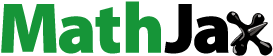
Abstract
Laboratory experiments were conducted to investigate the reduction of pollution load of paper mill effluent through phytoremediation technique using water caltrop (Trapa natans). Different concentrations, viz. 0% (control), 25, 50, 75, and 100% (absolute) were treated using T. natans for 60 days. Results showed that sewage effluent was loaded with various parameters, viz. total dissolved solids (TDS), electrical conductivity, total Kjeldhal nitrogen (TKN), phosphate (), calcium (Ca2+), magnesium (Mg2+), potassium (K+), cadmium (Cd), copper (Cu), iron (Fe), nickel (Ni), lead (Pb), and zinc (Zn). The maximum removal of different parameters was recorded after 60 days of phytoremediation experiments. Water caltrop (T. natans) significantly (p < 0.05/p < 0.01) removed TDS, BOD, COD, TKN,
, Ca2+, Mg2+ and K+, Cd, Cu, Fe, Ni, Pb, and Zn of the paper mill effluent after the phytoremediation. The removal of different pollutants from the paper mill effluent was gradually increased at lower concentration i.e. (from 20 to 50%) concentrations of the paper mill effluent. The maximum fresh weight, dry weight, and LAI of T. natans were observed with 50% concentration of the paper mill effluent at 45 days of phytoremediation experiments. Therefore, T. natans can be used for the treatment of paper mill effluent up to 50% concentration for 60 days using phytoremediation technique.
Public Interest Statement
Phytoremediation is emerging as a new technology for wastewater treatment in many developing countries. Phytoremediation is the use of plants to partially or substantially remediate selected contaminants in contaminated soil, sludge, sediment, ground water, surface water, and wastewater. It is cost-effective. The success of phytoremediation depends on the availability of plant species, ideally those native to the region. Plant species must be tested for their phytoremediation efficiency using different types of industrial effluent.
1. Introduction
Rapid urbanization and industrialization is placing an unprecedented pressure on water quality and demand. There are numerous other constraints such as inefficient infrastructure, weak, urban and municipal regulation, inadequate financial services which together bring deterioration in environment quality (Ali, Khan & Sajad, Citation2013; Kumar & Chopra, Citation2014; Padmapriya & Murugesan, Citation2012; Shah, Kumawat, Singh, & Wani, Citation2010). With the development of new industrial technologies and modernization of society raised the water demand in urban as well as rural areas. More requirement of water means more discharge of wastewater. This further enhances the pollution level in water bodies (Dar, Kumawat, Singh, & Wani, Citation2011; Shah et al., Citation2010; Singh, Tiwari, & Gupta, Citation2012).
Industrial pollution affects the quality of pedosphere, hydrosphere, atmosphere, lithosphere, and biosphere (Lone, He, Stoffella, & Yang, Citation2008; Kumar & Chopra, Citation2015; Tangahu et al., Citation2011. Toxic metal pollution of waters is a foremost environmental problem, and most conventional remediation approaches do not provide satisfactory solutions. The use of specifically selected and engineered metal-accumulating plants for environmental clean-up is an emerging technology called phytoremediation. Efforts have been made in the last two decades to reduce pollution sources and remedy the polluted soil and water resources (Alade & Ojoawo, Citation2009; Ali et al., Citation2013; Sooknah & Wilkie, Citation2004). Phytoremediation, being more cost-effective and having fewer side effects than physical and chemical approaches, has gained increasing popularity in both academic and practical circles. More than 400 plant species have been identified to have potential for soil and water remediation (Ali et al., Citation2013; Memon & Schröder, Citation2009; Mohammad et al., Citation2008).
Industrial untreated water is a major factor of water pollution (Ajibade, Adeniran, & Egbuna, Citation2013; Kumar & Chopra, Citation2014, 2015). Among different methods of wastewater treatment, phytoremediation is one of the most feasible methods for the treatment of wastewater (Pilon-Smits & Pilon, Citation2002; Letachowicz, Krawczyk, & Klink, Citation2006; Tangahu et al., Citation2011). A major environmental concern due to dispersal of industrial and urban wastes generated by human activities is the contamination of the environment (Ajibade et al., Citation2013; Chandra Sekhar, Kamala, Chary, Balaram, & Garcia, Citation2005; Liao & Chang, Citation2004). A wide range of inorganic and organic compounds cause contamination. Major component of inorganic contaminates are heavy metals (Allinson, Nishikawa, De Silva, Laurenson, & De Silva, Citation2002; Kulkarni, Ranade, & Wasif, Citation2008; Luqman et al., Citation2013).
Heavy metals are among the most important sorts of contaminant in the environment (Kumar & Chopra, Citation2014; Kutty, Ngatenah, Isa, & Malakahmad, Citation2009; Mahmood et al., Citation2005). Several methods have already been used to clean up the environment from these kinds of contaminants, but most of them are costly and difficult to get optimum results. Currently, phytoremediation is an effective and affordable technological solution used to extract or remove inactive metals and metal pollutants from contaminated soil and water (Kumar & Chopra, Citation2014; Meagher, Rugh, Kandasamy, Gragson, & Wang, Citation2000; Pehlivan, Özkan, Dinç, & Parlayici, Citation2009). The most common heavy metal contaminants are cadmium (Cd), chromium (Cr), copper (Cu), mercury (Hg), lead (Pb), and zinc (Zn) etc. Metal pollution has harmful effect on biological systems and does not undergo biodegradation. Toxic heavy metals such as Pb, Co, Cd can be differentiated from other pollutants, since they cannot be biodegraded but can be accumulated in living organisms, thus causing various diseases and disorders even in relatively low concentrations (Kumar & Chopra, Citation2014, 2015; Pehlivan et al., Citation2009; Tangahu et al., Citation2011).
Phytoremediation can be defined as the groups of technologies that use plants to remediation of soil, sludge, sediments, and water contaminated with organic and inorganic contaminants (Memon & Schröder, Citation2009; Rohit, Vinayak, & Avinash, Citation2015). In phytoremediation process plants remove, detoxify, or immobilize the contaminants of water. This technology has been receiving attention lately as an innovative, cost-effective alternative to the more established treatment methods used at hazardous waste sites (Baker, McGrath, Reeves, & Smith, Citation2000; Mahmood et al., Citation2005; Pehlivan et al., Citation2009). Phytoremediation utilizes a variety of plant biological processes and the physical characteristics of plants to aid in site remediation (Chaney, Li, Brown, Homer, & Malik, Citation2000; Mojiri, Citation2011; Palmer, Warwick, & Keller, Citation2001). Thus, phytoremediation has also been called green remediation, botano-remediation, agro-remediation, and vegetative remediation. Moreover, phytoremediation is a low-cost, solar energy-driven clean-up technique (Brooks, Robinson, Howes, & Chiarucci, Citation2001; Pollard, Powell, Harper, & Smith, Citation2002; Singh et al., Citation2012).
The success of phytoremediation or phytomining depends on the availability of plant species, ideally those native to the region of interestable to tolerate and accumulate high concentrations of heavy metals (Raskin & Ensley, Citation2000; Rohit et al., Citation2015; Shah et al., Citation2010). Some plants can accumulate remarkable levels of heavy metals. Many hyper accumulating species, characterized by their tolerance to toxic levels of metals such as arsenic (As), cobalt (Co), Cu, Zn, manganese (Mn), Pb, selenium (Se), nickel (Ni), and Cd, are often endemic to metal-rich substrates and are rare in their distribution (Ajibade et al., Citation2013; Mojiri, Citation2011; Singh et al., Citation2012). Various studies have been conducted on different types of industrial effluent using various plant species, but no significant scientific reports are cited on the phtoremediation of effluent using T. natans (Chaney et al., Citation2000; Rohit et al., Citation2015). Therefore, phytoremediation potential of different plant species using various types of waste effluent should be studied for the application of these species in biological treatments (Ali et al., Citation2013; Mojiri, Citation2011; Rohit et al., Citation2015). Keeping above in view, the present study was undertaken to study the reduction of pollution load of paper mill effluent by phytoremediation technique using water caltrop (Trapa natans L.).
2. Materials and methods
2.1. Experimental design
To study the reduction of pollution load of paper mill effluent by phytoremediation technique using water caltrop (T. natans), phytoremediation experiments were conducted in the Agro-ecology and Pollution Research Laboratory of the Department of Zoology and Environmental Science, Gurukula Kangri University, Haridwar (Uttarakhand), India. Borosilicate glass jars of capacity 10 liters were used for the phytoremediation experiments. Different concentrations of paper mill effluent, viz. 0% (Borewell water), 25% (25 ml paper mill effluent + 75 ml borewell water), 50% (50 ml paper mill effluent + 50 ml borewell water), 75% (75 ml paper mill effluent + 25 ml borewell water), and 100% (absolute effluent) were prepared and used for the phytoremediation treatment. Five-liter samples of paper mill effluent concentrations were filled in the glass jar separately and two healthy plants of known biomass of T. natans were transferred in the effluent and the experiments were conducted for 60 days. The experiments were performed with retention time at 24 h for 60 days for the maximum removal of pollutants. Five replicates of the experiment were maintained throughout the study period and placed in randomized complete block design.
2.2. Collection of effluent samples and analysis
Uttaranchal Pulp and Paper Mill, Roorkee, Haridwar (Uttarakhand), India (29°46′4″N 77°50′47″E) was selected for the collection of paper mill effluent samples. The pulp and paper mill is located about 10.0 km away from Roorkee, on Roorkee Disband Highway. The plants of water caltrop nut (T. natans) were collected from the local pond situated at Faizpur, Bijnor (Uttar Pradesh). The effluent samples were collected from the effluent disposal channel at pulp and paper mill in thoroughly cleaned plastic container. The collected effluent samples were brought to the laboratory and were analyzed before and after phytoremediation for various physico-chemical parameters and heavy metals, viz. total dissolved solids (TDS), pH, electrical conductivity (EC), total nitrogen (N), phosphorus (P), calcium (Ca), magnesium (Mg), potassium (K), iron (Fe), cadmium (Cd), chromium (Cr), nickel (Ni), lead (Pb), and zinc (Zn) using T. natans at 0, 15, 30, 45, and 60 days following standard techniques (American Public Health Association [APHA], Citation2012).
2.3. Determination of growth parameters and heavy metal analysis in T. natans
The growth parameters, viz. fresh, dry, and leaf area index (LAI) of T. natans before and after phytoremediation experiments at 0, 15, 30, 45, and 60 days were estimated following standard methods (Kumar & Chopra, Citation2014). For heavy metal analysis, 1.0 g of air-dried T. natans were taken in digestion tubes and 3 ml of concentrate HNO3 was added and digested in an electrically heated block for 1 h at 145°C. To this mixture, 4 ml of HClO4 was added and heated to 240°C for 1 h. The mixture was cooled and filtered through Whatman No. 42 filter paper. The volume was made to 50 ml by adding double-distilled water and used for analysis. Metals were analyzed using an atomic absorption spectrophotometer (PerkinElmer, Analyst 800 AAS, GenTech Scientific Inc., Arcade, NY) following methods (APHA, Citation2012).
2.4. Data interpretation and statistical analysis
Means were calculated with the help of MS Excel 2013. A one way analysis of variance (ANOVA) was used for data analysis to measure the variations among different parameters of the paper mill effluent before and after phytoremediation using T. natans at different days. Graphs were plotted with the help of Sigma plot, 2000.
3. Results and discussion
3.1. Characteristics of paper mill effluent
The physico-chemical characteristics of paper mill effluent are presented in Table . During the present investigation, the paper mill effluent was found to be alkaline (pH 7.96) and it is likely due to the applications of various alkalis to adjust the pH during effluent treatment. The most contents of TDS (980.00 mg/L), EC (1.44 dS/cm), BOD (1,295.00 mg/L), COD (1645.39 mg/L), TKN (142.38 mg/L), (64.95 mg/L), Ca2+ (306.45 mg/L), Mg2+ (92.45 mg/L), K+ (118.90 mg/L), Cd (4.12 mg/L), Cu (9.84 mg/L), Fe (12.65 mg/L), Ni (3.26 mg/L), Pb (4.07 mg/L) and Zn (11.19 mg/L) were recorded with 100% concentration of paper mill effluent before phytoremediation (Table ). The values of paper mill effluent parameters were recorded to be significantly (p < 0.05/p < 0.01/p < 0.001) different over the concentration in comparison to borewell water (control). The higher values of BOD and COD in the paper mill effluent are indicators of higher organic and inorganic pollution load (Kumar & Chopra, Citation2014). The higher values of EC of the paper mill effluent are likely associated with the presence of more cationic and anionic species in the paper mill effluent. The higher values of different heavy metals in the paper mill effluent might be due to the application of various dyes in the manufacturing of color papers. The higher contents of BOD and COD were in line with the results of Kumar and Chopra (Citation2015) who reported greater values of BOD and COD in the agro-based paper mill effluent. Luqman et al. (Citation2013) also reported the higher values of various heavy metals in the paper mill effluent.
Table 1. Characteristics of paper mill effluent and borewell water before phytoremediation experiment
3.2. Reduction of various pollutants after phytoremediation
Figures show the reduction of various parameters, viz. TDS, BOD, COD, TKN, , Ca2+, Mg2+, and K+ of paper mill effluent as per concentration after phytoremediation at different days, viz. 0 days (before phytoremediation), 15, 30, 45, and 60 days using T. natans. During the investigation, ANOVA analysis on data indicated that phtoremediation treatment of paper mill effluent using T. natans significantly (p < 0.05/p < 0.01) reduced the contents of TDS, BOD, COD, TKN,
, Ca2+, Mg2+, and K+ of paper mill effluent when compared to control experiments at different days (Table ; Figures ). Among different days of treatment, most reduction of TDS (140.00–770.00 mg/L), BOD (145–986 mg/L), COD (252.34–1402.88 mg/L), TKN (6.53–106.94 mg/L),
(3.45–35.94 mg/L), Ca2+ (31.89–252.79 mg/L), Mg2+ (4.04–52.88 mg/L), and K+ (6.34–67.33 mg/L) were recorded with 25–100% concentrations of the paper mill effluent after 60 days of the phytoremediation experiments using T. natans. It is interesting to note that the reduction of parameters was gradually increased with 15–45 days of phytoremediation experiments using T. natans. Thus, the maximum removal rate of different parameters was observed with 45 days of the phytoremediation experiments using T. natans. It might be due to that T. natans achieve its maximum vegetative growth due to uptake of different nutrients from the paper mill effluent. The findings are in line with Kutty et al. (Citation2009) who reported that Eichhorina crassipes was observed to be effective for the removal of different nutrients like N, P, K, Ca, Mg, Na, P from the municipal wastewater.
Figure 1. Change in TDS of paper mill effluent after phytoremediation using T. natans at different days.
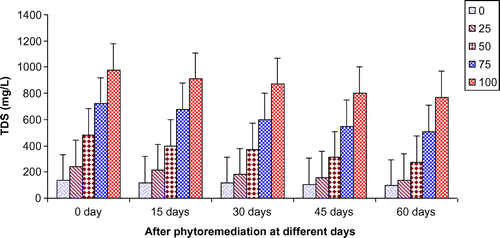
Figure 2. Change in BOD of paper mill effluent after phytoremediation using T. natans at different days.
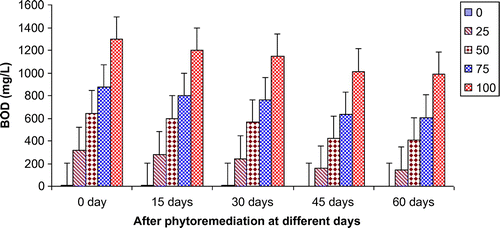
Figure 3. Change in COD of paper mill effluent after phytoremediation using T. natans at different days.
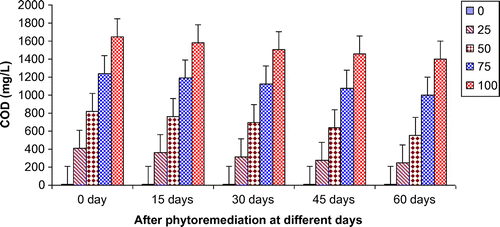
Figure 4. Change in TKN of paper mill effluent after phytoremediation using T. natans at different days.
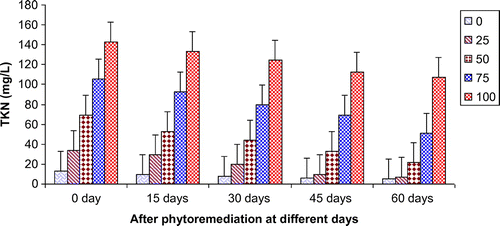
Figure 5. Change in PO43- of paper mill effluent after phytoremediation using T. natans at different days.
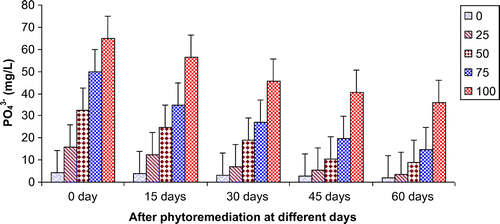
Figure 6. Change in Ca2+ of paper mill effluent after phytoremediation using T. natans at different days.
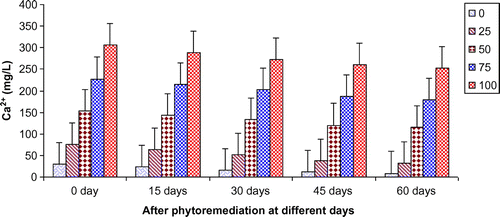
Figure 7. Change in Mg2+ of paper mill effluent after phytoremediation using T. natans at different days.
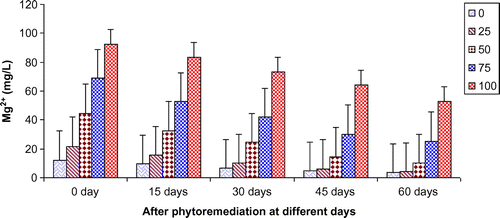
Figure 8. Change in K+ of paper mill effluent after phytoremediation using T. natans at different days.
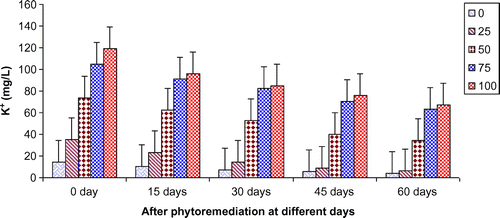
Table 2. ANOVA analysis for the phytoremediation of different parameters of paper mill effluent using T. natan
3.3. Reduction of heavy metals after phytoremediation
In the present study, ANOVA data indicated that phytoremediation of paper mill effluent using T. natans significantly (p < 0.05/p < 0.01) decreased the contents of Cd, Cu, Fe, Ni, Pb, and Zn after 60 days of the phytoremediation treatment (Table ; Figures ). The removal of heavy metals from 15 to 60 days were ranged Cd (0.67–3.65 mg/L), Cu (1.52–6.45 mg/L), Fe (1.88–8.75 mg/L), Ni (0.47–2.64 mg/L), Pb (0.54–3.66 mg/L) and Zn (1.84–7.62 mg/L) of 25–100% concentrations of the paper mill effluent after phytoremediation experiments using T. natans (Figures ). The reduction rate of heavy metals gradually increased from 25 to 50% concentrations of paper mill effluent with 15–45 days and it was slightly lower at 60 days and higher concentrations (i.e. 75 and 100%) of the paper mill effluent. It might be due the maximum tolerable uptake of Cd, Cu, Fe, Ni, Pb, and Zn by T. natans at 45 days and lower concentrations (i.e. 25 and 50%) of the paper mill effluent after phytoremediation experiments. Moreover, the diverse accumulation of Cd, Cu, Fe, Ni, Pb, and Zn were recorded during the phytoremediation treatments and it is likely due to the diverse chemical properties like oxido-reduction, ionization, stability, and sorption behavior of Cd, Cu, Fe, Ni, Pb, and Zn along with the optimum uptake efficiency and tolerance limit of T. natans used in the phytoremediation treatment. Thus, T. natans was observed to be effective for the removal of Cd, Cu, Fe, Ni, Pb, and Zn of the paper mill effluent concentrations from 25 to 50% using phytoremediation technique. Moreover, the gradual reduction of fresh, dry weight, and leaf area index (LAI) of T. natans at higher concentrations of paper mill effluent (75 and 100%) confirms the toxic effects of these heavy metals in T. natans. The findings are in agreement with (Ajibade et al., Citation2013) who reported that water hyacinth significantly decreased the contents of different heavy metals from the sewage effluent. Letachowicz et al. (Citation2006) also reported that Typha latifolia significantly reduced the contents of Cd, Cu, Ni, Pb, Mn, and Zn from the industrial effluent.
Figure 9. Change in Cd of paper mill effluent after phytoremediation using T. natans at different days.
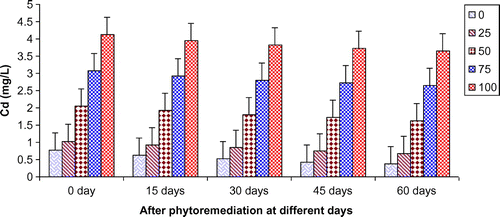
Figure 10. Change in Cu of paper mill effluent after phytoremediation using T. natans at different days.
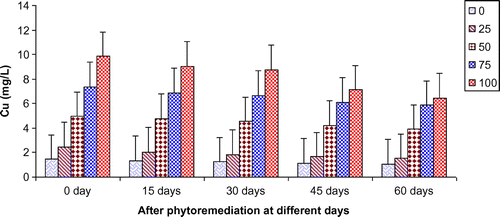
Figure 11. Change in Fe of paper mill effluent after phytoremediation using T. natans at different days.
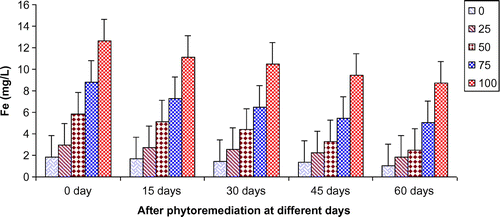
Figure 12. Change in Ni of paper mill effluent after phytoremediation using T. natans at different days.
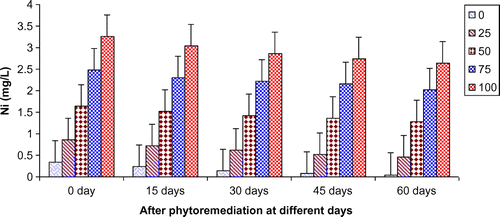
3.3. Changes in growth parameters of T. natans during phytoremediation
Table showed the changes in fresh, dry weight, and leaf area index (LAI) of T. natans at different days during phytoremediation experiments. During the present investigation, the maximum fresh weight (645.50 g), dry weight (98.78 g), and LAI (2.56) of T. natans were observed with 50% concentration of the paper mill effluent at 45 days of phytoremediation experiments. The ANOVA data indicated that fresh weight, dry weight, and LAI of T. natans were found to be significantly (p < 0.05) different after 30, 45, and 60 days of phytoremediation experiments (Table ). Moreover, the paper mill effluent concentration also showed significant (p < 0.05) effect on fresh weight, dry weight, and LAI of T. natans during the phytoremediation of paper mill effluent. The fresh weight, dry weight, and LAI of T. natans progressively increased from 25 to 50% and decreased from 75 to 100% concentrations of the paper mill effluent and it might be due the presence of more contents of heavy metals at higher concentrations (75 and 100%). The 50% concentrations support the fresh weight, dry weight, and LAI of T. natans and it might be due to the occurrence of optimum content of micro and macro nutrients to accomplish the maximum biomass during the phytoremediation experiments. Although, borewell water also contains various nutrients required by T. natans they are not sufficient as required by T. natans to achieve the maximum fresh weight, dry weight, and LAI in comparison to the different concentrations of the paper mill effluent. Moreover, the fresh weight, dry weight, and LAI of T. natans decreased at 100% concentration of the paper mill effluent in comparison to 50% concentration of paper mill effluent and it might be due to the excess contents of nutrients and heavy metals in the absolute (100%) concentration of the paper mill effluent. The findings are in the conformity with Dar et al. (Citation2011) who reported that the higher concentrations of sewage effluent significantly inhibited the vegetative growth of water hyacinth (Eichhornia crassipes) during the phytoremediation studies and it was due to the presence of higher content of heavy metals in the sewage effluent.
Table 3. Change in fresh, dry weight, and leaf area index (LAI) of T. natans at different days during phytoremediation experiments
4. Conclusions
The present study concluded that the paper mill effluent was loaded with various inorganic and organic pollutants. The phytoremediation experiment of paper mill effluent using T. natans was found effective for the reduction of various pollutants from the paper mill effluent. Among different days, the most reduction of TDS, BOD, COD, TKN, , Ca2+, Mg2+ and K+, Cd, Cu, Fe, Ni, Pb, and Zn were recorded at 60 days of the phytoremediation experiments using T. natans but the removal rate of the pollutants were accelerated from 15 to 45 days of the phytoremediation experiments. Moreover, the removal of pollutants after phytoremediation experiments using T. natans gradually increased from 25 to 50% concentrations of the paper mill effluent. The maximum fresh weight, dry weight, and LAI of T. natans were observed with 50% concentration of the paper mill effluent after 45 days of phytoremediation experiments. Therefore, up to 50% concentration of the paper mill effluent can be used for the phytoremediation experiments using T. natans for up to 45 days to achive the maximum removal rate of pollutants. Further studies should be required on the seasonal variation of the phytoremediation efficiency of water caltrop (T. natans) in view of climatic variable using paper mill effluent.
Additional information
Funding
Notes on contributors
Vinod Kumar
Vinod Kumar is working as an assistant professor of Environmental Science in the Department of Zoology and Environmental Science, Gurukula Kangri University, Haridwar (Uttarakhand), India. He has an academic experience of about 5 years and research experience of about 9 years. His interests of research area are Agro-ecology, Environmental Pollution Research, Wastewater Management and Bioremediation. He has published about 54 research papers in various national and international journals of repute. Vinod Kumar has credit with Google Citation: 160; h-index: 8 and i-10 index: 6. He is the founder and Editor of Journal of Applied and Natural Science. He is serving as editorial board member and reviewer of about 10 reputed international journals. Three students have completed their MSc dissertation and 2 students of PhD and 3 students of MSc are working their dissertation under his guidance on various aspects of Environmental Science.
References
- Ajibade, F. O., Adeniran, K. A., & Egbuna, C. K. (2013). Phytoremediation efficiencies of water hyacinth in removing heavy metals in domestic sewage (A Case Study of University of Ilorin, Nigeria). The International Journal of Engineering and Science, 2, 16–27.
- Alade, G. A., & Ojoawo, S. O. (2009). Purification of domestic sewage by water hyacinth (Eichhornia crassipes). International Journal of Environmental Technology and Management, 10, 286–294.10.1504/IJETM.2009.023735
- Ali, H., Khan, E., & Sajad, M. A. (2013). Phytoremediation of heavy metals—Concepts and applications. Chemosphere, 91, 869–881.10.1016/j.chemosphere.2013.01.075
- Allinson, G., Nishikawa, M., De Silva, S. S., Laurenson, L. J. B., & De Silva, K. (2002). Observations on metal concentrations in tilapia (oreochromis mossambicus) in reservoirs of south Sri Lanka. Ecotoxicology and Environmental Safety, 51, 197–202.10.1006/eesa.2001.2112
- Mojiri, A. (2011). Phytoremediation of heavy metals from municipal wastewater by Typhado mingensis. African Journal of Microbiology Research, 6, 643–647.
- American Public Health Association. (2012). Standard methods for the examination of water and waste water (21st ed.). Washington, DC: Author.
- Baker, A. J. M., McGrath, S. P., Reeves, R. D., & Smith, J. A. C. (2000). Metal hyperaccumlator plants: A review of the ecology and physiology of a biological resource for phytoremediation of metal polluted soils. In N. Terry, & G. Bañuelos (Eds.), Phytoremediation of Contaminated Soil and Water (pp. 85–108). Boca Raton, FL: Lewis.
- Brooks, R. R., Robinson, B. H., Howes, A. W., & Chiarucci, A. (2001). An evaluation of Berkheya coddii Roessler and Alyssum bertolonii Desv. for phytoremediation and phytomining of nickel. South African Journal of Science, 97, 558–560.
- Chandra Sekhar, K., Kamala, C. T., Chary, N. S., Balaram, V., & Garcia, G. (2005). Potential of Hemidesmus indicus for phytoextraction of lead from industrially contaminated soils. Chemosphere, 58, 507–514.10.1016/j.chemosphere.2004.09.022
- Chaney, R. L., Li, Y. M., Brown, S. L., Homer, F. A., & Malik, M. (2000). Improving metal hyper accumulator wild plants to commercial phytoextraction systems: Approaches and progress. In N. Terry, & G. Bañuelos (Eds.), Phytoremediation of contaminated soil and water (pp. 129–158). Boca Raton, FL: Lewis.
- Dar, S. H., Kumawat, D. M., Singh, N., & Wani, K. A. (2011). Sewage treatment potential of water hyacinth (Eichhornia crassipes). Research Journal of Environmental Sciences, 5, 377–385.10.3923/rjes.2011.377.385
- Kulkarni, B. V., Ranade, S. V., & Wasif, A. L. (2008). Phytoremediation of textile effluent by using water hyacinth: A polishing treatment. International Journal of Environment Management and Technology, 5, 18–27.
- Kumar, V., & Chopra, A. K. (2014). Ferti-irrigation effect of paper mill effluent on agronomical practices of phaseolus vulgaris (l.) in two seasons. Communications in Soil Science and Plant Analysis, 45, 2151–2170.10.1080/00103624.2014.929698
- Kumar, V., & Chopra, A. K. (2015). Fertigation with agro-residue-based paper mill effluent on a high-yield spinach variety. International Journal of Vegetable Science, 21, 69–97.10.1080/19315260.2013.825690
- Kutty, S. R. M., Ngatenah, S. N. I., Isa, M. H., & Malakahmad, A. (2009). Nutrients removal from municipal wastewater treatment plant effluent using Eichhorina crassipes. World Academic Science Engineering and Technology, 60, 826–831.
- Letachowicz, B., Krawczyk, J., & Klink, A. (2006). Accumulation of heavy metals in organs of Typha latifolia. Polish Journal of Environmental Studies, 15, 407–409.
- Liao, S. W., & Chang, W. L. (2004). Heavy metal phytoremediation by water hyacinth at constructed wetlands in Taiwan. Journal of Aquatic Plant Management, 42, 60–68.
- Lone, M. I., He, Z. L., Stoffella, P. J., & Yang, X. E. (2008). Phytoremediation of heavy metal polluted soils and water: Progresses and perspectives. Journal of Zhejiang University SCIENCE B, 9, 210–220.10.1631/jzus.B0710633
- Luqman, M., Butt, T., Tanvir, A., Atiq, M., Hussan, M. Z. Y., & Yaseen, M. (2013). Phytoremediation of polluted water by trees: A review. International Journal of Agricultural Research and Reviews, 1, 022–025.
- Mahmood, Q., Zheng, P., Islam, E., Hayat, Y., Hassan, M. J., Jilani, G., & Jin, R. C. (2005). Lab scale studies on water hyacinth (Eichhornia crassipes Marts Solms) for biotreatment of textile wastewater. Caspian Journal of Environmental Sciences, 3, 83–88.
- Meagher, R. B., Rugh, C. L., Kandasamy, M. K., Gragson, G., & Wang, N. J. (2000). Engineered phytoremediation of mercury pollution in soil and water using bacterial genes. In N. Terry, & G. Banuelos (Eds.), Phytoremediation of contaminated soil and water (pp. 201–221). Boca Raton, FL: Lewis.
- Memon, A. R., & Schröder, P. (2009). Implications of metal accumulation mechanisms to phytoremediation. Environmental Science and Pollution Research, 16, 162–175.10.1007/s11356-008-0079-z
- Padmapriya, G., & Murugesan, A. G. (2012). Phytoremediation of various heavy metals (Cu, Pb and Hg) from aqueous solution using water hyacinth and its toxicity on plants. International Journal of Environmental Biology, 2, 97–103.
- Palmer, E. F., Warwick, F., & Keller, W. (2001). Brassicaceae (cruciferae) family, plant biotechnology, and phytoremediation. International Journal of Phytoremediation, 3, 245–287.10.1080/15226510108500059
- Pehlivan, E., Özkan, A. M., Dinç, S., & Parlayici, Ş. (2009). Adsorption of Cu2+ and Pb2+ ion on dolomite powder. Journal of Hazardous Materials, 167, 1044–1049.10.1016/j.jhazmat.2009.01.096
- Pilon-Smits, E. A. H., & Pilon, M. (2002). Phytoremediation of metals using transgenic plants. Critical Reviews in Plant Sciences, 21, 439–456.10.1080/0735-260291044313
- Pollard, A. J., Powell, K. D., Harper, F. A., & Smith, J. A. C. (2002). The genetic basis of metal hyperaccumulation in plants. Critical Reviews in Plant Sciences, 21, 539–566.10.1080/0735-260291044359
- Raskin, I., & Ensley, B. D. (2000). Phytoremediation of toxic metals: Using plants to clean up the environment (pp. 53–70). New York, NY: John Wiley & Sons.
- Rohit, A. K., Vinayak H. L., & Avinash, B. (2015). Investigation of chromium phytoremediation and tolerance capacity of a weed, Portulaca oleracea L. in a hydroponic system. Water and Environment Journal, 10, 1111–12106.
- Shah, R. A., Kumawat, D. M., Singh, N., & Wani, K. A. (2010). Water hyacinth (Eichhornia crassipes) as a remediation tool for dye effluent pollution. International Journal Science and Nature, 1, 172–178.
- Singh, D., Tiwari, Archana, & Gupta, Richa (2012). Phytoremediation of lead from wastewater using aquatic plants. Journal of Agricultural Technology, 8, 1–11.
- Sooknah, R. D., & Wilkie, A. C. (2004). Nutrient removal by floating aquatic macrophytes cultured in anaerobically digested flushed dairy manure wastewater. Ecological Engineering, 22, 27–42.10.1016/j.ecoleng.2004.01.004
- Tangahu, B. V., Abdullah, S. R. S., Basri, H., Idris, M., Anuar, N., & Mukhlisin, M.. (2011). A review on heavy metals (As, Pb, and Hg) uptake by plants through phytoremediation. International Journal of Chemical Engineering, Article ID 939161. doi:10.1155/2011/939161