ABSTRACT
Converging evidence points towards a link between musical rhythm and linguistic syntax processing. Several potentially shared cognitive mechanisms and overlapping brain regions have been proposed to account for these findings. The present study explores the hypothesis that a domain-general cognitive system responsible for hierarchical structure building constitutes one such component. In two experiments, French-speaking adults listened to rhythmically regular, irregular or silent primes before completing a grammaticality judgement task on Jabberwocky sentences. Both experiments showed a priming effect only in the first three sentences after priming. Experiment 1 (block design) showed a disadvantage of the irregular condition compared to both other conditions. Experiment 2 (mixed design) showed an advantage in the regular condition compared to the irregular condition. Across the two experiments, grammaticality judgement performance correlated with rhythm discrimination. These findings provide evidence for a domain-general cognitive network responsible for hierarchical structure building in rhythm and language processing.
Introduction
It has recently been suggested that there are several brain networks and neural mechanisms common to musical rhythm and speech and language processing. Some of these overlapping brain regions include networks of cortical regions such as the left inferior frontal gyrus, the left supplementary motor area, the bilateral insula and subcortical brain regions such as the basal ganglia and the cerebellum (Fujii & Wan, Citation2014; Heard & Lee, Citation2019; Kotz et al., Citation2014; Kotz & Schwartze, Citation2010; Patel & Iversen, Citation2014; Schwartze & Kotz, Citation2016; Tierney & Kraus, Citation2014). Shared mechanisms include the precise encoding of low-level information in the acoustic signal, neural oscillations that are able to entrain (become synchronised in frequency, phase, or both) to external and other internal oscillations, and hierarchical cognitive control (Asano et al., Citation2021; Fiveash et al., Citation2021.; Ladányi et al., Citation2020).
One important line of research has proposed that musical rhythm and language processing both involve the internal construction of hierarchical sequences. They can be considered sequences as they are both ordered collections of elements that unfold over time. They can be considered hierarchical as both can be represented in a tree-like structure in which lower-level units are combined into higher-level constituents (Fitch & Martins, Citation2014). One clear illustration of the spontaneous tendency to find hierarchical structure in sequential events is the report that, when hearing a metronome of completely identical tones that contain no acoustic markings of groups of constituents, neurotypical adults without hearing, language, or music impairments (henceforth referred to as typical adults in the present paper) tend to perceive groups of 2–8 beats (Poudrier, Citation2020). Further evidence supporting this proposal comes from several neuroimaging studies showing neural tracking of acoustically absent structural (syntactic or prosodic) constituents in language, and similar entrainment mechanisms to an acoustically missing beat in music (Ding et al., Citation2016; Glushko et al., Citation2020; Kaufeld et al., Citation2020; Large et al., Citation2015; Tal et al., Citation2017). Indeed, converging evidence points to the existence of a domain-general cognitive system responsible for coding hierarchical structure (Martins et al., Citation2017, Citation2020). The capacity to create hierarchical representations has been proposed to be especially developed in the human species. Humans are able to generate hierarchies in a wide range of domains including metrical (as music and speech both have a metrical structure, we will use the term musical rhythmic structure to refer to metrical structure specifically in music), linguistic, visual, action, and social hierarchies (Altmann et al., Citation2003; Fitch & Friederici, Citation2012; Fitch & Martins, Citation2014; Hauser et al., Citation2010; Jackendoff, Citation2009; Martins et al., Citation2017; Zink et al., Citation2008).
Recent evidence confirms numerous interactions between language and musical rhythm processing in typical and atypical populations (see Fiveash et al., Citation2021; Ladányi et al., Citation2020 for recent reviews). Typically-developing (TD) preschool children’s ability to synchronise motor production to an external rhythm seems to be linked to precise neural encoding of the speech syllable envelope and to predict reading readiness measured by phonological awareness, auditory short-term memory, as well as rapid object and colour naming (Woodruff Carr et al., Citation2014). Rhythm discrimination performance reliably explains morphosyntactic production in 6-year-old children (Gordon et al., Citation2015), and correlates with syntactic comprehension in children between the ages of 7 and 17 (Lee et al., Citation2020). Children with developmental language disorders often underperform in rhythm discrimination, rhythm reproduction, tapping to a metronome or extracting and anticipating the beat of a metronome (Corriveau & Goswami, Citation2009; Flaugnacco et al., Citation2014).
In typical adults, rhythmic proficiency has also been shown to have an effect on speech and language processing: percussionists, who excel at rhythmic skills even compared to vocalists, outperform both vocalists and non-musicians in sentence-in-noise perception, but not in words-in-noise perception. The authors proposed that rhythmic patterns may not only help identify word boundaries, but also provide cues implying certain grammatical structures (Slater & Kraus, Citation2016; Yates et al., Citation2019). At the brain level, a recent activation likelihood estimate (ALE) meta-analysis has reported several overlapping brain regions involved in rhythm and syntax processing, including the left inferior frontal gyrus (pars opercularis), the left supplementary motor area, as well as the bilateral insula (Heard & Lee, Citation2019). Furthermore, patients with lesions or neurodegenerative diseases to the basal ganglia show impaired speech rhythm production, musical rhythm discrimination and a missing P600, an ERP-response traditionally associated with syntactic integration (Grahn & Brett, Citation2009; Osterhout & Holcomb, Citation1992).
Different types of rhythmic stimulation have been shown to influence language processing in children with developmental language disorder (DLD) and developmental dyslexia (DD), typically developing children, typical adults and adults with acquired neurodegenerative disorders or lesions to the basal ganglia. First, superimposing a highly regular (trochaic) speech rhythm on natural German sentences can facilitate semantic and syntactic integration in typical adults (Roncaglia-Denissen et al., Citation2013, Citation2015; Rothermich et al., Citation2012; Rothermich & Kotz, Citation2013), as well as restoring the missing P600 in adults with lesions to the basal ganglia (Kotz & Schmidt-Kassow, Citation2015). Similarly, priming German-speaking Parkinson’s patients with a march (but not a waltz) rhythm has also restored a missing P600 in syntactic integration (Kotz & Gunter, Citation2015). Second, cueing phrases or sentences with a rhythmic pattern whose structure matches the stress pattern of the language stimulus has been shown to lead to enhanced phonological processing in typical adults (Cason et al., Citation2015; Cason & Schön, Citation2012) and more accurate reproduction of the target sentence in prelingually deaf children with hearing aids compared to a mismatching cue (Cason et al., Citation2015). Lastly, exposure to a prime whose underlying musical rhythmic structure is easy to extract improves syntactic processing compared to an irregular musical prime, environmental noise, or silence in children with developmental language disorder, developmental dyslexia, as well as typically developing children (Bedoin et al., Citation2016; Canette, Bedoin, et al., Citation2020; Canette, Fiveash, et al., Citation2020; Przybylski et al., Citation2013) and typical adults (Canette, Lalitte, et al., Citation2020) in French, English (Chern et al., Citation2018), and Hungarian (Ladányi et al., Citation2021). In their original research with children with DLD, DD and typical development, Przybylski et al. (Citation2013) found that all groups performed better in an auditory grammaticality judgement task in French when presented with rhythmically regular than irregular musical primes. These results were replicated, albeit with a smaller effect size, when regular primes were contrasted to rhythmically neutral environmental sound scenes in French children with DLD and typically-developing controls, suggesting a beneficial effect of regular rhythms (Bedoin et al., Citation2016). These findings were later refined in Hungarian-speaking children with DLD and TD controls, who showed better grammatical judgement performance for rhythmically regular than irregular primes or a silent baseline (with no difference between the latter conditions, Ladányi et al., Citation2021). Interestingly, the rhythmic priming effect (RPE) seems to be specific to syntactic (or at least language) processing. No rhythmic priming effect has been found in Math and visuo-spatial control tasks in TD English children (Chern et al., Citation2018), semantic fluency in TD French children (Canette, Lalitte, et al., Citation2020), and a picture naming and a Stroop task in TD Hungarian children (Ladányi et al., Citation2021). The rhythmic priming effect on typical children also seems to depend on the duration of the primes used: 32-second primes were reported to lead to a clear effect, while 8s and 16s primes did not (Fiveash et al., Citation2021). In typical adults, rhythmic priming led to an enhanced P600 in response to gender, number and person agreement violations after a rhythmically regular prime compared to an irregular prime, and higher grammaticality judgement accuracy (specifically in the second half of the experiment) in materials containing more subtle morphosyntactic errors such as tense, mode or preposition errors (Canette, Bedoin, et al., Citation2020; Canette, Fiveash, et al., Citation2020). To our knowledge, no experiments on typical adults have included a regular prime, an irregular prime as well as a baseline condition, the inclusion of which is crucial in determining whether the rhythmic priming effect consists primarily in a beneficial effect of a regular rhythm, or a penalising effect of an irregular rhythm.
The present study
The present study was motivated by the hypothesis that a domain-general cognitive system responsible for internal abstract hierarchical structure building is recruited by both linguistic and non-linguistic (musical rhythmic) hierarchical structure processing. We studied two types of effects in typical adult participants: a short-term rhythmic stimulation effect and longer-term correlations between rhythm and language processing. Short-term effects of rhythmic stimulation on syntactic processing were tested using a rhythmic priming paradigm. Under the hypothesis that a shared cognitive system underlies hierarchical structure building in both the linguistic and non-linguistic domains, and that this system is activated by the overly explicit musical rhythmic structure of the regular prime, we expected a regular rhythmic prime with an easy-to-extract underlying structure to facilitate grammaticality judgements in the subsequent syntactic task compared to an irregular or a control condition (Prediction 1). Alternatively, it is also possible that exposure to a rhythmically irregular prime would penalise this system, by providing inconsistent cues to an underlying musical rhythmic structure or by using up more shared resources in an attempt to build the musical rhythmic structure of the prime, thus resulting in lower syntactic performance. Unlike typically developing or atypical populations that are affected mainly by the beneficial effect of a regular prime, a fully developed system might show a smaller degree of improvement from regular primes, and an otherwise optimally working language processing system may even be disturbed by the presence of an irregular prime. To evaluate the direction of the RPE, a silent baseline condition was also used besides rhythmically regular and irregular musical primes.
To focus on the structural (syntactic) processing of language and reduce lexico-semantic effects as much as possible, semi-artificial Jabberwocky stimuli were used in which nouns were replaced by pseudowords, but verbs and grammatical morphemes of natural French were preserved (Franck & Wagers, Citation2020). To our knowledge, the present study is the first to test the effects of rhythmic priming on Jabberwocky stimuli. Ungrammatical sentences involved agreement errors, since the presence of an attractor noun phrase was found to trigger a substantial number of errors in sentence production, as in “The label on the bottles are rusty” (Bock & Miller, Citation1991 and much following work). Sensitivity to attraction was also found in sentence comprehension, where it manifests in terms of a “grammatical illusion”, i.e. the illusion that a sentence with an agreement violation is actually correct in the presence of an attractor matching the verb in terms of its agreement features (Villata et al., Citation2018, Citation2020; Wagers et al., Citation2009). Importantly, sensitivity to attraction in agreement processing was found to be modulated by a number of fine syntactic constraints such as level of embeddedness (Franck et al., Citation2006, Citation2010), including in sentence comprehension (Franck et al., Citation2015). Moreover, similar factors were found to affect agreement processing in the comprehension of natural and jabberwocky sentences (Franck & Wagers, Citation2020). These findings make attraction in jabberwocky an ideal test case to tap into syntactic processing, while maximally reducing lexico-semantic effects.
Long-term relationships between rhythm and language were explored through correlations between grammaticality judgement accuracy and behavioural tests of participants’ rhythmic abilities. Specifically, we measured rhythm and beat discrimination using the rhythm and accent subtests of the short version of the standardized Profile of Music Perception Skills (PROMS, Law & Zentner, Citation2012; Zentner & Strauss, Citation2017). If a shared cognitive system underlies hierarchical structure building in both the linguistic and non-linguistic domains, we expect to find correlations between grammaticality judgements and rhythm and beat discrimination skills. Moreover, we expect a correlation between participants’ sensitivity to the regularity of the rhythmic prime in the grammaticality judgement task (defined as the difference between grammaticality judgement performance after a regular and an irregular prime, i.e. the rhythmic priming effect) and their ability to process rhythmic structures (Prediction 2; Gordon et al., Citation2015; Heard & Lee, Citation2019; Lee et al., Citation2020). Furthermore, selective auditory attention was measured using an auditory oddball paradigm (Schwartze et al., Citation2013) in order to evaluate to what extent auditory attention is involved in the rhythmic priming effect. If rhythmic priming is linked to attention, we expect to observe a correlation between sensitivity to the regularity of the prime and oddball performance (Prediction 3). Additionally, the oddball task also allowed for controlling auditory attention while testing Prediction 2 by investigating links between auditory and attention-dependent rhythm and syntax tasks.
To reiterate, the present study sought to test three predictions. First, we expect rhythmic priming to influence grammaticality judgement performance by activating a shared network responsible for hierarchical structure building in rhythm and syntax (Prediction 1). Second, we expect to observe correlations between participants’ rhythm discrimination skills and grammaticality judgement performance, as well as between their rhythm discrimination skills and their sensitivity to the RPE (Prediction 2). Finally, we expect to see a relationship between participants’ auditory selective attention and their sensitivity to the RPE (Prediction 3).
Prediction 1 was tested in two experiments. In Experiment 1, a block design was used in the rhythmic priming procedure such that participants would be exposed to one of the prime rhythms for eight experimental blocks, then to silence, and finally to the other prime rhythm. This design was chosen under the prediction that prolonged exposure to the same rhythmic prime would maximise the differences between the prime conditions. Experiment 2 adopted a mixed design as classically used in the rhythmic priming literature, in which the three prime conditions continuously alternated (Bedoin et al., Citation2016; Canette, Bedoin, et al., Citation2020; Canette, Fiveash, et al., Citation2020; Canette, Lalitte, et al., Citation2020; Chern et al., Citation2018; Ladányi et al., Citation2021; Przybylski et al., Citation2013). As the two experiments are identical apart from this design choice, Predictions 2 and 3 will be reported using the combined datasets of Experiments 1 and 2.
Experiment 1: block design
Participants
41 native French-speaking neurotypical adults participated in the experiment. Participants were between 18 and 33 years old (M = 21.923, SD = 2.938) and reported no history of psychiatric issues, neurological disorders, specific language impairment, hearing impairments, or amusia.
Participants gave informed consent prior to the start of the experiment, were not made aware of the purpose of the study, and were compensated with either course credits or 20 CHF for their time. The experiment was approved by the University of Geneva Research Ethics Committee (PSE.20191004.04).
Materials
Grammaticality judgement (GJ) task
Musical Stimuli: Two 32-second rhythmic primes (1 regular and 1 irregular) from Przybylski et al. (Citation2013) study were used. The primes contained the same number of tones and featured the same percussion instruments (a tam-tam at 175 Hz and a maracas at 466 Hz), but differed in the extent to which their underlying musical rhythmic structure was easy (regular prime) or difficult (irregular prime) to extract. In the regular prime, this structure relied on different temporal constituents of 125, 250, 500 and 1000 ms, and was reinforced by the two instruments playing simultaneously on six of the eight beats of the regular pattern. The irregular prime also contained regular intervals of 125 ms, but the occurrence of the percussion beats and the lack of simultaneously played events made it more difficult to construct a hierarchical structure. Additionally, a 32-second-long period of silence was generated using version 3.0.0 of Audacity(R) recording and editing software (Audacity Team, Citation2021) to serve as the silence condition.
Linguistic stimuli: A total of 144 Jabberwocky sentences were constructed that were either grammatically correct (N = 72) or incorrect (N = 72). Sentences of various syntactic structures were used, including simple subject-verb-object sentences (24), subject relatives (12), complement clauses (12), common (object-subject-verb) object relatives (72) and object relatives with stylistic inversion (object-verb-subject) (24). Every sentence contained a verb and 2 noun phrases (NP), one of which was the grammatical subject of the verb while the other was an attractor. The two NPs always mismatched in grammatical number such that if the subject was in the singular, the attractor was in the plural, and vice-versa. Ungrammatical sentences (50%) always contained a subject-verb number agreement violation where the verb agreed to the attractor NP instead of the subject NP. shows an overview of grammatical and ungrammatical sentences of all 5 structures. For a full list of sentences, please refer to Appendix 1.
Table 1. Examples of ungrammatical sentences of the five syntactic structures, respectively.
In every sentence, pseudo-words were used instead of nouns, but the verbs and grammatical morphemes of natural French were preserved. All of the pseudowords used were disyllabic, some were borrowed from Franck and Wagers (Citation2020)’s recent study on agreement attraction, while others were constructed such that they subscribe to the phonotactic rules of French. To ensure that the determiner preceding the pseudoword is always pronounced in its full form, none of the pseudowords were vowel-initial. Masculine (N = 152) and feminine (N = 136) pseudowords feature final syllables typical of masculine or feminine nouns in French.
Due to the structure of the experimental sentences, 132 out of 144 verbs were transitive. As the task was presented in the auditory modality, only verbs in which the difference between the third person plural and third person singular forms is phonologically realised were selected. The vast majority of our verbs were disyllabic (N = 82) or trisyllabic (N = 37), while the remaining were monosyllabic (N = 25). Monosyllabic words were not used in object relatives so as to avoid any comprehension difficulties stemming from a monosyllabic verb following a pseudoword.
Sentences were recorded with natural prosody by a female native French speaker. Noise reduction and normalisation were carried out in version 3.0.0 of Audacity(R) recording and editing software (Audacity Team, Citation2021).
Priming procedure: illustrates the priming procedure used in Experiment 1. Six sentences were presented auditorily after each prime. One miniblock contained a musical prime (or a 32-second period of silence) followed by 3 grammatical and 3 ungrammatical sentences in a randomised order. Mini blocks were constructed such that the relative ratio of different syntactic structures remained the same throughout the experiment, each containing 3 common relative clauses, 1 relative clause with stylistic inversion, 1 subject-verb-object sentence and either 1 complement clause or 1 subject relative clause. Eight mini blocks with the same musical prime were grouped into one block. Based on the order of presentation of the regular and irregular primes, two experimental lists were constructed and administered to two groups of participants (N = 21 and N = 20), respectively (8 × Regular – 8 × Silence – 8 × Irregular or 8 × Irregular – 8 × × Silence – 8 × Regular, Silence always appearing in the second block). Participants were instructed to press a response key to indicate, as quickly and accurately as possible, whether the sentence they heard was grammatical (S) or ungrammatical (K). This task was built and administered using E-Prime 3.0 software (E-Prime Inc, Citation2016).
Figure 1. Overview of the 2 experimental lists (Regular-Silence-Irregular and Irregular-Silence-Regular) used in Experiment 1. One 32-s rhythmic prime or period of silence was always followed by 6 sentences (3 common object relatives, 1 transposed object relative, 1 subject-verb-object sentence and 1 complement clause or subject relative; of which 3 grammatical and 3 ungrammatical). Eight miniblocks of the same musical prime were grouped into one block. Based on the order of presentation of the regular and irregular primes, and the two experimental lists were constructed and administered to two groups of participants, respectively
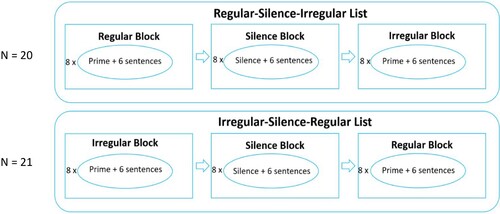
Discrimination sensitivity (d’) was computed as the dependent variable. This measure is based on the proportion of hits (correct responses for ungrammatical sentences), false alarms (incorrect responses for grammatical sentences), correct rejections (correct responses for grammatical sentences) and misses (incorrect responses for ungrammatical sentences). D’ was calculated as z(p[hits]) – z(p[FAs]), where p[hits] is the proportion of hits and p[FAs] is the proportion of false alarms. To avoid infinite values, scores of 1 were changed to 0.99 and scores of 0–0.01 (Stanislav & Todorov, Citation1999). Additionally, a participant-level index of sensitivity to the regularity of the prime (d’Regular-d’Irregular) was calculated for the correlation analyses.
Profile of music perception skills (PROMS)
The rhythm and beat (accent) subtests of the short version of the Profile of Music Perception Skills (PROMS, Law & Zentner, Citation2012; Zentner & Strauss, Citation2017) were used to evaluate participants’ ability to discriminate rhythmic and accent structures. In this task, participants heard the same rhythmic or accent sequence twice and had to decide whether the third sequence was identical or different on a 5-point Likert scale ranging from “Definitely Same” to “Definitely different”. A total of 8 rhythm and 10 beat trials were presented as per the standardised short PROMS test. This task was built and administered using LimeSurvey (LimeSurvey Project Team / Schmitz, Citation2015).
Composite scores of the PROMS rhythm subtest and the PROMS beat subtest were calculated based on the PROMS user guide. A PROMS combined score was obtained by adding up the two composite scores in accordance with the official PROMS guide.
Auditory oddball
Auditory attention was measured in an oddball paradigm in which participants heard 512 standard (600 Hz) and 128 deviant (660 Hz) tones, and were asked to report the number of deviant tones they heard. This task was built and administered using Presentation® software (Neurobehavioral Systems, Citation2021).
The dependent variable was precision calculated as the absolute value of the difference between the participant’s response and 128 (ABS(128-Response)).
General procedure
Participants were seated in a sound-isolated booth where they performed the experiment on a computer and heard the auditory stimuli through headphones. All participants completed the experimental tasks in the following order: Grammaticality judgement, Auditory Oddball, and PROMS. Experimental sessions, which included additional tasks which are not under analysis in this paper, took approximately 60 minutes.
Data analyses
Grammaticality judgement data (d’) was analysed using Linear Mixed Effects Regression Models in R (R. C. Team, Citation2018), using the lme4 package (Bates et al., Citation2015). Main effects and interactions are reported from the anova(model) function, while pairwise comparisons were realised using the emmeans package.
All models contained Participant as a random effect. As all previous rhythmic studies have reported a main effect of Prime over all 6 sentences presented after priming, an initial model with Prime (Regular vs. Silence vs. Irregular) as the only fixed effect and Participant was run on d’. Given the lack of a priming effect on 6 sentences and following visual inspection of the data, a more complex model that proved to be a significantly better fit to the data was constructed in an attempt to more precisely characterise our findings (χ²(9) = 33.545, p < 0.001). In Experiment 1, this model contained Prime (Regular vs. Silence vs. Irregular), Miniblockhalf coding sentences 1–3 and 4–6 after each prime (First vs. Second), and Order referring to the Order in which participants were exposed to the blocks of 8 identical primes (Regular-Silence-Irregular vs. Irregular-Silence-Regular). For this more complex model, d’ was calculated based on 177–208 observations in each condition (see Appendix 2 for further details).
Mixed models for grammaticality judgement d’ and descriptive statistics for additional tasks will be reported for Experiments 1 and 2, respectively. As Experiments 1 and 2 are identical with the exception of the priming paradigm (block design in Experiment 1 and mixed design in Experiment 2), correlation analyses will be reported for the two datasets put together in order to increase power.
Results
Grammaticality judgement task
All items below chance performance (0.5 accuracy) were removed from analysis. Consequently, a total of 118 out of 144 sentences per participant were preserved. Grammaticality judgement performance was generally high (M = 0.889, SD = 0.315) and its distribution right-skewed. Accuracy was generally higher for grammatical (M = 0.933, SD = 0.250) than ungrammatical (M = 0.842, SD = 0.365) trials. A total of 1 subject below 0.5 accuracy was removed.
For d’, the initial model with Prime as the only fixed effect yielded no significant main effect of Prime (F (2, 198) = 1.1423, p = 0.321), as indicated in . and shows the summary of the fixed effects of the model.
Figure 2. No significant main effect of Prime on grammaticality judgement d’ in Experiment 1. The dots connected by dashed lines represent individual participant d’ in the regular, silence, and irregular conditions, respectively.
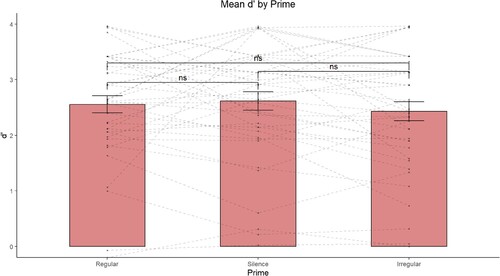
Table 2. Summary of fixed effects obtained using the summary(model) function of the lme4 package in R. Model: D’ ∼ Prime + 1|Participant.
Table 3. Main effects and interactions obtained using the anova(model) function in R. Model: D’ ∼ Prime + 1|Participant.
The model with Prime, Miniblockhalf, Order, and their interactions yielded no significant effect of Prime (F (2, 190) = 1.296, p = 0.276), a main effect of Miniblockhalf showing higher performance in sentences 1–3 after a prime than in sentences 4–6 (F (1, 190) = 4.867, p = 0.028), a significant Prime × Miniblockhalf interaction (F (2, 190) = 5.476, p = 0.005), and a significant Prime × Order interaction (F (2, 190) = 6.432, p = 0.002). Resolving the Prime × Miniblockhalf interaction with the Tukey correction showed that in the first Miniblockhalf, d’ in the Irregular condition was significantly lower than in the Regular (t = 2.956, p = 0.010) and Silence (t = 2.986 p = 0.009) conditions, with no significant differences between the Regular and Silence conditions (t = −0.031, p = 0.999). In the Second Miniblockhalf, no significant differences were found between the Prime conditions.
Resolving the Prime × Order interaction with the Tukey correction applied showed significantly lower d’ after an Irregular prime than after a Regular prime (t = 3.345, p = 0.003) and marginally lower d’ after an Irregular prime than after Silence (t = 2.189, p = 0.076) in the Irregular-Silence-Regular Order, but no significant differences between Prime conditions in the Regular-Silence-Irregular Order. shows the summary of the fixed effects of the model, while shows main effects and interactions obtained using the anova(model) function in R and .
Figure 3. Significant Prime × Miniblockhalf interaction in Experiment 1. Resolving the interaction reveals that in the first Miniblockhalf, d’ in the Irregular condition were significantly lower than in the Regular and Silence conditions, with no significant differences between the Regular and Silence conditions. In the Second Miniblockhalf, no significant differences were found between the Prime conditions. Furthermore, d’ after Silence were significantly higher in the First Miniblockhalf than in the Second, d’ in the Regular condition showed a trend in the same direction, while d’ in the Irregular condition showed a trend in the opposite direction.
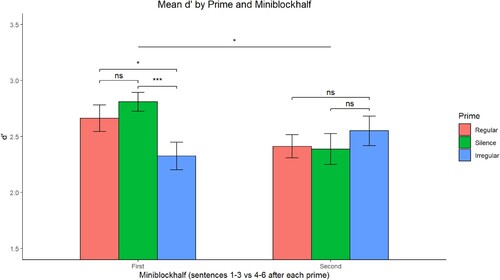
Figure 4. Significant Prime × Order interaction in Experiment 1. Resolving the interaction reveals significantly lower d’ after an Irregular prime than after a Regular prime or Silence in the Irregular-Silence-Regular Order, but no significant differences between Prime conditions in the Regular-Silence-Irregular Order. Furthermore, participants having completed the experiment in the Irregular-Silence-Regular Order underperformed those having completed the Regular-Silence-Irregular Order in the Irregular condition, but not after a Regular prime or Silence.
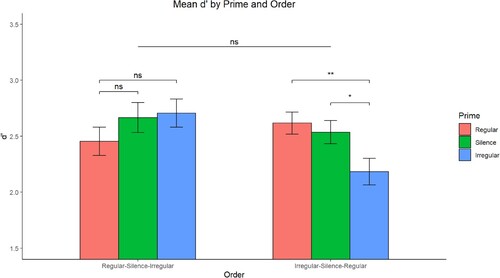
Table 4. Summary of fixed effects obtained using the summary(model) function of the lme4 package in R. Model: D’ ∼ Prime*Miniblockhalf*Order + 1|Participant.
Table 5. Main effects and interactions obtained using the anova(model) function in R. Model: D’ ∼ Prime*Miniblockhalf*Order + 1|Participant.
Profile of music perception skills
PROMS composite scores were normally distributed with no outliers (beat: W = 0.961, p = 0.170, rhythm: W = 0.967, p = 0.266, combined: W = 0.947, p = 0.056). Additionally, significant positive correlations were observed between PROMS rhythm and PROMS beat (r(41) = 0.60, p < 0.001), as well as between the combined PROMS score and both sub-tests (beat: r(41) = 0.881, p < 0.001, rhythm: r(41) = 0.888, p < 0.001).
Auditory oddball
In the Auditory Oddball task, two participants with a precision score of over 30 (an extremely high difference between the participant’s response and the correct number of deviant tones) were removed from analysis. Additionally, 3 further participants whose precision scores were over 2 standard deviations above the grand mean were also excluded from the final dataset (M = 5.630, SD = 5.758).
Discussion
Experiment 1 showed no significant priming effect when looking at all 6 sentences presented after each prime. Results showed that rhythmic priming can influence subsequent syntactic processing in Jabberwocky, specifically in the first 3 sentences that follow the rhythmic prime. This short-lived nature of the rhythmic priming effect contrasts with previous studies on children (Bedoin et al., Citation2016; Canette, Lalitte, et al., Citation2020; Chern et al., Citation2018; Ladányi et al., Citation2021; Przybylski et al., Citation2013) and adults (Canette, Bedoin, et al., Citation2020), which have thus far reported priming effects spanning across entire miniblocks of 4 or 6 sentences (and have therefore not reported how the priming effect evolved over the sentences that followed each prime). Interestingly, unlike previous studies that have used a baseline, the effect did not appear to be a facilitatory effect of rhythmic regularity: we observed lower performance in the Irregular condition compared to both the Regular and, albeit marginally, the Silence conditions rather than an advantage of the Regular condition over the Irregular prime and the Silent baseline. At least two crucial differences in population and stimuli can be identified between these studies and the present study. Experiments showing a beneficial effect of regular rhythmic priming over baseline tested children processing natural language, while we tested typical adults processing Jabberwocky. Typical adults could be closer to ceiling in their first language, thus leaving more room for a penalising effect of the irregular prime and less room for improvement due to regular rhythmic priming. Reducing lexical semantics by using Jabberwocky may have also changed the underlying mechanisms of rhythmic priming (see General discussion).
A third potential explanation lies in a confound introduced by the use of a block design. As reported above, when looking at all 6 sentences, the data showed a difference between the regular and irregular (and silence) conditions in the Irregular-Silence-Regular order, but not in the Regular-Silence-Irregular order. Due to the nature of the design, experimental blocks corresponded to Prime conditions. As such, we could reinterpret this observation by saying that in the Irregular-Silence-Regular order, performance in Block 1 of the experiment was lower than that in Blocks 2 and 3. Therefore, lower performance in this first block could be explained by a penalising effect of the irregular prime, a simple task-learning effect, or a combination of both. As Silence always appeared in the second block (i.e. never in the first), its position within our design may have introduced a confound, resulting in inflated performance in the Silence condition and thus preventing us from reliably comparing all 3 Prime conditions. To explore this hypothesis, and to rule out the possibility that the block design also played a role in the short-lived priming effect, Experiment 2 was built in a mixed design, similarly to what is typically used in that literature. In this design, mini blocks of the 3 different prime conditions alternated in one of the six possible order combinations, counterbalanced across participants, such that it would allow us to compare all 3 Prime conditions regardless of the order of presentation (8 × (Regular-Silence-Irregular), 8 × (Regular-Irregular-Silence), etc.). Experiment 2 was otherwise identical to Experiment 1.
Experiment 2: mixed design
Participants
Fifty-one native French-speaking neurotypical adults participated in the experiment. Participants were between 18–42 (M = 25.496, SD = 6.151) years of age and reported no history of psychiatric issues, neurological disorders, specific language impairment, hearing impairments, or amusia.
Participants gave informed consent prior to the start of the experiment, were not made aware of the purpose of the study, and compensated either course credits or 20 CHF for their time. The experiment was approved by the University of Geneva Research Ethics Committee (PSE.20191004.04).
Materials, procedure and data analyses
Grammaticality judgement task
Priming procedure: illustrates the priming procedure used in Experiment 2. Like in Experiment 1, one mini block contained a musical prime (or a 32-second period of silence) followed by 3 grammatical and 3 ungrammatical sentences in a randomised order. Mini blocks were constructed such that the relative ratio of different syntactic structures remained the same throughout the experiment, each containing 3 common relative clauses, 1 relative clause with stylistic inversion, 1 subject-verb-object sentence and either 1 complement clause or 1 subject relative clause. Unlike in Experiment 1, 3 mini blocks of the three different prime conditions were presented in an alternating order, which remained the same throughout the experiment in a given experimental list. Based on the order of presentation of the prime conditions (8 × (Regular-Silence-Irregular), 8 × (Regular-Irregular-Silence), 8 × (Irregular-Regular-Silence), 8 × (Irregular-Silence-Regular), 8 × (Silence-Irregular-Regular), 8 × (Silence-Regular-Irregular)), six experimental lists were constructed and distributed evenly across participants.
Figure 5. Example of one of the 6 experimental lists used in Experiment 2. One 32-s rhythmic prime or period of silence was always followed by 6 sentences (3 common object relatives, 1 transposed object relative, 1 subject-verb-object sentence and 1 complement clause or subject relative; of which 3 grammatical and 3 ungrammatical). Three miniblocks of the 3 different prime conditions were presented in an alternating order, which remained the same throughout the experiment in a given list. Based on the order of presentation of the prime conditions (8 × (Regular-Silence-Irregular), 8 × (Regular-Irregular-Silence), 8 × (Irregular-Regular-Silence), 8 × (Irregular-Silence-Regular), 8 × (Silence-Irregular-Regular), 8 × (Silence-Regular-Irregular)), six experimental lists were constructed and distributed evenly across participants
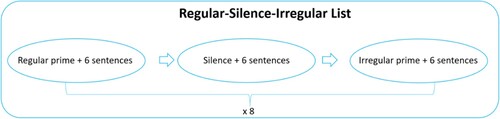
Experiment 2 was otherwise identical to Experiment 1, using the same tasks (rhythmically primed grammaticality judgement, auditory oddball, and PROMS) in the same order.
Data analyses
Like in Experiment 1, all mixed models used to analyse grammaticality judgement d’ contained Participant as a random effect. As all previous rhythmic studies have reported a main effect of Prime over all 6 sentences presented after priming, an initial model with Prime (Regular vs. Silence vs. Irregular) as the only fixed effect and Participant was run on d’. Given the lack of a priming effect on 6 sentences and following visual inspection of the data, a more complex model that proved to be a significantly better fit to the data was constructed in an attempt to more precisely characterise our findings (χ²(3) = 28.724, p < 0.001). In Experiment 2, this model contained Prime (Regular vs. Silence vs. Irregular) and Miniblockhalf coding sentences 1–3 and 4–6 after each prime (First vs. Second). For this more complex model, d’ was calculated based on 415–485 observations in each condition (see Appendix 3 for further details).
Results
Grammaticality judgement task
All items below chance performance (0.5 accuracy) were removed from analysis. Additionally, due to low and inconsistent performance as well as feedback from participants that these sentence structures were very unnatural in spoken French, all object relative sentences with stylistic inversion were also removed from analysis. A total of 25 out of 144 sentences per participant were removed. Grammaticality judgement performance was generally high (M = 0.906, SD = 0.292) and its distribution right-skewed. Accuracy was generally higher for grammatical (M = 0.946, SD = 0.225) than ungrammatical (M = 0.865, SD = 0.342) sentences. A total of 6 participants below 0.5 accuracy were removed.
The initial model on d’ with Prime as the only fixed effect showed no significant effect of Prime (F (2, 763) = 1.167, p = 0.312). shows the summary of the fixed effects of the model, while shows the main effects of Prime obtained using the anova(model) function in R ().
Figure 6. No significant main effect of Prime on grammaticality judgement d’ in Experiment 2. The dots connected by dashed lines represent individual participant d’ in the regular, silence, and irregular conditions, respectively.
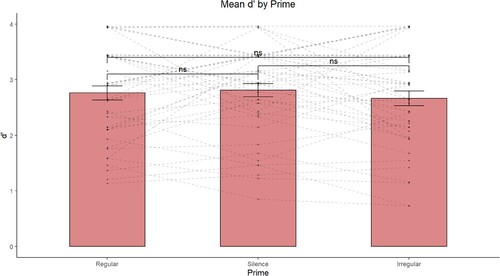
Table 6. Summary of fixed effects obtained using the summary(model) function of the lme4 package in R. Model: D’ ∼ Prime + 1|Participant.
Table 7. Main effects and interactions obtained using the anova(model) function in R. Model: D’ ∼ Prime + 1|Participant.
The model with Prime, Miniblockhalf and their interaction yielded no significant effect of Prime (F (2, 760) = 1.207, p = 0.300), a significant main effect of Miniblockhalf showing higher performance in the First than in the second Miniblockhalf (F (1, 760) = 13.276, p < 0.001), and a significant Prime × Miniblockhalf interaction (F (2, 760) = 7.902, p < 0.001). Post-hoc tests with the Tukey correction showed that in the first Miniblockhalf, d’ after a Regular prime was significantly higher than after an Irregular prime (t = 3.433, p = 0.002), with no other significant differences between the other conditions. In the Second Miniblockhalf, d’ after a Regular prime was lower than after Silence (t = −2.379, p = 0.046), with no significant differences between the other conditions. Furthermore, d’ after a Regular prime was significantly higher in the First Miniblockhalf than in the Second (t = 5.263, p < 0.001), while there were no significant differences between the First and Second miniblockhalves in the Irregular (t = −0.121, p = 0.903) and Silence (t = 1.170, p = 0.243) conditions. shows the summary of the fixed effects of the model, while shows main effects and interactions obtained using the anova(model) function in R ().
Figure 7. Significant Prime × Miniblockhalf interaction in Experiment 2. Resolving the interaction reveals that in the first Miniblockhalf, d’ after a Regular prime were significantly higher than after an Irregular prime, with no significant differences between the other two conditions. In the Second Miniblockhalf, d’ after a Regular prime were lower than after Silence, with no significant differences between the other conditions. Furthermore, d’ after a Regular prime were significantly higher in the First Miniblockhalf than in the Second, while there were no significant differences between the First and Second Miniblocks in the Irregular and Silence conditions.
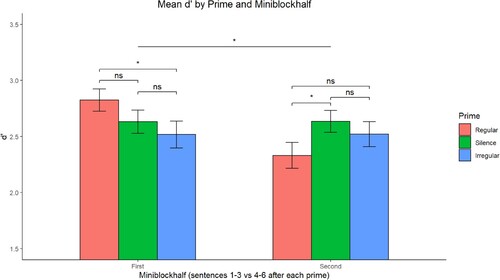
Table 8. Summary of fixed effects obtained using the summary(model) function of the lme4 package in R. Model: D’ ∼ Prime*Miniblockhalf + 1|Participant.
Table 9. Main effects and interactions obtained using the anova(model) function in R. Model: D’ ∼ Prime*Miniblockhalf + 1|Participant.
Profile of Music Perception Skills: PROMS composite scores were normally distributed with no outliers (beat: W = 0.966, p = 0.145, rhythm: W = 0.976, p = 0.392, combined: W = 0.981, p = 0.578). Additionally, significant positive correlations were observed between PROMS rhythm and PROMS beat (r(51) = 0.505, p < 0.001), as well as between the combined PROMS score and both sub-tests (beat: r(51) = 0.876, p < 0.001, rhythm: r(51) = 0.842, p < 0.001).
Auditory oddball: In the Auditory Oddball task, two participants with a precision score of over 30 (an extremely high difference between the participant’s response and the correct number of deviant tones) were removed from analysis. Additionally, 3 further participants whose precision scores were over 2 standard deviations above the grand mean were also excluded. This resulted in a total of 46 participants in the final dataset (M = 6.638, SD = 6.631).
Discussion
Experiment 2 was run to address a potential confound stemming from the block design of Experiment 1. Results showed (1) that rhythmic priming influenced grammaticality judgement d’ only in the first 3 sentences after the prime and (2) that in the first 3 sentences, grammaticality judgement performance was higher after a regular prime than after an irregular prime, with the silent baseline condition not significantly different from either.
Replicating Experiment 1, Experiment 2 showed a significant rhythmic priming effect only in the first 3 sentences following the prime, but not across all 6. These results are in contrast with studies reporting a rhythmic priming effect over 6 sentences in children (Bedoin et al., Citation2016; Canette, Lalitte, et al., Citation2020; Chern et al., Citation2018; Ladányi et al., Citation2021; Przybylski et al., Citation2013) and adults (Canette, Bedoin, et al., Citation2020). At least two key differences can be identified between the present study and those reporting a rhythmic priming effect over 6 sentences. As discussed before, one key difference between our study and those reporting a longer-lasting effect is the use of Jabberwocky. It is possible that reducing lexical semantics may have influenced the underlying mechanisms of rhythmic priming, thus reducing the duration of the effect. Another potential explanation may lie in the combination of the population and the material. Canette, Bedoin et al. (Citation2020) reported a behavioural rhythmic priming effect on adults processing sentences with more subtle morphosyntactic errors, while Canette, Fiveash et al. (Citation2021) found that in simpler sentences featuring subject-verb number agreement errors, rhythmic priming affects ERP-responses to ungrammatical sentences but not behavioural data. Both of these points are discussed in greater detail in the General Discussion.
As for the direction of the effect, Experiment 2 showed higher grammaticality judgement d’ after a Regular prime than after an Irregular prime, with Silence not significantly different from either. On the one hand, this result suggests that the primarily penalising effect observed in Experiment 1 was indeed due to a confound caused by the block design. On the other hand, unlike rhythmic priming experiments on children processing natural language sentences that report an advantage of Regular priming compared to Irregular priming and baseline, the present study on adults processing Jabberwocky shows that grammaticality judgement performance after Silence is between the two rhythm conditions, not significantly differing from either. In other words, it is possible that in adults processing Jabberwocky sentences, the rhythmic priming effect has two components: a beneficial effect of the Regular prime and a penalising effect of the Irregular prime. This point is discussed further in the General Discussion.
Experiments 1 and 2: correlations between linguistic and non-linguistic measures
Profile of Music Perception Skills: In the complete dataset with no outliers removed, PROMS composite scores were normally distributed with no outliers in any of the PROMS scores (beat: W = 0.977, p = 0.152, rhythm: W = 0.978, p = 0.192, combined: W = 0.983, p = 0.351).
Auditory oddball: In a total of 72 participants after cleaning, Oddball scores showed a non-normal distribution (M = 4.528, SD = 3.688, W = 0.735, p < 0.001).
Relationships between our tasks were evaluated through simple Spearman correlation analyses using the Hmisc package in R (69).
Grammaticality judgement d’ showed a significant positive correlation with PROMS beat (r(85) = 0.399, p < 0.001), PROMS rhythm (r(85) = 0.320, p = 0.003) and the combined measure (r(85) = 0.391, p < 0.001). These correlations remained significant when controlling for auditory selective attention (beat: r(76) = 0.383, p < 0.001, rhythm: r(76) = 0.375, p < 0.001, combined: r(76) = 0.410, p < 0.001). Looking separately at correlations between rhythmic skills and GJ performance after a regular and an irregular primes, the correlations between grammaticality judgement d’ in the irregular condition and PROMS beat (r(85) = 0.427, p < 0.001), PROMS rhythm (r(85) = 0.488, p < 0.001) and PROMS combined (r(85) = 0.497, p < 0.001) were stronger than those between Ggrammaticality judgement d’ after a regular prime (beat: r(85) = 0.314, p = 0.006; rhythm: r(85) = 0.264, p = 0.021; combined: r(85) = 0.319, p = 0.005). The pattern remained similar after controlling for auditory attention: rhythmic skills showed a stronger correlation with grammaticality judgement d’ after an irregular prime (beat: r(76) = 0.426, p < 0.001, rhythm: r(76) = 0.487, p < 0.001, combined: r(76) = 0.496, p < 0.001) than after a regular prime (beat: r(76) = 0.317, p = 0.006, rhythm: r(76) = 0.268, p = 0.020, combined: r(76) = 0.323, p = 0.005). The PROMS rhythm and PROMS combined scores also showed a trend for a negative correlation with sensitivity to the regularity of the rhythmic prime: the better participants performed in PROMS rhythm (r(85) = −0.223, p = 0.039) and combined (r(85) = −0.183, p = 0.095), the weaker the overall priming effect observed. Similar correlations were observed when controlling for auditory selective attention (beat: r(76) = −0.168, p = 0.149, rhythm: r(76) = −0.279, p = 0.015, combined: r(76) = −0.239, p = 0.039).
Oddball scores showed no significant correlations with overall grammaticality judgement d’ (r(76) = −0.044, p = 0.706) or sensitivity to regularity (r(76) = −0.113, p = 0.331). However, when looking at only the first three sentences after the prime, where both experiments showed a significant priming effect, results showed a trend that the more attentive participants were in the Oddball task, the stronger the overall rhythming priming effect was observed (r(76) = −0.205, p = 0.075). Interestingly, this effect specifically lies in the link between the attentional task and the regular condition, as indicated by the positive correlation between the Oddball task and d’ after a Regular prime (r(76) = −0.249, p = 0.030), but the lack of correlation after an Irregular prime (r(76) = 0.008, p = 0.947).
General discussion
The present study explored the hypothesis that there is a cognitive system responsible for coding abstract hierarchical structure, which is recruited by both language and musical rhythm processing (Asano et al., Citation2021; Fitch & Martins, Citation2014; Heard & Lee, Citation2019; Martins et al., Citation2017). We conducted two experiments that allowed us to specifically explore three predictions. According to Prediction 1, exposure to a rhythmically regular musical prime (whose underlying hierarchical structure is easier to extract) should activate this system, thus facilitating subsequent syntactic (hierarchical) processing. Alternatively, it is also possible that exposure to a rhythmically irregular prime would penalise this system, reducing syntactic performance in the irregular condition. Following Prediction 2, rhythm and beat discrimination skills are expected to correlate with overall grammaticality judgement performance and sensitivity to the regularity of the rhythmic prime are expected to correlate. Finally, Prediction 3 was that if the priming effect is at least partly an attentional effect, there would be a correlation between participants’ auditory selective attention and their sensitivity to the regularity of the prime. Our main interpretations of the observations made in the present study are as follows: (1) exposure to a rhythmically regular musical prime facilitates syntax processing in the three sentences immediately following the prime (but this effect diminishes in sentences 4-6) and (2) this facilitation effect correlates with participants’ auditory selective attention. (3) influence from a rhythmically irregular musical prime hinders subsequent syntactic processing, and (4) this inhibitory effect of an irregular prime is possibly compensated for by participants’ ability to extract musical rhythmic structures. We will now move on to summarising data points relevant to these interpretations and discussing them in greater detail.
Duration and direction of the priming effect
Data from both experiments of the present study showed that rhythmic priming can influence syntactic processing in Jabberwocky sentences, replicating results from natural language studies (Bedoin et al., Citation2016; Canette, Bedoin, et al., Citation2020; Przybylski et al., Citation2013). However, in both experiments, the observed priming effect was more short-lived than that reported in the rhythmic priming literature. While priming studies usually report effects throughout all of the 4, 6, or even 10 sentences presented after each prime, our results showed that the effect was restricted to the first 3 sentences presented after the prime. To our knowledge, the present study is the first to report on the evolution of the rhythmic priming effect over the 6 sentences presented after priming.
The results of the block and mixed design experiments differed in the direction of the observed priming effect. Experiment 1 showed a disadvantage of the irregular condition compared to both the regular and silence conditions. Experiment 2 showed higher grammaticality judgement accuracy in the regular than in the irregular condition, with no significant difference between the baseline and either prime condition. Given that GJ d’ in the baseline condition of Experiment 2 was right in between the two prime conditions, it suggests that in typical adults processing Jabberwocky sentences, the rhythmic priming effect is a combination of a facilitatory effect from the regular prime and a penalising effect of the irregular prime.
These results provide further evidence for a rhythmic priming effect in typical adults, though it seems that (1) the effect is shorter than the effects reported in earlier studies and (2) the precise direction of the effect is susceptible to the experimental design. Both of these points are discussed in greater detail in the sections below.
One key finding of the current study is that rhythmic priming can influence syntactic processing in Jabberwocky sentences in typical adults, but the duration of the effect appears to be shorter than the blocks of 6 sentences usually reported in the literature. Importantly, this finding was replicated in both the block and mixed design experiments, so the choice of design is unlikely to have influenced this result. In both experiments, this effect boiled down to higher performance in the Regular condition than in the irregular condition in sentences 1-3, which was reduced in sentences 4-6. One key difference between the current study and other rhythmic priming studies is the use of Jabberwocky sentences. Therefore, our finding suggests that it is possible that by attempting to focus on syntactic processing and reducing lexical semantic information, it was harder to construct the underlying hierarchical representation of the sentences. As mentioned before, converging evidence suggests that neural oscillations in different frequency bands track different levels of hierarchical (covert prosodic or syntactic) structure even if the speech signal contains no overt acoustic cues to these levels (Ding et al., Citation2016; Glushko et al., Citation2020). Interestingly, one recent Dutch study has shown that neural entrainment to these constituents is weaker in Jabberwocky than in natural Dutch sentences (Kaufeld et al., Citation2020). The shorter rhythmic priming effect reported in the present study may be another manifestation of the role of semantic information in hierarchical structure building, under the assumption that a weaker semantic structure provides a weaker ground on which a regular rhythm can imprint its hierarchical structure. While semantic information is not necessary for syntactic structure building, it may provide supporting cues, the absence of which might slightly penalise structure building. If the RPE is realised through a domain-general system responsible for hierarchical structure building, removing this supporting information from the material may have also influenced the RPE.
Alternatively, it may also be argued that the combination of testing a typical adult population and using highly repetitive subject-verb number agreement errors (as opposed to a combination of gender assignment, subject-verb number agreement and subject-verb person agreement used by several studies) has led to higher grammaticality judgement performance. Given the close-to-ceiling performance, there may have been less room for a priming effect. Indeed, recent priming studies on adults report no behavioural priming effect when using simple agreement errors, but they observe a behavioural effect when using more varied morphosyntactic errors (Canette, Bedoin, et al., Citation2020; Canette, Fiveash, et al., Citation2020). Nevertheless, grammaticality judgement performance in both experiments of the current study was generally higher in the first three sentences than in the last three sentences after each prime. As the observed rhythmic priming effect occurred in the sentences 1–3 where performance was generally higher, a potential ceiling effect is unlikely to account for the shortness of the priming effect reported here: if a ceiling effect was indeed to blame, that would probably manifest in the lack of a priming effect where overall performance is higher, not where it is lower. Furthermore, while the point at which participants have heard all the information necessary to judge whether the sentence was grammatical or not varied between structures (end of the sentence for object relative clauses, third to last word for subject relatives and simple subject-verb-object sentences), most of the present material consisted in object relative clauses. This distribution may have influenced participants’ listening strategies towards locally attending to the potential source of ungrammaticality. Nevertheless, grammaticality judgement accuracy was relatively stable across structures, though this is not directly comparable given the uneven distribution of syntactic structures in the present material.
Additionally, while the primes developed by Przybylski et al. (Citation2013) have repeatedly been used to generate a priming effect in typical and atypical children, it is also possible that the structural difference between the regular and the irregular prime is simply not enough to influence a fully developed structure processing system. Using primes whose musical rhythmic structures differ in clear and precise points may help bring further insight to this issue. Recent studies on children and adults have chosen to use more musical, more varied and multi-layered primes, creating irregular primes by randomising the order of acoustic events in each layer (Canette, Bedoin, et al., Citation2020; Canette, Fiveash, et al., Citation2020; Canette, Lalitte, et al., Citation2020). Introducing precise stepwise changes between the regular and irregular primes used in these experiments may help uncover what aspect(s) or musical rhythmic regularity contribute to the rhythmic priming effect.
One potential limitation of the analysis reported in the present study stems from the distribution of grammatical and ungrammatical sentences within each Miniblock. While the present material was controlled such that each Miniblock contained exactly 3 grammatical and 3 ungrammatical sentences, the same cannot be said for the distribution of grammatical and ungrammatical sentences in each Miniblock half, i.e. sentences 1–3 vs. 4–6 after each Prime. An uneven distribution of grammatical and ungrammatical sentences between sentences 1–3 and 4–6 may have influenced our results given the generally higher performance in grammatical sentences. Post-hoc analyses of the material show that randomisation of the present study resulted in a relatively, though not precisely, even distribution of grammatical and ungrammatical sentences. Future studies seeking to investigate the evolution of the RPE after the prime may consider controlling for this distribution more precisely.
Shared resources between rhythm and syntax: attention and structure?
In line with Prediction 2 and with previous findings in children (Gordon et al., Citation2015; Lee et al., Citation2020), the present data showed that grammaticality judgement accuracy correlated with rhythm and beat discrimination in typical adult participants, even after controlling for auditory selective attention. Additionally, we predicted that sensitivity to the regularity of the prime in the grammaticality judgement task would show a relationship with participants’ ability to process musical rhythmic structure. The results showed trends for a lower priming effect the better our participants were at rhythm and beat discrimination. Looking more closely at the data revealed that this effect stemmed from a stronger, positive correlation between rhythm and beat discrimination and grammaticality judgement performance in the irregular condition compared to the regular condition. In other words, it seems that participants who excel at building musical rhythmic structure are less disturbed by the presence of the irregular prime in the extraction of syntactic structure. Interestingly, this data point seems to suggest that, at least in typical adults processing Jabberwocky sentences, part of the rhythmic priming effect may lie in the penalising effect of the irregular prime, in line with our finding of a penalising effect in Experiment 1. Alternatively, it is also possible that as participants who excel building musical rhythmic structures also perform better at grammaticality judgement to begin with, there is less room for regular rhythmic stimulation to improve their already high performance. However, as noted before, a ceiling effect is unlikely to account for the present data as we observe a priming effect in sentences where performance is higher, not where it is lower. As noted above, it is possible that the priming effect observed in Experiment 2 is a combination of a facilitatory effect from the regular prime and a penalising effect of the irregular prime. Therefore, both of these explanations might contribute to the differences observed between rhythm discrimination abilities and grammaticality judgement performance following regular and irregular priming, respectively. In either case, the finding that the participants’ ability to discriminate rhythm and accent structures correlates with grammaticality judgement performance provides evidence for an overlap between rhythm and syntax processing in typical adults, similar to observations in the developmental literature (Gordon et al., Citation2015; Lee et al., Citation2020). It appears that this relationship is somewhat specific to rhythm and syntax, or at least that a general explanation based on auditory (selective) attention could not account for the results of the present study as the correlations remained stable after attention was controlled for in partial correlations. A shared system responsible for hierarchical structure processing may account for these results, especially as both PROMS and the grammaticality judgement task require that participants build internal structural (metrical or syntactic) representations to give a correct response.
In line with Prediction 3, data showed that in sentences 1–3 after the prime (which is where the expected priming effect was observed in our data), participants with better selective attention showed a greater priming effect. Specifically, these participants benefited more from the regular prime, suggesting that at least part of the priming effect we observed in typical adults processing Jabberwocky sentences stemmed from a facilitatory effect of regular priming, modulated by participants’ attentional resources. Taken together, these two data points paint a complex picture of the effects of rhythmic priming in typical adults processing Jabberwocky sentences: on the one hand, a regular rhythmic prime seems to improve subsequent syntactic performance, and this improvement is related to selective auditory attention. On the other hand, irregular rhythmic priming seems to penalise syntactic performance, but good musical rhythm structure processing skills are able to compensate for this effect.
A large number of rhythmic priming studies interpret their results within the framework of Dynamic Attending Theory (Jones & Boltz, Citation1989; Large & Jones, Citation1999). DAT proposes that attention can be described as a system of self-sustaining internal attentional oscillations which vary in oscillatory phase and period, and which are able to entrain to each other and external oscillators in the input. These priming papers suggest that entrainment to the musical rhythmic structure of a regular prime allows orienting attention in time, generating temporal predictions as to the occurrence of the next event. These predictions would then facilitate the processing, segmentation, sequencing, and integration of the speech signal. Specifically, entrainment of attentional oscillations at the beat level and related metrical levels are suggested to correspond to linguistic units in naturally spoken speech stimuli (Fiveash et al., Citation2021). However, in these rhythmic priming studies and in the present study, there is no direct correspondence between the musical rhythmic structure of the prime rhythm and that of the language stimuli. It has been reported that 2 Hz (the beat frequency of the primes) and 4 Hz (the harmonic and acoustic energy used in the primes) are relevant oscillatory frequencies to the alternation of accented syllables and to the average syllable rate, respectively (Canette, Bedoin, et al., Citation2020; Fiveash et al., Citation2021). However, it seems unclear precisely how these entrained oscillators would benefit behavioural grammaticality judgement performance, which has been reported to be unaffected by several speech rhythm manipulations (Kotz & Schmidt-Kassow, Citation2015; Roncaglia-Denissen et al., Citation2013; Roncaglia-Denissen et al., Citation2015). Alternatively, it is also possible that the rhythmic priming effect is realised through a cognitive system responsible for the construction of hierarchical structures. This shared cognitive system might include neurocognitive mechanisms such as hierarchical cognitive control and hierarchically organised prediction (Asano et al., Citation2021).
One recently proposed explanation for the facilitatory effect of a regular rhythm is twofold: on the one hand, a highly regular rhythm makes it possible for highly automatic processes to construct its metrical representation, thus relieving resources responsible for hierarchical control and enabling more of these resources to be used during subsequent syntax processing (Asano et al., Citation2021). Conversely, if the system is exposed to an irregular prime, purely automatic structure building processes fail to construct its metrical representation, and fewer resources will be available to be dedicated to syntactic processing, thus penalising performance. On the other hand, the highly predictable structure of the regular rhythmic prime could also actively support syntactic structure building by providing a predictable metrical grid (though the precise nature of the interplay between a predictable metrical structure and discrete rule-based predictions syntactic predictions is yet to be fully understood), thus improving syntactic performance (Asano et al., Citation2021; Kotz et al., Citation2009). Regardless of whether a DAT-based account, hierarchical cognitive control-based explanation or both can provide an adequate account for rhythmic priming, data from the present study seems to indicate that the beneficial effect of regular rhythmic priming is related to auditory selective attention.
Conclusion
The present study provides evidence that in typical adults processing Jabberwocky sentences, the rhythmic priming effect consists of at least two components: we observed that (1) exposure to a rhythmically regular musical prime facilitates subsequent syntax processing over about three sentences that follow a regular prime (which then diminishes in sentences 4-6), and (2) this facilitation effect correlates with participants’ attention. Furthermore, (3) influence from a rhythmically irregular musical prime hinders subsequent syntactic processing, and (4) this inhibitory effect of an irregular prime is compensated for by participants’ ability to extract musical rhythmic structures. Further research is necessary to gain more insights into the precise nature of the priming effect(s) in an attempt to construct a functional framework that adequately describes all of its dimensions, including the extent to which it is modulated by individual differences such as (but also beyond) rhythmic abilities and attention.
Supplemental Material
Download MS Word (200.9 KB)Acknowledgements
We would like to thank Teresa Guasti, Mauricio Martins, Daphné Bavelier, Antonella Sorace, Barbara Tillmann, and Diego Krivochen for their invaluable insight into rhythm processing, language processing and hierarchical structure building. We would also like to thank Lna Ali, Fabrice Guibentif, and Marie de Tellier for their work on the materials, data collection and analysis, as well as their scientific curiosity.
Disclosure statement
No potential conflict of interest was reported by the author(s).
Additional information
Funding
References
- Altmann, C. F., Bülthoff, H. H., & Kourtzi, Z. (2003). Perceptual organization of local elements into global shapes in the human visual cortex. Current Biology, 13(4), 342–349. https://doi.org/10.1016/S0960-9822(03)00052-6
- Asano, R., Boeckx, C., & Seifert, U. (2021). Hierarchical control as a shared neurocognitive mechanism for language and music. Cognition, 216, 104847. https://doi.org/10.1016/j.cognition.2021.104847
- Audacity Team. (2021). Audacity(R): Free Audio Editor and Recorder [Computer application] (Version 3.0.0). Audacity® software is copyright © 1999-2021 Audacity Team. Web site: https://audacityteam.org/ It is free software distributed under the terms of the GNU General Public License. The name Audacity® is a registered trademark
- Bates, D., Maechler, M., Bolker, B., Walker, S., Christensen, R. H. B., Singmann, H., & Al, E. (2015). Package “lme4”:Linear Mixed-Effects Models Using ‘Eigen’ and S4. https://cran.r-project.org/web/packages/lme4/index.html
- Bedoin, N., Brisseau, L., Molinier, P., Roch, D., & Tillmann, B. (2016). Temporally regular musical primes facilitate subsequent syntax processing in children with Specific Language Impairment. Frontiers in Neuroscience, 10, 1–11. https://doi.org/10.3389/fnins.2016.00245
- Bock, K., & Miller, C. A. (1991). Broken agreement. Cognitive Psychology, 23(1), 45–93. http://doi.org/10.1016/0010-0285(91)90003-7
- Canette, L. H., Bedoin, N., Lalitte, P., Bigand, E., Tillmann, B., Bedoin, N., Lalitte, P., & Bigand, E. (2020). The regularity of rhythmic primes influences syntax processing in adults. Auditory Perception & Cognition, 2(3), 163–179. https://doi.org/10.1080/25742442.2020.1752080
- Canette, L. H., Fiveash, A., Krzonowski, J., Corneyllie, A., Lalitte, P., Thompson, D., Trainor, L., Bedoin, N., & Tillmann, B. (2020). Regular rhythmic primes boost P600 in grammatical error processing in dyslexic adults and matched controls. Neuropsychologia, 138. https://doi.org/10.1016/j.neuropsychologia.2019.107324
- Canette, L. H., Lalitte, P., Bedoin, N., Pineau, M., Bigand, E., & Tillmann, B. (2020). Rhythmic and textural musical sequences differently influence syntax and semantic processing in children. Journal of Experimental Child Psychology, 191, 104711. https://doi.org/10.1016/j.jecp.2019.104711
- Cason, N., Astésano, C., & Schön, D. (2015). Bridging music and speech rhythm: Rhythmic priming and audio-motor training affect speech perception. Acta Psychologica, 155, 43–50. https://doi.org/10.1016/j.actpsy.2014.12.002
- Cason, N., Hidalgo, C., Isoard, F., Roman, S., & Schön, D. (2015). Rhythmic priming enhances speech production abilities: Evidence from prelingually deaf children. Neuropsychology, 29(1), 102–107. https://doi.org/10.1037/neu0000115
- Cason, N., & Schön, D. (2012). Rhythmic priming enhances the phonological processing of speech. Neuropsychologia, 50(11), 2652–2658. https://doi.org/10.1016/j.neuropsychologia.2012.07.018
- Chern, A., Tillmann, B., Vaughan, C., & Gordon, R. L. (2018). New evidence of a rhythmic priming effect that enhances grammaticality judgments in children. Journal of Experimental Child Psychology, 173, 371–379. https://doi.org/10.1016/j.jecp.2018.04.007
- Corriveau, K. H., & Goswami, U. (2009). Rhythmic motor entrainment in children with speech and language impairments: Tapping to the beat. Cortex, 45(1), 119–130. https://doi.org/10.1016/j.cortex.2007.09.008
- Ding, N., Melloni, L., Zhang, H., Tian, X., & Poeppel, D. (2016). Cortical tracking of hierarchical linguistic structures in connected speech. Nature Neuroscience, 19(1), 158–164. https://doi.org/10.1038/nn.4186
- E-Prime Inc. (2016). E-Prime 3.0.
- Fitch, W. T., & Friederici, A. D. (2012). Artificial grammar learning meets formal language theory: An overview. Philosophical Transactions of the Royal Society B: Biological Sciences, 367(1598), 1933–1955. https://doi.org/10.1098/rstb.2012.0103
- Fitch, W. T., & Martins, M. D. (2014). Hierarchical processing in music, language, and action: Lashley revisited. Annals of the New York Academy of Sciences, 1316(1), 87–104. https://doi.org/10.1111/nyas.12406
- Fiveash, A., Bedoin, N., Gordon, R. L., & Tillmann, B. (2021). Processing rhythm in speech and music: Shared mechanisms and implications for developmental speech and language disorders Anna. Neuropsychology. http://www.tjyybjb.ac.cn/CN/article/downloadArticleFile.do?attachType=PDF&id=9987
- Flaugnacco, E., Lopez, L., Terribili, C., Zoia, S., Buda, S., Tilli, S., Monasta, L., Montico, M., Sila, A., Ronfani, L., & Schön, D. (2014). Rhythm perception and production predict Reading abilities in developmental dyslexia. Frontiers in Human Neuroscience, 8, 1–14. https://doi.org/10.3389/fnhum.2014.00392
- Franck, J., Colonna, S., & Rizzi, L. (2015). Task-dependency and structure-dependency in number interference effects in sentence comprehension. Frontiers in Psychology, 6. http://doi.org/10.3389/fpsyg.2015.00349
- Franck, J., Lassi, G., Frauenfelder, U. H., & Rizzi, L. (2006). Agreement and movement: A syntactic analysis of attraction. Cognition, 101(1), 173–216. https://doi.org/10.1016/j.cognition.2005.10.003
- Franck, J., Soare, G., Frauenfelder, U. H., & Rizzi, L. (2010). Object interference in subject-verb agreement: The role of intermediate traces of movement. Journal of Memory and Language, 62(2), 166–182. https://doi.org/10.1016/j.jml.2009.11.001
- Franck, J., & Wagers, M. (2020). Hierarchical structure and memory mechanisms in agreement attraction. PLoS One, 15(5), e0232163. https://doi.org/10.1371/journal.pone.0232163
- Fujii, S., & Wan, C. Y. (2014). The role of rhythm in speech and language rehabilitation: The SEP hypothesis. Frontiers in Human Neuroscience, 8, 1–15. https://doi.org/10.3389/fnhum.2014.00777
- Glushko, A., Poeppel, D., & Steinhauer, K. (2020). Overt and covert prosody are reflected in neurophysiological responses previously attributed to grammatical processing. BioRxiv. https://doi.org/10.1101/2020.09.17.301994
- Gordon, R. L., Shivers, C. M., Wieland, E. A., Kotz, S. A., Yoder, P. J., & Devin Mcauley, J. (2015). Musical rhythm discrimination explains individual differences in grammar skills in children. Developmental Science, 18(4), 635–644. https://doi.org/10.1111/desc.12230
- Grahn, J. A., & Brett, M. (2009). Impairment of beat-based rhythm discrimination in Parkinson’s disease. Cortex, 45(1), 54–61. https://doi.org/10.1016/j.cortex.2008.01.005
- Hauser, M. D., Chomsky, N., & Fitch, W. T. (2010). The faculty of language: What is it, who has it, and how did it evolve? The Evolution of Human Language: Biolinguistic Perspectives, 298, 14–42. https://doi.org/10.1017/CBO9780511817755.002
- Heard, M., & Lee, Y. S. (2019). Shared neural resources of rhythm and syntax: An ALE Meta-Analysis. BioRxiv, 137, 822676. https://doi.org/10.1101/822676
- Jackendoff, R. (2009). Parallels and nonparallels between language and music. Music Perception, 26(3), 195–204. https://doi.org/10.1525/mp.2009.26.3.195
- Jones, M. R., & Boltz, M. (1989). Dynamic attending and responses to time. Psychological Review, 96(3), 459–491. https://doi.org/10.1037/0033-295X.96.3.459
- Kaufeld, G., Bosker, H. R., Alday, P., Meyer, A., & Martin, A. (2020). Linguistic structure and meaning organize neural oscillations into a content-specific hierarchy. Journal of Neuroscience, 40(49), 9467–9475. https://doi.org/10.1101/2020.02.05.935676
- Kotz, S. A., & Gunter, T. C. (2015). Can rhythmic auditory cuing remediate language-related deficits in Parkinson's disease? Annals of the New York Academy of Sciences, 1337(1), 62–68. https://doi.org/10.1111/nyas.12657
- Kotz, S. A., & Schmidt-Kassow, M. (2015). Basal ganglia contribution to rule expectancy and temporal predictability in speech. Cortex, 68, 48–60. https://doi.org/10.1016/j.cortex.2015.02.021
- Kotz, S. A., & Schwartze, M. (2010). Cortical speech processing unplugged: A timely subcortico-cortical framework. Trends in Cognitive Sciences, 14(9), 392–399. https://doi.org/10.1016/j.tics.2010.06.005
- Kotz, S. A., Schwartze, M., & Schmidt-Kassow, M. (2009). Non-motor basal ganglia functions: A review and proposal for a model of sensory predictability in auditory language perception. Cortex, 45(8), 982–990. https://doi.org/10.1016/j.cortex.2009.02.010
- Kotz, S. A., Stockert, A., & Schwartze, M. (2014). Cerebellum, temporal predictability and the updating of a mental model. Philosophical Transactions of the Royal Society B: Biological Sciences, 369(1658), 20130403, https://doi.org/10.1098/rstb.2013.0403
- Ladányi, E., Lukács, Á., & Gervain, J. (2021). Does rhythmic priming improve grammatical processing in Hungarian-speaking children with and without developmental language disorder? Developmental Science, 24(6), 1–12. https://doi.org/10.1111/desc.13112
- Ladányi, E., Persici, V., Fiveash, A., Tillmann, B., & Gordon, R. L. (2020). Is atypical rhythm a risk factor for developmental speech and language disorders? WIRES Cognitive Science, 11(5), 1–32. https://doi.org/10.1002/wcs.1528
- Large, E. W., Herrera, J. A., & Velasco, M. J. (2015). Neural networks for beat perception in musical rhythm. Frontiers in Systems Neuroscience, 9, 1–14. https://doi.org/10.3389/fnsys.2015.00159
- Large, E. W., & Jones, M. R. (1999). The dynamics of attending: How people track time-varying events. Psychological Review, 106(1), 119–159. https://doi.org/10.1037/0033-295X.106.1.119
- Law, L. N. C., & Zentner, M. (2012). Assessing musical abilities objectively: Construction and validation of the profile of music perception skills. PLoS One, 7(12), e52508. https://doi.org/10.1371/journal.pone.0052508
- Lee, Y. S., Ahn, S., Holt, R. F., & Schellenberg, E. G. (2020). Rhythm and syntax processing in school-age children. Developmental Psychology, 56(9), 1632–1641. https://doi.org/10.1037/dev0000969
- LimeSurvey Project Team / Schmitz, C. (2015). LimeSurvey: An Open Source survey tool (2.00). In limesurvey project, Hamburg, Germany. http://www.limesurvey.org
- Martins, M. J. D., Fischmeister, F. P. S., Gingras, B., Bianco, R., Puig-Waldmueller, E., Villringer, A., Fitch, W. T., & Beisteiner, R. (2020). Recursive music elucidates neural mechanisms supporting the generation and detection of melodic hierarchies. Brain Structure and Function, 225(7), 1997–2015. https://doi.org/10.1007/s00429-020-02105-7
- Martins, M. D., Gingras, B., Puig-Waldmueller, E., & Fitch, W. T. (2017). Cognitive representation of “musical fractals”: Processing hierarchy and recursion in the auditory domain. Cognition, 161, 31–45. https://doi.org/10.1016/j.cognition.2017.01.001
- Neurobehavioral Systems, Inc. (2021). Presentation® software (18.0). www.neurobs.com
- Osterhout, L., & Holcomb, P. J. (1992). Event-related brain potentials elicited by syntactic anomaly. Journal of Memory and Language, 31(6), 785–806. https://doi.org/10.1016/0749-596X(92)90039-Z
- Patel, A. D., & Iversen, J. R. (2014). The evolutionary neuroscience of musical beat perception: The Action Simulation for Auditory Prediction (ASAP) hypothesis. Frontiers in Systems Neuroscience, 8, 1–14. https://doi.org/10.3389/fnsys.2014.00057
- Poudrier, E. (2020). The influence of rate and accentuation on subjective rhythmization. Music Perception, 38(1), 27–45. https://doi.org/10.1525/mp.2020.38.1.27
- Przybylski, L., Bedoin, N., Krifi-Papoz, S., Herbillon, V., Roch, D., Léculier, L., Kotz, S. A., & Tillmann, B. (2013). Rhythmic auditory stimulation influences syntactic processing in children with developmental language disorders. Neuropsychology, 27(1), 121–131. https://doi.org/10.1037/a0031277
- R. C. Team. (2018). R: A language and environment for statistical computing. R Foundation for Statistical Computing.
- Roncaglia-Denissen, M. P., Schmidt-Kassow, M., Heine, A., & Kotz, S. A. (2015). On the impact of L2 speech rhythm on syntactic ambiguity resolution. Second Language Research, 31(2), 157–178. https://doi.org/10.1177/0267658314554497
- Roncaglia-Denissen, M. P., Schmidt-Kassow, M., & Kotz, S. A. (2013). Speech rhythm facilitates syntactic ambiguity resolution: ERP evidence. PLoS One. 8(2), e56000. https://doi.org/10.1371/journal.pone.0056000
- Rothermich, K., & Kotz, S. A. (2013). Predictions in speech comprehension: FMRI evidence on the meter-semantic interface. NeuroImage, 70, 89–100. https://doi.org/10.1016/j.neuroimage.2012.12.013
- Rothermich, K., Schmidt-Kassow, M., & Kotz, S. A. (2012). Rhythm’s gonna get you: Regular meter facilitates semantic sentence processing. Neuropsychologia, 50(2), 232–244. https://doi.org/10.1016/j.neuropsychologia.2011.10.025
- Schwartze, M., Farrugia, N., & Kotz, S. A. (2013). Dissociation of formal and temporal predictability in early auditory evoked potentials. Neuropsychologia, 51(2), 320–325. https://doi.org/10.1016/j.neuropsychologia.2012.09.037
- Schwartze, M., & Kotz, S. A. (2016). Contributions of cerebellar event-based temporal processing and preparatory function to speech perception. Brain and Language, 161, 28–32. https://doi.org/10.1016/j.bandl.2015.08.005
- Slater, J., & Kraus, N. (2016). The role of rhythm in perceiving speech in noise: a comparison of percussionists, vocalists and non-musicians. Cognitive Processing, 17(1), 79–87. https://doi.org/10.1007/s10339-015-0740-7
- Stanislaw, H., & Todorov, N. (1999). Calculation of signal detection theory measures. Behavior Research Methods, Instruments, & Computers, 31(1), 137–149. http://doi.org/10.3758/BF03207704
- Tal, I., Large, E. W., Rabinovitch, E., Wei, Y., Schroeder, C. E., Poeppel, D., & Golumbic, E. Z. (2017). Neural entrainment to the beat: The “missing-pulse” phenomenon. The Journal of Neuroscience, 37(26), 6331–6341. https://doi.org/10.1523/JNEUROSCI.2500-16.2017
- Tierney, A., & Kraus, N. (2014). Auditory-motor entrainment and phonological skills: Precise auditory timing hypothesis (PATH). Frontiers in Human Neuroscience, 8, 1–9. https://doi.org/10.3389/fnhum.2014.00949
- Villata, S., Franck, J., Villata, S., & Franck, J. (2020). Similarity-Based interference in agreement comprehension and production: Evidence from object agreement. Journal of Experimental Psychology: Learning, Memory, and Cognition, 46(1), 170–188. https://doi.org/10.1037/xlm0000718
- Villata, S., Tabor, W., & Franck, J. (2018). Encoding and retrieval interference in sentence comprehension: Evidence from agreement. Frontiers in Psychology, 9, 1–16. https://doi.org/10.3389/fpsyg.2018.00002
- Wagers, M. W., Lau, E. F., & Phillips, C. (2009). Agreement attraction in comprehension: Representations and processes. Journal of Memory and Language, 61(2), 206–237. https://doi.org/10.1016/j.jml.2009.04.002
- Woodruff Carr, K., White-Schwoch, T., Tierney, A. T., Strait, D. L., & Kraus, N. (2014). Beat synchronization predicts neural speech encoding and Reading readiness in preschoolers. Proceedings of the National Academy of Sciences, 111(40), 14559–14564. https://doi.org/10.1073/pnas.1406219111
- Yates, K. M., Moore, D. R., Amitay, S., & Barry, J. G. (2019). Sensitivity to melody, rhythm, and beat in supporting speech-in-noise perception in young adults. Ear & Hearing, 40(2), 358–367. https://doi.org/10.1097/AUD.0000000000000621
- Zentner, M., & Strauss, H. (2017). Assessing musical ability quickly and objectively: development and validation of the Short-PROMS and the Mini-PROMS. Annals of the New York Academy of Sciences, 1400(1), 33–45. https://doi.org/10.1111/nyas.13410
- Zink, C. F., Tong, Y., Chen, Q., Bassett, D. S., Stein, J. L., & Meyer-Lindenberg, A. (2008). Know your place: Neural processing of social hierarchy in humans. Neuron, 58(2), 273–283. https://doi.org/10.1016/j.neuron.2008.01.025