Abstract
Poly methyl methacrylate (PMMA) is the dominant acrylic that is widely used to produce partial and complete dentures. Denture fractures are caused by fatigue owing to repetitive masticatory, flexural and impact loads produced by dropping. The mechanical properties of dentures have been developed by reinforcing with various agents like rubbers, fillers and fibers. Progressively, novel dental materials reinforcement tactics have been developed following the advancement in Nano dentistry. Nonetheless, the issue pertaining to the superior mechanical properties of the PMMA still exists albeit it’s biocompatible and aesthetic properties. In recent years, the nanostructure materials have received much attention due to their high surface area to volume ratio that boosts interaction and delivers new biological, physical, and chemical characteristics. Many studies have evaluated the usage of nanotubes and nanofiber in dental composites, however, the data estimating the reinforcement issue of nanotubes and nanofibers in PMMA have been scarce. This article is aimed to review the progress related to the development of mechanical properties of denture base materials in addition to the latest advances and prospective developments for PMMA acrylic resins reinforcement. Poly methyl methacrylate PMMA composites, strengthening of dentures, Nano-fillers surface treatment, biomedical Nano additives for mechanical improvement, Nano-fillers of PMMA, biomedical ceramic Nano-fillers, biomedical metallic materials as Nano-fillers, hybrid reinforcement of PMMA, biocompatibility of polymeric PMMA composite, biomaterial for dental applications.
Public Interest Statement
This paper is aimed to review the existing literature on PMMA acrylic resin. PMMA acrylic resin is a common material in producing removable dentures to improve its mechanical properties, biocompatibility, thermal conductivity and surface roughness with the use of different nano-filler materials. PMMA is known as a biocompatible material with insufficient strength. Thus, many studies have been conducted by using different additives and processing methods to find a novel composite with superior mechanical, aging properties in addition to other specifications preferred for the oral cavity applications.
1. Introduction
Poly methyl methacrylate (PMMA) is an acrylic material widely used for denture applications owing to its useful attributes including processing simplicity, light weight, low cost, aesthetic properties, and stability in the oral environment (Sakaguchi & Powers, Citation2012). PMMA has many other advantages including superior biocompatibility, reliability, and absence of taste, odor, tissue irritation and toxicity (Cooper et al., Citation2004), teeth adhesion (Palitsch et al., Citation2012), insolubility in body fluids, relative ease of manipulation, good aesthetic appearance (J. K. Anusavice, Citation2003), and color stability (Bayindir et al., Citation2012). Moreover, PMMA based materials are preferred in various biomedical applications such as intraocular lenses (Shtilman, Citation2003), bone cement in orthopedic surgery (Kuhn, Citation2005), and removable dentures (Preshaw et al., Citation2011). Also, PMMA has been utilized for transparent glass substitutes, interior design, transparent dielectric film (Nagao et al., Citation2011), acrylic paints (Rubio et al., Citation2005), and microcellular foams
(Reglero Ruiz et al., Citation2011). Nevertheless, PMMA denture resin demonstrates poor surface characteristics and weak mechanical specifications comprising flexural strength, hardness, and fracture and impact strength (Murakami et al., Citation2013). Hence, PMMA acrylic requires enhancement in order to achieve a greater strengthening attribute. In recent years, nanotechnology has been utilized in dental applications in which many investigations have been conducted to discover the expected benefits and applications. Polymeric nanocomposites are made of nanoscale components involved in the fillers bound within the polymer matrix (Adhikari & Michler, Citation2009). A previous study reveals that nanoscale reinforcing agents could deliver new physical, mechanical and biomedical properties that generate innovative nanocomposites (Navidfar et al., Citation2016). Moreover, enhancement of PMMA has been done by means of grafting different nano-fillers (Chaijareenont et al., Citation2012). For example, alumina nanofiller with PMMA blend nanocomposite has been reported to impact the dielectric properties of the material. The new composite properties depend on the nature, morphology and size of the nanoparticles (Jordan et al., Citation2005). Nanomaterials offer superior physical properties such as density, but however, lack in terms of mechanical properties. Due to the emerging need for enhancement of the physical and mechanical characteristics, there have been numerous researches conducted in the field of nanocomposites (M.M. Gad et al., Citation2017). Existing published articles relating to the reinforcement of PMMA acrylic resin were reviewed. Subsequently, analysis of the modified characteristics of the produced composite, method of addition, surface treatment, bonding and interaction with PMMA polymer matrix, processing method, properties of the newly obtained composite, and the clinical performance of the new fabrications were also described.
1.1. Coupling agents
Coupling agents are commonly used in denture base applications in the processing stage, where they act as a molecular bond between the inorganic filler and the organic matrix. This interfacial connection is essential for transferring the applied load from the matrix to fillers where this bonding could significantly affect the mechanical specifications. The joined interface could decrease the water sorption and increase stability under wet and humid conditions and property retention (Lien & Vandewalle, Citation2010). Inorganic additives have hydrophilic properties (which are different from the organic matrix with hydrophobic properties). As such, the filler surface should be treated with a suitable coupling agent, such as silane to facilitate their bonding. (DeArmitt & Rothon, Citation2011). The coupling agent should be hydrolytically stable, provide a good waterproof bond and should not deteriorate in an aqueous working condition (Adhikari & Michler, Citation2009). Silane is known as an important
coupling agent, which is mainly used to facilitate the adhesion among dental composites. Nevertheless, silane degrades hydrolytically in the oral field and bond ineffectively to non-silica fillers, therefore, it is suitable to be used for the modification of silica materials rather than other ceramics. The titanate coupling agents (TCA) are recommended for surface modification, as they demonstrate superior bonding, improved homogeneous dispersion of fillers, better mechanical characteristics of PMMA composites and possess better surface modification effect with different types of nano-fillers (Nidal Wanis et al., Citation2017). The salinization process involves the creation of a layer of siloxane on the filler surface through the condensation process. Therefore, these layers are formed as a result of the silane interaction with the hydroxyl group (OH−), which is present on the filler surface, which will then induce the formation of the bonding between the inorganic filler with the organic polymer (Zhang & Matinlinna, Citation2011). Nevertheless, in some instances, such as in the presence of incomplete coatings, silane coupling agents γ-methacryloxypropyltrimethoxysilane (γ-MPS) aggregate on the surface of the salinized fillers and form an unstable bonding at the interface between the added filler and polymer matrix (Elshereksi et al., Citation2009; Lin et al., Citation2008). The indicated agglomeration will cause defects in the composites when exposed to an aqueous environment and cause degradation in the mechanical properties and an increase in the water sorption (Hooshmand et al., Citation2013). The absorbed saliva and liquid cause the hydrolysis of the salinized interfaces. Subsequently, this could lead to the opening of additional pathways for water diffusion inside the polymer composite resulting in cracks and deterioration in the composite (Guo et al., Citation2012; Matinlinna et al., Citation2004). On the contrary, treating the filler inorganic surface with the titanate coupling agent (TCAs) will result in the reaction of TCAs with the free proton available on the filler surface and forms a layer of titanium monomolecular, where this layer will cover the inorganic surface without the need for water for condensation (Goyal, Citation2006; Matinlinna et al., Citation2004). Titanates coupling agents usually react with the protons on the filler surface (H+), where the protons will produce a greater degree of linkage improving the performance of photorefractive effects and piezoelectric properties when compared with the interfacial deterioration data obtained with the use of the silane s coupling agents (Hajian et al., Citation2011; Matinlinna et al., Citation2004; Monte, Citation2002). (-a) presents the treatment mechanism and chemical bonding between the added filler and titanate coupling agent where three sites of reaction were observed on the filler surface. Contrary to that, (-b) illustrates the silane coupling agent forming only one functional radical site.
2. Nano additives reinforcement
Numerous studies have utilized additives to support denture acrylics, PMMA strengthening with metal oxides has enhanced physical and mechanical specifications, as well as patients’ sensitivity to cold and hot stimuli. Thus, healthier oral mucosa and better food sensation were predicted with the addition of fillers to denture acrylics (Asar et al., Citation2013; Sehajpal & Sood, Citation1989; Yadav et al., Citation2012). In recent years, nano-fillers grafting has been recommended to strengthen PMMA characteristics. The fine size, large surface area and nano-fillers uniform distribution have resulted in superior thermal characteristics and stability of PMMA in comparison with unreinforced PMMA (Asar et al., Citation2013; Safi, Citation2014; Sehajpal & Sood, Citation1989). Furthermore, the resin specifications strengthened by nanosized additives rely on the size, type, shape, and concentration of particles (Safi, Citation2014). As such, additional fillers will be studied in relation to PMMA reinforcement.
2.1. Alumina nanoparticles
Arora et al (P Arora et al., Citation2015) examined the effect of alumina (Al2O3) addition to the polymethylmethacrylate and revealed a positive impact on the acrylic resin properties. The addition of alumina to acrylic improved the thermal conductivity of dentures and provide the patient with comfortable and satisfactory feelings (Abdulhamed & Mohammed, Citation2010; N Arora et al., Citation2011; Ellakwa et al., Citation2008; Kul et al., Citation2016). Also, the involvement of aluminum oxide into the PMMA acrylic resin for denture use leads to an improvement in the flexural, tensile, impact, compressive strengths as well as surface hardness (N Arora et al., Citation2011; Asar et al., Citation2013; Ellakwa et al., Citation2008; X Zhang et al., Citation2012). Another study has indicated that the addition of Al2O3 to the PMMA considerably improved thermal conductivity, but not the development in the PMMA flexural strength (Kul et al., Citation2016). Abdulhamed et al., discovered that a specific addition of alumina will decrease both the PMMA tensile and impact strength, while it works to increase the hardness (Abdulhamed & Mohammed, Citation2010; Vojdani et al., Citation2012), flexural strength as depicted in while increasing the hardness as presented in .
Table 1. Mean flexural strength (MPa), standard deviation, minimum and maximum values
Table 2. Mean Vickers hardness number (kg/mm2), deviation, minimum and maximum values
Sehajpal et al stated that the denture resin deteriorated because of the stress concentration on the embedded material as well as an inadequate adhesive with the polymer. The surface modification of filler by, salinization, sandblasting and adhesive resins were proposed to increase the connection between the alumina ceramic surface and PMMA acrylic resin (Sehajpal & Sood, Citation1989). Alumina treatment with coupling agents improved acrylic flexural characteristics (Chaijareenont et al., Citation2012; Yadav & Elkawash, Citation2011). Moreover, saline-treated alumina considerably improved tensile, compressive, flexural strengths as well as the resin wear resistance (Chaijareenont et al., Citation2012). A study by Abdulhamed et al reported that there was no change in the surface roughness, however, water sorption was decreased in acrylic dentures reinforced with aluminum oxide at 5 wt. % (Abdulhamed & Mohammed, Citation2010; Vojdani et al., Citation2012). On the other hand, another study demonstrated a significant reduction in solubility and water sorption after the addition of alumina (Asar et al., Citation2013). Safi (Safi, Citation2014) revealed that nano-alumina (Al2O3) addition to PMMA enhanced the thermal stability in comparison with the unfilled control PMMA sample. Salinized Al2O3 NPs addition to acrylic reduced the coefficient of thermal expansion and improved the flexural strength, and this addition caused a reduction in water sorption as well as solubility (Jasim & Ismail, Citation2014). A recent study examined the (1 wt. %) alumina filler impact using two different groups of microwave-treated and untreated PMMA powder. The drawback of (1 wt. %) alumina-filled PMMA was that a mild to moderate inflammation was observed in the test groups (Abdulkareem & Hatim, Citation2015).
2.2. Zirconia nanoparticles and nanotubes
Many studies reported that filling PMMA acrylic with zirconia (ZrO2) particles significantly improved flexural strength (Abdulhamed & Mohammed, Citation2010; Abdulkareem & Hatim, Citation2015; N Arora et al., Citation2011; P Arora et al., Citation2015; Asar et al., Citation2013; Asopa et al., Citation2015; Ayad et al., Citation2008; Chaijareenont et al., Citation2012; Ellakwa et al., Citation2008; Franklin et al., Citation2005; Jasim & Ismail, Citation2014; Kul et al., Citation2016; Safi, Citation2014; Sehajpal & Sood, Citation1989; Vojdani et al., Citation2012; Yadav & Elkawash, Citation2011; X Zhang et al., Citation2012). Nevertheless, particle clustering and agglomeration inside the acrylic resulted in a deterioration in acrylic composite causing a small reduction in flexural strength (Monte, Citation2002). Also, findings demonstrated a significant increase in fracture toughness, impact strength, and PMMA hardness with the incorporation of ZrO2 filler (Asar et al., Citation2013; Franklin et al., Citation2005). Ayad et al revealed a trivial growth in surface hardness and impact strength of zirconium dioxide acrylic composite in contrast to the unfilled resin (control specimen) (Ayad et al., Citation2008), while, another study revealed a reduction in impact strength, as well as surface hardness upon the incorporation of 10wt.% ZrO2 and 20wt.% ZrO2 (Asopa et al., Citation2015). Moreover, ZrO2 addition significantly improved the PMMA thermal conductivity (Kul et al., Citation2016). The study on the effect of reinforcement of high-impact acrylic resin with zirconia demonstrated that the increase in the amount of solubility and water absorption were within the specified limits recommended by the American Dental Association (ADA), Specification No.12 (Al-Rais et al., Citation2005; Asopa et al., Citation2015; Ayad et al., Citation2008). Adding zirconia NPs has been proposed to enhance the mechanical characteristics of PMMA (Mohammed et al., Citation2016; Safi & Moudhaffar, Citation2011), with no significant changes in color specifications (Abdulhamed & Mohammed, Citation2010). Additionally, integrating zirconia NPs within the PMMA acrylic resin for denture applications has been shown to improve the flexural, impact (M Gad et al., Citation2016; Safi & Moudhaffar, Citation2011; XJ Zhang et al., Citation2011), fatigue, compressive strengths, and the fracture toughness as well as hardness (P Arora et al., Citation2015; Asar et al., Citation2013; Safi, Citation2014). Also, the antifungal effect of the material may play a protective role in patients who are vulnerable to fungal toxicities (Cierech, Kolenda et al., Citation2016). Alternatively, a study reported an increase in the amount of hardness and surface roughness of the nano-ZrO2/PMMA composite in addition to the increase in the amount of modified (ZrO2) addition. illustrates the morphology of the surface for composites filled with 5wt. % and 7wt. % of ZrO2-nanoparticles (Bian et al., Citation2007; Safi et al., Citation2012; Zhang et al., Citation2009). The low wettability of resin matrix and fillers decreased the ability to enhance the physical and mechanical specifications of PMMA. Consequently, a silane coupling agent was utilized to increase the bonding strengths between ZrO2 NPs and PMMA acrylic (Hameed & Abdul Rahman, Citation2015).
Figure 2. Surface morphology of dentures reinforced by; a- 5wt. % and; b- 7wt. % of salinized nano ZrO2 filler (Safi & Moudhaffar, Citation2011)
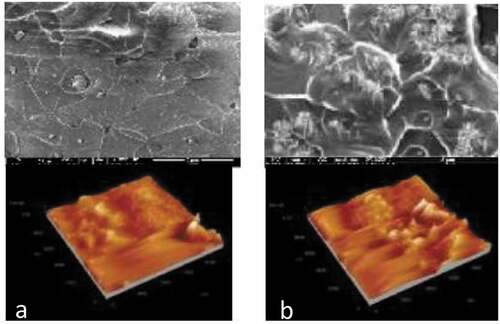
Research indicated that salinized zirconia NPs facilitated enhancements in the PMMA tensile strength and fatigue strength (Mohammed & Mudhaffar, Citation2012). Moreover, there was a substantial increase in the hardness and minimal improvement in the surface roughness following the merging of salinized zirconia NPs in acrylic resin, also there was a reduction in, porosity (Hameed & Abdul Rahman, Citation2015; Mohammed & Mudhaffar, Citation2012), solubility and water sorption (Mohammed & Mudhaffar, Citation2012). Nevertheless, the impact strength of the acrylic composite was improved, but there was no significant improvement observed in the tensile strength (Bian et al., Citation2007; Hameed & Abdul Rahman, Citation2015; Mohammed & Mudhaffar, Citation2012; Safi et al., Citation2012; Zhang et al., Citation2009). Preceding studies that investigated the effects of ZrO2 °n the PMMA denture properties revealed that ZrO2 NPs could improve the flexural as well as impact strengths (Asar et al., Citation2013; Ayad et al., Citation2008; M.M. Gad et al., Citation2017; Safi & Moudhaffar, Citation2011) (Mohammed et al., Citation2016). The maximum intensification resulted in a denture composite consisting (5 wt. %) of ZrO2 NPs (Safi & Moudhaffar, Citation2011). Moreover, it has been observed that the increase in the amount of nano-filler of more than 2.5 wt. % could cause agglomerations and clustering, which deteriorate the flexural and impact strength (M.M. Gad et al., Citation2017). As such, grafting of ZrO2 NPs into the acrylic developed the flexural strength; however, it reduced the impact strength, specifically at the high concentrations of ZrO2 NPs. In addition, ZrO2 nanotubes (NTs) exhibited better strengthening impact compared with ZrO2 NPs. Contrary to ZrO2 NPs, surface treatment would prevent the strengthening and reinforcement influence of ZrO2 NTs (Wei et al., Citation2014). However, flexural strength was improved with the addition of (2wt %) untreated ZrO2 NTs to the PMMA acrylic resin (Wei et al., Citation2014). Yu et.al (Wei et al., Citation2014), investigated the PMMA denture base reinforcement with ZrO2 NTs in which ZrO2 NTs had a greater influence of reinforcement than ZrO2 NPs. Additionally, composite flexural strength was maximized with (2.0 wt.%) grafting following the untreated ZrO2 NTs addition. provides the summary of ZrO2 additive fillers.
Table 3. The summary of ZrO2 additive fillers and its effect on denture base acrylic resin
2.3. Titanium dioxide nanoparticles and nanotubes
Many studies have been conducted on the effect of reinforcement with titanium dioxide (TiO2) particles to improve PMMA characteristics such as hardness, fracture toughness, and flexure strength of acrylic (Ahmed et al., Citation2016; Harini et al., Citation2014; Mohammed et al., Citation2016; Nejatian et al., Citation2006; XJ Zhang et al., Citation2011), as well as thermal conductivity (Zhang et al., Citation2009). An increase in the impact strength could be obtained with a slight increase in the amount of (TiO2) (Ahmed et al., Citation2016), also, a significant reduction in solubility and water sorption could be caused by titania grafting into PMMA acrylic material (Hamouda & Beyari, Citation2014). Nonetheless, several studies demonstrated that the PMMA flexural strength was limited and did not improve with the titania addition due to the particles clustering inside the acrylic that causes the creation of a weak area inside the composite (Franklin et al., Citation2005; Nejatian et al., Citation2006). Salih (Salih et al., Citation2015) revealed that modification of PMMA with TiO2 NPs could lead to a reduction in the coefficient of thermal expansion, elastic modulus, toughness and flexural strength at a filler percentage of 5wt. %. It was also found that the bond between the filler particles and the resin matrix could improve the composite characteristics (Ahmed et al., Citation2016; Harini et al., Citation2014). A study reported that the treatment with titania coupling agents TCAs for the (TiO2) powder produces a PMMA composite with improved mechanical properties (Elshereksi, Ghazali et al., Citation2014). Grafting of the PMMA acrylic resin by a salinized titania NPs could cause a development of the transverse and impact strengths, surface hardness, and a reduced solubility as well as water sorption. Alwan. et al conducted a study on the addition of 3 wt. % salinized titania NPs to PMMA acrylic and revealed that 3 wt% of salinized titania NPs to PMMA acrylic increased the surface roughness of the fabricated composite (Alwan & Alameer, Citation2015). Meanwhile, a recent study indicated that the (PMMA/TiO2)
nanotube composite is a promising antimicrobial material (Naji, Behroozibakhsh et al., Citation2018). shows the viable bacteria and fungi of TiO2-nanotube incorporated into the PMMA acrylic resin with three different weight percentages of 0wt. % (control), 2.5wt. % and 5wt. % (Naji, Sadat et al., Citation2018).
Table 4. Viable cell counts of Candida albicans, Lactobacillus acidophilus and Streptococcus mutans biofilms in non-UV activated resin and following activation with UV irradiation in a conventional denture base acrylic resin (Naji, Behroozibakhsh et al., 2018)
A further investigation was conducted on the PMMA embedded, individually, with fluoridated and non- fluoridated apatite coated titania treated with ultraviolet radiation suppressed Candida growth owing to the antifungal property of the UV radiation. Subsequently, the outcome of the aforementioned study reported the usage of such substances could provide a hygienic effect (Sawada et al., Citation2014; Shibata et al., Citation2007). Another study revealed that barium titanate (BaTiO3) caused a trivial reduction in fracture toughness when added to PMMA as a radio pacifier (Elshereksi et al., Citation2009). Moreover, a further investigation indicated that PMMA composite grafted with BaTiO3 is thermally stable; and an increase in the density could influence the retention of denture acrylic (Ahmed et al., Citation2016; Elshereksi, Mohamed et al., Citation2014). Sahar et.al (Naji, Behroozibakhsh et al., Citation2018) prepared TiO2 nanotubes (NT) by the alkaline hydrothermal process, where TiO2 was added to PMMA to examine the filler effect in the fabricated nanocomposite on the mechanical properties, and reported that hardness and flexural strength of samples adapted with (2.5 and 5 wt.%) titania NTs were significantly higher than the control samples. Moreover, fracture toughness was greater and improved in the fabricated filled samples, which were reinforced with up to (5 wt. %) NT-TiO2 (except for the one filled with 2.5% Titania NTs). Additionally, the study also examined the antimicrobial effect of TiO2 NTs using the same percentages of addition, where there was a significant reduction in planktonic cell count as well as biofilm formation on modified UV activated acrylic samples as compared with the control group. In relation to the mechanical improvement. Tandra et. al (Tandra et al., Citation2018) demonstrated that at a concentration of (1wt. %), nanoparticles TiO2 could increase the acrylic resin denture plate flexural strength. For the aesthetic and thermal study, Aziz (Aziz, Citation2018) revealed that microwave curing exhibited no effect on the stability of color as well as thermal conductivity as compared to the curing method by water bath, while the impact and color stability were enhanced through (3 wt. %) titania NPs with no effect on the thermal conductivity. presents a summary of TiO2 as a filler incorporation.
Table 5. The summary of TiO2 additive fillers and its effect on denture base acrylic resin
2.4. Zinc oxide nanoparticles and nanotubes
Salahuddin et al. (Salahuddin et al., Citation2018) reported the PMMA reinforcement with the filler of ZnO NPs as well as ZnO nanotubes, the thermal and mechanical properties of the obtained composites were improved for both groups. Besides that, there were considerable improvements reported in the impact and flexural strength values. The outcomes revealed that the increase in the amount of ZnO NPs (0.2–0.4 wt. %) caused an increase in the flexural strength. Contrarily, the flexural strength decreased at an amount of (0.8–1 wt. %) ZnO NPs. Nevertheless, the thermal stability of the nanocomposites was modified compared to the control sample or pure PMMA (Cierech, Wojnarowicz et al., Citation2016; Kamonkhantikul et al., Citation2017; Salahuddin et al., Citation2018). Vikram et.al (Santhanam Vikram & Chander, Citation2020) revealed that the addition of ZnO nanoparticles filler with different concentrations had improved the flexural strength of the composite. Moreover, Khan et.al (Khan et al., Citation2020) evaluated the flexural strength of acrylic resin strengthened by ZnO nanoparticles and the results were compared with conventional non-reinforced heat-cured acrylic. The study revealed that there was no significant improvement in the flexural strength of the obtained heat- cured PMMA denture resin along with the percentage of the increase in the amount of the added Zinc- Nano powder filler. Cierech. et al. investigated the ZnO NPs effect on PMMA, where the color of PMMA was white to some extent and the bleaching was both aesthetically and clinically acceptable. Nevertheless, the incorporation of ZnO was successful, where there was no difference in the roughness of nanocomposite compared to the pure PMMA control specimen (Cierech, Wojnarowicz et al., Citation2016). On the other hand, Kamonkhantikul., et al. investigated the effect of silanized zinc oxide nanoparticles on the antifungal,
mechanical as well as optical characteristics of acrylic resin. The ZnO-nanoparticle surfaces were treated in order to prevent the aggregation of fillers. When the silanized and non-silanized fabricated composites with the similar weight of filler-nanoparticles, were compared, Candida albicans formation was lower in the silanized group, while, the least growth was observed in the group of (5wt. %) silinazed filler. Furthermore, increased contact between silanized particles and Candida albicans may increase the antifungal effect. In the non-silanized group, the antifungal effect was detected only in the composites with (5wt. %) of ZnO-particles. However, the silanized groups had greater flexural strength values. Furthermore, the addition of ZnO NPs could cause discoloration (Kamonkhantikul et al., Citation2017). shows the mean and standard deviation of the growth of Candida albicans in each experimental salinized and non- salinized group, where the CFU refers to the colony-forming unit, Nosi refers to the non-silanized, and the Si is the silanized ones (Kamonkhantikul et al., Citation2017).
Figure 3. Mean and standard deviation of the growth of Candida albicans in each experimental group (Safi & Moudhaffar, Citation2011)
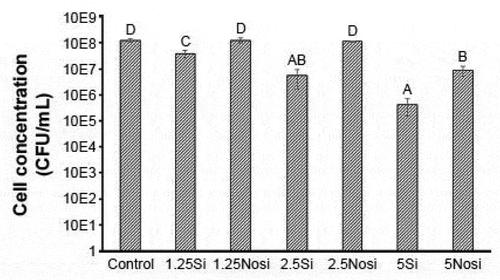
2.5. Silver nanoparticles
Many studies reported that the addition of a substantial amount of silver nanoparticles (Ag NPs) to the PMMA dentures composites exhibited antifungal characteristics (Acosta-Torres et al., Citation2012; Casemiro et al., Citation2007; Li et al., Citation2014; Monteiro et al., Citation2012; Suganya et al., Citation2014), despite the release of some ions of (Ag +) in vitro after 28 days by Ag-filler (Oei et al., Citation2012). Wady et al. revealed that the combination of Ag NPs in acrylic resin had no influence on bonds concerning the biofilm and Candida albicans accumulation regardless of the antifungal activity of AgNPs solution (Wady et al., Citation2012). Moreover, mechanical and physical specifications were enhanced by using a filler of silver NPs, this addition also improves the compressive
strength and thermal conductivity of the obtained dentures (Ghafari et al., Citation2014; Hamedi-Rad et al., Citation2014). Mahross et al. (Citation2015) stated that incorporating of Ag NPs can develop composite viscoelastic properties (Elshereksi et al., Citation2009). While, several studies reported that PMMA-AgNPs have poor color stability (Nam et al., Citation2012; Oei et al., Citation2012). Nevertheless, silver NPs reinforcement to the PMMA had no cytotoxic effects (Harini et al., Citation2014; Matinlinna et al., Citation2004). A study examined the effect of incorporating Ag NPs into PMMA acrylic reported a minimal change in tensile strength following the incorporation of PMMA material with the use of (0.2wt. %) in contrast to the non-filled PMMA acrylic resin. Meanwhile, the strength was significantly reduced following the grafting with (2wt. %) of silver-filler (Ghaffari et al., Citation2014). Grzegorz Chladek et.al (Chladek et al., Citation2019) evaluated the impact strength, flexural strength, hardness, wear resistance, solubility and water sorption of PMMA acrylic resin that had been strengthened by antibacterial silver-releasing filler after three months of storage in distilled water. The author investigated the effect of adding seven different percentages of Ag NPs filler which are (0, 0.25, 0.5, 1, 2, 4, and 8 wt. %). The results revealed a reduction of 18.4MPa to 5.5 MPa and 107MPa to 72 MPa for impact strength and flexural strength respectively. The positive outcomes of the study included the increase in flexural modulus, hardness and a reduction in volume loss through wear test. On the other hand, there was an improvement in solubility (0.95–1.49 µg/mm3) while the water sorption was relatively stable. specifies the similarity in reduction of the flexural strength for all samples with the rate of 9% to 12% (Chladek et al., Citation2019).
Figure 4. Mean flexural strength values with standard deviations (Elshereksi, Mohamed et al., Citation2014)
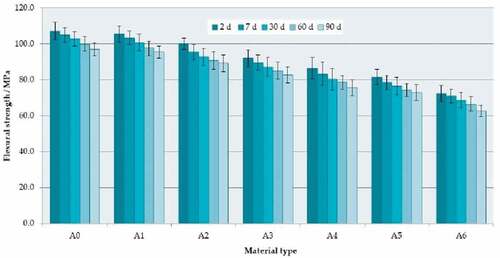
2.6. Silicon nanoparticles
The addition of silicon dioxide (SiO2) NPs as a PMMA filler could improve the surface hardness, thermal and mechanical properties, as well as transverse and impact strengths (Alnamel & Mudhaffer, Citation2014). Increasing the amount of added silica NPs will lead to accumulation and agglomeration as well as crack propagation causing a reduction in the fracture toughness and hardness of the obtained denture composite (Balos et al., Citation2014; Da Silva et al., Citation2012). Cevik et al, revealed that the incorporation of 1wt. % and 5 wt. % of unmodified SiO2 NPs did not improve the PMMA flexural strength and caused a negative effect in contrast to the control groups (P Cevik & Yildirim-Bicer, Citation2016). This was different from the surface modification of the added filler with saline material, the addition of (0.1wt.%, 0.5wt.%, 1wt.% and 5wt.%) from salinized SiO2 NP into a PMMA acrylic resin and processing by a microwaved heat‐cured furnace improved the PMMA composite flexural strength. Nevertheless, this reduced the hardness of the fabricated specimens (Da Silva et al., Citation2012). Kamil et.al (Kamil, Citation2018) evaluated the effect of the addition of silanized silicon carbide nanoparticles (Sic NPs) on the physical characteristics of PMMA acrylic resin. Incorporation of the silicon carbide filler improved the surface roughness, thermal conductivity and surface hardness at a filler-amount of 0.125 wt. %, while, this amount of filler reduced the transverse and impact strength. The thermal conductivity and surface hardness improved with an increased percentage of Sic NPs filling to 0.25 wt. %. Nonetheless, there was no significant decrease in transverse and impact strength and no influence on the surface roughness. and show the difference between the unfilled matrixes compared with the filled one. The results revealed that the filled matrix had non-uniform distribution of NPs in the matrix, hence void content was found to be higher in the filled matrix (Kamil, Citation2018).
Figure 5. Scanning electron microscope (A) PMMA without nanoparticles, (B) PMMA with nanoparticle aggregations (Kamonkhantikul et al., Citation2017)
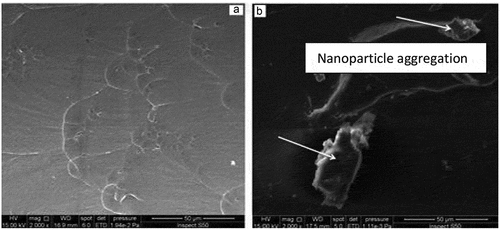
The decrease in the flexural strength is influenced by the distribution of filler particles in the matrix, however, increasing the amount of filler could reduce the flexural strength and facilitate the formation aggregation of nano-filler. Cevik et.al (Pinar Cevik & Yildirim-Bicer, Citation2016), investigated the influence of SiO2 NP and prepolymer at
percentages of 1wt. % and 5wt. % on PMMA acrylic samples on surface hardness, flexural strength, surface roughness as well as flexibility. The flexural strength of composite acrylic resin amalgamated with the prepolymer and silica was not significantly improved by the incorporation of the fillers. On the other hand, the flexibility and hardness of PMMA silica composites demonstrated a significant increase in properties as opposed to a decrease in surface roughness as the percentage of filler was increased.
2.7. Calcium carbonate nanoparticles
Reinforcement of PMMA polymer for denture applications via in-Situ polymerization method is done by filling the PMMA polymer with calcium carbonate nanoparticles (CaCO3). The incorporation of CaCO3 improved the Young’s modulus and maintained the impact strength of the fabricated composites. The presence of CaCO3 in the PMMA matrix resulted in abrasion resistance and modified the mechanism of wear and stiffness of the composites. Additionally, the tensile strength was improved compared to the non-filled PMMA acrylic material (control sample) (Chatterjee & Mishra, Citation2012). Shi et.al (Shi et al., Citation2004), investigated the PMMA grafting with modified CaCO3 NPs prepared by in-situ emulsion polymerization. CaCO3 NPs surfaces were treated by gamma-methacryloxypropyltrimethoxysilane (MPTMS) and were used for the (PMMA/CaCO3 NPs) composite preparation. The results revealed that the grafting efficiency and grafting ratio of PMMA on CaCO3 NPs modified with MPTMS were significantly higher than those on CaCO3 NPs treated by stearic acid. Also, the PMMA grafting ratio of the acrylic resin was improved with the increase in the weight ratio between MMA monomers and CaCO3 NPs, however, a decline in PMMA grafting efficiency was observed (Shi et al., Citation2004). Jagger et.al (Jagger et al., Citation1999) conducted a study on PMMA filled with modified CaCO3 NPs, where poly butyl acrylate (PBA) chains were grafted onto CaCO3 NPs surface to increase (inorganic nano-fillers/polymer) compatibility. The findings indicated that the modification of CaCO3 induced fine nanoparticles dispersion into PMMA as well as a strong interfacial bond between the two phases. Also, mechanical assessments have indicated that both modified and unmodified CaCO3 are accountable for the increase in the Young’s Modulus. Meanwhile, only PBA-grafted NPs maintain impact strength, which is strongly deteriorated by the addition of unmodified CaCO3. Notably, the presence of CaCO3 NPs could significantly improve the PMMA abrasion resistance as well as modifying its wear mechanism.
2.8. Nano carbon
Nano-carbon is one of the main branches of nanotechnology (Wang et al., Citation2015). Nonetheless, nanocarbon is an infrequent substance used to strengthen PMMA due to its cytotoxicity, difficulty in handling and polishing, as well as its poor aesthetics attribute (Mahmood, Citation2015). The addition of (1.5%) of single-wall carbon nanotubes (SWCNT) as polymethyl methacrylayte PMMA material filler could significantly increase the flexural and impact strengths of the acrylic, however it also causes deterioration in the hardness of the PMMA composite (Ibrahim, Citation2015).
shows the mean values for hardness, roughness, impact strength and transverse strength for the control group and 1.5wt. % SWCNTS incorporation into PMMA material (Ibrahim, Citation2015). Another study indicated that (2wt.%) of single-walled carbon nanotubes (SWCNT) could significantly improve the impact and flexural strengths, by fabricating the sample by conventional thermal processing of the acrylic resin, which is the new processing technique assisted with ultrasound, compared to the technique used for the 1.5wt.% preparation (Qasim et al., Citation2012). On the other hand, another study demonstrated that the flexural strength of PMMA acrylic was unaffected by the incorporation of SWCNT (Wang et al., Citation2014). Kim et.al (Kim et al., Citation2019) investigated the multiwall carbon nanotubes (MWCNT) incorporation into PMMA resin to form drug- free antimicrobial bonding characteristics. Strong anti-adhesive effects (35 ~ 95%) against three relevant microbial species (Staphylococcus aureus, Candida albicans, and Streptococcus mutans) without cytotoxicity effect on oral keratinocytes for the (1wt. %) CNT group compared to the control group of PMMA. Grafting PMMA with (0.5wt. %) as well as (1wt. %) of multi-walled carbon nanotubes (MWCNTs) enhanced both resilience and flexural strength, while the fatigue declined with a greater amount of MWCNTs (Kim et al., Citation2019). Also, (Wang et al., Citation2014) conducted a study to assess a poly methyl methacrylate denture
Figure 6. The mean values of mechanical characteristics for the control group and 1.5 % SWCNTS (Ibrahim, Citation2015)
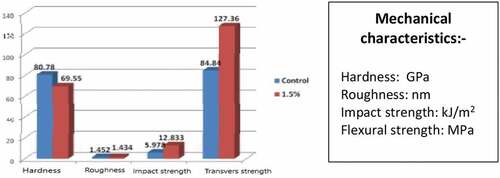
base resin modified with multi-wall carbon nanotubes MWCNTs in terms of its flexural strength, fatigue resistance, and resilience. The results indicated that 2 wt. % of MWCNTs were not useful for denture reinforcement owing to the insufficient dispersion of MWCNTs in the PMMA matrix. Meanwhile, filling of PMMA with 0.5 % and 1 % MWCNTs enhanced the flexural strength and resilience, however, all MWCNTs additions (0.5wt. %, 1wt. % and 2wt. %) revealed a poor fatigue resistance. Moreover, the morphological analysis using scanning electron microscopy displayed greater agglomerations presented on the fractured surface filled by 2 wt. % MWCNTs. describes the carbon family as filler incorporation.
Table 6. The summary of Nano carbon additive fillers and its effect on denture base acrylic resin
2.9. Nano diamond
Fahad A. Al-Harbi et.al (Al-Harbi et al., Citation2019) investigated the effect of adding three concentrations (0.5wt. %, 1.0wt.%, and 1.5wt. %) of Nano diamonds (NDs) on the produced heat polymerized acrylic resin mechanical properties. Studies indicated that the NDs addition to denture base acrylic improved the surface roughness and flexural strength at the lowest filler addition of (0.5wt. %), whereas the impact strength was reduced for the three different weight percentages (0.5wt. %, 1.0wt. %, and 1.5wt. %) in contrast to the control specimen of zero additives. The addition of 0.5 wt. % ND and 1wt. % NDs revealed a decrease in surface roughness compared to the control specimen. Mangal et.al (Mangal et al., Citation2019) studied the influence of Nano diamond (ND) filler on PMMA mechanical characteristics, the elastic modulus and flexural strength were evaluated using a three-point bending test, surface roughness has been estimated using atomic force microscopy (AFM) and surface hardness was calculated using the Vickers hardness test. The examination for fungal adhesion and viability was performed with Candida albicans. The study revealed that the amalgamation of (0.1wt. %, 0.3 wt. % and 0.5 wt. %) ND with auto-polymerized
PMMA resin substantially enhanced elastic modulus, flexural strength, and surface hardness as well as delivered a great biomedical and fungal resistance. illustrates the difference in surface roughness of the three samples including (0.1wt. %, 0.3 wt. % and 0.5 wt. %) ND comparing with a 5wt. % ZrO2 denture (Mangal et al., Citation2019).
Figure 7. (A) AFM shows average surface roughness of different groups of samples compared to 5wt. % of ZrO2 and control sample; (B) Comparison of the average surface roughness of the same group indicated in the field (A) (Nagao et al., Citation2011)
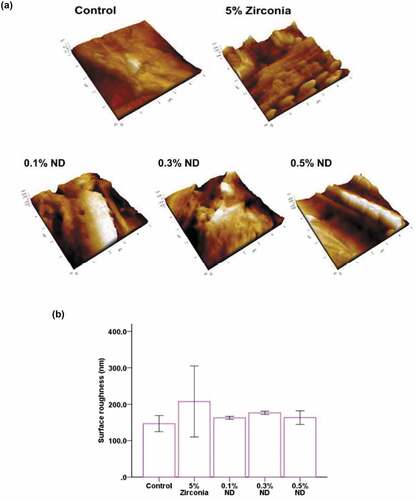
2.10. Hydroxyapatite Nanoparticles
The addition of hydroxyapatite (HA) as a filler to the PMMA acrylic material frequently produces an improvement in the mechanical properties of the fabricated composites. A study revealed that the use of hydroxyapatite (HA) as a filler improved the PMMA flexural strength and modulus of elasticity (Tham et al., Citation2010).
The interaction bonds between the PMMA matrix and HA filler were improved through the surface modification of the added particles by treatment with γ-methacryloxypropyltrimethoxysilane (γ-MPS) (Pan et al., Citation2013; Tham et al., Citation2010). Alternatively, the water plasticizing phenomena caused a decrease in flexural characteristics which causes a weakness in the PMMA/HA composite binding (Pan et al., Citation2013). HA NPs immersion in PMMA acrylic increased compression and fatigue strengths as well as thermal conductivity pertaining to unreinforced PMMA (Kul et al., Citation2016; Salih et al., Citation2015). Zebarjad et.al (Zebarjad et al., Citation2011) examined the influence of HA NPs in the PMMA nanocomposite mechanical characteristics in which wear test, compression, as well as three-point bending tests were performed. The findings revealed that the wear rate was reduced by increasing the amount of HA filler content when the testing samples were performed in both artificial saliva and atmospheric environment. The compression tests demonstrated that (2.5%) HA filler addition to PMMA enhanced ultimate compressive and yield strengths whereas a reduction in elongation at the break stage was detected. However, the incorporation of HA at more than (2.5 wt. %) caused a reduction in both ultimate compressive and compression yield strengths and also a growth in elongation at break. Furthermore, a three-point bending test indicated that the PMMA cements comprising (2.5%) HA filler had a maximum bending strength and modulus. Also, it has been indicated that there was no direct proportionality between the bending test results and the HA filler content, where, the HA filler involvement to PMMA (up to 10 wt. %) did not significantly change the bending properties (Zebarjad et al., Citation2011). Karadi et.al (Karadi & Basima, Citation2017) examined the effect of salinized Nano-hydroxyapatite (HA) on heat-cured denture base acrylic properties. Initially, HA nanoparticles were silanized with trimethacryloxypropyletrimethoxy saline (γ-MPS), then, HA filler has been mixed with methylmethacrylate monomer (MMA) and then, ultrasonicated in order to disperse the agglomerated nanoparticles. The results indicate that the addition of (2%) HA nanoparticles filler, substantially enhanced the impact strength, surface hardness and had a positive influence on solubility and water sorption. In the meantime, for the same percentage of (2%) HA nanoparticles concentration, a reduction in transverse strength and increase in the surface roughness have been reported for PMMA filled composite. provides the summary of the studies on hydroxyapatite HA as a filler in PMMA acrylic incorporation.
Table 7. The summary of HA additive fillers and its effect on denture base acrylic resin
2.11. Gold nanoparticles
Oyar et.al (Oyar et al., Citation2018) conducted a study to investigate the impact of gold nanoparticle on the flexural strength of PMMA at three different nano sizes of 45 nm, 55 nm, and 65 nm and two percentage of additions (0.05 wt. % and 0.2wt. %). Variations in PMMA flexural strength were observed with different concentrations of gold nanoparticles. Meanwhile, the size of the gold nanoparticles added to PMMA did not influence the flexural strength of the obtained PMMA dentures. A greater increase in the flexural strength of the fabricated composite was observed with lower concentrations of filler nano-gold (0.05%), in contrast to the higher gold-filler concentration of (0.20%). Nevertheless, the study conducted using nano gold-acrylic composite revealed that the gold-filler may improve the thermal and mechanical properties of the denture base obtained composite. As such, it a promising material for clinical applications. shows the differences observed between the incorporation of 0.05 wt. % Au-NP and 0.2wt. %Au-NP at three different sizes integrated into the PMMA resin (Oyar et al., Citation2018).
Figure 8. Differences between the control group composite and other groups of 0.05% Au and 0.2%Au composites (Da Silva et al., Citation2012)
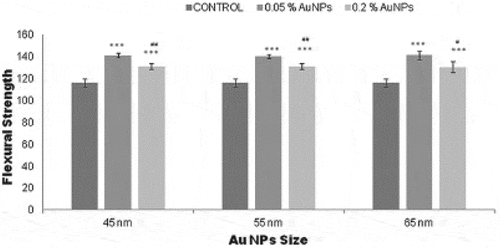
2.12. Seashell nano powder
Seashells with the structure of natural ceramics are analogous to the human bone as well as tooth structure. Karthick. et al. evaluated the hardness and tribological properties of the PMMA strengthened with seashell nano powder. The study revealed that tribological performance was significantly enhanced when incorporating (12 wt. %) seashell nano powder and also, the maximum value of microhardness was detected in the presence of (12 wt. %) seashell Nano powder. The PMMA acrylic composites filled with 16 wt.% and 20wt.% seashell nano powder revealed higher frictional forces compared to the one filled with 12 wt.%. Nevertheless, results revealed superior mechanical and physical properties by incorporating 12 wt. % seashell-Nano powder as filler, then followed by 8 wt. % nano-filled PMMA composite (Karthick et al., Citation2014).
2.13. Hybrid reinforcement
Hybrid reinforcement schemes are created to mix various metal oxides, fibers, or both to enhance the physical characteristics rather than adding each of these materials in the matrix individually (M.M. Gad et al., Citation2017; Salih et al., Citation2015; Yu et al., Citation2012). Evidence has shown that the hybrid reinforcement using both fillers and fibers could increase the impact strength (Chen & Liang, Citation2004). Hybrid reinforcement is performed through the following approaches: grafting with more than one type of fibers (Yu et al., Citation2012), merging a variety of ceramics and metal oxides (Basima & Aljafery, Citation2015; Safarabadia et al., Citation2014), grafting with both fibers and filler oxides together (Alhareb & Ahmad, Citation2011; Chen & Liang, Citation2004; Muklif & Ismail, Citation2015), or using a mixture of ceramic fillers (K.J. Anusavice, Citation2003; Zhang et al., Citation2014). A preceding study reported that the toughness and flexural strength of acrylic resins could be strengthened with a hybrid of fiber-reinforcing materials (Hari Prasad & Kalavathy, Citation2011). Prasad et al examined reinforcement with the use of silanized glass fiber, where the author discovered that the filler incorporation considerably improved the impact strength of PMMA to 126.43 ± 8.709 J at a complete fracture (Hari Prasad & Kalavathy, Citation2011). The incorporation of different materials as a hybrid improved the surface roughness of acrylic denture (Alhareb & Ahmad, Citation2011), flexural modulus, tensile strength (Alhareb et al., Citation2016), radiopacity (Safarabadia et al., Citation2014) and shrinkage reduction (Alhareb et al., Citation2015; Han et al., Citation2015), these hybrid additives enhanced the anti-bacterial characteristics without cytotoxic effects (Chen & Liang, Citation2004). The mixture of fibers and metal oxides improved hardness, impact strength (Muklif & Ismail, Citation2015), surface roughness, fatigue and compressive strength (Qasim et al., Citation2012). The improvement of flexural strength and impact strength were (94.05 ± 6.95 MPa) and (3.89 ± 0.46 kJ/m2) respectively, with the addition of (2.5wt. %nano-ZrO2 + 2.5wt. % GF) mixtures to the PMMA acrylic resin (M.M. Gad et al., Citation2017). Salman et.al (Wang et al., Citation2014) conducted a study to discover a novel nanocomposite of PMMA grafted with hybrid salinized of ZrO2/TiO2 and evaluated the transverse, impact strength as well as thermal conductivity. There were significant increase in the impact strength (9.838 kJ.m−2) and transverse strength (101.705 n.mm−2), with the incorporation of the hybrid of ZrO2/TiO2 at a percentage of 2wt. % in 1:1 ratio. Additionally, the 1:1 ratio produced a progressive influence on thermal conductivity. Gad et.al (Mohammed M. Gad et al., Citation2019) investigated the hybrid strengthening effects of glass fibers (GFs) and zirconium oxide nanoparticles at various amounts of mixtures on the impact and flexural strengths of PMMA. Nonetheless, the test sample had varying nano-ZrO2/GFs mixing amount of (5%) with PMMA, compared to the control sample, which had no filling additives. There were significant enhancements in the impact and flexural strengths of the last hybrid composites in contrast to unreinforced PMMA samples. Whereas, the optimal values of impact and flexural strengths were achieved at (2.5%) for both of ZrO2 NPs and GF mixing ratio, where the maximum flexural strength (94.05 ± 6.95 MPa), and impact strength (3.89 ± 0.46 kJ/m2) were recorded. Alhareb et.al (Alhareb, Citation2015) investigated the effect of mixing of nitrile butadiene rubber particles NBR, (5, 7.5, and 10 wt. %) with silanized ceramic (aluminum oxide, yttria stabilized zirconia, and silicon dioxide) of 5wt. %, to reinforce the mechanical properties of denture base of PMMA acrylic. Mechanical properties of fracture toughness (KIC) and impact strength (IS) of the produced PMMA composite strengthened by NBR particles with ceramic fillers have been improved, where the optimal values achieved for both IS and KIC were obtained with (10 wt.%) of NBR particles with (5 wt.%) Al2O3, giving the highest impact strength of 8.26.kJ.m−2, with optimal fracture toughness of 2.77 Mpa.m1/2. Aljafery et.al (Basima & Aljafery, Citation2015) studied the effect of 2wt.% filler of nanoparticles mixed at a ratio of (2:1, ZrO2: Al2O3) by weight, and investigated their effect on transverse strength, impact strength, hardness, roughness and denture base adaptation of heat-cured acrylic resin (PMMA). The results indicated that nano-fillers introduction into PMMA produced a substantial rise in the impact strength compared with the control group. The increase in the impact strength could be caused by the high interfacial shear strength between matrix and nano-filler resulted from the cross-linking formation. The transverse strength test analysis exhibited a significant increase with nanocomposite in contrast to the control group. This could be attributed to transformation toughening, in which when the adequate stress grows and crack begins to spread, a transformation of Al2O3 and ZrO2 reduces the crack propagation energy. Chen et.al (Rongrong et al., Citation2017) studied the biomedical properties, cytotoxicity and mechanical characteristics of the resulting composites of PMMA using the filling with salinized zirconia nanoparticles ZrO2 (2wt. %) and silanized Aluminum borate whiskers (ABW) (4wt. %) merged with antibacterial materials. The inorganic antibacterial substances used were, silver-supported titanium dioxide, titanium dioxide, silver-supported zirconium phosphate and tetrapod-like zinc oxide whiskers. The aforementioned materials were mixed individually at a percentage of (3wt. %) with (ZrO2- ABWs/PMMA) mixture to produce the testing samples. The results revealed that the composites of (ZrO2-ABWs/PMMA) mixed with silver-supported zirconium phosphate and tetrapod-like zinc oxide whiskers had the greater antibacterial effect, surface hardness, flexural strength and non-cytotoxicity. Abdel‑ Karim et.al (Basima & Aljafery, Citation2015), evaluated the effect of characterizing and synthesizing of inorganic zirconium oxide (ZrO2), organic [triethylene glycol dimethacrylate (TEGDMA) + bisphenol A diglycidyl ether dimethacrylate (Bis‑ GMA) + polyethylene glycol dimethacrylate (PEGDMA)] and hybrid (ZrO2 + Bis‑ GMA + TEGDMA) nanofibers as well as the use of these nanofibers for improving flexural modulus (FM), flexural strength (FS), fracture toughness (FT) and impact strength (IS) of polymethyl methacrylate (PMMA) resin. The findings demonstrated that (ZrO2), (Bis‑ GMA/TEGDMA/PTEGDMA) and (ZrO2/Bis‑ GM/TEGDMA) hybrid nanofibers produced by the electrospinning method enhanced substantially the flexural strength and flexural modulus, fracture toughness and impact strength of PMMA acrylic resin. Elmadani et.al (Elmadani et al., Citation2019) investigated the characterization and processing of hybrid PMMA composites through electrospun polystyrene (PS) fibers mixing with Nano-zirconia (ZrO2) as a hybrid material, the strengthening materials were selected in order to improve the mechanical and physical properties of the obtained hybrid composite. Surface modification of the filled inorganic particles by (3-Methacryloxypropyltrimethoxy silane MEMO) was achieved for increasing the reinforcement adhesion of the filler with the PMMA matrix. Hence, the PS fiber material is a promising solution to reduce the brittleness initiated from the use of ceramic nanoparticles filler, which has the tendency to agglomerate in the polymer matrix. Microhardness and impact test revealed that the optimal results were achieved by combining PS fibers and ZrO2/MEMO in an acrylate matrix, where the fibers were able to compensate for brittleness caused by the ceramic nanoparticles. In addition, the modified filler insertion in the PMMA matrix increased the contact area and bonding forces between the matrix and the PS fibers and produced the optimal combination of materials in the obtained composite.
Lilda et.al (Rebecca Lilda et al., Citation2020) estimated the flexural and impact strengths of high-impact acrylic resin strengthened with salinized titanium dioxide (1wt. %) and zirconium dioxide nanoparticles (5wt. %) as well as their hybrid combination with a percentage of the additive combination of (2wt. %) (1% ZrO2 and 1% TiO2). Nevertheless, the highest impact strength of the acrylic resin was observed with TiO2 filler- nanoparticles reinforcement, while, the maximum value of flexural strength in acrylic composite was detected in reinforcement with a hybrid mixture ZrO2/TiO2 in contrast to reinforcement with zirconium dioxide or titanium dioxide nanoparticles individually. Meanwhile, another study revealed that the inclusion of hybrid filler as a reinforcement for dentures is a promising option for enhancing the impact and flexural strengths of acrylic material. summarizes the different hybrid reinforcement materials that have been used in previous studies.
Table 8. Hybrid reinforcement added materials
3. Conclusion
Denture bases are frequently susceptible to a humid oral environment due to the continuous presence of saliva in the oral cavity. Improvements of denture acrylic characteristics could be obtained by filling
with nanomaterials, in particular, the incorporation of nanofibers or nanotubes significantly improved the PMMA properties than the nanoparticle fillers. This is mainly because the nanotubes and nanofibers have a higher surface area to volume ratio than the nanoparticle fillers. Moreover, hybrid mixing materials provide superior balance and stability between the properties of the mixed fillers and produce an optimal combination in the obtained composite. Therefore, the incorporation of vegetable fiber and natural fibers is suggested, however, this requires further investigations. Increasing the bonding strength between the added fillers and polymers is an important factor for improving the denture properties, where this could be achieved with the treatment of the filler surface by a coupling agent. Titanium-based coupling agents (TCA) exhibit satisfactory interfacial bonding, enhanced homogeneous filler dispersion, and improved mechanical properties of the composites. Moreover, TCAs have demonstrated superior hydrolytic stability in wet environments. In contrast, silane, when used as a treatment for a non-silica filler, degraded hydrolytically when exposed to aqueous environments despite the fact that, hydrolytic-stability of the composite is an essential requirement for dental materials. The curing method could increase the polymerization process and plays a significant role in the improvement of biocompatibility. As such, the water curing method could reduce the cytotoxicity of the composite compared to the microwave curing method, and also could increase the mechanical properties and reduce the residual monomers in the dentures. Therefore, filler incorporation, type of materials, hydrolytic stability, surface modification, processing, and curing methods influence the enhancements in mechanical, physical, thermal and biomedical properties. Future investigations are required in relevant to enhancement of the mechanical properties and increase the aging resistance to ensure the sustainability of the denture materials for a longer period.
Additional information
Funding
Notes on contributors
Ban Ali Sabri
We are a team of four researchers, specialized in material science and Nano applications with experience in the different engineering fields. Our different pool of experience gained through working in nanomaterials field facilitated the efforts to conduct this work. This paper summarizes the implemented work of reinforcing the Poly Methyl Methacrylate (PMMA) material through the addition of many different nanomaterials and processing aimed for the improvement of the PMMA mechanical and biomedical properties to be fit with the requirements of dental applications. The current review includes articles and research materials from multiple universities including Malaysia and Iraqi universities.
References
- Abdel‑ Karim, U. M., & El‑ Refaie, S. (2019). Synthesis of zirconia, organic and hybrid nanofibers for reinforcement of polymethyl methacrylate denture base: Evaluation of flexural strength and modulus, fracture toughness and impact strength. Tanta Dental Journal, 16, 12–25. https://doi.org/10.4103/tdj.tdj_23_18
- Abdulhamed, A. N., & Mohammed, A. M. (2010). Evaluation of thermal conductivity of alumina reinforced heat cure acrylic resin and some other properties. Journal of Baghdad College of Dentistry, 22, 1–7. https://www.semanticscholar.org/paper/Evaluation-of-thermal-conductivity-of-alumina-heat-Ali/98006cfbab6ac8a80509d4223fcf1f35cd138561?p2df
- Abdulkareem, M. M., & Hatim, N. A. (2015). Evaluation the biological effect of adding aluminum oxide, silver nanoparticles into microwave treated PMMA powder. International Journal of Enhanced Research in Science Technology & Engineering, 4, 172–178. https://www.semanticscholar.org/paper/Evaluation-the-biological-effect-of-adding-Aluminum-Abdulkareem-Hatim/493cdf2ec23c3478c9129bd6551b9771ec088a60
- Acosta-Torres, L. S., Mendieta, I., Nuñez-Anita, R. E., Cajero-Juárez, M., & Castaño, V. M. (2012). Cytocompatible antifungal acrylic resin containing silver nanoparticles for dentures. International Journal of Nanomedicine, 7, 1–10. https://www.ncbi.nlm.nih.gov/pmc/articles/PMC3435119/
- Adhikari, R., & Michler, G. H. (2009). Polymer nanocomposites characterization by microscopy. Polymer Reviews, 49(3), 141–180. https://doi.org/10.1080/15583720903048094
- Ahmed, M. A., & Ebrahim, M. I. (2014). Effect of zirconium oxide nano-fillers addition on the flexural strength, fracture toughness, and hardness of heat-polymerized acrylic resin. World Journal of Nano Science and Engineering, 4(2), 50–57. https://doi.org/10.4236/wjnse.2014.42008
- Ahmed, M. A., El-Shennawy, M., Althomali, Y. M., & Omar, A. A. (2016). Effect of titanium dioxide nano particles incorporation on mechanical and physical properties on two different types of acrylic resin denture base. World Journal of Nano Science and Engineering, 6(3), 111–1119. https://doi.org/10.4236/wjnse.2016.63011
- Al-Harbi, F. A., Abdel-Halim, M. S., Gad, M. M., Fouda, S. M., Baba, N. Z., AlRumaih, H. S., & Akhtar, S. (2019). Effect of nanodiamond addition on flexural strength, impact strength, and surface roughness of pmma denture base. Journal of Prosthodontics, 28(1), 417–425. https://doi.org/10.1111/jopr.12969
- Alhareb, A. O. Akil HBM and Ahmad ZAB.(2015). Poly (methyl methacrylate) denture base composites enhancement by various combinations of nitrile butadiene rubber/treated ceramic fillers”. Journal of Thermoplastic Composite Materials, 30(8), 1069-1090. https://journals.sagepub.com/doi/abs/10.1177/0892705715616856
- Alhareb, A. O., & Ahmad, Z. A. (2011). Effect of Al2O3/ZrO2 reinforcement on the mechanical properties of PMMA denture base. Journal of Reinforced Plastics and Composites, 30(1), 1–8. https://doi.org/10.1177/0731684410379511
- Alhareb, A. O., Akil, H. M., & Ahmad, Z. A. (2015). Mechanical properties of PMMA denture base reinforced by nitrile rubber particles with Al2O3/YSZ fillers. Procedia Manufacturing, 2, 301–306. https://doi.org/10.1016/j.promfg.2015.07.053
- Alhareb, A. O., Akil, H. M., & Ahmad, Z. A. (2016). Influence of Al2O3/Y-TSZ mixture as filler loading on the radiopacity of PMMA denture base composites. Procedia Chemistry, 19, 646–650. https://doi.org/10.1016/j.proche.2016.03.065
- Alnamel, H. A., & Mudhaffer, M. (2014). The effect of silicon dioxide nano-fillers reinforcement on some properties of heat-cure polymethylmethacrylate denture base material. Journal of Baghdad College of Dentistry, 26(1), 32–36. https://doi.org/10.12816/0015142
- Al-Rais, R. Y., Al-Nakkash, W. A., & Al-Bakri, A. K. (2005). Filler reinforced acrylic denture base material. part 2- effect of water sorption on dimensional changes and transverse strength. Journal of Baghdad College of Dentistry, 17, 6–10. https://www.semanticscholar.org/paper/Filler-reinforced-acrylic-denture-base-material.-2-Widad-Rais/950fbf27f10fccac805a7eb8410a50dc4ed1f3e2?p2df
- Alwan, S. A., & Alameer, S. S. (2015). The effect of the addition of silanized nano titania fillers on some physical and mechanical properties of heat cured acrylic denture base materials. Journal of Baghdad College of Dentistry, 27(1), 86–91. https://doi.org/10.12816/0015269
- Anusavice, J. K. (2003). Phillip’s science of dental materials. WB Saunders.
- Anusavice, K. J. (2003). Phillip’s science of dental materials (11th ed.). Saunders: Elsevier Inc..
- Arora, N., Jain, V., Chawla, A., & Mathur, V. P. (2011). Effect of addition of sapphire (Aluminum oxide) or silver fillers on the flexural strength, thermal diffusivity and water sorption of heat-polymerized acrylic resins. International Journal of Prosthodontics and Restorative Dentistry, 1(1), 21–27. https://doi.org/10.5005/jp-journals-10019-1004
- Arora, P., Singh, S. P., & Arora, V. (2015). Effect of alumina addition on properties of polymethylmethacrylate – A comprehensive review. International Journal of Biotech Trends and Technology, 9, 1–7. https://www.semanticscholar.org/paper/Effect-of-Alumina-Addition-on-Properties-of-Poly-A-Arora-Singh/ab1f0075bfd7a20efb58c3d78e54dba9bc396ca5
- Asar, N. V., Albayrak, H., Korkmaz, T., & Turkyilmaz, I. (2013). Influence of various metal oxides on mechanical and physical properties of heat-cured polymethylmethacrylate denture base resins. The Journal of Advanced Prosthodontics, 5(3), 241–247. https://doi.org/10.4047/jap.2013.5.3.241
- Asopa, V., Suresh, S., Khandelwal, M., Sharma, V., Asopa, S. S., & Kairal, L. S. (2015). A comparative evaluation of properties of zirconia reinforced high-impact acrylic resin with that of high-impact acrylic resin. The Saudi Journal for Dental Research, 6(2), 146–151. https://doi.org/10.1016/j.sjdr.2015.02.003
- Ayad, N. M., Dawi, M. F., & Fatah, A. A. (2008). Effect of reinforcement of high-impact acrylic resin with micro-Zirconia on some physical and mechanical properties. Revista De Clínica E Pesquisa Odontológica, 4(3), 145–151. http://dx.doi.org/10.7213/aor.v4i3.23218
- Aziz, H. K. (2018). TiO2-nanofillers effects on some properties of highly- impact resin using different processing techniques. The Open Dentistry Journal, 12(1), 202–212. https://doi.org/10.2174/1874210601812010202
- Balos, S., Pilic, B., Markovic, D., Pavlicevic, J., & Luzanin, O. (2014). Polymethylmethacrylate nanocomposites with low silica addition. The Journal of Prosthetic Dentistry, 111(4), 327–334. https://doi.org/10.1016/j.prosdent.2013.06.021
- Basima, M. A., & Aljafery, A. M. A. (2015). Effect of addition ZrO2-Al2O3 nanoparticles mixture on some properties and denture base adaptation of heat cured acrylic resin denture base material. Journal of Baghdad College of Dentistry, 27(3), 15–21. https://doi.org/10.12816/0015028
- Bayindir, F., Kurklu, D., & Yanikoglu, N. D. (2012). The effect of staining solutions on the color stability of provisional prosthodontic materials. Journal of Dentistry, 40(2), e41–e46. https://doi.org/10.1016/j.jdent.2012.07.014
- Bian, Y. M., Zhang, X. Y., Zhu, B. S., Yu, W. Q., Ruan, D. P., & Min, M. (2007). Influence of different amount of silane coupling agent on the flexural strength of PMMA/nanometer ZrO2 composites. Shanghai Kou Qiang Yi Xue, 16(3), 319–323. PMID: 17660924
- Casemiro, L. A., Gomes Martins, C. H., Pires-de-souza Fde, C., & Panzeri, H. (2007). Antimicrobial and mechanical properties of acrylic resins with incorporated silver–zinc zeolite – Part I. Gerodontology, 25(3), 187–194. https://doi.org/10.1111/j.1741-2358.2007.00198.x
- Cevik, P., & Yildirim-Bicer, A. Z. 2016. The effect of silica and prepolymer nanoparticles on the mechanical properties of denture base acrylic resin. Journal of Prosthodontics : Official Journal of the American College of Prosthodontists, (Nov), 29. Epub. https://doi.org/10.1111/jopr.12573
- Cevik, P., & Yildirim-Bicer, A. Z. (2016). The effect of silica and prepolymer nanoparticles on the mechanical properties of denture base acrylic resin. Journal of Prosthodontics, 27(8), 1–8. https://doi.org/10.1111/jopr.12573
- Chaijareenont, P., Takahashi, H., Nishiyama, N., & Arksornnukit, M. (2012). Effect of different amounts of 3-methacryloxypropyltrimethoxysilane on the flexural properties and wear resistance of alumina reinforced PMMA. Dental Materials Journal, 31(4), 623–628. https://doi.org/10.4012/dmj.2012-056
- Chatterjee, A., & Mishra, S., Rheological, thermal and mechanical properties of nano-calcium carbonate (CaCO3)/poly (methyl methacrylate) (PMMA) core-shell nanoparticles reinforced polypropylene (PP) composites, Accepted June 12, 2012.
- Chen, S., & Liang, W. (2004). Effects of fillers on fiber reinforced acrylic denture base resins. Mid-Taiwan Journal of Medicine, 9(4), 203–210. https://doi.org/10.6558/MTJM.2004.9(4).2
- Chladek, G., Pakieła, K., Pakieła, W., Z˙mudzki, J., Adamiak, M., & Krawczyk, C. (2019). Effect of antibacterial silver-releasing filler on the physicochemical properties of poly (methyl methacrylate) denture base material. Materials, 12(24), 1–24. https://doi.org/10.3390/ma12244146
- Cierech, M., Kolenda, A., Grudniak, A. M., Wojnarowicz, J., Woźniak, B., Gołaś, M., Swoboda-Kopeć, E., Łojkowski, W., & Mierzwińska-Nastalska, E. (2016). Significance of polymethylmethacrylate (PMMA) modification by zinc oxide nanoparticles for fungal biofilm formation. International Journal of Pharmaceutics, 510(1), 323–335. https://doi.org/10.1016/j.ijpharm.2016.06.052
- Cierech, M., Wojnarowicz, J., Szmigiel, D., Bączkowski, B. O. H. D. A. N., Grudniak, A. M., Wolska, K. I., Łojkowski, W., & Mierzwińska-Nastalska, E. L. Ż. B. I. E. T. A. (2016). Preparation and characterization of ZnOPMMA resin nanocomposites for denture bases. Acta of Bioengineering and Biomechanics, 18(2), 31–41. https://doi.org/10.5277/ABB-00232-2014-04
- Cooper, S., Visser, S., Hergenrother, R., & Lamba, N. (2004). Polymers. In B. Ratner, A. Hoffman, & F. Schoen (Eds.), Biomaterials science: an introduction to materials in medicine (2nd ed., pp. 67–80). Elsevier Academic Press.
- Da Silva, L. H., Feitosa, S. A., Valera, M. C., de Araujo, M. A., & Tango, R. N. (2012). Effect of the addition of silanated silica on the mechanical properties of microwave heat-cured acrylic resin. Gerodontology, 29(2), 1019–1023. https://doi.org/10.1111/j.1741-2358.2011.00604.x
- DeArmitt, C., & Rothon, R. (2011). Dispersants and coupling agents. In M. Kutz (Ed.), Applied plastics engineering handbook: Processing and materials (pp. 441–454). Elsevier.
- Ellakwa, A. E., Morsy, M. A., & El-Sheikh, A. M. (2008). Effect of aluminum oxide addition on the flexural strength and thermal diffusivity of heat-polymerized acrylic resin. Journal of Prosthodontics, 17(6), 439–444. https://doi.org/10.1111/j.1532-849X.2008.00318.x
- Elmadani, A. A., Radović, I., Tomić, N. Z., Petrović, M., Stojanović, D. B., Jančić Heinemann, R., & Radojević, V. (2019). Hybrid denture acrylic composites with nanozirconia and electrospun polystyrenefibers. Plos One, 4(12), e0226528. https://doi.org/10.1371/journal.pone.0226528
- Elshereksi, N. W., Ghazali, M. J., Muchtar, A., & Azhari, C. H. (2014). Perspectives for Titanium-derived fillers usage on denture base composite construction: A review article. Advances in Materials Science and Engineering, 1, 1–14. https://doi.org/10.1155/2014/746252
- Elshereksi, N. W., Mohamed, S. H., Arifin, A., & Mohd Ishak, Z. A. (2009). Effect of filler incorporation on the fracture toughness properties of denture base poly (methyl methacrylate). Journal of Physical Sciences, 20(2), 1–12.
- Elshereksi, N. W., Mohamed, S. M., Arifin, Z., & Mohd Ishak, Z. A. (2014). Thermal characterization of poly (methyl methacrylate) filled with barium titanate as denture base material. Journal of Physical Sciences, 25(2), 15–27.
- Franklin, P., Wood, D. J., & Bubb, N. L. (2005). Reinforcement of poly (methyl methacrylate) denture base with glass flake. Dental Materials, 21(4), 365–370. https://doi.org/10.1016/j.dental.2004.07.002
- Gad, M., ArRejaie, A. S., Abdel-Halim, M. S., & Rahoma, A. (2016). The reinforcement effect of nano-zirconia on the transverse strength of repaired acrylic denture base. International Journal of Dentistry, 2016, 7094056. https://doi.org/10.1155/2016/7094056
- Gad, M. M., Fouda, S. M., Al-Harbi, F., N¨ap¨ankangas, A., . R., & Raustia, A. (2017). PMMA denture base material enhancement: A review of fiber, filler, and nanofiller addition. International Journal of Nanomedicine, 12, 3801–3812. https://doi.org/10.2147/IJN.S130722
- Gad, M. M., Al-Thobity, A. M., Rahoma, A., Abualsaud, R., Al-Harbi, F. A., & Akhtar, S. (2019). Reinforcement of PMMA denture base material with a mixture of zro2 nanoparticles and glass fibers. International Journal of Dentistry, 2019, 1–11. https://doi.org/10.1155/2019/2489393
- Ghafari, T., Hamedi-Rad, F., & Ezzati, B. (2014). Does addition of silver nanoparticles to denture base resin increase its thermal conductivity? Journal of Dental Sciences, 32, 139–144.
- Ghaffari, T., Hamedi-rad, F., & Ezzati, B. (2014). In Vitro comparison of compressive and tensile strengths of acrylic resins reinforced by silver nanoparticles at 2% and 0.2% concentrations. Journal of Dental Research, Dental Clinics, Dental Prospects, 8(4), 204–209. https://doi.org/10.5681/joddd.2014.037
- Goyal, S. (2006). Silanes: chemistry and applications. The Journal of Indian Prosthodontic Society, 6(1), 14–18. https://doi.org/10.4103/0972-4052.25876
- Guo, G., Fan, Y., Zhang, J. F., Hagan, J. L., & Xu, X. (2012). Novel dental composites reinforced with zirconia- silica ceramic nanofibers. Dental Materials, 28(4), 360–368. https://doi.org/10.1016/j.dental.2011.11.006
- Hajian, M., Koohmareh, G. A., & Mostaghasi, A. (2011). Investigation of the effects of titanate as coupling agent and some inorganic nanoparticles as fillers on mechanical properties and morphology of soft PVC. International Journal of Polymer Science, 2011, 1–9. https://doi.org/10.1155/2011/238619
- Hamedi-Rad, F., Ghaffari, T., Rezaii, F., & Ramazani, A. (2014). Effect of nanosilver on thermal and mechanical properties of acrylic base complete dentures. Journal of Dentistry (Tehran, Iran), 11(5), 495–505. PMCID: PMC4290768
- Hameed, H. K., & Abdul Rahman, H. (2015). The effect of addition nano particle ZrO2 on some properties of autoclave processed heat cures acrylic denture base material. Journal of Baghdad College of Dentistry, 27(1), 32–39. https://doi.org/10.12816/0015262
- Hamouda, I. M., & Beyari, M. M. (2014). Addition of glass fibers and titanium dioxide nanoparticles to the acrylic resin denture base material: Comparative study with the conventional and high-impact types. Oral Health and Dental Management, 13(1), 107–112.
- Han, Z., Zhu, B., Chen, R., Huang, Z., Zhu, C., & Zhang, X. (2015). Effect of silver- supported materials on the mechanical and antibacterial properties of reinforced acrylic resin composites. Materials & Design (1980-2015), 65, 1245–1252. https://doi.org/10.1016/j.matdes.2014.10.023
- Hari Prasad, A., & Kalavathy, M. H. S. (2011). Effect of glass fiber and silane treated glass fiber reinforcement on impact strength of maxillary complete denture. Ann Essen Dental, 4(4), 7–12. https://doi.org/10.5368/aedj.2011.3.4.1.2
- Harini, P.,Mohamed, K., & Padmanabhan, T.V.(2014).Effect of titanium dioxide nanoparticles on the flexural strength of polymethylmethacrylate: An in vitro study.Indian Journal of Dental Research : Official Publication of Indian Society for Dental Research,25(4),459–463.https://doi.org/10.4103/0970-9290.142531
- Hooshmand, T., Matinlinna, J. P., Keshvad, A., Eskandarion, S., & Zamani, F. (2013). Bond strength of a dental leucite-based glass ceramic to a resin cement using different silane coupling agents. Journal of the Mechanical Behavior of Biomedical Materials, 17, 327–332. https://doi.org/10.1016/j.jmbbm.2012.08.020
- Ibrahim, R. A. (2015). The effect of adding single walled carbon nanotube with different concentrations on mechanical properties of heat-cure acrylic denture base material. Journal of Baghdad College of Dentistry, 27(3), 28–32. https://doi.org/10.12816/0015031
- Jagger, D. C., Harrison, A., & Jandt, K. D. (1999). The reinforcement of dentures. Journal of Oral Rehabilitation, 26(3), 185–194. https://doi.org/10.1046/j.1365-2842.1999.00375.x
- Jasim, B. S., & Ismail, I. J. (2014). The effect of silanized alumina nano-fillers addition on some physical and mechanical properties of heat cured polymethylmethacrylate denture base material. Journal of Baghdad College of Dentistry, 26(2), 18–23. https://doi.org/10.12816/0015190
- Jordan, J., . K., Jacob, K. I., Tannenbaum, R., Sharaf, M. A., & Jasiuk, I. (2005). Experimental trends in polymer nanocomposites—a review. Materials Science and Engineering: A, 393(1–2), 1–11. https://doi.org/10.1016/j.msea.2004.09.044
- Kamil, A. S. (2018). Hikmet Jameel Al-Judy, “Effect of addition of silanized silicon carbide nanoparticles on some physical properties of heat cured acrylic denture base material”. Journal of Research in Medical and Dental Science, 6(6), 86–95. www.jrmds.in eISSN No.2347-2367: pISSN No.2347-2545
- Kamonkhantikul, K., Arksornnukit, M., & Takahashi, H. (2017). Antifungal, optical, and mechanical properties of polymethylmethacrylate material incorporated with silanized zinc oxide nanoparticles. International Journal of Nanomedicine, 12(2017), 2353–2360. https://doi.org/10.2147/IJN.S132116
- Karadi, R. H., & Basima, M. A. (2017). Effect of modified nanohydroxyapatite fillers addition on some properties of heat cured acrylic denture base materials. Journal of Baghdad College of Dentistry, 29(2), 49–54. https://doi.org/10.12816/0038749
- Karthick, R., Sirisha, P., & Sankar, M. R. (2014). Mechanical and tribological properties of PMMA-sea shell based biocomposite for dental application. Procedia Materials Science, 6, 1989–2000. https://doi.org/10.1016/j.mspro.2014.07.234
- Khan, H., Raza, M., & Khan, T. A. (2020). Comparison of flexural strength of Zinc nanoparticles reinforced resin with conventional heat cure acrylic resin. Pakistan Oral & Dental Journal, 40(1), 51–54.
- Kim, K.-I., Kim, D.-A., Patel, K. D., Shin, U. S., Kim, H.-W., Lee, J.-H., & Lee, H.-H., Carbon nanotube incorporation in PMMA to prevent microbial adhesion, scientific reports, 2019, 1–11. Springer Nature
- Kuhn, K. (2005). What is bone cement? In S. Breusch & H. Malchau (Eds.), The well-cemented total hip arthroplasty: theory and practice (pp. 52–60). Springer Medizin.
- Kul, E., Aladağ, L. İ., & Yeşildal, R. (2016). Evaluation of thermal conductivity and flexural strength properties of poly (methyl methacrylate denture) base material reinforced with different fillers. The Journal of Prosthetic Dentistry, 116(5), 803–810. https://doi.org/10.1016/j.prosdent.2016.03.006
- Li, Z., Sun, J., Lan, J., & Qingguo, Q. (2014). Effect of a denture base acrylic resin containing silver nanoparticles on Candida albicans adhesion and biofilm formation. Gerodontology, 28(2), 1–8. https://doi.org/10.1111/ger.12142
- Lien, W., & Vandewalle, K. S. (2010). Physical properties of a new siloranebased restorative system. Dental Materials : Official Publication of the Academy of Dental Materials, 26(4), 337–344. https://doi.org/10.1016/j.dental.2009.12.004
- Lin, S., Cai, Q., Ji, J., Sui, G., Yu, Y., Yang, X., Ma, Q., Wei, Y., & Deng, X. (2008). Electrospun nanofiber reinforced and toughened composites through in situ nano-interface formation. Composites Science and Technology, 68(15–16), 3322–3329. https://doi.org/10.1016/j.compscitech.2008.08.033
- Mahmood, W. S. (2015). The effect of incorporating carbon nanotubes on impact, transverse strength, hardness, and roughness to high-impact denture base material. Journal of Baghdad College of Dentistry, 27(1), 96–99. https://doi.org/10.12816/0015271
- Mahross, H. Z., & Baroudi, K. (2015). Effect of silver nanoparticles incorporation on viscoelastic properties of acrylic resin denture base material. European Journal of Dentistry, 9(2), 207–212. https://doi.org/10.4103/1305-7456.156821
- Mangal, U., Kim, J.-Y., Seo, J.-Y., Kwon, J.-S., & Choi, S.-H. (2019). Novel poly (methyl methacrylate) containing nanodiamond to improve the mechanical properties and fungal resistance. Materials, 12(20)1–17. https://doi.org/10.3390/ma12203438
- Matinlinna, J. P., Lassila, L. V. J., Özcan, M., Yli-Urpo, A., & Vallittu, P. K. (2004). An introduction to silanes and their clinical applications in dentistry. The International Journal of Prosthodontics, 17(2), 155–164. PMID: 15119865
- Mohammed, D., & Mudhaffar, M. (2012). Effect of modified zirconium oxide nano-fillers addition on some properties of heat cure acrylic denture base material. Journal of Baghdad College of Dentistry, 24(4), 1–7.
- Mohammed, M. G., Ahmed, R., Ahmad, M., & Aws, S. A. (2016). Influence of incorporation of ZrO2 nanoparticles on the repair strength of polymethyl methacrylate denture bases. International Journal of Nanomedicine, 11, 5633–5643. https://doi.org/10.2147/IJN.S120054
- Monte, S. J. (2002). Neoalkoxy titanate and zirconate coupling agent additives in thermoplastics. Polymers and Polymer Composites, 10(2), 121–172. https://doi.org/10.1177/096739110201000202
- Monteiro, D. R., Gorup, L. F., Takamiya, A. S., de Camargo, E. R., Filho, A. C., & Barbosa, D. B. (2012). Silver distribution and release from an antimicrobial denture base resin containing silver colloidal nanoparticles. Journal of Prosthodontics, 21(1), 7–15. https://doi.org/10.1111/j.1532-849X.2011.00772.x
- Muklif, O. R., & Ismail, I. J. (2015). Studying the effect of addition a composite of silanized nano- Al2O3 and plasma treated polypropylene fibers on some physical and mechanical properties of heat cured PMMA denture base material. Journal of Baghdad College of Dentistry, 27(3), 22–27. https://doi.org/10.12816/0015029
- Murakami, N., Wakabayashi, N., Matsushima, R., Kishida, A., & Igarashi, Y. (2013). Effect of high-pressure polymerization on mechanical properties of PMMA denture base resin. Journal of the Mechanical Behavior of Biomedical Materials, 20, 98–104. https://doi.org/10.1016/j.jmbbm.2012.12.011
- Nagao, D., Kinoshita, T., Watanabe, A., & Konno, M. (2011). Fabrication of highly refractive, transparent BaTiO3/poly (methyl methacrylate) composite films with high permittivities. Polymer International, 60(8), 1180–1184. https://doi.org/10.1002/pi.3057
- Naji, S. A., Behroozibakhsh, M., Sadat, T., Kashi, J., Eslami, H., Masaeli, R., Mahgoli, H., Tahriri, M., Lahiji, M. G., & Rakhshan, V. (2018). Effects of incorporation of 2.5 and 5 wt% TiO2 nanotubes on fracture toughness, flexural strength, and microhardness of denture base poly methyl methacrylate (PMMA). The Journal of Advanced Prosthodontics, 10(2), 113–121. https://doi.org/10.4047/jap.2018.10.2.113
- Naji, S. A., Sadat, T., Kashi, J., Pourhajibagher, M., Behroozibakhsh, M., Masaeli, R., & Bahador, A. (2018). Evaluation of antimicrobial properties of conventional poly(methyl methacrylate) denture base resin materials containing hydrothermally synthesised anatase TiO2 nanotubes against cariogenic bacteria and candida albicans. Iranian Journal of Pharmaceutical Research, 17(2), 161–172. PMID: 31011350
- Nam, K. Y., Lee, C. H., & Lee, C. J. (2012). Antifungal and physical characteristics of modified denture base acrylic incorporated with silver nanoparticles. Gerodontology, 29(2), 413–419. https://doi.org/10.1111/j.1741–2358.2011.00489.x
- Navidfar, A., Azdast, T., & Karimzad, G. A. Influence of processing condition and carbon nanotube on mechanical properties of injection molded multi-walled carbon nanotube/poly(methyl methacrylate) nanocomposites. (2016). Journal of Applied Polymer Science, 133(31), 1–9. Article ID 43738. https://doi.org/10.1002/app.43738
- Nejatian, T., Johnson, A., & Noort, R. V. (2006). Reinforcement of denture base resin. Advances in Science and Technology, 49, 124–129. https://doi.org/10.4028/www.scientific.net/AST.49.124
- Nidal Wanis, E., Mariyam, G., Andanastuti, M., & AZHARI, C. H. (2017). Review of titanate coupling agents and their application for dental composite fabrication. Dental Materials Journal, 36(5), 539–552. https://doi.org/10.4012/dmj.2016-014
- Oei, J. D., Zhao, W. W., Chu, L., DeSilva, M. N., Ghimire, A., Rawls, H. R., & Whang, K. (2012). Antimicrobial acrylic materials with in situ generated silver nanoparticles. Journal of Biomedical Materials Research, 100(2), 409–415. https://doi.org/10.1002/jbm.b.31963
- Oyar, P., Sana, F. A., Nasseri, B., & Durkan, R. (2018). Effect of green gold nanoparticles synthesized with plant on the flexural strength of heat- polymerized acrylic resin. Nigerian Journal of Clinical Practice, 21(10), 1291–1295. https://doi.org/10.4103/njcp.njcp_388_17
- Palitsch, A., Hannig, M., Ferger, P., & Balkenhol, M. (2012). Bonding of acrylic denture teeth to MMA/PMMA and light-curing denture base materials: The role of conditioning liquids. Journal of Dentistry, 40(3), 210–221. https://doi.org/10.1016/j.jdent.2011.12.010
- Pan, Y., Fengwei, L., Xu, D., Jiang, X., Yu, H., & Zhu, M. (2013). Novel acrylic resin denture base with enhanced mechanical properties by the incorporation of PMMA-modified hydroxyapatite. Progress in Natural Science: Materials International, 23(1), 89–93. https://doi.org/10.1016/j.pnsc.2013.01.016
- Preshaw, P. M., Walls, A. W. G., Jakubovics, N. S., Moynihan, P. J., Jepson, N. J. A., & Loewy, Z. (2011). Association of removable partial denture use with oral and systemic health. Journal of Dentistry, 39(11), 711–719. https://doi.org/10.1016/j.jdent.2011.08.018
- Qasim, S. B., Al Kheraif, A., & Ramakrishaniah, R. (2012). An investigation into the impact and flexural strength of light cure denture resin reinforced with carbon nanotubes. World Applied Sciences Journal, 18(6), 808–812. https://doi.org/10.5829/idosi.wasj.2012.18.06.942
- Rebecca Lilda, G., Rupesh, P. L., Unni Pympallil, B. S., Salagundi, P. N., N. T, P., & Poonacha, V. (2020). A comparative evaluation of the impact and flexural strengths of high- impact acrylic resin reinforced with silane zirconium dioxide and titanium dioxide nanoparticles and its hybrid combination - an invitro study. International Journal of Advanced Research, 8(2), 781–791. https://doi.org/10.21474/IJAR01/10509
- Reglero Ruiz, J. A., Saiz-Arroyo, C., Dumon, M., Rodríguez-Perez, M. A., & Gonzalez, L. (2011). Production, cellular structure andthermal conductivity of microcellular (methyl methacrylate) - (butyl acrylate)-(methyl methacrylate) triblock copolymers. Polymer International, 60(1), 146–152. https://doi.org/10.1002/pi.2931
- Rongrong, C., Zhihui, H., Zhuoli, H., Junu, K. A. R. K. I., Chenxin, W., Bangshang, Z., & Xiuyin, Z. (2017). Antibacterial activity, cytotoxicity and mechanical behavior of nano- enhanced denture base resin with different kinds of inorganic antibacterial agents. Dental Materials Journal, 36(6), 693–699. https://doi.org/10.4012/dmj.2016-301
- Rubio, E., Almaral, J., Ramı́rez-Bon, R., Castano, V., & Rodrı́guez, V. (2005). Organic-inorganic hybrid coating (poly (methyl methacrylate)/monodisperse silica). Optical Materials, 27(7), 1266–1269. https://doi.org/10.1016/j.optmat.2004.11.022
- Safarabadia, M., Khansarib, N. M., & Rezaeic, A. (2014). An experimental investigation of HA/Al2O3 nanoparticles on mechanical properties of restoration materials. Engineering Solid Mechanics, 2(3), 173–182. https://doi.org/10.5267/j.esm.2014.4.006
- Safi, I. N. (2014). Evaluation the effect of nano-fillers (TiO2, AL2O3, SiO2) addition on glass transition temperature, E-moudulus and coefficient of thermal expansion of acrylic denture base material. Journal of Baghdad College of Dentistry, 26(1), 37–41. https://doi.org/10.12816/0015162
- Safi, I. N., Hassanen, K. A., & Ali, N. A. (2012). Assessment of zirconium oxide nano-fillers incorporation and silanation on impact, tensile strength and color alteration of heat polymerized acrylic resin. Journal of Baghdad College of Dentistry, 24(2), 36–42.
- Safi, I. N., & Moudhaffar, M. (2011). evaluation the effect of modified Nano filler addition on some properties of the heat cure acrylic risen denture base material. A master thesis, Department of Prosthodontics, College of Dentistry, University of Baghdad.
- Sakaguchi, R. L., & Powers, J. M. (2012). Craig’s restorative dental materials (pp. 163–176). Elsevier Health Sciences.
- Salahuddin, N., El‐Kemary, M., & Ibrahim, E. (2018). Reinforcement of polymethyl methacrylate denture base resin with ZnO nanostructures. Applied Ceramic Technology, 15(2), 448–459.
- Salih, S. I., Oleiwi, J. K., & Hamad, Q. A. (2015). Investigation of fatigue and compression strength for the PMMA reinforced by different system for denture applications. Journal of Biomedical Materials Research, 3(1), 5–13. https://doi.org/10.11648/j.ijbmr.20150301.13
- Salman, T. A., & Khalaf, H. A. (2015). The influence of adding of modified ZrO2-TiO2 nanoparticles on certain physical and mechanical properties of heat polymerized acrylic resin. Journal of Baghdad College of Dentistry, 27(3), 33–39. https://doi.org/10.12816/0015032
- Santhanam Vikram, N., & Chander, G. (2020). Effect of zinc oxide nanoparticles on the flexural strength of polymethylmethacrylate denture base resin. European Oral Research, 54(1), 31–35. https://doi.org/10.26650/eor.20200063
- Sawada, T., Sawada, T., Kumasaka, T., Hamada, N., Shibata, T., Nonami, T., & Kimoto, K. (2014). Self-cleaning effects of acrylic resin containing fluoridated apatite-coated titanium dioxide. Gerodontology, 31(1), 68–75. https://doi.org/10.1111/ger.12052
- Sehajpal, S. B., & Sood, V. K. (1989). Effect of metal fillers on some physical properties of acrylic resin. The Journal of Prosthetic Dentistry, 61(6), 746–751. https://doi.org/10.1016/S0022-3913(89)80055-1
- Shi, J.-M., Bao, Y.-Z., Huang, Z.-M., & Weng, Z.-X. (2004). Preparation of poly (methyl methacrylate)/nanometer calcium carbonate composite by in-situ emulsion polymerization. Journal of Zhejiang University. Science, 5(6), 709–713. https://doi.org/10.1631/jzus.2004.0709
- Shibata, T., Hamada, N., Kimoto, K., SAWADA, T., SAWADA, T., KUMADA, H., UMEMOTO, T., & TOYODA, M. (2007). Antifungal effect of acrylic resin containing apatite-coated TiO2 photocatalyst. Dental Materials Journal, 26(3), 437–444. https://doi.org/10.4012/dmj.26.437
- Shtilman, M. I. (2003). Polymeric Biomaterials. VSP BV.
- Suganya, S., Ahila, S. C., Kumar, B. M., & Kumar, M. V. (2014). Evaluation and comparison of anti-Candida effect of heat cure polymethylmethacrylate resin enforced with silver nanoparticles and conventional heat cure resins: an in vitro study. Indian Journal of Dental Research, 25(2), 204–207. https://doi.org/10.4103/0970-9290.135923
- Tandra, E., Wahyuningtyas, E., & Sugiatno, E. (2018). The effect of nanoparticles TiO2 on the flexural strength of acrylic resin denture plate. Padjadjaran Journal of Dentistry, 30(1), 35–40. https://doi.org/10.24198/pjd.vol30no1.16110
- Tham, W. L., Chow, W. S., & Mohd Ishak, Z. A. (2010). Simulated body fluid and water absorption effects on poly (methyl methacrylate)/hydroxyapatite denture base composites. Express Polymer Letters, 4(9), 517–528. https://doi.org/10.3144/expresspolymlett.2010.66
- Vojdani, M., Bagheri, R., & Khaledi, A. R. (2012). Effects of aluminum oxide addition on the flexural strength, surface hardness, and roughness of heat-polymerized acrylic resin. Journal of Dental Sciences, 7(3), 238–244. https://doi.org/10.1016/j.jds.2012.05.008
- Wady, A. F., Machado, A. L., Zucolotto, V., Zamperini, C. A., Berni, E., & Vergani, C. E. (2012). Evaluation of Candida albicans adhesion and biofilm formation on a denture base acrylic resin containing silver nanoparticles. Journal of Applied Microbiology, 112(6), 1163–1172. https://doi.org/10.1111/j.1365-2672.2012.05293.x
- Wang, R., Kayacan, R., & Küçükeşmen, C. (2015). Nanotubes/polymethyl methacrylate composite resins as denture base materials. In M. Zhang, R. R. Naik, & L. Dai (Eds.), Carbon nanomaterials for biomedical applications (1st ed., pp. 227–240). Springer International Publishing.
- Wang, R., Tao, J., Yu, B., & Dai, L. (2014). Characterization of multiwalled carbon nanotube-polymethyl- methacrylate composite resins as denture base materials. The Journal of Prosthetic Dentistry, 111(4), 318–326. https://doi.org/10.1016/j.prosdent.2013.07.017
- Wei, Y., Wang, X., Tang, Q., Guo, M., & Zhao, J. (2014). Reinforcement of denture base PMMA with ZrO2 nanotubes. Journal of the Mechanical Behavior of Biomedical Materials, 32, 192–197. https://doi.org/10.1016/j.jmbbm.2014.01.003
- Yadav, N. S., & Elkawash, H. (2011). Flexural strength of denture base resin reinforced with aluminum oxide and processed by different processing techniques. Journal of Advanced Medical and Dental Sciences Research, 2(1), 33–36.
- Yadav, P., Mittal, R., Sood, V. K., & Garg, R. (2012). Effect of incorporation of silane-treated silver and aluminum microparticles on strength and thermal conductivity of PMMA. Journal of Prosthodontics, 21(7), 546–551. https://doi.org/10.1111/j.1532-849X.2012.00873.x
- Yu, S. H., Lee, Y., Oh, S., Cho, H. W., Oda, Y., & Bae, J. M. (2012). Reinforcing effects of different fibers on denture base resin based on the fiber type, concentration, and combination. Dental Materials Journal, 31(6), 1039–1046. https://doi.org/10.4012/dmj.2012-020
- Zebarjad, S. M., Amir, Y., Sdrabadi, T. E., Yaghmaei, A., Naderi, B., & Naderi, B. (2011). A study on mechanical properties of PMMA/hydroxyapatite nanocomposite. Engineering, 3(8), 795–801. https://doi.org/10.4236/eng.2011.38096
- Zhang, M., & Matinlinna, J. P. (2011). E-glass fiber reinforced composites in dental applications. Silicon, 4(1), 73–78. https://doi.org/10.1007/s12633-011-9075-x
- Zhang, X., Zhang, X., Zhu, B., Lin, K., & Chang, J. (2012). Mechanical and thermal properties of denture PMMA reinforced with silanized aluminum borate whiskers. Dental Materials Journal, 31(6), 903–908. https://doi.org/10.4012/dmj.2012-016
- Zhang, X. J., Zhang, X. Y., Zhu, B. S., & Qian, C. Effect of nano ZrO2 on flexural strength and surface hardness of polymethylmethacrylate. Shanghai Kou Qiang Yi Xue. 2011; 20(4):358–363.
- Zhang, X. Y., Wu, W. L., Bian, Y. M., Zhu, B. S., & Yu, W. Q. (2009). The effect of different dispersive methods on flexural strength nano-ZrO2 reinforced denture polymethylmethacrylate. Shanghai Kou Qiang Yi Xue = Shanghai Journal of Stomatology, 18(3), 313–316.
- Zhang, X. Y., Zhang, X. J., Huang, Z. L., Zhu, B. S., & Chen, R. R. (2014). Hybrid effects of zirconia nanoparticles with aluminum borate whiskers on mechanical properties of denture base resin PMMA. Dental Materials Journal, 33(1), 141–146. https://doi.org/10.4012/dmj.2013-054