral blood flow than deoxy-Hb and total-Hb concentrations (Hoshi, Citation2003). The raw oxy-Hb data were high-pass filtered at 0.02 Hz to remove signal drift (Taga, Asakawa, Maki, Konishi, & Koizumi, Citation2003). Data were averaged for each participant and each condition and converted into z-scores (Otsuka et al., Citation2007; Schroeter, Zysset, Kruggel, & von Cramon, Citation2003), so that the mean values and standard deviations for the base line period (5 s before picture presentation) were 0 and 1, respectively. We averaged the oxy-Hb values during the picture presentation period (8000 ms). We analyzed the same channels as in the Japanese study, that is, channels 29, 30, 32, and 33, which were estimated as including the left dorsolateral prefrontal cortex and the left IFG. Some previous NIRS studies have reported that the oxy-Hb values in these regions were increased by the cognitive load in language-related tasks such as picture naming, word fluency, and sentence correctness judgment (Kovelman et al., Citation2009; Noguchi, Takeuchi, & Sakai, Citation2002; Watanabe et al., Citation1998).
3. Results
3.1. Utterance latency and duration
The utterance latency results are shown in . We conducted a two-way analysis of variance (ANOVA) with word order (2: SVO vs. VOS) and patient animacy (2: animate vs. inanimate) as the within-participant factors. The analyses revealed a significant main effect of word order (F (1, 12) = 18.37, p < .01, = .60), indicating that utterance latency was shorter in the SVO condition than in the VOS condition. On the other hand, the main effect of patient animacy (F (1, 12) = 0.03, p = .87,
= .00) and the interaction (F (1, 12) = 0.01, p = .93,
= .00) were not significant. In other words, utterance latency was not modulated by patient animacy.
Table 1. The means and standard deviations of utterance latency (ms) for word order and patient animacy conditions.
We also calculated the utterance durations and offset times ( and ). The utterance durations did not differ significantly among the different conditions: the main effect of word order (F (1, 12) = 0.58, p = .46, = .05), the main effect of patient animacy (F (1, 12) = 1.48, p = .25,
= .11), and the interaction (F (1, 12) = 0.13, p = .73,
= .01). As for the utterance offsets, a significant main effect of word order was found (F (1, 12) = 8.52, p < .05,
= .42), indicating that the utterances ended earlier in the SVO condition than in the VOS condition. On the other hand, neither the main effect of patient animacy (F (1, 12) = 1.09, p = .32,
= .08) nor the interaction (F (1, 12) = 0.05, p = .83,
= .00) was significant. This pattern of the utterance offset time parallels that of the utterance latency.
Table 2. The means and standard deviations of utterance duration (ms) for word order and patient animacy conditions.
Table 3. The means and standard deviations of utterance offset (ms) for word order and patient animacy conditions.
3.2. Eye movement
We analyzed the data for 6000 ms from picture onset because the mean utterance offsets were less than 6000 ms (see and ). The time courses of the target advantage score for each experimental condition are shown in .
Figure 7. Mean target advantage scores (the proportion of looks to the agent – the proportion of looks to the patient) for each experimental condition. The vertical dotted lines represent the boundaries of the time windows which were used for statistical analyses.
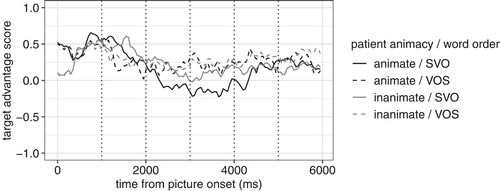
We divided the data into six 1000-ms time windows (see ) and conducted three-way by-participant (F1) and by-item (F2) ANOVAs, using word order (2), patient animacy (2), and time window (6: 0–1000 ms, 1000–2000 ms, 2000–3000 ms, 3000–4000 ms, 4000–5000 ms, 5000–6000 ms) as independent variables. The mean target advantage score for the corresponding 1000-ms time window was taken as the dependent variable. Greenhouse-Geisser corrections were used where applicable.
The ANOVAs demonstrated a significant main effect of word order (F1 (1, 12) = 20.05, p < .01, = .63; F2 (1, 18) = 6.80, p < .05,
= .27), a significant main effect of time window (F1 (5, 60) = 9.20, p < .01,
= .43; F2 (5, 90) = 11.43, p < .01,
= .39), and a significant interaction between word order and time window (F1 (5, 60) = 5.20, p < .01,
= .30; F2 (5, 90) = 8.64, p < .01,
= .32). The interaction between animacy and time window was significant in the by-participant analysis but not in the by-item analysis (F1 (5, 60) = 5.20, p < .05,
= .24; F2 (5, 90) = 1.55, p = .21,
= .08). The main effect of animacy (F1 (1, 12) = 1.89, p = .19,
= .14; F2 (1, 18) = 0.23, p = .64,
= .01), the interaction between word order and animacy (F1 (1, 12) = 1.42, p = .26,
= .11; F2 (1, 18) = 2.60, p = .12,
= .13), and the three-way interaction (F1 (5, 60) = 1.11, p = .36,
= .08; F2 (5, 90) = 1.83, p = .14,
= .09) were not found to be significant.
Since the interaction between word order and time window was significant, we conducted follow-up analyses to test the simple-main effects of word order for each time window. The analyses revealed that the simple-main effect of word order was significant for the 1000–2000 ms time window (F1 (1, 12) = 14.72, p < .01, = .55; F2 (1, 18) = 11.46, p < .01,
= .39). This indicated that, for this time window, the target advantage score was higher in the SVO word order condition than in the VOS condition. The simple-main effect was also significant for the 3000–4000 ms window (F1 (1, 12) = 16.33, p < .01,
= .58; F2 (1, 18) = 15.29, p < .01,
= .46), and approached significance for the 4000–5000 ms window (F1 (1, 12) = 4.49, p = .06,
= .27; F2 (1, 18) = 13.53, p < .01,
= .43). Contrary to the case of the 1000–2000 ms window, the target advantage scores were higher in the VOS condition than in the SVO condition for 3000–4000 ms and 4000–5000 ms windows. For the 0–1000 ms window (F1 (1, 12) = 0.58, p = .46,
= .05; F2 (1, 18) = 5.31, p < .05,
= .23), the simple-main effect was significant in the by-item analysis but not in the by-participant analysis. No significant simple-main effects were found for the 2000–3000 ms (F1 (1, 12) = 1.97, p = .19,
= .14; F2 (1, 18) = 0.97, p = .34,
= .05) and 5000–6000 ms (F1 (1, 12) = 2.68, p = .13,
= .18; F2 (1, 18) = 1.72, p = .21,
= .09) windows.
We also tested simple-main effects of time window for each word order condition. The analyses found a significant simple-main effect of time window in the SVO condition (F1 (5, 60) = 12.21, p < .01, = .50; F2 (5, 90) = 21.42, p < .01,
= .54): a multiple comparison (Holm’s method) revealed (i) that the target advantage score of the 0–1000 ms window was significantly higher than that of the 2000–3000 ms (p1 < .01, p2 < .01), 3000–4000 ms (p1 < .01, p2 < .01), and 4000–5000 ms (p1 < .05, p2 < .01) windows, and (ii) that the target advantage score of the 1000–2000ms window was significantly higher than that of the 2000–3000 ms (p1 < .01, p2 < .01), 3000–4000 ms (p1 < .01, p2 < .01), and 4000–5000 ms (p1 < .05, p2 < .01) windows. The differences between the 0–1000 ms and 5000–6000 ms windows, between the 1000–2000ms and 5000–6000 ms windows, between the 3000–4000 ms and 4000–5000 ms windows, and between the 3000–4000 ms and 5000–6000 ms windows were significant in the by-item analysis but not in the by-participant analysis (p1s > .05, p2s < .05). No other significant differences between time windows were found in both the by-participant and by-item analyses (p1s, p2s > .05), in the SVO condition. In the VOS condition, no significant simple-main effect of time window was found (F1 (5, 60) = 2.02, p = .09,
= .14; F2 (5, 90) = 2.39, p = .07,
= .12).
In addition to the ANOVAs, we performed one-sample t-tests, which compare the target advantage score with a zero value (i.e. examine whether the score is significantly positive or negative). Since the analyses were conducted separately for each time window, we will report the p-values adjusted by the Holm’s method. The analyses revealed that the target advantage scores were significantly positive in the 0–1000 ms (t1 (12) = 18.39, p < .01; t2 (19) = 5.17, p < .01), 1000–2000ms (t1 (12) = 7.59, p < .01; t2 (19) = 6.36, p < .01), 4000–5000 ms (t1 (12) = 4.38, p < .01; t2 (19) = 3.45, p < .01), and 5000–6000 ms (t1 (12) = 4.73, p < .01; t2 (19) = 4.48, p < .01) windows. The target advantage scores did not significantly differ from zero in the 2000–3000 ms (t1 (12) = 2.53, p < .05; t2 (19) = 1.95, p = .13) and 3000–4000 ms windows (t1 (12) = 2.85, p < .05; t2 (19) = 1.54, p = .14).
Furthermore, we conducted the same one-sample t-tests for each word order condition. The analyses revealed that in the SVO condition, target advantage scores were significantly positive in the 0–1000 ms (t1 (12) = 17.69, p < .01; t2 (19) = 5.75, p < .01), and 1000–2000ms (t1 (12) = 7.87, p < .01; t2 (19) = 8.09, p < .01) windows. The target advantage scores did not significantly differ from zero in the 2000–3000 ms (t1 (12) = 1.40, p = .37; t2 (19) = 1.39, p = .36), 3000–4000 ms (t1 (12) = −0.45, p = .66; t2 (19) = −0.08, p = .94), 4000–5000 ms (t1 (12) = 1.96, p = .22; t2 (19) = 2.26, p = .11), and 5000–6000 ms (t1 (12) = 2.75, p = .07; t2 (19) = 3.26, p < .05) windows. On the other hand, in the VOS condition, the target advantage score was significantly positive in all of the time windows (0–1000 ms: t1 (12) = 15.73, p < .01; t2 (19) = 4.47, p < .01; 1000–2000ms: t1 (12) = 6.21, p < .01; t2 (19) = 4.13, p < .01; 2000–3000 ms: t1 (12) = 3.65, p < .01; t2 (19) = 2.32, p < .05; 3000–4000 ms: t1 (12) = 4.37, p < .01; t2 (19) = 3.17, p < .05; 4000–5000 ms: t1 (12) = 7.49, p < .01; t2 (19) = 4.97, p < .01; 5000–6000 ms: t1 (12) = 6.20, p < .01; t2 (19) = 5.60, p < .01).
3.3 Brain activity
The averaged z-scores of the oxy-Hb values in the interested channels are shown in . A two-way ANOVA with word order (2) × patient animacy (2) revealed a significant main effect of word order only in ch-29 (F (1, 12) = 5.08, p < .05, = .30), indicating that the participants exhibited lower values in the VOS condition than the SVO condition. The main effect of word order was not significant in the other channels (ch-30: F (1, 12) = 0.71, p = .42,
= .06; ch-31: F (1, 12) = 0.02, p = .90,
= .02; ch-33: F (1, 12) = 0.22, p = .65,
= .02). Both the main effect of patient animacy (ch-29: F (1, 12) = 1.45, p = .25,
= .11; ch-30: F (1, 12) = 4.19, p = .06,
= .26; ch-31: F (1, 12) = 0.28, p = .61,
= .02; ch-33: F (1, 12) = 0.00, p = .97,
= .00) and the two-way interaction (ch-29: F (1, 12) = 0.15, p = .70,
= .01; ch-30: F (1, 12) = 0.27, p = .61,
= .02; ch-31: F (1, 12) = 0.43, p = .53,
= .03; ch-33: F (1, 12) = 4.72, p = .05,
= .28) were not significant in any of the four channels.
4. Discussion
We began this paper by stating the following four predictions (or expectations) drawn from the findings in previous studies. If subject-initial sentences are easier to initiate than verb-initial sentences because of the head-marking nature of Kaqchikel and the conceptual saliency of the agent among speakers of Kaqchikel, then (1) utterance latency should be shorter for SVO than VOS sentences. If the agent is conceptually more salient than the patient for speakers of Kaqchikel, (2) they should pay more attention to the agent than to the patient during sentence production. (3) Despite (1) and (2), the cognitive load during sentence production should be higher for SVO sentences than for the corresponding VOS sentences, because the former are syntactically more complex than the latter. Furthermore, if there occurs larger similarity-based competition between the subject and the object in VOS than in SVO, (4) the difference between SVO and VOS in cognitive load should be smaller in the inanimate patient condition than in the human patient condition. The results of the Kaqchikel experiment reported in the previous section confirmed all these predictions except the last. This constituted empirical support for the conclusion that Kaqchikel speakers preferentially employ the SVO word order at least partly because of the conceptual saliency of the agent. However, SVO incurs a greater cognitive load than the syntactically basic VOS word order primarily due to its syntactic complexity during both sentence production and sentence comprehension.
The utterance latencies were shorter for SOV than OSV in Japanese (Takeshima et al., Citation2014), and shorter for SVO than VOS in Kaqchikel. What is common between these results is that the subject-initial word orders had the shorter utterance latencies, meaning that utterance latency is not correlated with the syntactic complexity of the entire utterance, as SOV is syntactically simpler than OSV in Japanese and SVO is syntactically more complex than VOS in Kaqchikel. The results fall in line with the view that the conceptual saliency of the initial constituent has a relatively large effect on how early the utterance starts. Another possible factor in the longer latency of VOS utterances in Kaqchikel is, as mentioned in the Introduction, the conceptually and morphologically complex verb, with two agreement markers for the subject and object that are required to initiate such an utterance. This may also be a reason why the difference in utterance latency between the two word orders is larger in Kaqchikel (SVO vs. VOS) than in Japanese (SOV vs. OSV).
The conceptual saliency of the agent received further support from the eye tracking data. Both Japanese and Kaqchikel speakers initially fixated on the agent preferentially and continued to do so for most of the subsequent time periods. An initial fixation on the agent was observed even when OSV sentences were being produced in Japanese, which shows that speakers initially pay more attention to the agent (subject) than the patient (object) regardless of the word order of the utterance to be generated. While producing SVO sentences, Kaqchikel speakers looked quickly at the agent, continued fixating on this character until the onset of speech, and finally shifted their gaze to the patient (a). Kaqchikel VOS sentences exhibited a different fixation pattern than either SVO sentences in Kaqchikel or SOV and OSV sentences in Japanese. During the production of the latter three types, speakers initially fixated on the agent and then shifted their attention to the patient to some extent. In addition, the speakers in the Japanese experiment finally reverted to the agent in both the SOV and OSV conditions. During the production of VOS sentences in Kaqchikel, however, such a bi- or tri-phasic pattern was not observed. The relative ratios of agent and patient fixations were fairly uniform across the entire time span (b). This result is similar to Norcliffe et al.’s (Citation2015) observation that the patterns of eye fixation during the conceptual encoding and linguistic encoding periods differ between VOS and SVO sentence production in Tzeltal. This suggests that, while producing VOS sentences, Kaqchikel (and Tzeltal) speakers process agent, patient, and action, as well as their corresponding linguistic representations, more in parallel (or less linearly) than when producing SVO sentences. This may be because initiating an utterance with a verb in a head-marking language such as Kaqchikel requires both agent and patient, as well as action, to have been processed to a certain degree in order to determine the form of the verb before its onset.
Figure 9. The proportion of looks to the agent/patient AOI during the picture presentation for (a) SVO and (b) VOS utterances. The black vertical (dotted) lines represent speech onset (offset).
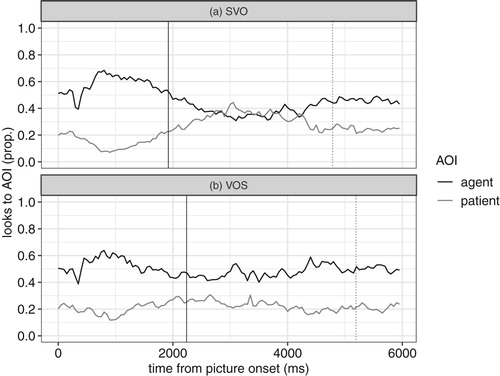
The brain activation data obtained using NIRS revealed that the production of OSV utterances yielded a higher left IFG activation than did the production of SOV utterances in Japanese, and the production of SVO utterances did the same with respect to VOS utterances in Kaqchikel.Footnote4 This clearly shows that sentences with a syntactically derived word order require more cognitive resources to represent than comparable sentences with the syntactically basic word order in a given language not only in sentence comprehension but also in sentence production both in SO and OS languages.
Finally, we could not find evidence for larger similarity-based competition effects in VOS sentences than SVO sentences. The NIRS data show that the differences in the cognitive loads between SVO and VOS sentences are comparable in the human patient condition and the inanimate patient condition. This is in line with the results of Kiyama et al.’s (Citation2013) Kaqchikel sentence comprehension experiment that VOS was processed faster than SVO regardless of the animacy of the object, and that the effect of the object animacy and the interaction between the word order and the object animacy were not significant. This indicates that the similarity-based competition, if present, may not be the primary factor for the higher production ratio of SVO in the reversible event condition than in the non-reversible event condition. Although it is beyond the scope of this paper to elucidate the reasons for this effect of event reversibility on word order preference, communicative approaches such as the noisy channel hypothesis by Gibson et al. (Citation2013) and the role conflict hypothesis by Hall, Mayberry, and Ferreira (Citation2013) seem to be a promising avenue to pursue.
5. Conclusion
The results of the eye-tracked picture description experiment reported in this paper support the conclusion that although Kaqchikel speakers preferentially use the SVO word order partly because of the saliency of the agent and the complexity of the verbal predicate, SVO sentences require more processing resources than do VOS sentences both in comprehension and production.
Acknowledgment
Earlier versions of this paper were presented at the 68th Meeting of the Tohoku Psychological Association (Akita University, Japan) and the 53rd Meeting of the Korean Society for Cognitive and Biological Psychology (Jeju Island, Republic of Korea) as well as MIT and Harvard University. We are grateful to the participants and audience for their feedback and to two anonymous reviewers for their insightful and instructive comments on an earlier draft. We also thank Juan Esteban Ajsivinac Sian, Filiberto Patal Majzul, Lolmay Pedro Oscar García Matzar, and Yoshiho Yasugi for their invaluable support of the current study throughout its various stages. This work was supported in part by JSPS KAKENHI Grant Numbers 15H02603, 19H05589.
Disclosure statement
No potential conflict of interest was reported by the authors.
ORCID
Masatoshi Koizumi http://orcid.org/0000-0003-0719-7128
Additional information
Funding
Notes
1 The syntactically basic word order of a language is defined here as the word order associated with the simplest syntactic structures among grammatically possible transitive sentences with a nominal subject and a nominal object in that language. In Japanese, for instance, the syntactically basic word order is SOV, and OSV is derived from it by scrambling the object across the subject. OSV sentences are, therefore, syntactically more complex than are comparable SOV sentences, as schematically shown in (i). In (ib) the fronted object and the gap in the object position forms a filler-gap dependency, which is lacking in (ia).
[SOV]
[Oi [S gapi V]]
2 The first two factors mentioned below are in line with the general idea of availability-based models of sentence production, according to which speakers favour sentence structures in which more readily available words are placed earlier. In contrast, efficiency-based theories focus more on ease of comprehension. See Ros, Santesteban, Fukumura, and Laka (Citation2015) for a review. See also Koizumi et al. (Citation2014) for the related notions of the universal cognitive view vs. the individual grammar view. Koizumi et al. (Citation2014) argue that the cognitive load during sentence comprehension is primarily determined by grammatical processes operating on linguistic representations (in congruent with the individual grammar view), whereas word order selection in sentence production more faithfully reflects event apprehension and preverbal message construction at the stage of conceptual processing (consistent with the universal cognition view).
3 The categories of utterances excluded from analysis and their ratios in each of the SVO and VOS conditions are as follows: transitive sentences in a word order other than the specified one (SVO = 2.69 ± 3.30%, VOS = 8.08 ± 13.77%, p = .21), intransitive sentences (SVO = 6.15 ± 4.16%, VOS = 6.54 ± 6.25%, p = .75), adjectival sentences (SVO = 11.92 ± 12.00%, VOS = 9.23 ± 7.87%, p = .25), passive sentences (SVO = 0.38 ± 1.39%, VOS = 2.69 ± 8.32%, p = .35), antipassive sentences (SVO = 1.92 ± 3.84%, VOS = 4.23 ± 7.32%, p = .19), and others (SVO = 2.31 ± 3.88%, VOS = 1.92 ± 3.25%, p = .67). The removed responses were equally distributed between the two word order conditions, and there was no significant difference in each category as shown above nor in total (SVO = 25.38 ± 12.33%, VOS = 32.69 ± 16.28%, p = .19).
4 The activated channel (channel 29) in this study and the one (channel 32) reported in Takeshima et al. (Citation2014) are adjacent to each other. Although they are not the same, given the relatively low spatial resolution and rough spatial correspondence of the NIRS system, compared to the magnetic resonance imaging system (Strangman, Culver, Thompson, & Boas, Citation2002), it is reasonable to conclude that similar brain regions (i.e., regions in the left IFG) activated in our current and previous studies. Note that some previous studies have analyzed NIRS signals by combining multi-channels responses together and have interpreted global brain activities (e.g., Minagawa-Kawai, Mori, Furuya, Hayashi, & Sato, Citation2002; Minagawa-Kawai, Mori, Sato, & Koizumi, Citation2004). It is beyond the scope of the present study to identify the precise locus of activation.
References
- Aissen, J. L. (1992). Topic and focus in Mayan. Language, 68, 43–80. doi: 10.1353/lan.1992.0017
- Ajsivinac Sian, J. E., García Matzar, L. P. O., Cutzal, M. C., & Alonzo Guaján, I. E. (2004). Gramática descriptiva del idioma maya Kaqchikel: Rutzijoxik rucholik ri Kaqchikel ch’ab’äl. Chimaltenango: Comunidad Lingüística Kaqchikel de la Academia de las Lenguas Mayas de Guatemala.
- Bader, M., & Meng, M. (1999). Subject-object ambiguities in German embedded clauses: An across-the-board comparison. Journal of Psycholinguistic Research, 28, 121–143. doi: 10.1023/A:1023206208142
- Bates, E. (1976). Language and context: The acquisition of pragmatics. Cambridge: Academic Press.
- Bock, J. K., & Warren, R. K. (1985). Conceptual accessibility and syntactic structure in sentence formulation. Cognition, 21, 47–67. doi: 10.1016/0010-0277(85)90023-X
- Brody, J. (1984). Some problems with the concept of basic word order. Linguistics, 22, 711–736. doi: 10.1515/ling.1984.22.5.711
- Chujo, K. (1983). The interrelationships among strategies for sentence comprehension. The Japanese Journal of Psychology, 54, 250–256. (published in Japanese). doi: 10.4992/jjpsy.54.250
- De Smedt, K. (1990). IPF: An incremental parallel formulator. In R. Dale, C. Mellish, & M. Zock (Eds.), Current research in natural language generation (pp. 167–192). London: Academic Press.
- England, N. C. (1991). Changes in basic word order in Mayan languages. International Journal of American Linguistics, 57, 446–486. doi: 10.1086/ijal.57.4.3519735
- Erdocia, K., Laka, I., Mestres-Missé, A., & Rodriguez-Fornells, A. (2009). Syntactic complexity and ambiguity resolution in a free word order language: Behavioral and electrophysiological evidences from Basque. Brain and Language, 109, 1–17. doi: 10.1016/j.bandl.2008.12.003
- García Matzar, P. O., & Rodríguez Guaján, J. O. (1997). Rukemik ri Kaqchikel chi’: Gramática kaqchikel. Ciudad de Guatemala: Editorial Cholsamaj.
- Gennari, S. P., Mirković, J., & MacDonald, M. C. (2012). Animacy and competition in relative clause production: A cross-linguistic investigation. Cognitive Psychology, 65, 141–176. doi: 10.1016/j.cogpsych.2012.03.002
- Gibson, E. (2000). Dependency locality theory: A distance-based theory of linguistic complexity. In A. Marantz, Y. Miyashita, & W. O’Neil (Eds.), Image, language, brain: Papers from the first Mind Articulation Project Symposium (pp. 95–126). Cambridge: MIT Press.
- Gibson, E., Piantadosi, S. T., Brink, K., Bergen, L., Lim, E., & Saxe, R. (2013). A noisy-channel account of crosslinguistic word-order variation. Psychological Science, 24, 1079–1088. doi: 10.1177/0956797612463705
- Gleitman, L. R., January, D., Nappa, R., & Trueswell, J. C. (2007). On the give and take between event apprehension and utterance formulation. Journal of Memory and Language, 57, 544–569. doi: 10.1016/j.jml.2007.01.007
- Grewe, T., Bornkessel-Schlesewsky, I., Zysset, S., Wiese, R., von Cramon, D. Y., & Schlesewsky, M. (2007). The role of the posterior superior temporal sulcus in the processing of unmarked transitivity. NeuroImage, 35, 343–352. doi: 10.1016/j.neuroimage.2006.11.045
- Griffin, Z. M., & Bock, K. (2000). What the eyes say about speaking. Psychological Science, 11, 274–279. doi: 10.1111/1467-9280.00255
- Hagiwara, H., Soshi, T., Ishihara, M., & Imanaka, K. (2007). A topographical study on the event-related potential correlates of scrambled word order in Japanese complex sentences. Journal of Cognitive Neuroscience, 19, 175–193. doi: 10.1162/jocn.2007.19.2.175
- Hakulinen, A., & Karlsson, F. (1980). Finnish syntax in text: Methodology and some results of a quantitative study. Nordic Journal of Linguistics, 3, 93–129. doi: 10.1017/S0332586500000536
- Hall, M. L., Mayberry, R. I., & Ferreira, V. S. (2013). Cognitive constraints on constituent order: Evidence from elicited pantomime. Cognition, 129, 1–17. doi: 10.1016/j.cognition.2013.05.004
- Hawkins, J. A. (2004). Efficiency and complexity in grammars. Oxford: Oxford University Press.
- Homae, F., Watanabe, H., Nakano, T., & Taga, G. (2007). Prosodic processing in the developing brain. Neuroscience Research, 59, 29–39. doi: 10.1016/j.neures.2007.05.005
- Hoshi, Y. (2003). Functional near-infrared optical imaging: Utility and limitations in human brain mapping. Psychophysiology, 40, 511–520. doi: 10.1111/1469-8986.00053
- Imamura, S., & Koizumi, M. (2011). A centering analysis of word order in Japanese. Tohoku Studies in Linguistics, 20, 59–74.
- Kaiser, E., & Trueswell, J. C. (2004). The role of discourse context in the processing of a flexible word-order language. Cognition, 94, 113–147. doi: 10.1016/j.cognition.2004.01.002
- Kempen, G., & Hoenkamp, E. (1987). An incremental procedural grammar for sentence formulation. Cognitive Science, 11, 201–258. doi: 10.1207/s15516709cog1102_5
- Kim, J. (2012). Priming effects in scrambled sentences in Korean. Culture, 75, 228–213. (published in Japanese).
- Kim, J., Koizumi, M., Ikuta, N., Fukumitsu, Y., Kimura, N., Iwata, K., … Kawashima, R. (2009). Scrambling effects on the processing of Japanese sentences: An fMRI study. Journal of Neurolinguistics, 22, 151–166. doi: 10.1016/j.jneuroling.2008.07.005
- Kinno, R., Kawamura, M., Shioda, S., & Sakai, K. L. (2008). Neural correlates of non-canonical syntactic processing revealed by a picture-sentence matching task. Human Brain Mapping, 29, 1015–1027. doi: 10.1002/hbm.20441
- Kiyama, S., Tamaoka, K., Kim, J., & Koizumi, M. (2013). Effect of animacy on word order processing in Kaqchikel Maya. Open Journal of Modern Linguistics, 3, 203–207. doi: 10.4236/ojml.2013.33027
- Koizumi, M., & Imamura, S. (2017). Interaction between syntactic structure and information structure in the processing of a head-final language. Journal of Psycholinguistic Research, 46, 247–260. doi: 10.1007/s10936-016-9433-3
- Koizumi, M., & Kim, J. (2016). Greater left inferior frontal activation for SVO than VOS during sentence comprehension in Kaqchikel. Frontiers in Psychology, 7, 1541–1549. doi: 10.3389/fpsyg.2016.01541
- Koizumi, M., Yasugi, Y., Tamaoka, K., Kiyama, S., Kim, J., Ajsivinac Sian, J. E., & García Matzar, P. O. (2014). On the (non) universality of the preference for subject-object word order in sentence comprehension: A sentence-processing study in Kaqchikel Maya. Language, 90, 722–736. doi: 10.1353/lan.2014.0068
- Kovelman, I., Shalinsky, M. K., White, K., Schmitt, S. N., Berens, M. S., Paymer, N., & Petitto, L.-A. (2009). Dual language use in sign-speech bimodal bilinguals: FNIRS brain-imaging evidence. Brain and Language, 109, 112–123. doi: 10.1016/j.bandl.2008.09.008
- Kronmüller, E., & Barr, D. J. (2015). Referential precedents in spoken language comprehension: A review and meta-analysis. Journal of Memory and Language, 83, 1–19. doi: 10.1016/j.jml.2015.03.008
- Kubo, T., Ono, H., Tanaka, M., Koizumi, M., & Sakai, H. (2015). Mechanisms for VOS sentence production in Kaqchikel: Evidence from animacy effects on choice of word order. Cognitive Studies, 22, 591–603. (published in Japanese).
- Lee, E. K., Brown-Schmidt, S., & Watson, D. W. (2013). Ways of looking ahead: Incrementality in language production. Cognition, 129, 544–562. doi: 10.1016/j.cognition.2013.08.007
- Marantz, A. (2005). Generative linguistics within the cognitive neuroscience of language. The Linguistic Review, 22, 429–445. doi: 10.1515/tlir.2005.22.2-4.429
- Maxwell, J. M., & Little, W. E. (2006). Tijonïk Kaqchikel oxlajuj aj -Curso de idioma y cultura maya Kaqchikel. Guatemala: Editorial Junajpu.
- Mazuka, R., Itoh, K., & Kondo, T. (2002). Costs of scrambling in Japanese sentence processing. In M. Nakayama (Ed.), Sentence processing in East Asian languages (pp. 131–166). Stanford: CSLI Publications.
- McDonald, J. L., Bock, K., & Kelly, M. H. (1993). Word and world order: Semantic, phonological, and metrical determinants of serial position. Cognitive Psychology, 25, 188–230. doi: 10.1006/cogp.1993.1005
- Minagawa-Kawai, Y., Mori, K., Furuya, I., Hayashi, R., & Sato, Y. (2002). Assessing cerebral representations of short and long vowel categories by NIRS. Neuroreport, 13, 581–584. doi: 10.1097/00001756-200204160-00009
- Minagawa-Kawai, Y., Mori, K., Sato, Y., & Koizumi, T. (2004). Differential cortical responses in second language learners to different vowel contrasts. Neuroreport, 15, 899–903. doi: 10.1097/00001756-200404090-00033
- Momma, S., & Phillips, C. (2018). The relationship between parsing and generation. Annual Review of Linguistics, 4, 233–254. doi: 10.1146/annurev-linguistics-011817-045719
- Myachykov, A., & Tomlin, R. S. (2008). Perceptual priming and structural choice in Russian sentence production. Journal of Cognitive Science, 9, 31–48. doi: 10.17791/jcs.2008.9.1.31
- Noguchi, Y., Takeuchi, T., & Sakai, K. L. (2002). Lateralized activation in the inferior frontal cortex during syntactic processing: Event-related optical topography study. Human Brain Mapping, 17, 89–99. doi: 10.1002/hbm.10050
- Norcliffe, E., Konopka, A. E., Brown, P., & Levinson, S. C. (2015). Word order affects the time course of sentence formulation in Tzeltal. Language, Cognition and Neuroscience, 30, 1187–1208. doi: 10.1080/23273798.2015.1006238
- O’Grady, W. (1997). Syntactic development. Chicago: University of Chicago Press.
- Oldfield, R. C. (1971). The assessment and analysis of handedness: The Edinburgh inventory. Neuropsychologia, 9, 97–113. doi: 10.1016/0028-3932(71)90067-4
- Otsuka, Y., Nakato, E., Kanazawa, S., Yamaguchi, M. K., Watanabe, S., & Kakigi, R. (2007). Neural activation to upright and inverted faces in infants measured by near infrared spectroscopy. NeuroImage, 34, 399–406. doi: 10.1016/j.neuroimage.2006.08.013
- Pritchett, B., & Whitman, J. (1995). Syntactic representation and interpretive preference. In R. Mazuka & N. Nagai (Eds.), Japanese sentence processing. Lawrence Erlbaum and associates (pp. 65–76). New York: Psychology Press.
- Rodríguez Guaján, J. O. (1994). Rutz’ib’axik ri Kaqchikel: Manual de redacción Kaqchikel. Ciudad de Guatemala: Editorial Cholsamaj.
- Ros, I., Santesteban, M., Fukumura, K., & Laka, I. (2015). Aiming at shorter dependencies: The role of agreement morphology. Language, Cognition and Neuroscience, 30, 1156–1174. doi: 10.1080/23273798.2014.994009
- Rösler, F., Pechmann, T., Streb, J., Roeder, B., & Hennighausen, E. (1998). Parsing of sentences in a language with varying word order: Word-by-word variations of processing demands are revealed by event-related brain potentials. Journal of Memory and Language, 38, 150–176. doi: 10.1006/jmla.1997.2551
- Schroeter, M., Zysset, S., Kruggel, F., & von Cramon, D. (2003). Age dependency of the hemodynamic response as measured by functional near-infrared spectroscopy. NeuroImage, 19, 555–564. doi: 10.1016/S1053-8119(03)00155-1
- Sekerina, I. A. (1997). The syntax and processing of Russian scrambled constructions in Russian (Doctoral Dissertation). City University of New York.
- Shibata, H., Sugiyama, T., Suzuki, M., Kim, J., Gyoba, J., & Koizumi, M. (2006). Nihongo-setsunai-kakimazebun-no konsekiitishuuhen-ni okeru shorikatei-no kentou. Cognitive Studies, 13, 301–315. (published in Japanese).
- Slobin, D. I., & Bever, T. G. (1982). Children use canonical sentence schemas: A crosslinguistic study of word order and inflections. Cognition, 12, 229–265. doi: 10.1016/0010-0277(82)90033-6
- Strangman, G., Culver, J. P., Thompson, J. H., & Boas, D. A. (2002). A quantitative comparison of simultaneous BOLD fMRI and NIRS recordings during functional brain activation. NeuroImage, 17, 719–731. doi: 10.1006/nimg.2002.1227
- Symeonidou, I., Dumontheil, I., Chow, W. Y., & Breheny, R. (2016). Development of online use of theory of mind during adolescence: An eye-tracking study. Journal of Experimental Child Psychology, 149, 81–97. doi: 10.1016/j.jecp.2015.11.007
- Taga, G., Asakawa, K., Maki, A., Konishi, Y., & Koizumi, H. (2003). Brain imaging in awake infants by near-infrared optical topography. Proceedings of the National Academy of Sciences, 100, 10722–10727. doi: 10.1073/pnas.1932552100
- Takeshima, Y., Saito, G., Tachibana, R., Asaoka, R., Gyoba, J., & Koizumi, M. (2014). Processing loads related to word order preference during sentence production in Japanese: An NIRS and eye tracking study. Tohoku Psycholog. Folia, 73, 36–45.
- Tamaoka, K., Kanduboda, P. B. A., & Sakai, H. (2011). Effects of word order alternation on the sentence processing of Sinhalese written and spoken forms. Open Journal of Modern Linguistics, 1, 24–32. doi: 10.4236/ojml.2011.12004
- Tamaoka, K., & Koizumi, M. (2006). Issues on the scrambling effects in the processing of Japanese sentences: Reply to Miyamoto and Nakamura (2005) regarding the experimental study by Koizumi and Tamaoka (2004). Gengo Kenkyu, 129, 181–226.
- Tamaoka, K., Sakai, H., Kawahara, J., Miyaoka, Y., Lim, H., & Koizumi, M. (2005). Priority information used for the processing of Japanese sentences: Thematic roles, case particles or grammatical functions? Journal of Psycholinguistic Research, 34, 281–332. doi: 10.1007/s10936-005-3641-6
- Tanaka, M. N., Branigan, H. P., McLean, J. F., & Pickering, M. J. (2011). Conceptual influences on word order and voice in sentence production: Evidence from Japanese. Journal of Memory and Language, 65, 318–330. doi: 10.1016/j.jml.2011.04.009
- Tichoc, C. R., Sian, J. E. A., García Matzar, L. P. O., Espantzay, I. C., Cutzal, M. C., & Guajan, E. A. (2000). Runuk’ul pa rub’eyal rutz’ib’axik ri Kaqchikel ch’ab’ äl: Gramática normativa del idioma Maya Kaqchikel. Chimaltenango: Comunidad Lingüística Kaqchikel de la Academia de las Lenguas Mayas de Guatemala.
- Ueno, M., & Kluender, R. (2003). Event-related brain indices of Japanese scrambling. Brain and Language, 86, 243–271. doi: 10.1016/S0093-934X(02)00543-6
- Watanabe, E., Maki, A., Kawaguchi, F., Takashiro, K., Yamashita, Y., Koizumi, H., & Mayanagi, Y. (1998). Non-invasive assessment of language dominance with near-infrared spectroscopic mapping. Neuroscience Letters, 256, 49–52. doi: 10.1016/S0304-3940(98)00754-X
- Yano, M., Yasunaga, D., & Koizumi, M. (2017). Event-related brain indices of gap-filling processing in Kaqchikel. In S. R. Harris (Ed.), Event-Related Potential (ERP): Methods, outcomes, research insights (pp. 89–122). Hauppauge: NOVA Science Publishers.
- Yasunaga, D., Yano, M., Yasugi, Y., & Koizumi, M. (2015). Is the subject-before-object preference universal? An event-related potential study in the Kaqchikel Mayan language. Language, Cognition and Neuroscience, 30, 1209–1229. doi: 10.1080/23273798.2015.1080372