Abstract
This study aims to examine tactile sensitivity effects on the pinch force and endurance of elderly people, and propose solutions extending from the statistical outcomes using the theory of inventive problem-solving (TRIZ). Pinch force and endurance time data were collected among 32 subjects aged 55–65 years according to a non-probability sampling method. Each participant received both treatments of increased and decreased tactile sensitivity through an experimental research design. Subjects pinched an experimental apparatus with different tactile sensitivities, and sustained their pinch for as long as possible. The results suggested that a significant difference existed between pinch force from reduced and increased tactile sensitivities, with similar outcomes for endurance. Resolving contradictions from the results led to the partial-action principle, which suggested that elderly people should pinch using less force when they lack the strength to achieve a certain force level, rather than exert a high force in a single attempt. The segmentation and other-way-around principles were also recommended. Su-Field analysis found that harmful effects from pinching can be neutralised using intermediary materials between the fingers and object, such as rubber. The analysis proposed using optical or acoustic fields, where light sensors or buzzers could act as mechanisms to signal users when sufficient force is achieved. These TRIZ-stimulated solutions provide new insights in resolving poor pinch performance caused by degrading tactile sensitivity.
PUBLIC INTEREST STATEMENT
As the world’s population ages at an alarming rate, the elderly’s ability to maintain independence in physical well-being comes into question. Elderly people often suffer from a weakened grip due to arthritis or hand pain. Basic day-to-day activities like removing jar lids and unbuttoning shirts become daunting, frustrating and sometimes painful for them. While researchers have associated aging with loss of grip sensitivity and overexertion of force, this study investigates how elderly people’s pinch force and endurance change with different pinch sensitivities. The investigation outcomes are “dissected” further with TRIZ (the theory of inventive problem-solving) for the recommendation of tangible and relatable solutions to pinch grip issues among elderly people. This study not only enlightens readers with insights on TRIZ-stimulated ideas but also on how the TRIZ approach can work as a systematic basis of innovation that extends investigative outcomes to aid the elderly in pinch grips.
1. Introduction
As much as we want to stay young, age is never just a number as the human body changes every day. The United Nations define an elderly person as someone aged 65 years and above (World Health Organisation, Citation2016). With the increase of age, the pace of life starts to slow down because of the reduction in twitch tension and firing rate of motor units, which negatively affects overall muscle health (Duchateau & Hainaut, Citation1990).
As of the end of 2020, a total of 3.5 million (10.7%) Malaysians reached the age of 60 (Department of Statistics Malaysia, Citation2017). This figure is concerning because elderlies tend to be less healthy than the younger population. They become more vulnerable to physical disabilities and mental illnesses (Kostopoulos et al., Citation2018). Some of the significant health issues they face occur in their hands and fingers. For example, they experience weakness in pinching and gripping objects, which can even lead to carpal tunnel syndrome (CTS). Osteoarthritis is also a common disease among elderlies which frequently impairs hand function (Zhang et al., Citation2002).
In a longitudinal investigation of musculoskeletal impairments and their physical disabilities, findings confirmed that physical disabilities are significantly caused by musculoskeletal degradation (Jover et al., Citation2015). There are recorded examples of poor hand functions in particularly basic activities of daily living. For instance, it was found that many elderlies have trouble opening or unscrewing the caps of bottles, especially those from medicine containers (Rahman et al., Citation2002; Rice et al., Citation1998). In association to this issue, one of the common day-to-day tasks that elderly people struggle with includes pinching.
Pinching is the action of griping something between the thumb and fingers without involving the palm. A few commonly known pinch techniques include the tip pinch, lateral pinch and three-jaw-chuck pinch (Ellis et al., Citation2004; Shih & Ou, Citation2005). It is important to understand the different pinching mechanisms and techniques. Although people usually pinch with techniques based on their experience and familiarity, the pinch force exertion is not comparable across different techniques (Ng et al., Citation2014). A study by Heffernan and Freivalds (Citation2000) found that the optimum pinch technique for die handlers was a combination of a small pinch span (1.27–3.81 cm) and a 45° inward slant. There have been studies on different aspects of finger pinching, including a study on the relationship between grip force and grip stiffness (Höppner et al., Citation2013). When the grip kinematics and force transfer function from muscle to finger are changed, one can actively change the increase in grip stiffness with force using wrist flexion and thus change the grip stability. However, precautions should be taken as all deviating wrist positions were found to degrade pinch strength (Bhardwaj et al., Citation2011). Among all components, finger strength is one of the major components often measured among elderly patients with stroke (Canning et al., Citation2004; Harris & Eng, Citation2007).
1.1. Problem statement
Several studies have looked into the variations in handgrip force and endurance among elderly people (Desrosiers et al., Citation1997; Massy-Westropp et al., Citation2011; Nilsen et al., Citation2012; Nurul Shahida et al., Citation2015; Wallström & Nordenskiöld, Citation2001) in addition to some studies on hand and pinch strengths among the elderly (Bae et al., Citation2015; Shin et al., Citation2012; Lam et al., Citation2015; Wu et al., Citation2015). Researchers have also looked into the effects of aging on touch (Wickremaratchi & Llewelyn, Citation2006), and a possibility in the reduction of grip capacity in cases of reduced tactile sensitivity such as numbness, glove use, or sensory deficit (Enders & Jin Seo, Citation2011).
However, there appear to be few studies that examine reduced and increased tactile sensitivity effects on the pinch force and pinch endurance of elderly people. Furthermore, such studies are often statistical in nature, and provide inferences on various effects to enhance the body of knowledge concerning pinch grips. There has yet to be a research that uses an algorithmic problem-solving approach such as the theory of inventive problem-solving (TRIZ) to systematically extend the statistical outcomes of such studies into tangible ideas that address pinch grip issues among elderly people. Thus, the following research questions can be proposed.
RQ1: What are the effects of reduced and increased tactile sensitivities on the elderly’s pinch force and pinch endurance?
RQ2: How can TRIZ systematically extend the statistical outcomes on pinch force and pinch endurance into tangible solutions that address pinch grip issues among the elderly?
Hence, this study aims to not only uncover the effects of tactile sensitivity on the pinch force and endurance among elderly people but also look into how TRIZ can be used to potentially resolve the issues that arise from the outcomes of the statistical inferences.
Pinch force and endurance time are selected as output variables for this study. Endurance time is measured using a stopwatch, while pinch force is measured using flexiforce pressure sensors. The data are analysed using two-sample variance and two-sample t-tests, and the TRIZ tools and techniques involved in this study include engineering contradiction, physical contradiction, substance field modelling, 40 inventive principles and 76 standard inventive solutions.
1.2. Literature review
The change in aging muscle can come in two forms, namely forms that are normal (age-related sarcopenia) and abnormal (cancer-related anorexia, sarcopenic obesity, sarcopenia in cirrhosis, sarcopenia in type 2 diabetes). Sarcopenia is the age-related decrease in lean muscle mass (Evans, Citation2010; Siparsky et al., Citation2014). This phenomenon changes a person’s activeness and reduces his/her quality of life. There are three stages of sarcopenia in old people as defined by the European Working Group on Sarcopenia in Older People (EWGSOP) in 2010 (Mitchell et al., Citation2012). Pre-sarcopenia is only the loss of muscle mass. Sarcopenia includes muscle loss that occurs together with reduced strength or physical performance, while severe sarcopenia entails muscle loss together with loss of strength and physical performance.
Muscle mass directly corresponds to age and changes accordingly as shown in . Generally, people would experience increased muscle mass in their youth, maintained muscle mass during midlife and decreased muscle mass in later years (Dodds et al., Citation2014). Quantifiably speaking, after the age of 50 years, people would begin to lose an approximated 1–2 % of their muscle mass each year (Keller & Engelhardt, Citation2014; Marcell, Citation2003). Consequently, the probability of sarcopenia increases.
Figure 1. Changes in muscle mass and strength with respect to age (Sayer et al., Citation2008).
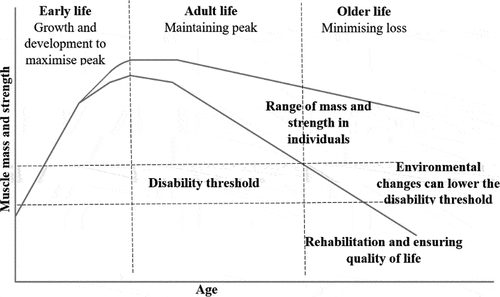
Sarcopenia is also significantly affected by the metabolic changes of aging (Evans, Citation2010). These changes come in the form of increased insulin resistance and higher body fat percentage, resulting in an elderly person’s decrease in muscle turnover and repair abilities. Besides chronic inflammation, factors such as lower physical activeness, hormone excretion and nutrition contribute to the complex changes in the aging body, which play a role in the decrease of the body’s muscle mass (Bainbridge et al., Citation2011).
The human skeletal muscle has been described with the term “plasticity” because of the malleability of muscle fibres (Pette, Citation2007). Plasticity is a common term in neurophysiology, while in the context of muscle physiology, the terminally differentiated muscle fibre is known as a versatile entity rather than a fixed unit. This feature of the muscle is advantageous for the elderlies as with training, it can adapt to demands throughout the human life span. In other studies, Heath et al. (Citation1981) and Seals et al. (Citation1984) found that with training, adults of ages between 60 and 80 can experience a 20–30 % increase in aerobic fitness. Central cardiovascular strengthening and peripheral muscle adaptation are causes for the aerobic fitness improvements. Therefore, it is vital that elderlies continue to remain active to continually drive muscles. The importance of keeping the fingers active with different types of movements is further emphasised in a study on midlife female dentists whereby dentists with low variations in work task history are at a higher risk of possessing a low pinch grip strength (Ding et al., Citation2013).
Type I and type II fibres are age-related changes in muscle fibre distribution. Type I fibres are highly resistant to fatigue and capable of metabolising fat for energy expenditure while type II fibres contract faster and produce a large tension output but fatigue quickly. Physically, type I fibres are small, slow-contracting and low-tension output fibres, while type II fibres are large fibres. Although the exact numbers are unknown, it was found that aging leads to an increased percentage of type I fibres compared to type II (Frontera et al., Citation2000). The lesser number of type II fibres could possibly be the reason for muscle strength decrease as people age (Paillard, Citation2017).
According to researchers, the increase of type I fibres could be due to motor nerves having a trophic influence on muscle fibres (Mantilla & Sieck, Citation2008). The most probable explanation to this occurrence would be the reorganisation of aging motor units. Initially, when the number of active motor units decrease, the remaining motor unit pool increases in size (Brown, Citation1972). This increase in size of active motor units is possibly due to new collaterals from motoneurons in the type I pool branching out to non-functioning fibres. Higher tension type II fibres will contribute less to tension output as low tension type I fibres would be predominant. In summary, elderlies would have smaller and weaker muscle mass due to the loss of type II fibres (Kirkendall & Garrett, Citation1998; Paillard, Citation2017).
Tactile sensory feedback is essential for motion, temperature and force sensing (Badawy & Alfred, Citation2020). Elderly people often have a diminished tactile sensitivity and decreased hand dexterity. These issues could lead to the act of over-gripping objects during activities of daily living and cause hand-related injuries. The preceding predicament begs the need for researchers to conduct more studies on anthropometry and ergonomic risk factors in order to design special devices for elderly people (Syed Naqvi, Citation1993). In a certain study, elderly and young adults were requested to use a pulp pinch (a pinch technique that uses the thumb and index finger) to grip a small object with varying slipperiness. The study concluded that old people on average employ twice as much force as young people when they pinch. This excessive force is a response to poor tactile sensitivity (Cole, Citation1991). For this study, the pulp pinch was selected as the type of pinch technique to be experimented on according to supports from previous studies (Cole, Citation1991; Li, Wei, Yue et al., Citation2016a, Citation2016b. It was also suggested that the pulp pinch is one of the more commonly used dual finger pinching techniques with regard to maximum strength applications for small objects (Nilsen et al., Citation2012).
Besides friction, tactile sensitivity allows individuals to detect other physical signals such as force, temperature, vibration and electricity (Tomimoto, Citation2011). The fingerprints also function as restriction for slipping occurrences and support for optimum friction. The friction coefficient can change depending on environmental conditions such as the humidity of the surroundings and sliding surface (Hyun-Yong et al., Citation1996).
2. Materials and methods
The targeted test subjects for the experiment were elderlies above the age of 55. Participants above the age of 55 years, who were physically healthy with no heart failure, neurological diseases, joint injuries, muscle diseases or injuries of the fingers, hands and arms were eligible to participate in this study.
2.1. Sampling
The experimental research design followed a non-probability sampling method. A total of 32 subjects were recruited for this experiment as 30 samples are often the recommended minimum size for preliminary studies (Serdar et al., Citation2021). Subjects between the ages of 55–65 years old were randomly selected based on a set of exclusion criteria for the experiment which was conducted in a span of 20 days (from 8 to 27 October 2019). These criteria included (a) experiencing acute pain in the right hand and fingers or having any form of hand-related injuries, (b) having medical conditions such as high blood pressure, post-stroke conditions or hospitalised during the last 6 months, and (c) unable to live an active lifestyle.
Besides these criteria, an equal amount of elderlies with frequent and infrequent hand usages were selected. The same balance in gender was also ensured during the participant selection. In order to replicate pinching action, an experimental apparatus was fabricated as shown in (A). Subjects were required to wear gloves with sensors attached to their fingertips as shown in (D). (B) shows a participant pinching a binder clip mounted on the experimental apparatus using the experimental gloves.
Figure 2. Apparatus used in strength and endurance pinch testing. (a) Test apparatus with cylindrical palm rest and adjustable pinching position. (b) Finger pinch test on binder clip. (c) Stop watch measuring pinch duration. (d) Experiment gloves with Tekscan Grip System.
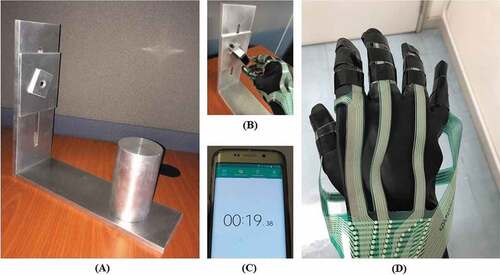
All participants gave their written informed consent prior to the experiments, in which all procedures and protocols were approved by the Research Ethics Committee (REC) of the Technology Transfer Office (TTO), Multimedia University Malaysia. The research ethics approval for the project was granted with the approval ID EA0032019, and the approval letter was endorsed by the TTO Director cum REC Secretariat of the university.
2.2. Measurement
The experiment was conducted to collect data on pinch force and endurance time. In order to obtain results for pinch force, subjects were required to pinch the apparatus at their maximum limit. The maximum pinch force was recorded using the I-Scan software. When a flexiforce sensor is paired together with the I-Scan software, the system is expected to have an accuracy of ± 3% (Tekscan, Citation2020). For the endurance time, subjects were asked to sustain their pinching activity for as long as possible (Li et al., Citation2015, Citation2013). From (C), endurance time was recorded using a stopwatch. The tactile sensitivity in this experiment was controlled by using different types of gloves. Cotton gloves were used for reduced tactile sensitivity while rubber gloves were used for increased tactile sensitivity. It is important to note that the authors had no access to information that could possibly identify the individual participants during or after the data collection.
2.3. Data analysis
For both sets of data (pinch force and pinch endurance time), a two-sample variance test and t-test were performed. The two-sample variance test was performed to test if a significant difference in the variance existed. The common reason to compare variance is a check for homogeneity of variance before further tests like ANOVA or t-tests are performed (Hosken et al., Citation2018). Using Minitab version 16, the assumption of homogeneity of variance was done with Levene’s test and F-test. The Levene’s test applies to any continuous distribution while the F-test is based on the normal distribution. If equal variances are assumed (null hypothesis supported, and alternative hypothesis rejected), there is a better possibility of minimising the Type I error, which is the rejection of a true null hypothesis (known as a “false positive” finding).
With the assumption of homogeneity in variance, the pooled estimate for the error term for the t-statistic can be used. The t-test was used as it is more accurate than a corresponding normal test and is just as powerful (Liu & Sun, Citation2019). The t-test can determine if a significant difference between the means of two groups existed. Assuming the dependent variable fits a normal distribution, the probability of a particular outcome becomes identifiable. The statistical t-test results would assist the researchers in identifying contradictions related to the problem in order to continue with the problem-solving approach using TRIZ methodology.
2.4. Further analysis using the TRIZ methodology
TRIZ is a Russian Acronym which stands for the theory of inventive problem-solving. It is an algorithmic problem-solving technique which uses logic rather than intuition to stimulate the aptitude in solving problems innovatively (Yeoh et al., Citation2015). TRIZ follows a systematic process that is highly reliable, predictable and repeatable (Yeoh, Citation2014). Furthermore, TRIZ is applicable across all fields of study and can address all sorts of problems related to technology, engineering, business, social science, arts, culture and philosophy (Souchkov et al., Citation2007). shows the TRIZ process used in this study.
With the statistical information, further analysis using the TRIZ methodology can be conducted. This systematic approach would allow for the formulation of TRIZ engineering contradictions, physical contradictions and substance field models, and the generation of TRIZ principles which support the conceptualisation process.
3. Results and discussion
3.1. Two-sample variance test for pinch force
For this study, the hypotheses proposed for the two-sample variance test are as such:
H01a: There is no significant difference in the elderly individual’s pinch force variance under the conditions of reduced and increased tactile sensitivities.
H1a: There is a significant difference in the elderly individual’s pinch force variance under the conditions of reduced and increased tactile sensitivities.
H01a refers to the null hypothesis, while H1a refers to the alternative hypothesis. The results of the two-sample variance tests for the elderly individual’s pinch force with differing sensitivities are shown in . The results show that for both test methods (F-test and Levene’s test), there is no significant difference in the elderly individual’s pinch force variance with reduced and increased tactile sensitivities (p > 0.05). Therefore, H01a is supported and H1a is rejected, and the variance is assumed to be equal. Further analysis on the data would require the assumption of equal variance to be considered.
Table 1. Two-sample variance tests for the elderly individual’s pinch force variance under the conditions of reduced and increased tactile sensitivities
3.2. Two-sample t-test for the mean pinch force
In Minitab version 16, checking the “assume equal variances” checkbox was needed when conducting the two-sample t-test. The hypotheses proposed for the two-sample t-test are as such
H01b: There is no significant difference in the elderly individual’s mean pinch force under the conditions of reduced and increased tactile sensitivities.
H1b: There is a significant difference in the elderly individual’s mean pinch force under the conditions of reduced and increased tactile sensitivities.
H01b refers to the null hypothesis, while H1b refers to the alternative hypothesis. The results of the two-sample t-test for the elderly individual’s mean pinch force under the conditions of reduced and increased tactile sensitivities are shown in . The results suggest that there is enough evidence for a significant difference to exist in the elderly individual’s mean pinch force generated under the conditions of reduced and increased tactile sensitivities (p < 0.05). Hence, H01b is rejected, and H1b is supported.
Table 2. Two-sample t-test for elderly individual’s mean pinch force under the conditions of reduced and increased tactile sensitivities
3.3. Two-sample variance test for endurance time
A two-sample variance test is performed in order to see if a significant difference existed in the endurance time variance for elderly individuals under the conditions of reduced and increased tactile sensitivities. For this study, the hypotheses proposed for the two-sample variance test are as such:
H02a: There is no significant difference in the elderly individual’s endurance time variance under the conditions of reduced and increased tactile sensitivities.
H2a: There is a significant difference in the elderly individual’s endurance time variance under the conditions of reduced and increased tactile sensitivities.
H02a refers to the null hypothesis, while H2a refers to the alternative hypothesis. The results of the two-sample variance tests for this section are shown in . For Levene’s test, it was found that there is no significant difference in the elderly individual’s endurance time variance under the conditions of reduced and increased tactile sensitivities (p > 0.05). However, the F-test method revealed that a significant difference existed in the elderly individual’s endurance time variance under the conditions of reduced and increased tactile sensitivity (p < 0.05). Since one of the test methods suggested that there is a possible heterogeneity in variance, it would be safer to assume that the variance in this case is unequal. With this assumption, the F-test results are accepted. Therefore, H02a is rejected and H2a is supported. Further analysis on the data would require the assumption of unequal variance to be considered.
Table 3. Two-sample variance tests for the elderly individual’s pinch endurance time variance under the conditions of reduced and increased tactile sensitivities
3.4. Two-sample t-test for the mean endurance time
The violation in the assumption of homogeneity of variance can be overcome by not using the pooled estimate for the error term for the t-statistic. Instead, an adjustment to the degrees of freedom is used with the Welch–Satterthwaite method. In order to perform this analysis in Minitab version 16, the researchers would just need to uncheck the “assume equal variances” checkbox when conducting the two-sample t-test. The hypotheses proposed for the two-sample t-test is as such:
H02b: There is no significant difference in the elderly individual’s mean endurance time under the conditions of reduced and increased tactile sensitivities.
H2b: There is a significant difference in the elderly individual’s mean endurance time under the conditions of reduced and increased tactile sensitivities.
H02b refers to the null hypothesis, while H2b refers to the alternative hypothesis. The results of the two-sample t-test for the elderly individual’s mean endurance time are shown in . The results suggest that there is enough evidence for a significant difference to exist in the elderly individual’s mean endurance time under the conditions of reduced and increased tactile sensitivities (p < 0.05). Hence, H02b is rejected, and H2b is supported.
Table 4. Two-sample t-test for the elderly individual’s mean endurance time under the conditions of reduced and increased tactile sensitivities
From the pinch force results, participants on average were found to apply less force when pinching with increased tactile sensitivity. With increased tactile sensitivity, elderly people are able to pinch objects securely. Hence, they tend to relax the force application to prevent the overexertion of finger muscles. Pinch endurance on the other hand was found to have a slightly lower mean result in measurements using the rubber gloves (increased tactile sensitivity), which differ from findings on the increase in finger endurance when friction is increased (Amca et al., Citation2012; Fuss & Niegl, Citation2012).
Pinch endurance in this experiment was quantified through the total pinching time of a binder clip. The subjects would have to overcome the potential energy in the clip and sustain it over a period of time. During this period, it was possible that the increased tactile sensitivity allowed for a more efficient pinch, thus enabling the elderly to open the clip with less effort. With the required pinch force reduced, it was likely that the elderly sustained the pinch throughout the testing period with their same initial pinch force which was lower than the ramped-up initial force applied using the cotton gloves. The shorter endurance time may have been caused by the lower overall effort applied by the elderly person. This lesser overall effort with lower pinch force may have hindered the elderly subjects in keeping the clip open for a longer period of time. However, more psychophysical data and observations might be required to verify this conjecture.
3.5. Discussion on RQ1: reduced and increased tactile sensitivity effects
The results confirmed that a change in tactile sensitivity significantly affected the pinch force and pinch endurance of the elderly (p < 0.05). Upon further investigation, it was found that the mean pinch force generated from reduced tactile sensitivity was higher compared to the one generated from increased tactile sensitivity (Pinch force for reduced tactile sensitivity = 242.6 N, Pinch force for increased tactile sensitivity = 211.6 N). This finding suggests that the overall pinching force of the elderly population is higher with lower tactile sensitivity as compared to that of with higher tactile sensitivity.
Additional observations revealed that the mean endurance time to which the elderly people used to sustain their pinch under the condition of reduced tactile sensitivity was higher compared to the one under the condition of increased tactile sensitivity (Endurance time for reduced tactile sensitivity = 2.425 s, Endurance time for increased tactile sensitivity = 1.808 s). This finding suggests that the pinching endurance of the elderly population under reduced tactile sensitivity conditions is higher as compared to that of increased tactile sensitivity conditions. Endurance in this study is the ability to sustain the pulp pinch for as long as possible. The overestimation of endurance time might be related to an inaccurate estimation of muscle fatigue. Muscle fatigue was found to affect the coordination angle and force control of fingers (Hu et al., Citation2018). This factor along with tactile feedback may have influenced the endurance ability of elderly people.
3.6. Engineering contradiction
An engineering contradiction is a situation, in which an attempt to improve one parameter of a system leads to the worsening of another parameter. It has the following structure:
If … (manipulative variable changes)
Then … (responding variable #1 improves)
But … (responding variable #2 worsens)
Using the TRIZ method, an engineering contradiction from the experimental results can be constructed as such:
If the elderly person pinched with reduced tactile sensitivity,
Then they would tend to hold their pinch tightly for a longer duration,
But they tend to overexert their pinch grip.
After establishing the engineering contradiction, proposed recommendations can be filtered out through the identification of the appropriate TRIZ inventive principles. The TRIZ inventive principles are simple ways to resolve engineering contradictions. There are 40 TRIZ inventive principles altogether. The inventive principles can be extracted from the contradiction matrix by first identifying the appropriate TRIZ system parameters linked to the engineering contradictions. There is a total of 39 TRIZ system parameters.
present the system parameters and inventive principles for the engineering contradiction. The possible outcome of this study could be to potentially design an ergonomic device that assists its user in performing a longer and more sustainable pinch grip without overexerting the fingers. With this outcome in mind, the inventive principle of partial action was selected.
Table 5. System parameters for engineering contradiction
Table 6. Inventive principles from system parameters of engineering contradiction
3.7. Physical contradiction
A physical contradiction involves two opposing requirements on a single object (Ko et al., Citation2016). These requirements cause conflict in the functionality of that particular object. It is also an engineering system that requires conflicting properties from the same parameter. The steps to resolve a physical contradiction are listed as such:
1.Construct two opposing requirements for one parameter of a system.
2.Determine the correct strategy to resolve the contradiction.
3.Identify the most suitable inventive principles which will lead to ideas that solve the problem.
For this study, the physical contradiction can be formulated as such:
Elderlies need to pinch with reduced tactile sensitivity in order to hold their pinch tightly for a longer duration, AND elderlies need to pinch with increased tactile sensitivity in order to not overexert their pinch grip.
The strategy used to resolve the physical contradiction is known as separation in space. This strategy separates the operating space to pinch tightly and the operating space to avoid overexertion. These parameters are to be fulfilled at the same time but can be at different spaces. Based on the 40 inventive principles, the inventive principles used for the separation-in-space strategy include:
#1 Segmentation
#2 Taking out
#3 Local quality
#17 Another dimension
#13 The other way around
#14 Curvature
#7 Nested doll
#30 Flexible shells or thin films
#4 Asymmetry
#24 Intermediary
#26 Copying
The segmentation and other-way-around principles were selected for further deliberation on possible recommended solutions in resolving the physical contradiction.
3.8. Substance-field analysis
The substance-field (Su-Field) analysis is another TRIZ problem-solving tool. It is used to analyse and improve the efficacy of technical systems. The most important phase of the Su-Field analysis is the last step which is to manually implement the solution to a system (Yan et al., Citation2013). The Su-Field normally comprises Mechanical, Acoustic, Thermal, Chemical, Electric and Magnetic (MATCHEM) fields. A field makes up the most basic model of an interaction. Any interaction can be formulated as the interaction between two substances. The statistical findings and TRIZ contradictions of this study suggested that elderlies with poor tactile sensitivity in their fingers tend to pinch more forcefully, causing stress to their fingers. Using the Su-Field analysis, the interactions between the fingers and pinched object can be modelled. The type of model identified would determine the class of standard inventive solutions (from the 76 TRIZ standard inventive solutions) to be used for the particular modelled problem.
presents the Su-Field model of this study. S1 refers to the first substance which represents fingers. S2 represents the pinched object. F1 is the mechanical field which includes the interaction between S1 and S2. This model is known as a harmful Su-Field model, which is normally represented by a curly arrow from S1 to S2. The model implies that the fingers are harmfully pinching the object. From the list of 76 standard inventive solutions, class 1.2 is used as a reference to resolve the problem. This class includes various methods of eliminating or neutralising harmful effects. Sub-classes 1.2.1 and 1.2.4 were finally chosen as the solution models.
3.9. Discussion on RQ2: TRIZ extends statistical outcomes into tangible solutions
3.9.1. Engineering contradiction—partial action
Although grip force may be highly suboptimal, it can benefit the elderlies (Shim et al., Citation2004). A higher grip force would prevent objects from leaving the hand even if load force changes. These forces could be accelerations in a vertical direction or changes in the grip force. Among less steady elderlies, occurrences like these can be expected (Burnett et al., Citation2000; Enoka et al., Citation2003). Elderlies were found to produce an average grip force that was twice as large as the grip force produced by young people during a precision pinch grip experiment (Cole, Citation1991). This excess grip force represents a strategic response to tactile sensitivity impairment which also contributes to impaired dexterity.
Excess force produces a stronger pinch grip yet places more stress on finger muscles, and can cause long-term injuries. With partial action, an appropriate strategy would be to assist elderlies in ensuring that they do not overexert their fingers in a pinching application. This strategy can be executed by pinching using less of the originally desired force when the exact intended force level is difficult to achieve, rather than exerting a high pinch force in a single attempt. In fact, research found that elderlies often utilise a probing pinch approach which causes a larger force fluctuation (Enoka et al., Citation2003). This fluctuation is a natural response to the inability of elderlies to ramp up finger force. Increasing friction would naturally cause people to grip more securely, which then allows for better pinch force control. Another more unconventional method would be to explore the use of dampers which can absorb the extra force without compensating strength and firmness of the pinch.
3.9.2. Physical contradiction
Segmentation. Besides finger strength, finger individuation is an important component of hand function. Finger individuation is the ability to discreetly manipulate fingers. Studies have shown that stroke patients were unable to produce independent finger force and unable to synchronise their multi-finger force (Kim et al., Citation2014). Independent finger movements were found to be restricted as the motor cortex or corticospinal tract worsened (Lang & Schieber, Citation2003; Schieber et al., Citation2009). According to the aforesaid research, this issue was particularly obvious for the middle, ring and little finger as these fingers were found to be substantially impaired. While finger individuation was found to be severely impaired among stroke patients, the general elderlies are not spared from this predicament. Researchers found that the deep long flexors of fingers were unable to selectively contract, hence limiting independency (Kilbreath & Gandevia, Citation1994). In a study among healthy adults, mechanical coupling was a major cause of finger independency reduction especially in the index, middle and ring fingers (Lang & Schieber, Citation2004).
When gripping small objects, one must have the individuation ability to position fingers in the correct orientation and exert enough force to grip the object (Wolbrecht et al., Citation2018). This understanding of how each finger changes differently with respect to age should change the method of designing a finger pinching assistant tool. Instead of studying pinching as a single finger’s action, segmentation suggests that breaking the action down to smaller components of individual fingers would be more appropriate as supported by past research (Kilbreath & Gandevia, Citation1994; Lang & Schieber, Citation2004). With the use of this principle in the design of a pinch enhancing tool, more emphasis should be given to weaker fingers like the middle, ring and little fingers in order to compensate for the severe impaired individuation experienced.
The other way around. The elderlies were found to produce a higher antagonistic moment which acts against the direction of total moment (Shim et al., Citation2004). Similar to the produced excess force, this action can be viewed as an inefficient griping method, though it increases stability. Antagonist moments increase the apparent stiffness of the hand and can be a passive resistance to variations in torque. In other studies, researchers found that the central nervous system was unable to organise finger synergies such that finger forces tend to cancel each other out (Latash et al., Citation2001, Citation2002). Researchers concluded that the central nervous system requires some time to organise the stabilisation in the finger force of negative covariation (Shim et al., Citation2003). During prehensile tasks, fingers pre-produce a nonzero force which acts against external torque (Shim et al., Citation2004). This situation occurs instinctively to increase the safety margins of elderlies as they use their fingers.
Using the other-way-around principle, it could be beneficial to view antagonist moments from another perspective. Instead of trying to eliminate these natural age-related responses of the fingers, factoring them into future studies or medical devices would enable people to age gracefully as their bodies intend. A device utilising the other-way-around principle in this case would pay attention to the preloading force of the elderly’s fingers. Instead of designing something that restricts initial force application entirely, the device should allow fingers to apply preloading force first before assisting it with mechanisms. This device would also allow older individuals to remain active as a countermeasure for expected loss of strength and endurance. Elderlies often experience decreased walking speed, sit-to-stand transition time, frequent falls and worsening balance as physical strength decreases. However, a study among nonagenarians with resistance training showed an improved strength of up to 175 % and improvements in functional mobility (Fiatarone et al., Citation1990). It is never too late to start being active as researchers found that for many patients, age-related effects on skeletal muscles are still reversible (Fiatarone & Evans, Citation1993).
3.9.3. Substance-field analysis
Sub-class 1.2.1: Removal of harmful effect by introducing S3. shows a chain Su-Field model, which is a modified version of the previous harmful Su-Field by adding another field (F2) and substance (S3). S3 is added to separate substances S1 and S2. It should be a substance that can potentially reduce the harm on the fingers while pinching. In this scenario, S3 can include adding friction between the fingers and pinched object. This proposition, which can take the form of rubber pads or gloves, improves tactile sensitivity, leading to a plausible increase in force accuracy and reduced overexertion. F2 in the diagram remains as a mechanical field.
For the elderlies, the mechanical action of pinching causes a harmful effect. As people age, the tactile sensitivity of hands becomes impaired (Cole, Citation1991; Ranganathan et al., Citation2001; Rantanen et al., Citation1999). Because of this impairment, elderlies are unable to pinch with the accurate amount of force and often overexert their fingers to secure a stable grip. This action harms the fingers and causes long-term injuries. Adding a new substance between the finger and object could potentially eliminate this effect.
Rubber gloves are popular tools used for increasing friction between the fingers for any hand-holding application. In various industries such as sports, culinary arts and manufacturing, rubber gloves can significantly improve the pinching ability by increasing contact friction. Elderlies could also benefit from pinching with extra friction. This idea can be incorporated into devices for activities of daily living. One example includes the NuMuv Grip-Aid as shown in . The NuMuv Grip-Aid is a device that aids people with grasping issues by providing a larger grasp surface for the user to work with (Obmaces, Citation2015). This soft, egg-shaped holder works easily with toothbrushes, eating utensils, pencils and other objects. A study in 2015 discovered a progressive increase in grip-to-load force during the precision pinch of low-mass objects (less than 30 g) (Hiramatsu et al., Citation2015). The same study discussed the possibility of how a decreased level of tactile feedback plays a role in inefficient low-mass grip force control. With reference to the analogy of this invention, a similar tool can be introduced for the elderly to increase finger friction and reduce the harmful effects caused by slippery pinching.
Figure 6. NuMuv grip-aid, a device for hand mobility issues (Obmaces, Citation2015).
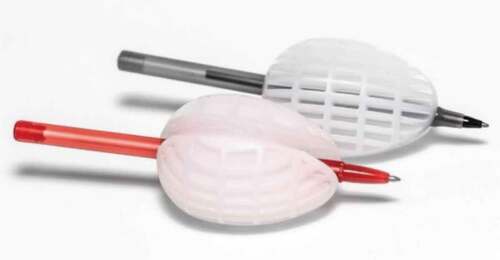
Sub-class 1.2.4: Counteracting the harmful effect of F1 by neutralising it with F2. presents a double Su-Field model, which is a modified version of the original harmful Su-Field by adding another field (F2) to neutralise the harmful effects of F1. The mechanical field from F1 produced by the fingers pinching the object generates harmful effects. These effects can be negated by adding F2. Some of the possible fields could include the optic or acoustic field. This solution model does not separate S1 and S2 and ensures that the fingers remain in contact with the pinched object. As people age, eyesight begins to deteriorate. Poor eyesight has a strong correlation with poor finger pinching (Lin et al., Citation2019; Ribeiro & Oliveira, Citation2007; Shumway-Cook & Woollacott, Citation2007). The inability to clearly gauge objects would lead to the inability to properly apply the appropriate force. The incorporation of LED sensors on a device that aids pinching would provide a clear signal to prompt the user once sufficient force is detected for the pinching action.
It is important to consider other forms of mediums where information on pinching can be easily processed by elderlies. Auditory feedback can be one of the possible substitutes to visual sensors (Levy-Tzedek et al., Citation2012; Portnoy et al., Citation2015). With sufficient practice, auditory feedback could assist day-to-day activities and prevent household accidents. Auditory feedback, like an alarm or buzzer, can assist by providing a response on the pinch force to elderlies. A pincer chopstick developed by Chang et al. (Citation2007) is an example of a substituting device for people with impaired hands. Without any prior experience, users with this pincer chopstick on their non-dominant hand performed better as compared to when using traditional chopsticks. This idea ensures that elderlies pinch with the adequate amount of force to prevent injuries. Besides that, it also prevents the elderly from sustaining the high pinch force for an extended duration, and prevents unnecessary holding postures which can cause muscle fatigue and cumulative trauma disorders such as CTS (Syed Naqvi, Citation1996). summarises all the the TRIZ tools with the selected principles or sub-classes and recommended solutions.
Table 7. The TRIZ tools with their principles/sub-classes and recommended solution
4. Conclusion
This study successfully addressed the first research question (RQ1) by evidencing that the effects of reduced and increased tactile sensitivities on the elderly’s pinch force and pinch endurance are significant. In contrast to increased tactile sensitivity, elderly people pinched using about 15% more force and sustained the pinch using 34% more endurance time with reduced tactile sensitivity. This finding confirmed that elderlies with poor tactile sensitivity in their fingers commonly pinched objects with excessive force and extended durations. Such acts could harm their fingers if not assisted with the right tools or devices. Hence, a device that can intelligently assist elderlies in pinching objects with predictive and accurate stress detection abilities could be developed and incorporated into various products and designs.
This study also addressed the second research question (RQ2) by demonstrating the use of TRIZ in systematically extending the statistical outcomes from RQ1 into tangible solutions that address pinch grip issues among the elderly. The engineering contradiction, physical contradiction and Su-Field modelling techniques led to the proposal of creative solutions through specific inventive principles. Some of these solutions such as the inclusion of audio sensors and utilisation of preloading in finger strength can be considered as recommendations in resolving pinch performance problems due to the lack of tactile sensitivity in fingers.
With aging being the main cause of sarcopenia, elderly people are at high risk of facing degradation in hand functions, and this problem could severely affect their quality of life. The silver lining for elderlies would be that with proper exercise, muscles can get stronger and functional enough for them to prevent or prolong the degenerative loss of skeletal muscle mass. However, a major gap still exists in the development of assistive tools that can support the hand functions of elderly people who are already suffering from sarcopenia or any other frailty syndrome.
Despite the findings of this study, more experiments and tests are required to filter and finally design a tool with real value to elderlies with regard to pinching activities. Nonetheless, this study still provides researchers with preliminary and mechanistic insights on design directions, ideas and concepts that can be undertaken to resolve pinch grip issues among the elderly. The success of producing such designs could disentangle the problems in accommodating the use of fragile fingers and deteriorating muscles among elderly people and provide avenues in resolving more issues related to pinch grips.
4.1. Limitation
In this study, both pinch force and pinch endurance have been used to quantify the pinching abilities of elderly people when tactile sensitivity is manipulated. Although these are both proven and reliable parameters, the inclusion of finger dexterity in the experiment would have been beneficial for the analysis. Manual dexterity correlates with aging and affects the independence of elderlies. The complexity of dexterity measurements which are prone to adaptive strategies would require more thought-out experimental procedures.
4.2. Recommendation for future research
Based on the research outcomes and recommended solutions from the TRIZ methodology, this study can be extended to the product design of a novel pinch assistive device for elderlies especially during applications of reduced tactile sensitivity. Such a device could supplement the pinch performance of elderlies by
•increasing the contact friction between fingers and pinch objects
•isolating each finger to identify particular weaknesses in finger digits
In essence, this study draws the attention of researchers to some initial directions for the design of a suitable assistive device.
Data accessibility
The experimental data have been deposited in the Figshare data repository, which can be found at: https://doi.org/10.6084/m9.figshare.9805274.v1
Author’s declaration
There is no conflict of interest among the authors.
Correction
This article has been corrected with minor changes. These changes do not impact the academic content of the article.
Acknowledgements
This research article is in partial fulfilment of the requirements for the Master of Engineering Science degree at the Faculty of Engineering and Technology, Multimedia University. The researchers gratefully thank the faculty and university for their support in allowing this research to be carried out. The researchers also thank the Technology Transfer Office (formerly known as the Collaboration and Innovation Centre) of Multimedia University for granting the research ethics approval for the project and public disclosure approval for this paper. The researchers are grateful to Mr Kai Loon Toh for his assistance in data collection, and to Mr Kian Siong Jee for his direct or indirect contributions related to this research. Special thanks go to Ms Chiew Fen Ng for her constructive criticism of the manuscript. Finally, the researchers thank the anonymous reviewers for their useful suggestions.
Additional information
Funding
Notes on contributors
Dominic Wen How Tan
Mr Dominic WH Tan is a Master of Engineering Science (MEngSc) student in the Faculty of Engineering and Technology (FET), Multimedia University (MMU). His research interests include design and ergonomics. He is involved in this study’s conceptualisation, analysis and discussion works.
Poh Kiat Ng
Ir Ts Dr Poh Kiat Ng is the Deputy Dean of FET, MMU. He received his PhD from Universiti Teknikal Malaysia Melaka (UTeM). His research interests include design, ergonomics and TRIZ. He is involved in this study’s methodology, analysis and editing works, and is the main supervisor in Dominic’s MEngSc study.
Ervina Efzan Mhd Noor
Associate Professor Ts Dr Ervina Efzan Mhd Noor is the Director of the Research Programme and Collaboration Centre (RPCC) in MMU. She received her PhD from Universiti Sains Malaysia (USM). Her research interests include soldering, corrosion and composite materials. She is involved in this study’s introductory and editing works, and is the co-supervisor in Dominic’s MEngSc study.
References
- Amca, A., Vigouroux, L., Arıtan, S., & Berton, E. (2012). The effect of chalk on the finger–hold friction coefficient in rock climbing. Sports Biomechanics, 11(4), 1–21. https://doi.org/10.1080/14763141.2012.724700
- Badawy, A., & Alfred, R. (2020). Myoelectric prosthetic hand with a proprioceptive feedback system. Journal of King Saud University - Engineering Sciences, 32(8), 388-395. https://doi.org/10.1016/j.jksues.2019.05.002
- Bae, J. H., Kang, S. H., Seo, K. M., Kim, D.-K., Shin, H. I., & Shin, H. E. (2015). Relationship between grip and pinch strength and activities of daily living in stroke patients. Annals of Rehabilitation Medicine, 39(5), 752–762. https://doi.org/10.5535/arm.2015.39.5.752
- Bainbridge, D., Seow, H., Sussman, J., Pond, G., Martelli-Reid, L., Herbert, C., & Evans, W. (2011). Multidisciplinary health care professionals’ perceptions of the use and utility of a symptom assessment system for oncology patients. Journal of Oncology Practice, 7(1), 19–23. https://doi.org/10.1200/JOP.2010.000015
- Bhardwaj, P., Nayak, S. S., Kiswar, A. M., & Sabapathy, S. R. (2011). Effect of static wrist position on grip strength. Indian Journal of Plastic Surgery: Official Publication of the Association of Plastic Surgeons of India, 44(1), 55–58. https://doi.org/10.4103/0970-0358.81440
- Brown, W. F. (1972). A method for estimating the number of motor units in thenar muscles and the changes in motor unit count with ageing. Journal of Neurology, Neurosurgery, and Psychiatry, 35(6), 845–852. https://doi.org/10.1136/jnnp.35.6.845
- Burnett, R. A., Laidlaw, D. H., & Enoka, R. M. (2000). Coactivation of the antagonist muscle does not covary with steadiness in old adults. Journal of Applied Physiology, 89(1), 61–71. https://doi.org/10.1152/jappl.2000.89.1.61
- Canning, C. G., Ada, L., Adams, R., & O’Dwyer, N. J. (2004). Loss of strength contributes more to physical disability after stroke than loss of dexterity. Clinical Rehabilitation, 18(3), 300–308. https://doi.org/10.1191/0269215504cr715oa.
- Chang, B.-C., Huang, B.-S., Chen, C.-K., & Wang, S.-J. (2007). The pincer chopsticks: The investigation of a new utensil in pinching function. Applied Ergonomics, 38(3), 385–390. https://doi.org/10.1016/j.apergo.2006.03.009
- Cole, K. J. (1991). Grasp force control in older adults. Journal of Motor Behavior, 23((4),), 251–258. https://doi.org/10.1080/00222895.1991.9942036
- Department of Statistics Malaysia. (2017). Ageing auguest department of statistics Malaysia. Department of Statistics Malaysia. Retrieved from https://www.dosm.gov.my/v1/
- Desrosiers, J., Bravo, G., & Hébert, R. (1997). Isometric grip endurance of healthy elderly men and women. Archives of Gerontology and Geriatrics, 24(1), 75–85. https://doi.org/10.1016/S0167-4943(96)00756-X
- Ding, H., Leino-Arjas, P., Murtomaa, H., Takala, E.-P., & Solovieva, S. (2013). Variation in work tasks in relation to pinch grip strength among middle-aged female dentists. Applied Ergonomics, 44(6), 977–981. https://doi.org/10.1016/j.apergo.2013.03.014
- Dodds, R. M., Syddall, H. E., Cooper, R., Benzeval, M., Deary, I. J., Dennison, E. M., & Sayer, A. A. (2014). Grip strength across the life course: Normative data from twelve British studies. PloS One, 9(12), e113637–e113637. https://doi.org/10.1371/journal.pone.0113637
- Duchateau, J., & Hainaut, K. (1990). Effects of immobilization on contractile properties, recruitment and firing rates of human motor units. The Journal of Physiology, 422(1), 55–65. https://doi.org/10.1113/jphysiol.1990.sp017972
- Ellis, E. A., Bloswick, D. S., Sesek, R. F., Mann, C., Thiese, M. S., & Hegman, K. T. 2004. The effect of pinch grip on upper extremity cumulative trauma disorders in female garment workers. Master of Science Dissertation University of Utah.
- Enders, L. R., & Jin Seo, N. (2011). Phalanx force magnitude and trajectory deviation increased during power grip with an increased coefficient of friction at the hand–object interface. Journal of Biomechanics, 44(8), 1447–1453. https://doi.org/10.1016/j.jbiomech.2011.03.020
- Enoka, R. M., Christou, E. A., Hunter, S. K., Kornatz, K. W., Semmler, J. G., Taylor, A. M., & Tracy, B. L. (2003). Mechanisms that contribute to differences in motor performance between young and old adults. Journal of Electromyography and Kinesiology, 13(1), 1–12. https://doi.org/10.1016/S1050-6411(02)00084-6
- Evans, W. J. (2010). Skeletal muscle loss: Cachexia, sarcopenia, and inactivity. The American Journal of Clinical Nutrition, 91(4), 1123S–1127S. https://doi.org/10.3945/ajcn.2010.28608A
- Fiatarone, M. A., & Evans, W. J. (1993). 11 the etiology and reversibility of muscle dysfunction in the aged. Journal of Gerontology, 48(Special_Issue), 77–83. https://doi.org/10.1093/geronj/48.Special_Issue.77
- Fiatarone, M. A., Marks, E. C., Ryan, N. D., Meredith, C. N., Lipsitz, L. A., & Evans, W. J. (1990). High-intensity strength training in nonagenarians: effects on skeletal muscle. JAMA, 263(22), 3029–3034. https://doi.org/10.1001/jama.1990.03440220053029
- Frontera, W. R., Suh, D., Krivickas, L. S., Hughes, V. A., Goldstein, R., & Roubenoff, R. (2000). Skeletal muscle fiber quality in older men and women. American Journal of Physiology. Cell Physiology, 279(3), C611–618. https://doi.org/10.1152/ajpcell.2000.279.3.C611
- Fuss, F., & Niegl, G. (2012). The importance of friction between hand and hold in rock climbing. Sports Technology, 5(3–4), 90–99. https://doi.org/10.1080/19346182.2012.755539
- Harris, J. E., & Eng, J. J. (2007). Paretic upper-limb strength best explains arm activity in people with stroke. Physical Therapy, 87(1), 88-97. https://doi.org/10.2522/ptj.20060065
- Heath, G. W., Hagberg, J. M., Ehsani, A. A., & Holloszy, J. O. (1981). A physiological comparison of young and older endurance athletes. Journal of Applied Physiology: Respiratory, Environmental and Exercise Physiology, 51(3), 634–640. https://doi.org/10.1152/jappl.1981.51.3.634
- Heffernan, C., & Freivalds, A. (2000). Optimum pinch grips in the handling of dies. Applied Ergonomics, 31(4), 409–414. https://doi.org/10.1016/S0003-6870(99)00064-2
- Hiramatsu, Y., Kimura, D., Kadota, K., Ito, T., & Kinoshita, H. (2015). Control of precision grip force in lifting and holding of low-mass objects. PlLoS One, 10(9), e0138506. https://doi.org/10.1371/journal.pone.0138506
- Höppner, H., McIntyre, J., & van der Smagt, P. (2013). Task dependency of grip stiffness—a study of human grip force and grip stiffness dependency during two different tasks with same grip forces. PloS One, 8(12), e80889. https://doi.org/10.1371/journal.pone.0080889
- Hosken, D. J., Buss, D. L., & Hodgson, D. J. (2018). Beware the F test (or, how to compare variances). Animal Behaviour, 136, 119–126. https://doi.org/10.1016/j.anbehav.2017.12.014
- Hu, W., Wei, N., Li, Z.-M., & Li, K. (2018). Effects of muscle fatigue on directional coordination of fingertip forces during precision grip. PloS One, 13(12), e0208740. https://doi.org/10.1371/journal.pone.0208740
- Hyun-Yong, H., Shimada, A., & Kawamura, S. (1996, 22-28 April 1996). Analysis of friction on human fingers and design of artificial fingers. Paper presented at the proceedings of IEEE international conference on robotics and automation. Minneapolis, Minnesota.
- Jover, J. A., Lajas, C., Leon, L., Carmona, L., Serra, J. A., Reoyo, A., Rodriguez-Rodriguez, L., & Abasolo, L., & for the Acute Physical Disability in the Elderly, G. (2015). Incidence of physical disability related to musculoskeletal disorders in the elderly: results from a primary care–based registry. Arthritis Care & Research, 67(1), 89–93. https://doi.org/10.1002/acr.22420
- Keller, K., & Engelhardt, M. (2014). Strength and muscle mass loss with aging process. Age and strength loss. Muscles, Ligaments and Tendons Journal, 3(4), 346–350. https://doi.org/10.32098/mltj.04.2013.17
- Kilbreath, S. L., & Gandevia, S. C. (1994). Limited independent flexion of the thumb and fingers in human subjects. The Journal of Physiology ( Pt 3). 479(3), 487–497. https://doi.org/10.1113/jphysiol.1994.sp020312.
- Kim, Y., Kim, W.-S., & Yoon, B. (2014). The effect of stroke on motor selectivity for force control in single- and multi-finger force production tasks. NeuroRehabilitation, 34(3), 429–435. https://doi.org/10.3233/NRE-141050
- Kirkendall, D. T., & Garrett, W. E. (1998). The effects of aging and training on skeletal muscle. The American Journal of Sports Medicine, 26(4), 598–602. https://doi.org/10.1177/03635465980260042401
- Ko, Y.-T., Lu, -C.-C., & Lee, L.-H. (2016). A contradiction-based approach for innovative product design. MATEC web conf., 68(05001), 1–5. https://doi.org/10.1051/matecconf/20166805001
- Kostopoulos, P., Kyritsis, A. I., Ricard, V., Deriaz, M., & Konstantas, D. (2018). Enhance daily live and health of elderly people. Procedia Computer Science, 130, 967–972. https://doi.org/10.1016/j.procs.2018.04.097
- Lang, C. E., & Schieber, M. H. (2003). Differential impairment of individuated finger movements in humans after damage to the motor cortex or the corticospinal tract. Journal of Neurophysiology, 90(2), 1160–1170. https://doi.org/10.1152/jn.00130.2003
- Lang, C. E., & Schieber, M. H. (2004). Human finger independence: limitations due to passive mechanical coupling versus active neuromuscular control. Journal of Neurophysiology, 92(5), 2802–2810. https://doi.org/10.1152/jn.00480.2004
- Latash, M. L., Scholz, J. F., Danion, F., & Schöner, G. (2001). Structure of motor variability in marginally redundant multifinger force production tasks. Experimental Brain Research, 141(2), 153–165. https://doi.org/10.1007/s002210100861
- Latash, M. L., Scholz, J. F., Danion, F., & Schöner, G. (2002). Finger coordination during discrete and oscillatory force production tasks. Experimental Brain Research, 146(4), 419–432. https://doi.org/10.1007/s00221-002-1196-4
- Levy-Tzedek, S., Hanassy, S., Abboud, S., Maidenbaum, S., & Amedi, A. (2012). Fast, accurate reaching movements with a visual-to-auditory sensory substitution device. Restorative Neurology and Neuroscience, 30(4), 313–323. https://doi.org/10.3233/RNN-2012-110219
- Li, K., Evans, P. J., Seitz, W. H., Jr., & Li, Z.-M. (2015). Carpal tunnel syndrome impairs sustained precision pinch performance. Clinical Neurophysiology: Official Journal of the International Federation of Clinical Neurophysiology, 126(1), 194–201. https://doi.org/10.1016/j.clinph.2014.05.004
- Li, K., Marquardt, T. L., & Li, Z.-M. (2013). Removal of visual feedback lowers structural variability of inter-digit force coordination during sustained precision pinch. Neuroscience Letters, 545, 1–5. https://doi.org/10.1016/j.neulet.2013.04.011
- Li, K., Wei, N., & Yue, S. (2016a, 15-17 Oct. 2016). Effects of tactile sensitivity on fingertip center-of-pressure distribution during stable precision grip. Paper presented at the 2016 9th International Congress on Image and Signal Processing, BioMedical Engineering and Informatics (CISP-BMEI).
- Li, K., Wei, N., & Yue, S. (2016b). Effects of tactile sensitivity on structural variability of digit forces during stable precision grip. BioMed Research International, 2016(8314561), 1–7. https://doi.org/10.1155/2016/8314561.
- Lin, C.-H., Sung, W.-H., Chiang, S.-L., Lee, S.-C., Lu, L.-H., Wang, P.-C., & Wang, X.-M. (2019). Influence of aging and visual feedback on the stability of hand grip control in elderly adults. Experimental Gerontology, 119, 74–81. https://doi.org/10.1016/j.exger.2019.01.024
- Liu, C., & Sun, Y. (2019). A simple and trustworthy asymptotic t test in difference-in-differences regressions. Journal of Econometrics, 210(2), 327–362. https://doi.org/10.1016/j.jeconom.2019.02.003
- Mantilla, C. B., & Sieck, G. C. (2008). Trophic factor expression in phrenic motor neurons. Respiratory Physiology & Neurobiology, 164(1–2), 252–262. https://doi.org/10.1016/j.resp.2008.07.018
- Marcell, T. J. (2003). Review article: sarcopenia: causes, consequences, and preventions. The Journals of Gerontology: Series A, 58(10), M911–M916. https://doi.org/10.1093/gerona/58.10.M911
- Massy-Westropp, N. M., Gill, T. K., Taylor, A. W., Bohannon, R. W., & Hill, C. L. (2011). Hand grip strength: Age and gender stratified normative data in a population-based study. BMC Research Notes, 4(1), 127. https://doi.org/10.1186/1756-0500-4-127
- Mitchell, W. K., Williams, J., Atherton, P., Larvin, M., Lund, J., & Narici, M. (2012). Sarcopenia, dynapenia, and the impact of advancing age on human skeletal muscle size and strength; a quantitative review. Frontiers in Physiology, 3, 260. https://doi.org/10.3389/fphys.2012.00260
- Naqvi, S. (1993). Need for anthropometric database for developing countries. Journal of King Saud University - Engineering Sciences, 5(1), 153–154. https://doi.org/10.1016/S1018-3639(18)30577-4
- Naqvi, S. (1996). Critical human factor issues in quality inspection tasks. Journal of King Saud University - Engineering Sciences, 8(1), 133–140. https://doi.org/10.1016/S1018-3639(18)30644-5
- Ng, P. K., Bee, M. C., Saptari, A., & Mohamad, N. A. (2014). A review of different pinch techniques. Theoretical Issues in Ergonomics Science, 15(5), 517–533. https://doi.org/10.1080/1463922X.2013.796539
- Nilsen, T., Hermann, M., Eriksen, C. S., Dagfinrud, H., Mowinckel, P., & Kjeken, I. (2012). Grip force and pinch grip in an adult population: reference values and factors associated with grip force, Scandinavian Journal of Occupational Therapy, 19(3), 288–296. https://doi.org/10.3109/11038128.2011.553687
- Nurul Shahida, M. S., Siti Zawiah, M. D., & Case, K. (2015). The relationship between anthropometry and hand grip strength among elderly Malaysians. International Journal of Industrial Ergonomics, 50, 17–25. https://doi.org/10.1016/j.ergon.2015.09.006
- Obmaces, R. (2015). Indiegogo. Grip-Aid: A device for hand mobility issues. Indiegogo. Retrieved from https://www.indiegogo.com/projects/grip-aid-a-device-for-hand-mobility-issues#/
- Paillard, T. (2017). Relationship between muscle function, muscle typology and postural performance according to different postural conditions in young and older adults. Frontiers in Physiology, 8(585), 1–6. https://doi.org/10.3389/fphys.2017.00585
- Pette, D. (2007). Skeletal muscle plasticity – History, facts and concepts (pp. 1–27).
- Portnoy, S., Halaby, O., Dekel-Chen, D., & Dierick, F. (2015). Effect of an auditory feedback substitution, tactilo-kinesthetic, or visual feedback on kinematics of pouring water from kettle into cup. Applied Ergonomics, 51, 44–49. https://doi.org/10.1016/j.apergo.2015.04.008
- Rahman, N., Thomas, J. J., & Rice, M. S. (2002). The relationship between hand strength and the forces used to access containers by well elderly persons. American Journal of Occupational Therapy, 56((1),), 78–85. https://doi.org/10.5014/ajot.56.1.78
- Ranganathan, V. K., Siemionow, V., Sahgal, V., & Yue, G. H. (2001). Effects of aging on hand function. Journal of the American Geriatrics Society, 49((11),), 1478–1484. https://doi.org/10.1046/j.1532-5415.2001.4911240.x
- Rantanen, T., Guralnik, J. M., Foley, D., Masaki, K., Leveille, S., Curb, J. D., & White, L. (1999). Midlife hand grip strength as a predictor of old age disability. JAMA, 281(6), 558–560. https://doi.org/10.1001/jama.281.6.558
- Ribeiro, F., & Oliveira, J. (2007). Aging effects on joint proprioception: The role of physical activity in proprioception preservation. European Review of Aging and Physical Activity, 4(2), 71–76. https://doi.org/10.1007/s11556-007-0026-x
- Rice, M. S., Leonard, C., & Carter, M. (1998). Grip strengths and required forces in accessing everyday containers in a normal population. American Journal of Occupational Therapy, 52(8), 621–626. https://doi.org/10.5014/ajot.52.8.621
- Sayer, A. A., Syddall, H., Martin, H., Patel, H., Baylis, D., & Cooper, C. (2008). The developmental origins of sarcopenia. The Journal of Nutrition, Health & Aging, 12(7), 427–432. https://doi.org/10.1007/bf02982703
- Schieber, M. H., Lang, C. E., Reilly, K. T., McNulty, P., & Sirigu, A. (2009). Selective activation of human finger muscles after stroke or amputation. In D. Sternad (Ed.), Progress in motor control: a multidisciplinary perspective (pp. 559–575). Springer US.
- Seals, D. R., Hagberg, J. M., Hurley, B. F., Ehsani, A. A., & Holloszy, J. O. (1984). Endurance training in older men and women. I. Cardiovascular responses to exercise. Journal of Applied Physiology, 57(4), 1024–1029. https://doi.org/10.1152/jappl.1984.57.4.1024
- Serdar, C. C., Cihan, M., Yücel, D., & Serdar, M. A. (2021). Sample size, power and effect size revisited: Simplified and practical approaches in pre-clinical, clinical and laboratory studies. Biochemia Medica, 31((1),), 010502. https://doi.org/10.11613/BM.2021.010502
- Shih, Y.-C., & Ou, Y.-C. (2005). Influences of span and wrist posture on peak chuck pinch strength and time needed to reach peak strength. International Journal of Industrial Ergonomics, 35(6), 527–536. https://doi.org/10.1016/j.ergon.2004.12.002
- Shim, J. K., Latash, M. L., & Zatsiorsky, V. M. (2003). The human central nervous system needs time to organize task-specific covariation of finger forces. Neuroscience Letters, 353(1), 72–74. https://doi.org/10.1016/j.neulet.2003.08.079
- Shim, J. K., Lay, B. S., Zatsiorsky, V. M., & Latash, M. L. (2004). Age-related changes in finger coordination in static prehension tasks. Journal of Applied Physiology (Bethesda, MD.: 1985), 97((1),), 213–224. https://doi.org/10.1152/japplphysiol.00045.2004
- Shin, H., Moon, S. W., Kim, G.-S., Park, J. D., Kim, J. H., Jung, M. J., Yoon, C. H., Lee, E. S., & Oh, M.-K. (2012). Reliability of the pinch strength with digitalized pinch dynamometer. Annals of Rehabilitation Medicine, 36(3), 394–399. https://doi.org/10.5535/arm.2012.36.3.394
- Shumway-Cook, A., & Woollacott, M. H. (2007). Motor Control:. Translating Research Into Clinical Practice: Lippincott Williams & Wilkins.
- Siparsky, P. N., Kirkendall, D. T., & Garrett, W. E., Jr. (2014). Muscle changes in aging: Understanding sarcopenia. Sports Health, 6(1), 36–40. https://doi.org/10.1177/1941738113502296
- Souchkov, V., Hoeboer, R., & Zutphen, M. V. (2007, February 5). TRIZ for business: application of RCA+ to analyse and solve business and management problems. The TRIZ Journal, 1–9. http://gg.gg/5u7sk).
- Tekscan. (2020). FlexiForce load/force sensors and system. pressure mapping, force measurement and tactile sensors. Tekscan, Inc. Retrieved from https://www.tekscan.com/flexiforce-load-force-sensors-and-systems
- Tomimoto, M. (2011). The frictional pattern of tactile sensations in anthropomorphic fingertip. Tribology International, 44(11), 1340–1347. https://doi.org/10.1016/j.triboint.2010.12.004
- Wallström, Å., & Nordenskiöld, U. (2001). Assessing hand grip endurance with repetitive maximal isometric contractions. Journal of Hand Therapy, 14((4),), 279–285. https://doi.org/10.1016/S0894-1130(01)80006-5
- Lam, N. W., Goh, H.-T., Kamaruzzaman, S. B., Chin, A., Poi, P. J. H., & Tan, M. P. (2015). Normative data for handgrip strength and key pinch strength, stratified by age and gender for a multi-ethnic Asian population, Singapore Medical Journal, 57(10), 578–584. https://doi.org/10.11622/smedj.2015164
- Wickremaratchi, M. M., & Llewelyn, J. G. (2006). Effects of ageing on touch. Postgraduate Medical Journal, 82(967), 301–304. https://doi.org/10.1136/pgmj.2005.039651
- Wolbrecht, E. T., Rowe, J. B., Chan, V., Ingemanson, M. L., Cramer, S. C., & Reinkensmeyer, D. J. (2018). Finger strength, individuation, and their interaction: Relationship to hand function and corticospinal tract injury after stroke. Clinical Neurophysiology, 129(4), 797–808. https://doi.org/10.1016/j.clinph.2018.01.057
- World Health Organisation. (2016). Health statistics and information systems: proposed working definition of an older person in Africa for the MDS project. World Health Organisation. Retrieved from http://www.who.int/healthinfo/survey/ageingdefnolder/en/
- Wu, H.-C., Chiu, M.-C., & Hou, C.-H. (2015). Nail clipper ergonomic evaluation and redesign for the elderly. International Journal of Industrial Ergonomics, 45, 64–70. https://doi.org/10.1016/j.ergon.2014.12.002
- Yan, W., Zanni-Merk, C., Rousselot, F., Cavallucci, D., & Collet, P. (2013). A new method of using physical effects in su-field analysis based on ontology reasoning. Procedia Computer Science, 22, 30–39. https://doi.org/10.1016/j.procs.2013.09.078
- Yeoh, T. S. (2014). TRIZ: systematic innovation in business and management (1st ed.). Firstfruits Publishing.
- Yeoh, T. S., Yeoh, T. J., & Song, C. L. (2015). TRIZ: systematic innovation in manufacturing (1st ed.). Firstfruits Publishing.
- Zhang, Y., Niu, J., Kelly-Hayes, M., Chaisson, C. E., Aliabadi, P., & Felson, D. T. (2002). Prevalence of symptomatic hand osteoarthritis and its impact on functional status among the elderly: the Framingham study. American Journal of Epidemiology, 156(11), 1021–1027. https://doi.org/10.1093/aje/kwf141