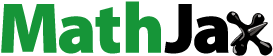
Abstract
The subject of physics can sometimes seem esoteric and challenging to engineering students. It would be beneficial to create a more conducive learning environment for them by combining words, mathematical equations, graphs, and diagrams to increase their problem-solving abilities. It has been demonstrated in mechanics that employing multiple representations is an effective strategy when solving problems. This study aimed to examine engineering students’ use of multiple representations to solve mechanics problems, as well as to identify the challenges they encountered when applying this approach. The study was carried out at a public university in Rwanda. A mixed-methods research design was employed with a study population of 140 engineering students, 20 of whom were selected to be interviewed. The quantitative data were collected through achievement tests and analyzed using SPSS, and the qualitative data were also collected through a guided interview and analyzed thematically. The current study revealed that engineering students prefer to use mathematical representations rather than diagrams and graphics representations when solving mechanics problems. Additionally, the study revealed that students encountered several difficulties when applying this approach, such as difficulties visualizing abstract concepts, a lack of familiarity with different representations, and difficulties drawing free-body diagrams and interpreting them. The study suggests that efforts should be made to boost engineering students’ use of various representations in solving mechanics problems through curriculum reforms, teaching-oriented interventions, and the provision of suitable technological resources.
SUMMARY OF THE RESEARCH PAPER
The current study at a selected public university in Rwanda looks at engineering students’ use of multiple representations (MRs) in mechanics problem-solving. This study examines the common difficulties students have comprehending and implementing MRs like diagrams, graphs, and equations. We hope to suggest practical solutions that improve students’ problem-solving abilities by analyzing their competency and identifying barriers. This study is consistent with larger initiatives to improve physics education methodologies, highlighting the significance of incorporating a variety of MRs. This study contributes valuable insights to the improvement of teaching and learning in mechanics in tertiary education, as well as informing educational initiatives aimed at enhancing students’ capacities in using multiple representations (MRs) to solve both physics and real-world problems.
Reviewing Editor:
1. Introduction
1.1. Background of the study
Physics has a negative reputation, and fewer students choose physics as a career choice (Euler, Citation2004). There are numerous causes for this, including: First and foremost, the physics subject is regarded as abstract, difficult, uninteresting, unappealing, unrelated to and disconnected from ordinary life (Martín-Blas et al., Citation2010). High school Physics is recognized as a challenging and hard course for students pursuing engineering as their career (Elmehdi et al., Citation2019). Sometimes engineering students feel compelled to study physics to broaden their understanding of fundamental scientific ideas and concepts, but the traditional way of studying does not appeal to them. As a response, we must foster a climate that encourages students to learn, analyze, solve problems, and be creative in explaining basic facts rather than memorizing them.
Problem-solving is one of the competencies that students must develop and highlight in the 21st-century learning paradigm because it is one of the key aims of learning physics. Students can shape their problem-solving abilities or attitudes by applying their experience and information in everyday circumstances. Students can apply their knowledge and problem-solving abilities after graduation and in the workplace (Yolenta et al., Citation2019). The key to problem-solving effectiveness is studying and comprehending several types of representations of physics concepts (Nurrahmawati et al., Citation2019). Problem-solving is also an essential element of engineering education. The deficit of problem-solving abilities in higher education is mostly due to a lack of preceding education training in developing problem-solving skills (Yolenta et al., Citation2019). Multiple representations have been proven to be an effective way of problem resolution in mechanics, a critical area of research in engineering. Multiple representations relate to the use of multiple modes of representation to express the same problem or notion, such as graphs, diagrams, equations, and words. This approach has been shown to improve students’ understanding of abstract concepts, facilitate the transfer of knowledge, and foster their abilities to solve problems. Despite the potential benefits of adopting numerous representations in mechanics problem solving, engineering students confront a number of problems when employing this method. These difficulties may include trouble envisioning abstract concepts, a lack of familiarity with various representations, and a lack of access to technology tools. The purpose of this study was to investigate engineering students’ usage of multiple representations when learning and solving mechanics problems.
1.2. Statement of research problem and motivation
The process of solving problems is an important part of the teaching and learning of physics since it helps students gain an understanding of the subject (Wicaksono and Korom, Citation2022). A critical feature of teaching and learning mechanics problem-solving by utilizing the various representation techniques is that students have both internal (mental) and exterior representations. To solve mechanics problems, representations consist of graphs, symbols, verbal statements, and diagrams, which form a model-based teaching and learning strategy. The capacity of students to understand each representation is essential for resolving each problem in a different representation. There are numerous types of representation in mechanics problems, namely word or verbal, pictorial, diagrammatic, symbolic, graphic, and numerical forms. Students with multi-representation abilities can portray themselves in a variety of ways (Saputra et al., Citation2019) . But the use of multiple representations in solving mechanics problems among engineering students remains unclear in Rwanda. Typically, engineering students memorize several formulas and plug values into formulas without understanding how to prove or illustrate them as it was revealed during problem identification. This causes students to struggle with issues such as understanding how to adapt techniques for problem-solving to new situations, not knowing or misusing the principle to be employed in solving a problem, misunderstanding the formula, misunderstandings between the quantities that describe the situation, not knowing the way to determine the value based on what has been given, lack of the abilities to determine the desired quantity depending on data gathered from a graph, and so on (Nguyen & Rebello, Citation2011). This is disheartening for students, particularly those studying engineering who did not intend to pursue it as a career. Many students grow disheartened and believe they will flunk physics. These students require cognitive tools as well as practice in problem-solving approaches. Multiple representations could help individuals deal with problems without feeling frustrated. This problem was identified by reviewing the literature and validating the potential of the problem using a pilot study. The examples of the problems used in piloting are attached in the Appendix A. Therefore, the present research aimed to evaluate engineering students’ use of multiple representations in mechanics problem-solving at a public university in Rwanda.
1.3. Objectives of the study
The current study was intended to evaluate engineering students’ use of multiple representations in mechanics problem-solving and their common challenges while using multiple representations at a public university in Rwanda. The specific objectives were:
To assess the engineering students’ use of multiple representations in mechanics problem-solving.
To identify the challenges that engineering students face while solving mechanics problems using multiple representations.
To suggest the possible solutions to the challenges in mechanics problem-solving for engineering students.
1.4. Research questions
This study was guided by the following research questions:
How do students in engineering solve mechanics problems using numerous representations?
What are the common challenges that engineering students face while solving mechanics problems using multiple representations?
What are the proposed solutions to overcome those challenges encountered when solving mechanics problems?
1.5. Significance of the research
The current study looked at how students in engineering use different representations when solving mechanics problems, as well as the challenges that they encountered when doing so. It created a research-based dataset from which physics for engineers’ teaching and learning can be developed to answer students’ challenges and requests for aid while handling problems in verbal, graphical, pictorial, and mathematical representations (Nguyen & Rebello, Citation2011). The primary goal of this research is to help engineering students enhance their problem-solving abilities in mechanics by using different representations. It revealed the challenges faced by students in engineering when they advance to multiple representation abilities, which is an essential ability that every engineer and scientist should have in the twenty-first century as they need it to translate problem-solving competencies into different applications in a modern technological society. According to a recent research study, there is no better combination of representations in mechanics problem-solving than another; it all relies on the type of problem to solve (Munfaridah et al., Citation2021). The present research may also assist students in engineering to successfully develop higher conceptual understanding as well as problem-solving abilities, particularly in shifting from one representation to the next in mechanics problem-solving without irritation. In general, the research findings will assist the university in improving engineering students’ problem-solving ability and conceptual grasp of mechanics. It also emphasizes the significance of learning experiences that allow students to transfer abilities in problem-solving across representations. It also advocates for additional investigation into similar challenges in different problem contexts and domains.
2. Literature review
Physics courses are often taken by engineering students during their secondary school education and their first year at a university or college (Tms & Sirait, Citation2016). This is primarily due to the importance of physics in understanding the underlying workings of the universe. As a result, it is critical to grasp that our environment is inextricably linked to physical laws and principles that regulate every aspect of our daily lives (Boyuk, Citation2011). In the first semester of engineering, physics courses not only familiarize students with fundamental physics principles but also encourage the development of unique problem-solving skills and abilities in representation (Bego et al., Citation2019). Physics plays an unquestionable importance in university scientific instruction. Nonetheless, teaching and studying physics remain difficult tasks. This problem can be minimized by using multiple representations, which combine diverse forms of representation to express abstract concepts in a more tangible manner, hence improving comprehensibility for a broad audience (Munfaridah et al., Citation2021).
Several researches, like Nguyen and Rebello (Citation2011) and Distrik et al. (Citation2021), have found that various representations play an important role in improving students’ problem-solving skills in physics education. Problem-solving abilities are among the most important talents that students must develop when studying physics. A student must excel in both quantitative and qualitative skills to effectively approach difficulties. Students must be able to represent a problem in several ways. This ability is primary evidence of their thorough mastery of the subject topic. Yolenta et al. (Citation2019) also discovered that students’ problem-solving abilities were limited, with only 15.26% presenting a solid grasp of problem understanding, 2.76% in problem-solving planning, 1% in problem-solving execution, and 4.6% in the re-checking phase. Using several types of representation is beneficial when solving physics problems, comprehending circumstances, expressing links between variables, and explaining mathematical relationships. According to Sambell (Citation2010), problem-solving, analytical thinking, innovation, creativity, teamwork, and communication are all essential components of 21st-century competencies. Problem-solving approaches are frequently utilized to enhance these abilities, especially when it comes to improving problem-solving abilities. Per Nurrahmawati et al. (Citation2019) research, there has been a huge increase in the concept of adapting different types of representations in the field of physics education in recent decades. The ability of a student to comprehend and explore different ways of describing a given physics concept is based on their ability to solve physics problems.
The employment of different types of representation is vital in aiding students’ comprehension of physics concepts and is important to the learning experience in the field of physics. Formulas, diagrams, graphs, textual descriptions, and/or symbols must always be used to acquire an in-depth understanding. Several research studies have shown that multiple representations improve students’ knowledge of physics concepts (Akçay et al., Citation2020; Brenner et al., Citation1997). Many methods of representation, such as mathematical representations, diagrams, graphs, tables, descriptive language, visual examples, symbols, and equation manipulation, can help students lessen the difficulties they have when learning physics (Munfaridah et al., Citation2021; Orulebaja et al., Citation2021). This technique also aids in the development and manipulation of many types of representation. Therefore, multiple representations are beneficial for improving conceptual understanding in the context of physics education because of their significant emphasis on absorbing concepts and engaging in qualitative reasoning during the learning process (Dufresne et al., Citation1997). Presenting information using different representations can be beneficial, aiding in solving problems and reducing the stress on memory.
Using several representations in mechanics problem-solving allows engineering students to have a deeper conceptual understanding. A well-known representation can be used to interpret or comprehend a more abstract one. Graphs, for example, can help clarify the meaning of an equation. When students are taught to utilize numerous representations at the same time, they gain a more comprehensive understanding of the idea. For example, when learning Newton’s law, students create visual sketches, motion diagrams, force diagrams, graphs, and mathematical equations. These various representations inspire students to delve deeper into the idea, resulting in a more complete grasp (Tms & Sirait, Citation2016). The following is an example of a mechanics problem solved by employing numerous representations: The example is centered on Newton’s laws of motion and force equilibrium.
Problem
Verbal representation
The two boxes (box A of mass 5.0kg and box B of mass 2.0kg) are connected by a string that passes over a pulley. The kinetic friction coefficient of Box A's interaction with the table is 0.20. We are not taking into account the mass of the rope and pulley, as well as any friction within the pulley. This permits us to presume that the force applied to one end of the cord is equal to the force applied to the other end. Our goal is to calculate the overall system acceleration, represented as 'a’, which will be consistent in magnitude for both boxes as long as the cord does not stretch. Box A remains motionless in the vertical direction, indicating that the normal force properly counteracts the gravitational force (weight) according to Newton’s second law. This box experiences two forces in the horizontal plane
which represent the tension in the cord (the exact magnitude of which is unknown), and friction force. For box B, the force of gravity pulls down, and the cord pulls up with a force
As a result, box A accelerates to the right, and box B accelerates down.
Pictorial representation
The diagram below shows two boxes connected by a string that passes over a pulley.
Numerical representation
Solution:
For box A,
We want to get horizontal acceleration, and thus and
For box B,
[Notice that if the
is not equal to
]
Solving for and
and by substituting this into the equation of box B:
Now we solve for and put in numerical values:
which is the acceleration of box A to the right, and box B down. If we wish, we can calculate
using the first equation:
NOTE: Box B is not in freefall. It does not fall at because another force,
acts upwards on it (Giancoli, Citation2005).
To solve the problem, students must first create a visual diagram (see ) and then a vector diagram (see ). Because of its practicality, a vector diagram plays an important part in solving physics problems. The free-body diagram is a type of vector diagram that simplifies the depiction of an object by displaying it as a single point at its origin. In the provided problem scenario, the vector diagram is a free-body diagram. A vector encompasses both direction and magnitude, whether illustrated with an arrow or expressed as a set of x and y components. Working with a vector diagram makes it easier to deconstruct its components for mathematical representation, as seen in the preceding instances. Drawing the vector diagram aids students in determining which mathematical functions to apply to the problems. The route to a solution becomes clearer when using different representations in solving problems (Bego et al., Citation2019).
2.1. Difficulties with multiple representations in mechanics problem-solving
Dong-Hai and Rebello’s (2011) study revealed that students had a variety of difficulties when solving mechanics problems using different representations. Their difficulties originated from an inability to relate mathematical principles to physics in a variety of contexts, notably in graphical and mathematical representations. The research also noticed that students occasionally lacked knowledge of or improperly applied the applicable principle, were unfamiliar with the formula and how to substitute values into it, and struggled to comprehend, analyze, and extract data from graphs to compute the needed quantity. In general, students find difficulties when using graphical representations (Abdurrahman et al., Citation2019). According to current research findings, when students are provided with hints, they are capable of solving problems utilizing alternate representations rather than merely mathematical representations (Nguyen & Rebello, Citation2011).
In the field of engineering, newcomers revealed that their primary usage of textbooks while solving problem-solving was to look for sample problems with comparable solution approaches to the one they were presently working on. As a result, their prospects for developing mental frameworks known as schemas, which allow individuals to recognize a problem as belonging to a category of problems treated using a common set of rules, are limited. Expert problem solvers, on the other hand, excel at both acquiring schemas and effectively applying them to solving problems (Johnson-Glauch & Herman, Citation2019). In their study, Lucas and Lewis (Citation2019) also discovered a prevalent problem among engineering students when solving mechanics problems was the lack of a free-body diagram. This absence resulted in a slew of analytical errors (Lucas & Lewis, Citation2019). Incorporating free-body diagrams into problem descriptions may theoretically contribute to both an augmentation and a decrease in the problems associated with problem-solving. Mešić et al. (Citation2017) argue that personally crafted representations, rather than pre-made ones, are more accessible to students due to their conformity to their inner perceptions. Munfaridah et al. (Citation2021) study, which focused on engineering, physics, and pre-service teacher programs other than mechanics, revealed that students struggled with understanding, creating, and transitioning between various representations in electromagnetism. To address these issues, they developed the Vector Field Representations test (VFR) to assess students’ difficulties and collected data through individual semi-structured interviews with students from four universities in three countries. The data analysis revealed that the issues with each representation, such as field vectors, field lines, and symbols, were caused by two critical factors: magnitude and direction. They revealed that many students struggled with (a) adding vectors, (b) failing to recognize the density of field lines as an indicator of field size, and (c) mixing field line features with equipotential lines (Munfaridah et al., Citation2021). Based on the above studies, the use of multiple representations is regarded as a successful teaching strategy in mechanics, but students face several challenges when implementing it in their educational process, particularly when switching between different types of representation. This highlights the significance of first investigating students’ concerns before developing assistance systems to address specific obstacles associated with multiple representations.
According to many experts, students have difficulty translating across presentations due to changes in the forms of representations and the way they process information (Ainsworth & Loizou, Citation2003). Prior research on solving problems using different forms of representation has revealed that translation between representations is typically the most difficult component of mechanics’ teaching-learning process (Ainsworth & VanLabeke, Citation2004). In this regard, students have challenges distinguishing conceptually relevant information from static pictorial representations. Nonetheless, students frequently encounter challenges when transferring between different types of representation, and these difficulties can be exacerbated when switching between representations of different types. As a result, the purpose of this research is to assess the use of multiple representations
2.2. Theoretical framework
Research suggests that students perform better when they interact with appropriate representations, and solving problems with multiple representations is preferable (Ainsworth, Citation2006). This study uses the Design, Functions, and Tasks (DeFT) framework to explore the use of multiple representations in mechanics problem-solving. DeFT considers design parameters, instructional roles, and cognitive processes. Understanding the role of Multiple Representations (MRs) is crucial for understanding their influence on the learning process. Tytler et al. (Citation2013) and Opfermann et al. (Citation2017) studies explore the benefits of using appropriate representations and the cognitive challenges associated with learning from a single representation. Ainsworth (Citation2006) outlined the conditions for effective use of Multiple Representations (MRs) in educational settings, emphasizing the benefits of acquiring complex or novel scientific knowledge. Current research focuses on learning with MRs within the DeFT (Design, Functions, and Tasks) framework, which involves the simultaneous use of diagrams, formulas, and data tables (Becker et al., Citation2020). MRs can complement each other by providing supplementary information or enabling alternate approaches to information processing. They can also aid in selecting the best representation for specific learning activities in specific educational situations (Abdurrahman et al., Citation2019). This framework shown also that the representations in learning involve how familiar representations influence each other’s perception and how intrinsic properties of one representation can prompt the use of another. Better comprehension is fostered when learners mix knowledge from different forms of representations. MRs can enhance the learning process, but their use depends on student requirements and can increase cognitive demands. Cognitive flexibility theory suggests that constructing and transitioning between diverse viewpoints is essential for effective learning. Malone et al. (Citation2020) study discovered that when mathematics activities were augmented with visual representations, learning outcomes improved while cognitive effort decreased. Failure to make links between representations can seriously hamper learning. Ainsworth and Loizou (Citation2003) also compared students’ capacity to learn estimating using mathematical, pictorial, or a combination of both representations and found that using a hybrid system with both representations hampered task learning due to a lack of interrelationship between the representations. Visual representation encouraged a perceptual approach, while mathematical representation encouraged rule formulation through symbol manipulation. Understanding the role of MRs in problem-solving and their potential benefits is crucial for effective learning. In this study, the theory played an important role when engineering students at the chosen university were guided with it when using multiple representations in solving mechanics problems and revealing the challenges accounted for while using this problem-solving approach.
3. Research methodology
3.1. Research approach
This research looked at engineering students’ use of multiple representations in mechanics problem solving, a concept that defies single-method measurement and comprehension (Creswell & Clark, Citation2011). This research employed a mixed-methods research approach that integrated quantitative and qualitative methods. The quantitative method was used to investigate the students’ use of various representations when solving mechanics problems, while the qualitative method used interviews with a sample of 20 students to refine the quantitative results. The research technique includes gathering both quantitative and qualitative data on students’ use of various representations, as well as the obstacles they faced and recommended solutions to them. The mixed-methods approach was intended to provide a thorough grasp of the study problem.
3.2. Research design
The current research adopted a mixed methods explanatory sequential design research approach to investigate engineering students’ use of multiple representations and their difficulties when solving mechanics problems (Kroll & Neri, Citation2009). In this design, quantitative data were first collected and analyzed, then qualitative data were collected, analyzed, and used to explain the quantitative results (Creswell & Plano Clark, Citation2011). The study approached the problem under study using both quantitative and qualitative data. Mechanics achievement tests were used to collect quantitative data, which was analyzed using descriptive and inferential statistics. Students’ interviews were used to collect qualitative data on the use of numerous representations in the mechanics of problem-solving and related difficulties
3.3. Sample and sampling methods
Purposive sampling was used in the study, which involves the deliberate selection of individuals capable of providing the required data (Hambali et al., Citation2017). As recommended by Creswell (Citation2012), this method was chosen for its time efficiency and ability to deliver insights from a specific demographic, ensuring a deep grasp of the research subject. The study included 140 first-year students in engineering studying in the "Physics for Engineers I" course at the selected public university in Rwanda. The sample included 66 students studying electronics and telecommunications engineering and 74 students studying electrical power engineering and were chosen based on their common physics background in high school. The study entailed collecting problem-solving tests from all students and interviews with a group of 20 students which was selected based on their performance so that 10 best-performing students and 10 poor-performing students were selected from both groups.
3.4. Instruments
3.4.1. Achievement test
This achievement test was constructed by the researcher byreviewing the literature (e.g. Lucas & Lewis, Citation2019; Orulebaja et al., Citation2021; Siswanto et al., Citation2018) and some recommended textbooks and past examination papers used at the university. The first draft of the instrument consisting of 25 questions was shared with experts in physics education who advised on the relevance, accuracy, and coverage of the test items. After correcting and removing redundant questions, the final achievement test comprised ten mechanics questions. See the Appendix. It was pilot-tested with 22 students and the overall Cronbach’s alpha reliability was found to be 0.807, indicating acceptable reliability. describes the categories of problem-solving representations used by engineering students when solving mechanics problems in current research.
Table 1. Categorization of questions.
3.4.2. Interview guide
The interview guide was composed of five questions based on two practical problems. The five questions were developed by the first author and verified by experts for conformity, following the steps used for the achievement test. The interview guide was used to collect qualitative data regarding students’ perceptions on the mechanics problem-solving, challenges, and their proposed solutions. The five interview questions are attached in an Appendix B.
3.5. Data collection methods
In the current study, engineering students were evaluated for their use of multiple representations in mechanics problem-solving, their difficulties encountered during these tasks, and their potential solutions. For data collection, the following tools were used: An achievement test was used to collect quantitative data on engineering students’ use of different representations in solving mechanics problems. The achievement test was first given to two distinct groups: students majoring in Electronics and Telecommunication Engineering and students majoring in Electrical Power Engineering, both of whom were tasked with solving mechanics problems using multiple representations. Interviews were conducted following the analysis of the quantitative data produced by the achievement tests. Participants were tasked with articulating their thought processes while resolving problems on written assessments. The interviewer offered verbal cues when students encountered difficulties or deviated from the correct approach. Each interview session was audio and video-recorded to get an accurate record of the participants’ responses. The average duration of the interviews was 45 minutes. Furthermore, the participants’ written responses were gathered for further analysis. The purpose of these interviews was to learn what students do to deal with mechanics problems, what problems they run into, and what solutions they recommend.
3.6. Data analysis methods
The quantitative data collected from the achievement tests, which assessed how these engineering students used numerous representations to solve mechanics problems, was analyzed and structured using descriptive statistics and inferential statistics using SPSS. In addition, to incorporate the students’ interpretations of the achievement test results, a qualitative follow-up approach was used. The comments from the interviews represented their viewpoints on the quantitative findings. Firstly, the interviews were transcribed verbatim for further analysis. The unstructured responses to open-ended questions in the textual data were classified and thematically examined. Five steps adopted by Mapulanga et al. (Citation2023) were followed to analyze the transcripts: (i) reading and re-reading the interview transcripts (ii) coding selected extracts of transcripts (iii) formulating appropriate themes and collecting data for each theme (iv) comparing the themes with the coded extracts (v) refining and defining the specifics of the formulated themes (iv) selecting appropriate examples of extracts for reporting the results. The initial analysis was performed by the first author and an independent researcher, while the second and third authors reviewed the first analysis and proposed adjustments where necessary. Then the authors reviewed the proposals and the process was repeated until agreement was attained.
3.7. Trustworthiness
The study’s trustworthiness was assessed following a variety of criteria, including credibility, confirmability, dependability, and transferability. To ensure credibility, the authors spent a significant amount of time with the data. Furthermore, the second and third authors reviewed the first data analysis on a regular basis. The data collection and analysis processes were described in depth to ensure the study’s dependability. To ensure confirmability, the study used DeFT as a theoretical framework to guide the design of the study and data analysis techniques. In addition, qualitative data were provided verbatim, and data collection and analytical techniques were thoroughly detailed.
3.8. Ethical considerations
Prioritizing research ethics is crucial in any study (Lodico et al., Citation2010). This study obtained formal authorization from the relevant authorities, including the University of Rwanda College of Education Ethical Committee, the University of Rwanda College of Science and Technology’s principal, the Dean of the School of Engineering, and consent from physics lecturers and students. The study was conducted in one college of a selected public university in Rwanda, where the chosen sample was drawn. All collected data was treated with strict confidentiality and used solely for the study’s purposes. The study ensures the integrity of the research process.
4. Results and interpretations
The findings of the study are presented in this section. The findings are presented according to the research questions.
4.1. Research question 1: How do students in engineering solve mechanics problems using numerous representations?
Results of students’ performance are shown in . The results show that engineering students perform well in graphical interpretation (M = 94.29, SD = 23.29) and perform poorly in mathematical calculations from verbal representations (M = 13.93, SD = 10.92). This shows that engineering students have expertise in analyzing mechanics graphs, which is consistent with the findings of Saputra et al. (Citation2019), who discovered that students excel at graph interpretation as compared to doing calculations from graphs.
Table 2. Descriptive statistics of the engineering students’ performance.
shows engineering students’ overall performance in mechanics problem-solving. The results show that the overall performance of engineering students was below 50% (M = 37.2 and SD = 16.7). The overall performance is unsatisfactory, highlighting the need for intervention to train these students in the utilization of multiple representations in mechanics problem-solving.
Table 3. Overall performance of engineering students in mechanics problems solving.
represents the one-way ANOVA results of engineering students’ mechanics problem-solving. The one-way ANOVA results show- a significant difference across all categories (p < 0.001), showing that the engineering students in this study had difficulties using numerous representations.
Table 4. One-way ANOVA engineering students’ performance results.
represents the cross-comparison between the representations to evaluate their efficiency in solving mechanics problems. The results show that there was a significant difference between each category and the other remaining categories (p ≤ 0.004). The insignificant difference was only observed between Category 2 and Category 4 (p = 1.000). This shows that each representation is independent of one another.
Table 5. Post hoc multiple comparisons engineering students’ performance results.
To acquire a better understanding of the difficulties in solving mechanics problems using multiple representations, interviews were performed to assess the difficulties engineering students had while solving mechanics problems utilizing different representations. The evaluation showed a variety of difficulties, including those related to the physics context, mathematical calculations, and the construction of free-body diagrams.
4.2. Research question 2: What are the common challenges engineering students face while solving mechanics problems using multiple representations?
Specific kinds of students’ challenges were identified through guided interviews with students, as shown in , which includes associated themes and their frequencies. Apart from the students’ use of various representations, this study found that engineering students confronted a variety of challenges while using multiple representations in mechanics problems. When these students moved on to the graphical problem, they recognized the method for calculating the area under the graph, as seen in the following excerpt:
Table 6. Summary of students’ difficulties in solving mechanics problems from interview.
Student: “This problem also has friction, but it tells you the friction through an equation graph.”
Researcher: “Okay, so how would you find work in this problem?”
Student: “It would be in the area.”
Researcher: “Which area?”
Student: “The area under the curve.”
This reduced the difficulties experienced by students while solving graphical problems, resulting in a decrease in the overall average number of difficulties faced.
The body was moving on an inclined plane under gravitational force and asked to find the friction force on that body. It was not easy for many of the students because they did not really understand the concept of an inclined plane. Some students were unsure what to do with such a problem, so they inquired of the interviewer about the direction of the body on an inclined plane. Another difficulty came from knowing the forces acting on a body and their directions and also the relationship between those forces. This is demonstrated in the following example:
Student: “I am not sure what to do with this problem… Is this body moving up or down?”
Researcher: “It is moving down.”
Student: “So what are the forces acting on that body in its motion?”
Researcher: “Gravitational force and frictional force?”
Student: “Ooooh… I guess.”
Researcher: “You need to find the frictional force acting on that body when it is moving down.” What is the relationship between the two forces?”
Student: “mgcosθ”.
Researcher: “Where is that angle?”
Student: “It is the angle of an inclined plane.”
4.2.1. Frequency of identified difficulties
According to the data in , a substantial percentage of students reported difficulties constructing free-body diagrams and recognizing all of the forces acting on an object, with 66% indicating difficulties in this context. Following that, 28% of engineering students reported difficulties understanding the problems given, while 20% had difficulties with mathematical manipulations. Furthermore, just 8% of engineering students had problems with graphical representations. This data shows that students struggle most with the creation and comprehension of free-body diagrams.
4.2.2. Research question 3: What are the proposed solutions to overcome those challenges encountered when solving mechanics problems?
shows the proposed potential solutions by engineering students interviewed in this research, such as providing free-body diagrams for each question proposed at 34%, training students in the use of various representations from lower schools proposed at 28%, particularly in the context of free-body diagrams, and conducting numerous practice exercises to improve their proficiency in applying multiple representations to solve mechanics problems proposed at 60%.
Table 7. Summary of the proposed solutions to students’ difficulties in solving mechanics problems from the interview.
5. Discussions of the results
The current research paper’s principal purpose is to evaluate the use of various methods of representation and the difficulties that engineering students encounter when tackling mechanics problems. Engineering students, according to the research, rely primarily on mathematical representations while solving mechanics problems, resulting in low performance in their first year of university physics for engineers. However, the study discovered that their academic performance could be improved when engineering students solve mechanics problems using diagrammatic and graphical representations rather than mathematical ones. These findings support prior research by emphasizing the need to use free-body diagrams or graphs to solve mechanics problems to increase the accuracy of numerical calculations (Davis & Lorimer, Citation2018; Id et al., Pratiwi et al., Citation2021; Rosengrant et al., Citation2007, Citation2009; Wong et al., Citation2011). According to the findings of this study, when solving mechanics problems, engineering students typically prefer mathematical representations over other types of representations. This decision is the result of most students’ incapacity to comprehend the significance of connecting various types of representation. In contrast, research findings demonstrate that students find it easier to answer problems provided by symbolic representations. Furthermore, students prefer symbolic representations while having difficulty with verbal representations, pictures, and tables. This shows that while solving problems, students usually rely on a single-representation approach. As a result, there is a need to improve students’ ability to solve problems using multiple representations. As a result, a study of students’ abilities to apply numerous representations and the factors determining this competency is critical. This study can be used to design practical learning approaches that improve students’ capacity to employ different representations effectively.
All students had difficulties in drawing free-body diagram and doing calculations from them. A large number of students had no trouble determining the area under the graph but had a lot of challenges creating the free-body diagram. These difficulties were not due to the difficulty of the problem but to the inability of engineering students to represent mechanics problems in pictorial representations. This promoted the poor performance of engineering students in mechanics problem solving. This was in line with the research done by Nguyen and Rebello (Citation2011) which found that when students try to apply their problem-solving skills across different representations, they face significant challenges. When studying problem sequences presented in various ways, it appears that no single sequence outperforms the others. Instead, it appears that the success of a sequence is dependent on the precise context of the problem to be solved. This is consistent also with the findings of another research published by Yolenta et al. (Citation2019) which found that engineering students faced difficulties in a variety of areas, including identifying relevant information within problems, transforming problems into different formats, expressing critical problem-related details, identifying applicable concepts, basic principles, laws, or physical math formulas for solving problems, replacing numerical data into formulas, carrying out mathematical computations to find unknown parameters, and solving problems with clarity, logical coherence, and maintaining consistent usage of appropriate quantities and units. Among these challenges are concerns with mathematical and pictorial representations. These difficulties result from students’ inability to apply their mathematics knowledge and skills to real-world physics circumstances (Nguyen & Rebello, Citation2011). In reality, there have been few studies that have particularly looked into the particular difficulties that engineering students may encounter while dealing with different sorts of representations and shifting from one representation to another. Nonetheless, research has shown that using a free-body diagram is a critical step in resolving mechanics-related problems. When students can construct a free-body diagram correctly, their chances of correctly solving mechanics problems increase dramatically compared to when the representation is inaccurate or when a free-body diagram is not used in the problem-solving process (Rosengrant et al., Citation2006). This emphasizes the critical role of the free-body diagram technique in understanding and mastering the domain of mechanics. The use of graphical illustrations can also be used when attempting mechanics problem-solving. Graphs are visual representations of physical phenomena on paper in the form of diagrams or sketches, allowing for a more concrete interpretation and comprehension, particularly when dealing with two or three-dimensional problems. The use of visuals not only aids in the achievement of true results but also improves the mental processes involved. It facilitates a two-way link by streamlining the transition between the physical scenario and its numerical representation (Kraige & Meriam, Citation2012). Ultimately, improving proficiency in mechanics problem-solving involves intervention in the form of training engineering students to apply numerous representations proficiently. This can be accomplished with plenty of practice tasks and the incorporation of technology into the teaching-learning process.
6. Conclusions and recommendations
The study emphasizes the significance of adopting numerous representations for solving mechanics problems, particularly for engineering students. Free-body diagrams or graphs were found to significantly enhance problem-solving abilities. Students, on the other hand, frequently struggle with diagrams and functional representations as they try to apply mathematical principles to physics settings. According to interviews, many students can answer problems correctly and acquire procedures in representations other than numerical representations, which they may not have had much opportunity to study in their physics class. Different problem sequences in different representations may provide different results, but students appear to gain their capacity to transfer from one representations to another. The research recommended the University curriculum revisions to include multiple representations utilization in physics and mechanics courses. This could include creating modules that require students to deal with diverse representation formats to improve their understanding of the subject. Faculty members should investigate novel methods of teaching, such as technology-enhanced learning materials and user-friendly simulations, to stimulate active interaction with different representations. Assessment approaches should also contain questions that require students to solve problems using numerous representations. This strategy can improve engineering students’ abilities in employing different representations in mechanics problem-solving and their overall learning experience in this crucial discipline.
Ethical approval
The University Of Rwanda College Of Education’s Research and Innovation Department approved this study (reference number 03/DRI-CE/103/EN/gi/2022).
Summary of the research paper.docx
Download MS Word (11.8 KB)Public interest statement.docx
Download MS Word (11.9 KB)Acknowledgement
We thank the University of Rwanda College of Education, African Centre for Excellence of Innovative Teaching and Learning Mathematics and Sciences (UR CE-ACEITLMS) who funded this study. We also thank the students and teachers who contributed actively to this study.
Disclosure statement
No potential conflict of interest was reported by the author(s).
Data availability statement
The data used in this study are available on this link DOI: 10.17632/jvny2fpybf.1
Additional information
Funding
Notes on contributors
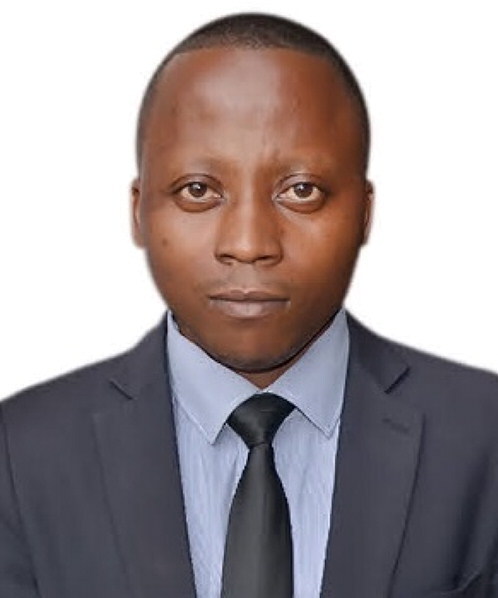
Theogene Niyomufasha
Theogene Niyomufasha University of Rwanda-College of Education (UR-CE), African Centre of Excellence for Innovative Teaching and Learning Mathematics and Science (ACEITLMS), Rwanda.
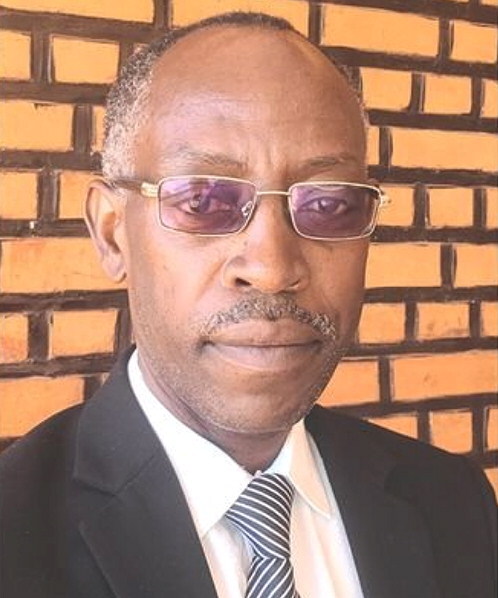
Celestin Ntivuguruzwa
Celestin Ntivuguruzwa University of Rwanda-College of Education (UR-CE), African Centre of Excellence for Innovative Teaching and Learning Mathematics and Science (ACEITLMS), Rwanda.
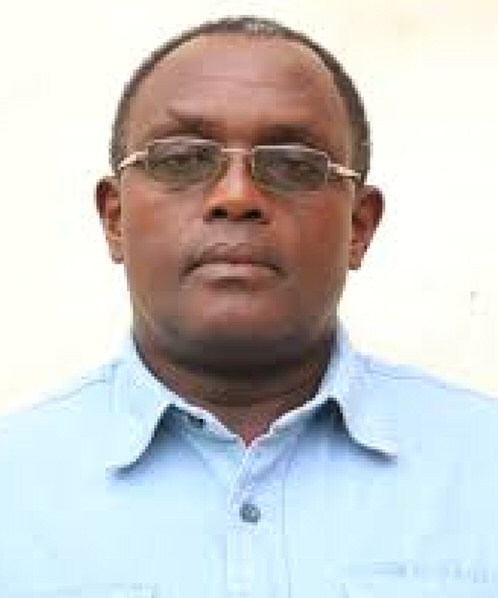
Leon Rugema Mugabo
Dr. Leon Mugabo University of Rwanda-College of Education (UR-CE), African Centre of Excellence for Innovative Teaching and Learning Mathematics and Science (ACEITLMS), Rwanda.
References
- Abdurrahman, A., Setyaningsih, C. A., & Jalmo, T. (2019). Implementating multiple representation-based worksheet to develop critical thinking skills. Journal of Turkish Science Education, 16(1), 138–155. https://doi.org/10.12973/tused.10271a
- Ainsworth, S. (2006). DeFT : A conceptual framework for considering learning with multiple representations. Learning and Instruction, 16(3), 183–198. https://doi.org/10.1016/j.learninstruc.2006.03.001
- Ainsworth, S., & Loizou, A. T. (2003). The effects of self-explaining when learning with text or diagrams. Cognitive Science, 27(4), 669–681. https://doi.org/10.1016/S0364-0213(03)00033-8
- Ainsworth, S., & VanLabeke, N. (2004). Multiple forms of dynamic representation. Learning and Instruction, 14(3), 241–255. https://doi.org/10.1016/j.learninstruc.2004.06.002
- Akçay, H., Kapici, H. O., & Akçay, B. (2020). Analysis of the representations in Turkish middle school science textbooks from 2002 to 2017. Participatory Educational Research, 7(3), 192–216. https://doi.org/10.17275/per.20.42.7.3
- Becker, S., Klein, P., Gößling, A., & Kuhn, J. (2020). Investigating dynamic visualizations of multiple representations using mobile video analysis in physics lessons effects on emotion, cognitive load and conceptual understanding. Zeitschrift Für Didaktik Der Naturwissenschaften, 26(1), 123–142. https://doi.org/10.1007/s40573-020-00116-9
- Bego, C. R., Chastain, R. J., Pyles, L. M., & Decaro, M. S. (2019 Multiple representations in physics: Deliberate practice does not improve exam scores [Paper presentation]. Proceedings - Frontiers in Education Conference, FIE, 2018-Octob, 1–7. https://doi.org/10.1109/FIE.2018.8658730
- Boyuk, H. K. ve U. (2011). Attitude towards physics lessons and physical experiments of the high school students. European Journal of Physics Education, 2(1), 23–31.
- Brenner, M. E., Mayer, R. E., Moseley, B., Brar, T., Durán, R., Reed, B. S., & Webb, D. (1997). Learning by understanding: The role of multiple representations in learning algebra. American Educational Research Journal, 34(4), 663–689. https://doi.org/10.3102/00028312034004663
- Creswell, J. W. (2012). Educational research Planning, conducting, and evaluating quantitative and qualitative research (4th ed.). MA Pearson.
- Creswell, J., & Plano Clark, V. (2011). Designing and conducting mixed methods research (2nd ed.). Sage. https://doi.org/10.4135/9781483398839.n19
- Davis, J. A., & Lorimer, S. (2018). Student performance on drawing free body diagrams and the effect on problem solving [Paper presentation]. ASEE Annual Conference and Exposition, Conference Proceedings, 2018-June. https://doi.org/10.18260/1-2–31013
- Distrik, I. W., Imam Supardi, Z. A., & Jatmiko, B. (2021). The effects of multiple representations-based learning in improving concept understanding and problem-solving ability. Journal of Physics: Conference Series, 1796(1), 012044. https://doi.org/10.1088/1742-6596/1796/1/012044
- Dufresne, R. J., Gerace, W. J., & Leonard, W. J. (1997). Solving physics problems with multiple representations. The Physics Teacher, 35(5), 270–275. https://doi.org/10.1119/1.2344681
- Elmehdi, H. M., Dalah, E. Z., & Ibrahem, A. M. (2019). Impact of flipped physics classes on the performance of engineering students: University of Sharjah Case Study [Paper presentation]. 2019 Advances in Science and Engineering Technology International Conferences, ASET 2019, May. https://doi.org/10.1109/ICASET.2019.8714477
- Euler, M. (2004). Quality development: Challenges to physics education. Quality Development in Teacher Education and Training, Girep Book of Selected Papers, Forum, Udine, 17–30. http://www.fisica.uniud.it/∼cird/girepseminar2003/abstracts/pdf/gt0.pdf
- Giancoli, D. c. (2005). PHYSICS Principles with applications (6th ed.). Pearson Education, Inc.
- Hambali, K. M. K., Sintang, S., Awang, A., Karim, K. N. M., Rahman, N. F. A., Ramli, W. A. W., Senin, N., Abidin, A. Z., Ismail, A. Z., Ali, W. Z. K. W., & Noor, R. M. (2017). al-Wasatiyyah in the practice of religious tolerance among the families of new Muslims in sustaining a well-being society. Humanomics, 33(2), 211–220. https://doi.org/10.1108/H-02-2017-0025
- Johnson-Glauch, N., & Herman, L. G. (2019). Engineering representations guide student problem-solving in statics. Journal of Engineering Education, 108(2), 220–247. https://doi.org/10.1002/jee.20258
- Kraige, J. L., & Meriam, L. G. (2012). Engineering mechanics: dynamics (7th ed.). John Wiley & Sons, Inc. http://surl.li/fwcqx
- Kroll, T., & Neri, M. (2009). Designs for mixed methods research. In Mixed methods research for nursing and the health sciences (pp. 31–49). Blackwell Publishing Ltd. https://doi.org/10.1002/9781444316490.ch3
- Lodico, M. G., Spaulding, D. T., & Voegtle, K. H. (2010). Methods in educational research : from theory to practice (2nd ed.). Jossey-Bass.
- Lucas, L. L., & Lewis, E. B. (2019). High school students’ use of representations in physics problem solving. School Science and Mathematics, 119(6), 327–339. https://doi.org/10.1111/ssm.12357
- Malone, S., Altmeyer, K., Vogel, M., & Brünken, R. (2020). Homogeneous and heterogeneous multiple representations in equation-solving problems : An eye-tracking study. November 2019, 1–18. https://doi.org/10.1111/jcal.12426
- Mapulanga, T., Ameyaw, Y., Nshogoza, G., & Sinyangwe, E. (2023). Improving secondary school biology teachers’ topic-specific pedagogical content knowledge: evidence from lesson studies. Journal of Baltic Science Education, 22(1), 20–36. https://doi.org/10.33225/jbse/23.22.20
- Martín-Blas, T., Seidel, L., & Serrano-Fernández, A. (2010). Enhancing Force Concept Inventory diagnostics to identify dominant misconceptions in first-year engineering physics. European Journal of Engineering Education, 35(6), 597–606. https://doi.org/10.1080/03043797.2010.497552
- Mešić, V., Mahmutović, S., Hasović, E., & Nataša, E. (2017). Free-body diagrams and problem solving in mechanics: An example of the effectiveness of self-constructed representations. European Journal of Physics Education, 7(3), 53–67.
- Munfaridah, N., Avraamidou, L., & Goedhart, M. (2021). The use of multiple representations in undergraduate physics education: What do we know and where do we go from here? Eurasia Journal of Mathematics, Science and Technology Education, 17(1), em1934. https://doi.org/10.29333/ejmste/9577
- Nguyen, D., & Rebello, N. S. (2011). Students ’ difficulties with multiple representations in introductory mechanics. US-China Education Review, 8(5), 559–569.
- Nurrahmawati, Sa’dijah, C., Sudirman, & Muksar, M. (2019). Multiple representations ’ ability in solving word problem. International Conference on Emerging Trends in Engineering, Technology, and Management (ICETETM-2019), 8(1C2), 737–745.
- Opfermann, M., Schmeck, A., & Fischer, H. E. (2017). Multiple representations in physics education. In D. F. Treagust, H. E. Fischer, & R. Duit (Eds.), Models and modeling in science education (10th ed., pp. 1–329). Springer. https://doi.org/10.1007/978-3-319-58914-5
- Orulebaja, Y. T., Owolabi, O. L., & Akintoye, H. (2021). Effects of Multiple representations and Problem- solving learning strategies on Physics students’ problem-solving abilities. International Journal for Innovation Education and Research, 9(4), 350–365. https://doi.org/10.31686/ijier.vol9.iss4.3045
- Pratiwi, I. K., Kusairi, S., & Sunaryono. (2021). Analyzing students ’ skill in drawing a free- body diagram [Paper presentation]. AIP Conference Proceedings 2330, 050009, 1–6. https://doi.org/10.1063/5.0043440
- Rosengrant, D., Etkina, E., & Van Heuvelen, A. (2007). An overview of recent research on multiple representations [Paper presentation]. AIP Conference Proceedings, 883, 149–152. https://doi.org/10.1063/1.2508714
- Rosengrant, D., Heuvelen, A. V., & Etkina, E. (2006). Case study: Students ’ use of multiple representations in problem solving [Paper presentation]. AIP Conference Proceedings, 818(1), 49–52. https://doi.org/10.1063/1.2177020
- Rosengrant, D., Van Heuvelen, A., & Etkina, E. (2009). Do students use and understand free-body diagrams? Physical Review Special Topics - Physics Education Research, 5(1), 1–13. https://doi.org/10.1103/PhysRevSTPER.5.010108
- Sambell, K. (2010). Enquiry-based learning and formative assessment environments: student perspectives. Practitioner Research in Higher Education, 4(1), 52–61. http://insight.cumbria.ac.uk/id/eprint/1338/
- Saputra, A. T., Paramitha, D. W., & Sarah, S. (2019). Problem-solving approach in multiple representations of qualitative and quantitative problems in kinematics motion. Jurnal Ilmiah Pendidikan Fisika Al-Biruni, 8(1), 89–98. https://doi.org/10.24042/jipfalbiruni.v8i1.3801
- Siswanto, J., Susantini, E., & Jatmiko, B. (2018). Practicality and effectiveness of the IBMR teaching model to improve physics problem solving skills. Journal of Baltic Science Education, 17(3), 381–394. https://doi.org/10.33225/jbse/18.17.381
- Tms, H., & Sirait, J. (2016). Representations based physics instruction to enhance students’ problem solving. American Journal of Educational Research, 4(1), 1–4. https://doi.org/10.12691/education-4-1-1
- Tytler, R., Prain, V., Hubber, P., & Waldrip, B. (2013). Constructing representations to learn in science. Sense Publishers. https://doi.org/10.1007/978-94-6209-203-7
- Wicaksono, A. G. C., & Korom, E. (2022). Review of problem-solving measurement: an assessment developed in the Indonesian context. Participatory Educational Research, 9(1), 116–136. https://doi.org/10.17275/per.22.7.9.1
- Wong, D., Poo, S. P., Hock, N. E., & Kang, W. L. (2011). Learning with multiple representations: An example of a revision lesson in mechanics. Physics Education, 46(2), 178–186. https://doi.org/10.1088/0031-9120/46/2/005
- Yolenta, D., Jatmiko, B., & Prastowo, T. (2019). The effectiveness of the learning devices using investigation-based multiple representation to improve students ’ problem- solving ability on reflection and refraction materials. Mathematics, Informatics, Science, and Education International Conference (MISEIC 2019), 95, 156–160.
Appendix
Appendix A: Some mechanics acheivement test problems
When does acceleration of the moving object is said to be negative?
(A) AB and DE, (B) AB and EF, (C) DE and EF, (D) BC and DE, (e) CD and EF.
How long does the object travel from time = 5hours to time = 12hours in the diagram below?
A uniform horizontal beam with a length of 8.00m and a weight of 200N is attached to a wall by a pin connection. Its far end is supported by a cable that makes an angle of 53.0° with the beam. If a 600N person stands 2.00m from the wall, find the tension in the cable as well as the magnitude and direction of the force exerted by the wall on the beam.
A billiard ball of mass mA = 0.400kg moving with a speed vA = 200m/s strikes a second ball, initially at rest, of mass mB = 0.400kg. As a result of the collision, the first is deflected off at an angle of 30° with a speed of vA = 1.20m/s. (a) Taking the x to be the original direction of motion of ball A, write down the equations expressing the conservation of momentum for the components in the x and y directions separately, (b) solve these equations for the speed, vB', and angle α, of ball B. Assume the collision is elastic.
The skier in the figure below has just begun descending the 30° slope. Assuming the coefficient of kinetic friction is 0.10, calculate (a) her acceleration and (b) the speed she will reach after 4.0s.
The force on an object, acting along the x axis, varies as shown in the figure below. Determine the work done by this force to move the object (a) from
to
and (b) from
to
A 140kg horizontal beam is supported at each end. A 320kg piano rests a quarter of the way from one end. What is the vertical force on each of the supports?
A 75kg adult sits at one end of a 9.0m long board. His 25kg child sits on the other end. (a) Where should the pivot be placed so that the board is balanced, ignoring the board’s mass? (b) Find the pivot point if the board is uniform and has a mass of 15kg.
A 650N force acts in a northwesterly direction. A second 650N force must be exerted in what direction so that the resultant of the two forces points westward? Illustrate your answer with a vector diagram.
The figure below shows a block (mass mA) on a smooth horizontal surface, connected by a thin cord that passes over a pulley to a second block (mB), which hangs vertically. (a) Draw a free-body diagram for each block, showing the force of gravity on each, the force (tension) exerted by the cord, and any normal force. (b) Apply Newton’s second law to find formulas for the acceleration of the system and the tension in the cord. Ignore friction and the masses of the pulley and cord.
Appendix B: Some interview problems
.
How would you find work in this graph problems above?
What are the forces acting on that body in its motion?
What is the relationship between the two forces?
What are the common challenges the engineering students faced while solving mechanics problems using multiple representations?
Explain some possible solutions to help overcome those challenges encountered when solving mechanics problems?