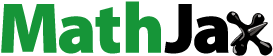
Abstract
Chalcogenide glasses are promising contenders for use in radiation shielding applications because of their remarkable radiation shielding properties, such as transparency and good thermal stability. In the current study, the shielding parameters for gamma rays and neutrons are estimated for different compositions of Se75−x–Te25–Agx (0 ≤ x ≤ 10 mole %) chalcogenide glasses using the Phy-X/PSD online software. We referred to the investigated Se75−x–Te25–Agx (x = 0, 2, 4, 6, 8, and 10 mole %) glasses by coded abbreviations as (STA1, STA2, STA3, STA4, STA5 and STA6 respectively). The linear attenuation coefficient (LAC), mass attenuation coefficient (MAC), effective atomic number (Zeff), effective electron density (Neff), half-value layer (HVL), tenth-value layer (TVL), mean free path (MFP), energy absorption buildup factor (EABF), and exposure buildup factor (EBF) in the photon energy range of 0.15–15 MeV. The specified energy range (0.015–15 MeV) corresponds to the energies emitted from radioactive sources such as (241Am, 55Fe, 133Ba, 60Co, 109Cd, 131I, 152Eu, 22Na, and 137Cs) which may be used in medical, industrial, and nuclear facilities where workers may frequently be exposed to harmful radiations. In the current work, we investigate shielding materials to protect people from radiation-related harm. Furthermore, the fast neutron removal cross section (FNRCS) is estimated. The findings refer to increasing the Ag content results in an enhancement in the shielding characteristics for the glass under investigation. The lowest Zeff values (36.657–37.465) occur at a photon energy of 0.02 MeV; the Zeff values then increase to reach their maxima (43.259–44.347) at a photon energy of 0.06 MeV. The MFP, TVL, and HVL values decrease with increasing Ag content, especially in the intermediate and high photon energy ranges. The results show low HVL and MFP values compared with those obtained for traditional and commercial glasses, especially in the intermediate and high photon energy ranges. The Zeff values increase as the Ag content increases. The FNRCS values are in the range of 0.083–0.092 cm−1 for the investigated glasses. The FNRCS values of these glasses are higher than those of some commercial glasses. The most performance sample for radiation shielding between the investigated glasses is STA6 corresponds to the higher concentration of Ag.
1. Introduction
Ionizing radiation is an energy source that has many useful applications in agriculture, industry, medicine, and research. However, these radiations have harmful effects on both the environment and people since they cause atom disturbance, create negative electrons and positive ions, and cause biological harm as reported by Beir (Citation1990). Neutrons, X-rays, gamma rays, and charged particles are some of the most common harmful ionizing radiations. Gamma rays are regard as the extremely dangerous ionizing radiation since they have no mass nor charge and can reach a deep penetration through long distances as mentioned by Gokce et al. (Citation2018).
Protecting the environment and people from harmful radiation has become the focus of considerable research. Several attempts have been made to control and reduce harmful radiation exposure through radiation shielding. Developing cheap and easy-to-process shielding materials that can offer reliable protection against the harmful effect of radiations has become essential. For many years, lead (Pb) has been used in different shielding applications attributed to its hardness, low cost, and good capability to protect against ionizing radiations as explained in previous works of AbuAlRoos et al. (Citation2019) and Al-Buriahi, El-Agawany et al. (Citation2020). However, the use of lead has been recently limited because of its toxicity, which has forced many researchers to find alternative shielding materials as Al-Hadeethi et al. (Citation2020), Hsiao et al. (Citation2011), Ogawa et al. (Citation2008), and Hulbert and Carlson (Citation2009). Concrete and rocks are the most commonly used materials in shielding. Also, Al-Buriahi and Singh (Citation2020), Obaid, Gaikwad and Pawar (Citation2018), Tekin and Kilicoglu (Citation2020), and Akman et al. (Citation2019), Obaid, Sayyed et al. (Citation2018) and Al-Buriahi, Abouhaswa et al. (Citation2020) have been suggested alloys and polymers as candidate materials for radiation shielding; however, they have several practical limitations and are not transparent.
Recently, glasses have attracted considerable attention for use in shielding products attributed to their remarkable characteristics for radiation shielding, such as transparency, good thermal stability, and fabrication flexibility in various sizes and shapes as reported by Yankov et al. (Citation2012) and Guo et al. (Citation2016). The chalcogen elements S, Se, and Te provide the basis for the versatile materials known as chalcogenide glasses. These glasses are utilized in a variety of technological applications as introduced by Schindler et al. (Citation2009), Hewak (Citation2011), Ailavajhala et al. (Citation2014), and Donghui et al. (Citation2006). There is great interest toward chalcogenide glass-ceramics in the field of radiation shielding because of their promising physical, optical, and thermal properties. Also, they are very sensitive to irradiations because of their flexible structures as given by Mikla et al. (Citation2018). Chalcogenide glasses were investigated by many researchers for shielding applications. Te-based glasses exhibit high thermal stability and fast crystallization times, which makes it applicable in many different applications as presented by Mhareb et al. (Citation2021). Al-Buriahi and Mann (Citation2019) investigated the radiation-shielding capabilities of Te, Nb, and W glass composite. Alzahrani et al. (Citation2021) studied the radiation protection of a TeO2-Na2O-TiO glasses. Both investigations showed the importance of Te element for radiation shielding in the investigated glass systems. Amorphous Se–Te glasses show high photosensitivity and high hardness. Ag-doped chalcogenide glasses attract many researchers for fundamental research in many applications including radiation shielding. Ge-Se-Sb-Ag composites were investigated by Kebaili et al. (Citation2021) for the radiation shielding ability. They show that the Ge-Se-Sb-Ag glasses exhibit good ability to gamma-ray and also beta -ray shielding. Shielding properties for (GeS2)60-(Sb2S3)40-x-(CdCl2)x (0 ≤ x ≤ 40 mole %) chalcogenide glasses were examined by El-Agawany et al. (Citation2020). These glasses showed good protection properties against gamma rays and neutrons. In this research Se–Te–Ag chalcogenide glass with a new composite was chosen for further investigation concerning shielding properties. This can help to can adopt the best compositions for such materials in shielding applications.
It is difficult to conduct experimental radiation studies because of high cost, possible biological risks and the existence of strict regulations for environment protection. As a result, different software packages (e.g., XCOM) as well as Monte Carlo simulations with different codes have been developed to optimize the glass shielding properties before fabrication by Issa et al. (Citation2019), Rammah, Askin et al. (Citation2019), Rammah, El-Agawany et al. (Citation2019), Rammah et al. (Citation2018), El-Agawany et al. (Citation2019), Al-Hadeethi et al. (Citation2019), and Kumar et al. (Citation2020); these properties determine the glass performance in applications related to radiation protection.
Şakar et al. (Citation2020) recently developed a newly open-source online software package, namely, Phy-X/PSD, which has been employed to estimate the various shielding parameters of several materials in different photon energy ranges. This software has been used by many researchers to estimate the shielding aspects of various compositions as introduced by Al-Hadeethi and Sayyed (Citation2020, Citation2020b), Al-Hadeethi, Sayyed, Agar et al. (Citation2020) and El-Qahtani et al. (Citation2021). Alzahrani, Kavas et al. (Citation2021), Al-Harbi et al. (Citation2021), Kurtulus et al. (Citation2021), Cinan et al. (Citation2021), Cinan et al. (Citation2021), Kavas et al. (Citation2022), Mariselvam and Liu (Citation2022), Cinan et al. (Citation2022), and Al-Baradi et al. (Citation2022).
The most important parameters for radiation shielding are (i) the linear attenuation coefficient (LAC), this is dependent on the incident photon energy, material density, and atomic number as reported by Wood (Citation1982) and Akkurt et al. (Citation2005); (ii) the half-value layer (HVL), which describes the material thickness that is essential to attenuate the incident energy to its half-value; (iii) the energy absorption buildup factor (EABF); (iv) the mean-free path (MFP); (v) the exposure buildup factor (EBF); (vi) the effective electron density (Neff) and effective atomic number (Zeff), which have been evaluated in many works for various materials investigated by Perisanoglu et al. (Citation2020), Al-Buriahi et al. (Citation2020), Tonguc et al. (Citation2018), Al-Buriahi and Rammah (Citation2019), Al-Buriahi and Tonguc (Citation2019), Mahmoud et al. (Citation2020), and Gaikwad et al. (Citation2018).
In the current study, the shielding parameters were evaluated for different compositions of Se75−x–Te25–Agx (0 ≤ x ≤ 10 mole %) glasses using the Phy-X/PSD software package. Numerous shielding parameters were evaluated at 0.015–15 MeV. Compared to other research in the literature, this work is significantly more thorough, and the evaluation of the theoretical data across all aspects demonstrates the study’s originality.
2. Materials and procedures
2.1. Chalcogenide glass description
Promising chalcogenide glasses with the chemical formula Se75−x–Te25–Agx (x = 0, 2, 4, 6, 8, and 10 mole %) were chosen in the current study. Similar Se–Te–Ag glass samples were prepared in a previous work introduced by Mishra et al. (Citation2011) using the conventional quenching method. The different glass samples are here referred to as STA1 (x = 0 mol%), STA2 (x = 2 mol%), STA3 (x = 4 mol%), STA4 (x = 6 mol%), STA5 (x = 8 mol%), and STA6 (x = 10 mol%). The glass density was calculated theoretically. The chemical formula and density of the STA glasses are presented in Table . The increase in density from 5.298 g/cm3 for STA1 to 5.937 g/cm3 for STA6 is due to the Ag element having a higher density (10.49 g/cm3) than the Se element (4.79 g/cm3) it replaces.
Table 1. Chemical properties of the Se75−x–Te25–Agx (x = 0, 2, 4, 6, 8, and 10 at%) chalcogenide glasses
2.2. Phy-X/PSD software
The Phy-X/PSD software was employed by Şakar et al. (Citation2020) to calculate various shielding parameters at different energy values. The material chemical composition (in mole or weight fraction) and density are required to calculate the different parameters. The calculation of the shielding parameters can be performed at 0.015–15 MeV of photon energies. Eighteen shielding parameters can be computed accurately and quickly using this software. The specified photon energies (0.015–15 MeV) existed as an option in Phy-X/PSD Software because it is matching the energies emitted from the radioactive source as (241Am, 55Fe, 133Ba, 60Co, 109Cd, 131I, 152Eu, 22Na, and 137Cs) which may be used in Medical, industrial and nuclear facilities that has workers may frequently affected by harmful radiations, since in the present work we aim to investigate shielding materials to protect the human being from negative effects of radiations. The obtained parameters can then be easily saved in Excel files.
2.3. Theoretical expressions of the shielding parameters
The parameter μ (cm−1) of the incident gamma rays of the investigated STA glasses can be described using the Beer–Lambert relationship, which is shown in EquationEq. (1)(1)
(1) as reported by Jackson and Hawkes (Citation1981).
The incident radiation intensity I0 decreases exponentially as the photons travel through the STA glass. I represents the transmitted radiation intensity obtained after the photons pass through an STA glass of thickness d. µ (cm−1) describes the interaction probability between the glass and the incident photons, which depends on d. The MAC (μm; in cm2/g) depends on µ (cm−1) and on the density ρ (g/cm3) of the STA glasses.
dm (in g/cm2) and wj represent the mass thickness of the STA glasses and the fraction weight of the jth included element, respectively.
The HVL and TVL parameters are used to describe the thickness essential to diminish the photon intensity to 50% and 10% of its initial value, respectively, as mentioned by Şakar et al. (Citation2020):
MFP is an important parameter that specifies the average distance traveled by a photon during two consecutive collisions with the glass nucleus or electrons, and it can be evaluated according to the following expression as reported by Şakar et al. (Citation2020):
Zeff is the number of electrons in the glass sample that participate in the atom–photon interaction. Zeff is given by the followed relation as mentioned by Han and Demir (Citation2009, Citation2010) and Şakar et al. (Citation2020):
σa (cm2/gm) is the total atomic cross section; σe (in cm2/gm) is the total electronic cross section; NA is the Avogadro number; fj,Zj, and Aj are the mole fraction, atomic number, and atomic weight of the jth involved element, respectively.
The effective conductivity (Ceff; in S/m) can be calculated according to the next expression mentioned by Manjunatha (Citation2017):
τ is the average lifetime of the electrons at the Fermi surface.
To use the Beer–Lambert relationship, three conditions should be satisfied: (1) the absorbing material should be very thin; (2) the incident radiation should be monochromatic; (3) the incident beam should be narrow. If one of these conditions is not met, the relationship cannot be used, and correction factors, known as buildup factors, must be introduced. The buildup factors are the EABF and EBF paramters. Fitting with a geometric progression was used to determine the buildup factors as mentioned by El-Qahtani et al. (Citation2021).
2.4. Neutron attenuation characteristics
The interaction between the neutrons and material may occur via nuclear fission, neutron capture, nuclear spallation processes, and elastic and inelastic scattering. The FNRCS (ΣR) parameter is used to measure the material ability to attenuate neutrons and can be determined using the following equation:
Here, (ΣR/ρ)I and ρi are the mass removal cross section (MRCS) and partial density of the ith constitute element, respectively. The MRCS values were taken from the literature of Chilton et al. (Citation1984) and Kaplan (Citation1989).
3. Results and discussion
The MAC values (cm2g−1) were computed for all the STA glasses investigated. Figure shows that all the glass samples exhibit a high MAC value at a low photon energy. Furthermore, the MAC value decreases exponentially for all the glass samples. At energies of 0.015–0.1 MeV, in all samples, the MAC value decreases sharply because of the photoelectric effect, which is relative to E−3.5 as reported by Hubbell and Seltzer (Citation1995). For example, sample STA1 shows a decrease in the MAC value from 84.88 cm2g−1 (at 0.015 MeV) to 1.038 cm2g−1 (at 0.1 MeV), whereas sample STA6 shows a decrease from 78.193 cm2g−1 (at 0.015 MeV) to 1.122 cm2g−1 (at 0.1 MeV). At 0.015–0.03 MeV, the MAC value decreases as the Ag concentration increases, which is because of the K-absorption edge of the most abundant elements (Se, Te), as presented in Table . At a photon energy ranging from 0.1 to 2 MeV, a slow reduction in the MAC value can be noticed because of the Compton scattering effect, which is relative to E−1. At a higher photon energy, the MAC value remains basically constant attributed to the pair production interaction between the glass and the photon, which is relative to log E as mentioned by Mahmoud et al. (Citation2019). Increasing the Ag concentration does not result in any observable effect at an energy exceeding 0.1 MeV. The LAC value presented against energies 0.015 to 15 MeV in Figure . Both the LAC and MAC values show the same trend for the STA glasses investigated.
Table 2. Absorption edges of the constituent elements under investigations as illustrated by Hubbell and Seltzer (Citation1995)
The MAC and LAC values give a direct indication about the better shielding properties, the greater MAC and LAC values are better for the radiation-shielding ability. Furthermore, the LAC values of the STA glasses were compared with those of some commercial glasses as RS-253, RS 253-G18, RS 323-G19, RS-360 and RS-520, which appeared on the website of SCHOTT (SCHOTT, Citation2022). The LAC values obtained for the STA glasses and those of the mentioned commercial glasses at photon energies 0.2, 0.662 and 1.25 MeV are presented in Table . The investigated STA glasses show higher shielding ability than that of the RS-253 and RS 253-G18 commercial glasses at all the selected photo energy. Also, it can be observed that the shielding properties of the examined STA glasses is very near for that of the RS-360 and RS-520 commercial glasses at 0.662 and 1.25 MeV.
Table 3. LAC values of the STA1–STA6 glasses compared with those of commercial glasses as given at SCHOTT (Citation2022) company website SCHOTT
Hence, the commercial glasses here are RS-520 which is a code of the chemical composition (27SiO2-71PbO-Na2O-K2O), RS-253-G18 which is a code of the chemical composition (70SiO2-10B2O3-9K2O-8Na2O-BaO-2CeO2) and (RS-360) which is a code of the chemical composition (45SiO2-9K2O-Na2O-45PbO).
For many applications, such as in medicine and nuclear physics, the optimization of the glass thickness is very important. The HVL and TVL factors of the STA glasses were computed using the LAC values and are displayed in Figures , respectively. The HVL and TVL trends are identical for all the glasses. The HVL values are small at an incident photon energy of less than 0.1 MeV (the region dominated by the photoelectric effect). For a photon energy higher than 0.1 MeV (the area where pair creation and the Compton effect are dominant), the HVL values increase rapidly until they reach the maximum at 6 MeV and then decrease. The HVL peak values are 4.019 cm for STA1, 3.909 cm for STA2, 3.806 cm for STA3, 3.708 cm for STA4, 3.616 cm for STA5, and 3.529 cm for STA6. The STA6 has the smallest HVL value over the whole examined energies because it has the highest density and LAC value. Figure shows that the STA6 has the lowest TVL value among the investigated glasses. It can be shown that increasing the Ag content results in an improvement in the shielding factors of the STA glasses at intermediate and high photon energies. The better the shielding properties of the material is characterized by less HVL and TVL values. In this study, the STA1-STA6 glass samples have HVL values 0.02, 0.019, 0.0184, 0.177, 0.0171 and 0.0164 at 0.05 MeV and these value increase to 3.527, 3.425, 3.329, 3.238, 3.153 and 3.0723 at 15 MeV, respectively. The HVL values for the STA glasses were compared with that calculated in the literature for some glass composite. For examples Al-Hadeethi and Sayyed (Citation2020) have got HVL values for SeO2–Ag2O–TeO2 glasses 1.364, 1.304, 1.251, 1.213, 1.181, and 1.156 cm, at 0.05 MeV, and the values increased to 3.319, 3.153, 3.006, 2.900, 2.795, and 2.724 cm, at 15 MeV, respectively. It can be shown that the STA glasses show better shielding effects at low photon energy compared with that of the mentioned glasses. Also, El-Agawany et al. (Citation2020) examined the radiation protection properties of ternary Ge–Sb–S chalcogenide glasses composite. They got thin HVL value 0.0042 cm at 0.015 MeV and 5.5160 cm at 15 MeV, respectively, whereas our investigated STA glasses HVL values vary between 0.0015 cm at 0.015 MeV and 3.07 cm at 15 MeV. It can be concluded that STA glasses showed lower HVL values, which indicating that STA glasses showed better radiation features.
The MFP value versus energies 0.015–15 MeV is illustrated in Figure . It is obvious from figure that MFP value exhibits the same trend as those of the TVL and HVL values. The behavior of MFP can be interpreted in the same way as mentioned for the TVL and HVL parameters. Increasing the Ag content results in a reduction in the MFP value of the glasses at intermediate and high energies. The STA6 glass sample is the best candidate for gamma-ray shielding.
The HVL and MFP values of the STA glasses were compared to those of some commercial glasses (RS-253-G18, RS-520 and RS-360), traditional glasses (barite, chromite, magnetite, and ferrite) and As40Se40Sn20 chalcogenide glass (ASS5) investigated previously by El-Qahtani et al. (Citation2021) according to Phy-X/PSD Software. As shown in Figures , the STA glasses under investigation exhibit low HVL values compared with those of the abovementioned traditional, commercial glasses and ASS5 chalcogenide glass except RS-520, especially at intermediate and high photon energies. The same trend can be noticed for the MEP values as shown in Figure , which emphasizes the superior shielding characteristics of the chalcogenide STA glasses.
Figure 6. HVL values of the STA1–STA6 chalcogenide glasses compared with those of some commercial, traditional glasses and chalcogenide glass. Also, the inset shows a zoomed view of the curves.
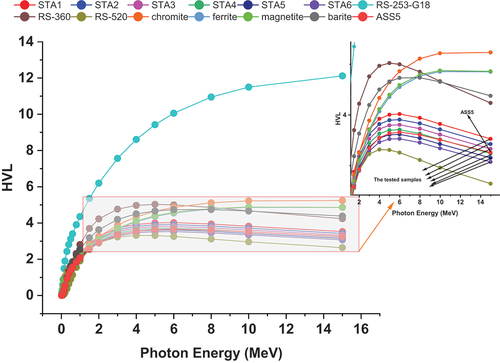
Figure 7. MFP values of the STA1–STA6 chalcogenide glasses compared with those of some commercial, traditional glasses and chalcogenide glass. Also, the inset shows a zoomed view of the curves.
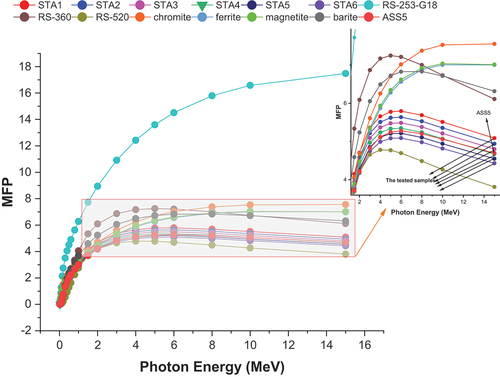
The interaction probability of the glass unit volume per atom and per electron is described by the ACS and ECS parameters, respectively. High ACS and ECS values indicate excellent radiation protection characteristics. Figures show the ACS and ECS values as a function of the photon energies at 0.015–15 MeV for the STA glasses, respectively. The figures show that at lower photon energies (E ˂ 0.1 MeV), the ECS and ACS values are high. The ECS value varies from 3.50397E−22 to 6.46854E−24, while the ACS value varies from 1.28446E−20 to 2.79612E−22. In the region dominated by the photoelectric effect (0.015–0.1 MeV), the ECS and ACS values decrease sharply. In the photon energy range of 0.1–2 MeV, which is dominated by the Compton effect, the ECS and ACS values decrease at a slower rate, at high photon energy range, that is dominated by the pair production (E ˃ 2 MeV), the ECS and ACS values remain basically constant.
Furthermore, Zeff is an important parameter that describes the glass ability to attenuate photons. Glasses with a high Zeff value are considered good candidates for gamma-ray shielding. Figure shows Zeff as a function of the photon energy for the STA glasses. The lowest values of Zeff (36.657–37.465) occur at 0.02 MeV; the Zeff values then increase to reach their maxima (43.259–44.347) at 0.06 MeV. A sharp decrease in the values occur at a photon energy of 0.4 MeV. The Zeff values then decrease exponentially in the photon energy range of 0.5–1 MeV, and they remain almost constant in the photon energy range of 1.5–15 MeV. The STA6 sample exhibits the highest Zeff value; this sample possesses the highest Ag content, and it is thus the best applicant for shielding among the tested STA glasses.
Figure shows the variation of Neff with the photon energy. It can be observed that Neff has the same trend as Zeff. The STA1 sample (with the lowest Ag content) has the highest Neff value at the photon energies of 0.05 and 0.06 MeV, which correspond approximately to the K-absorption edges of the Ag and Te elements. Ceff is another parameter that depends on Neff, and its trend with the photon energy is illustrated in Figure . It can be observed that the Ceff values increase as the Ag content increases for the STA glasses.
The R factor is calculated from the ratio of (μ/ρ)Compton and (μ/ρ)total. Figure illustrates the R variation with energies of 0.015–15 MeV. The R values of all the STA glasses are the highest at 1 and 1.5 MeV, where the Compton effect is more likely to occur. The variation of Zeq with the photon energy is shown in Figure . The Zeq values increase at energy range of 0.015–1 MeV and reach their highest values at 1 MeV; they then decrease slightly at higher energies. At intermediate and high energies, the Zeq values increase with increasing Ag content.
Figures illustrate the EABF and EBF values of the STA glasses varied with energies at several MFP values. The EABF and EBF values are minimum at lower energies attributed to the photoelectric effect; indeed, at this energy range, the incident photon energy is absorbed by the STA glasses. The STA glasses show a sudden increase in the EBF and EABF values at a photon energy of around 0.03 MeV. The peak intensity of the EBF and EABF values decreases as the Ag content increases, which is related to the development in radiation protection properties with increasing Ag content. A moderate increase in the EBF and EABF values occurs at energies of 0.15–0.8 MeV because of the Compton effect. At higher energies, the EBF and EABF values decrease slightly. The STA6 possesses the lowest values of EBF and EABF.
In neutron protection, the neutron attenuation is described by FNRCS or ∑R. Phy-X/PSD software was used to calculate the FNRCS values of the STA glasses and compare them to certain conventional, commercial, and chalcogenide glasses. The FNRCS values are shown in Figure in the range of 0.083–0.092 cm−1 for the STA glasses. From the figure, the FNRCS of STA system are higher than those of RS-520, RS-253-G18, and RS-360 glasses. It is worth noting FNRCS values previously published by El-Agawany et al. (Citation2020) for a chalcogenide glass system, the values were between (0.0691 and 0.0695 cm−1) which refers to the superior of our system for neutron shielding. Additionally, STA6 sample has the highest FNRCS value between the investigated STA glasses, hence Ag doping enhance the neutron protection ability.
4. Conclusion
Gamma-ray shielding parameters were evaluated for different compositions of Se75−x–Te25–Agx (0 ≤ x ≤ 10 mole %) chalcogenide glasses at photon energies of 0.015–15 MeV utilizing the Phy-X/PSD software. The LAC, MAC, Zeff, Neff, HVL, TVL, MFP, EABF, and EBF factors were evaluated. The LAC and MAC values were found to increase with increasing Ag content. Furthermore, the investigated STA glasses show higher LAC values which mean better protecting capability than that of the RS-253 and RS 253-G18 commercial glasses at energies 0.2, 0.662 and 1.25 MeV. The STA1-STA6 glass samples have HVL values 0.02, 0.019, 0.0184, 0.177, 0.0171 and 0.0164 at 0.05 MeV and these value increase to 3.527, 3.425, 3.329, 3.238, 3.153 and 3.0723 at 15 MeV, respectively. The STA glasses under investigation exhibit low HVL values in comparison with those of certain traditional, commercial and chalcogenide glasses except RS-520, particularly at medium and high energies. The HVL, MFP, and TVL values and decreased with increasing Ag content, specifically at medium and high energies. The lowest Zeff values (36.657–37.465) occurred at energy of 0.02 MeV; these values then increased to reach their highest values (43.259–44.347) at energy of 0.06 MeV. Furthermore, the FNRCS (ΣR) values, which are important for neutron shielding, were also estimated. When compared to the RS-520, RS-253-G18, and RS-360 glasses, the FNRCS values of the STA glasses were found to be greater. The STA glasses under investigation show promising shielding features, which can be considered for future work with different composite and with different doping element like Bismuth (Bi) or Antimony (Sb), and using different software.
Acknowledgements
The authors express their gratitude to the Princess Nourah bint Abdulrahman University Researchers Supporting Project number (PNURSP2022R38), Princess Nourah bint Abdulrahman University, Riyadh, Saudi Arabia.
Disclosure statement
No potential conflict of interest was reported by the author(s).
Additional information
Funding
References
- AbuAlRoos, N. J., Amin, N. A. B., & Zainon, R. (2019). Conventional and new lead-free radiation shielding materials for radiation protection in nuclear medicine: A review. Radiation Physics and Chemistry, 165, 108439. https://doi.org/10.1016/j.radphyschem.2019.108439
- Ailavajhala, M. S., Gonzalez-Velo, Y., Poweleit, C. D., Barnaby, H. J., Kozicki, M. N., Butt, D. P., & Mitkova, M. (2014). New functionality of chalcogenide glasses for radiation sensing of nuclear wastes. Journal of Hazardous Materials, 269, 68–20. https://doi.org/10.1016/j.jhazmat.2013.11.050
- Akkurt, I., Mavi, B., Akkurt, A., Basyigit, C., Kilincarslan, S., & Yalim, H. A. (2005). Study on Z dependence of partial and total mass attenuation coefficients. Journal of Quantitative Spectroscopy & Radiative Transfer, 94(3–4), 379–385. https://doi.org/10.1016/j.jqsrt.2004.09.024
- Akman, F., Kaçal, M. R., Sayyed, M. I., & Karataş, H. A. (2019). Study of gamma radiation attenuation properties of some selected ternary alloys. Journal of Alloys and Compounds, 782, 315–322. https://doi.org/10.1016/j.jallcom.2018.12.221
- Al-Baradi, A. M., Alotaibi, B. M., Alharbi, N., Abd El-Rehim, A. F., & Shaaban, K. S. (2022). Gamma radiation shielding and mechanical studies on highly dense lithium iron borosilicate glasses modified by zinc oxide. Silicon. 1-9. https://doi.org/10.1007/s12633-022-01801-9
- Al-Buriahi, M. S., Abouhaswa, A. S., Tekin, H. O., Sriwunkum, C., El-Agawany, F. I., Nutaro, T., Kavaz, E., & Rammah, Y. S. (2020). Structure, optical, gamma-ray and neutron shielding properties of NiO doped B2O3-BaCO3-Li2O3 glass systems. Ceramics International, 46(2), 1711–1721. https://doi.org/10.1016/j.ceramint.2019.09.144
- Al-Buriahi, M. S., El-Agawany, F. I., Sriwunkum, C., Akyıldırım, H., Arslan, H., Tonguc, B. T., El-Mallawany, R., & Rammah, Y. S. (2020). Influence of Bi2O3/PbO on nuclear shielding characteristics of lead-zinc-tellurite glasses. Physica B: Condensed Matter, 581, 411946. https://doi.org/10.1016/j.physb.2019.411946
- Al-Buriahi, M. S., & Mann, K. S. (2019). Radiation shielding investigations for selected tellurite-based glasses be-longing to the TNW system. Materials Research Express, 6(10), 105206. https://doi.org/10.1088/2053-1591/ab3f85
- Al-Buriahi, M. S., & Rammah, Y. S. (2019). Electronic polarizability, dielectric, and gamma-ray shielding properties of some tellurite-based glasses. Applied Physics A, 125(10), 678. https://doi.org/10.1007/s00339-019-2976-z
- Al-Buriahi, M. S., & Singh, V. P. (2020). Comparison of shielding properties of various marble concretes using GEANT4 simulation and experimental data. Journal of the Australian Ceramic Society, 56(3), 1127–1133. https://doi.org/10.1007/s41779-020-00457-1
- Al-Buriahi, M. S., & Tonguc, B. T. (2019). Study on gamma-ray buildup factors of bismuth borate glasses. Applied Physics A, 125(7), 482. https://doi.org/10.1007/s00339-019-2777-4
- Al-Hadeethi, Y., & Sayyed, M. I. (2020). Radiation attenuation properties of Bi2O3–Na2O– V2O5– TiO2–TeO2 glass system using Phy-X/PSD software. Ceramics International, 46(4), 4795–4800. https://doi.org/10.1016/j.ceramint.2019.10.212
- Al-Hadeethi, Y., & Sayyed, M. I. (2020). Using Phy-X/PSD to investigate gamma photons in SeO2–Ag2O–TeO2 glass systems for shielding applications. Ceramics International, 46(8), 12416–12421. https://doi.org/10.1016/j.ceramint.2020.02.003
- Al-Hadeethi, Y., Sayyed, M. I., & Agar, O. (2020). Ionizing photons attenuation characterization of quaternary tellurite–zinc–niobium–gadolinium glasses using Phy-X/PSD software. Journal of Non-Crystalline Solids, 538, 120044. https://doi.org/10.1016/j.jnoncrysol.2020.120044
- Al-Hadeethi, Y., Sayyed, M. I., Mohammed, H., & Rimondini, L. (2020). X-ray photons attenuation characteristics for two tellurite based glass systems at dental diagnostic energies. Ceramics International, 46(1), 251–257. https://doi.org/10.1016/j.ceramint.2019.08.258
- Al-Hadeethi, Y., Sayyed, M. I., & Rammah, Y. S. (2019). Investigations of the physical, structural, optical and gamma-rays shielding features of B2O3 – Bi2O3 – ZnO – CaO glasses. Ceramics International, 45(16), 20724–20732. https://doi.org/10.1016/j.ceramint.2019.07.056
- Al-Harbi, N., Sayyed, M. I., Kurtulus, R., Kamislioglu, M., Kumar, A., Alhuthali, A. M. S., Kavas, T., & Al-Hadeethi, Y. (2021). Understanding the role of Bi2O3 in the P2O5-CaO-Na2O-K2O glass system in terms of physical, structural and radiation shielding properties. Journal of Materials Science: Materials in Electronics, 32(9), 11649–11665. https://doi.org/10.1007/s10854-021-05775-z
- Alzahrani, J. S., Alothman, M. A., Eke, C., Al-Ghamdi, H., Aloraini, D. A., & Al-Buriahi, M. S. (2021). Simulating the radiation shielding properties of TeO2–Na2O–TiO glass system using PHITS Monte Carlo code. Computational Materials Science, 196, 110566. https://doi.org/10.1016/j.commatsci.2021.110566
- Alzahrani, J. S., Kavas, T., Kurtulus, R., & Al-Buriahi, M. S. (2021). Evaluations of physical and mechanical properties, and photon attenuation characteristics on lithium-germanate glass containing ZnO. Optik, 248. https://doi.org/10.1016/j.ijleo.2021.168078
- Beir, V. (1990). Health effects of exposure to low levels of ionizing radiation. Biological Effects of Ionizing Radiations, 22–45. https://doi.org/10.17226/1224. .
- Chilton, A. B., Shultis, J. K., & Faw, R. E. (1984). Principles of radiation shielding. Prentice-Hall.
- Cinan, Z. M., Baskan, T., Erol, B., Mutlu, S., Misirlioglu, Y., Yilmaz, S. S., & Yilmaz, A. H. (2021). Gamma irradiation, thermal conductivity, and phase change tests of the cement-hyperbranched poly amino-ester-block-poly cabrolactone-polyurathane plaster-lead oxide and arsenic oxide composite for development of radiation shielding material. International Journal of Energy Research, 45(15), 20729–20762. https://doi.org/10.1002/er.7136
- Cinan, Z. M., Erol, B., Baskan, T., Mutlu, S., Ortac, B., Yilmaz, S. S., & Yilmaz, A. H. (2022). Radiation shielding tests of crosslinked polystyrene-b-polyethyleneglycol block copolymers blended with nanostructured selenium dioxide and boron nitride particles. Nanomaterials, 12(3), 297. https://doi.org/10.3390/nano12030297
- Cinan, Z. M., Erol, B., Baskan, T., Mutlu, S., Yilmaz, S. S., & Yilmaz, A. H. (2021). Gamma irradiation and the radiation shielding characteristics: For the lead oxide doped the crosslinked polystyrene-b-polyethyleneglycol block copolymers and the polystyrene-b-polyethyleneglycol-boron nitride nanocomposites. Polymers, 13(19), 3246. https://doi.org/10.3390/polym13193246
- Donghui, Z., Hua, W., Guorong, C., Baccaro, S., Cecilia, A., Falconieri, M., & Pilloni, L. (2006). Gamma‐ray‐induced multi‐effect on properties of chalcogenide glasses. Journal of the American Ceramic Society, 89(11), 3582–3584. https://doi.org/10.1111/j.1551-2916.2006.01268.x
- El-Agawany, F. I., Kavaz, E., Perişanoğlu, U., Al-Buriahi, M., & Rammah, Y. S. (2019). Sm2O3 effects on mass stopping power/projected range and nuclear shielding characteristics of TeO2–ZnO glass systems. Applied Physics A, 125(12), 838. https://doi.org/10.1007/s00339-019-3129-0
- El-Agawany, F. I., Mahmoud, K. A., Kavaz, E., El-Mallawany, R., & Rammah, Y. S. (2020). Evaluation of nuclear radiation shielding competence for ternary Ge–Sb–S chalcogenide glasses. Applied Physics A, 126(4), 1–11. https://doi.org/10.1007/s00339-020-3426-7
- El-Qahtani, Z. M. H., Shaaban, E. R., & Soraya, M. M. (2021). Attenuation characteristics of high-energy radiation on As-Se-Sn chalcogenide glassy alloy. Chalcogenide Letters, 18(6), 311–326. http://chalcogen.ro/311_QahtaniZM.pdf.
- Gaikwad, D. K., Obaid, S. S., Sayyed, M. I., Bhosale, R. R., Awasarmol, V. V., Kumar, A., Shirsat, M. D., & Pawar, P. P. (2018). Comparative study of gamma ray shielding competence of WO3-TeO2-PbO glass system to different glasses and concretes. Materials Chemistry and Physics, 213, 508–517. https://doi.org/10.1016/j.matchemphys.2018.04.019
- Gokce, H. S., Yalcinkaya, C., & Tuyan, M. (2018). Optimization of reactive powder concrete by means of barite aggregate for both neutrons and gamma rays. Construction and Building Materials, 189, 470–477. https://doi.org/10.1016/j.conbuildmat.2018.09.022
- Guo, H., Wang, Y., Gong, Y., Yin, H., Mo, Z., Tang, Y., & Chi, L. (2016). Optical band gap and photoluminescence in heavily Tb3+ doped GeO2-B2O3-SiO2-Ga2O3 magneto-optical glasses. Journal of Alloys and Compounds, 686, 635–640. https://doi.org/10.1016/j.jallcom.2016.06.074
- Han, I., & Demir, L. (2009). Studies on effective atomic numbers, electron densities from mass attenuation coefficients in TixCo1-x and CoxCu1-x alloys. Nuclear Instruments & Methods in Physics Research Section B-Beam Interactions with Materials and Atoms, 267(21–22), 3505–3510. https://doi.org/10.1016/j.nimb.2009.08.022.
- Han, I., & Demir, L. (2010). Studies on effective atomic numbers, electron densities and mass attenuation coefficients in Au alloys. Journal of X-Ray Science and Technology, 18(1), 39–46. https://doi.org/10.3233/XST-2010-0238
- Hewak, D. W. (2011). The promise of chalcogenides. Nature Photonics, 5(8), 474. https://doi.org/10.1038/nphoton.2011.155
- Hsiao, C. L., Wu, K. H., & Wan, K. S. (2011). Effects of environmental lead exposure on T-helper cell-specific cytokines in children. Journal of Immunotoxicology, 8(4), 284–287. https://doi.org/10.3109/1547691X.2011.592162
- Hubbell, J. H., & Seltzer, S. M. (1995). Tables of x-ray mass attenuation coefficients and mass energy-absorption coefficients 1 keV to 20 meV for elements z = 1 to 92 and 48 additional substances of dosimetric interest (National Institute of Standards and Technology (NIST)). p. Medium: P; Size: 116 p.
- Hulbert, S. M., & Carlson, K. A. (2009). Is lead dust within nuclear medicine departments a hazard to pediatric patients? Journal of Nuclear Medicine Technology, 37(3), 170–172. https://doi.org/10.2967/jnmt.109.062281
- Issa, S. A. M. et al. (2019). Radiation shielding and mechanical properties of Al2O3-Na2O-B2O3-Bi2O3 glasses using MCNPX Monte Carlo code. Materials Chemistry and Physics, 223, 209–219. https://doi.org/10.1016/j.matchemphys.2018.10.064.
- Jackson, D. F., & Hawkes, D. J. (1981). X-ray attenuation coefficients of elements and mixtures. Physics Reports, 70(3), 169–233. https://doi.org/10.1016/0370-1573(81)90014-4
- Kaplan, M. F. (1989). Concrete radiation shielding: Nuclear physics, concrete properties, design and construction. Longman Scientific & Technical.
- Kavas, T., Alsufyani, S. J., Alrowaili, Z. A., Tamam, N., Kurtulus, R., Olarinoye, I. O., & Al-Buriahi, M. S. (2022). Influence of iron (III) oxide on the optical, mechanical, physical, and radiation shielding properties of sodium-barium-vanadate glass system. Optik, 257. https://doi.org/10.1016/j.ijleo.2022.168844
- Kebaili, I., Boukhris, I., Al-Buriahi, M. S., Alalawi, A., & Sayyed, M. I. (2021). Ge-Se-Sb-Ag chalcogenide glasses for nuclear radiation shielding applications. Ceramics International, 47(1), 1303–1309. https://doi.org/10.1016/j.ceramint.2020.08.251
- Kumar, A., Gaikwad, D. K., Obaid, S. S., Tekin, H. O., Agar, O., & Sayyed, M. I. (2020). Experimental studies and Monte Carlo simulations on gamma ray shielding competence of (30+x) PbO10WO3 10Na2O − 10MgO – (40-x) B2O3 glasses. Progress in Nuclear Energy, 119, 103047. https://doi.org/10.1016/j.pnucene.2019.103047
- Kurtulus, R., Kavas, T., Akkurt, I., Gunoglu, K., Tekin, H. O., & Kurtulus, C. (2021). A comprehensive study on novel alumino-borosilicate glass reinforced with Bi2O3 for radiation shielding applications: Synthesis, spectrometer, XCOM, and MCNP-X works. Journal of Materials Science-Materials in Electronics, 32(10), 13882–13896. https://doi.org/10.1007/s10854-021-05964-w
- Mahmoud, K. A., Sayyed, M. I., & Tashlykov, O. L. (2019). Gamma ray shielding characteristics and exposure buildup factor for some natural rocks using MCNP-5 code. Nuclear Engineering and Technology, 51(7), 1835–1841. https://doi.org/10.1016/j.net.2019.05.013
- Mahmoud, K. A., Tashlykov, O. L., El Wakil, A. F., & El Aassy, I. E. (2020). Aggregates grain size and press rate dependence of the shielding parameters for some concretes. Progress in Nuclear Energy, 118, 103092. https://doi.org/10.1016/j.pnucene.2019.103092
- Manjunatha, H. C. (2017). A study of gamma attenuation parameters in poly methyl methacrylate and Kapton. Radiation Physics and Chemistry, 137, 254–259. https://doi.org/10.1016/j.radphyschem.2016.01.024
- Mariselvam, K., & Liu, J. C. (2022). Concentration effect of Tm3+ ions doped B2O3-Li2CO3-BaCO3-CaF2-ZnO glasses: Blue laser and radiation shielding investigations. Optics and Laser Technology, 154, 108262. https://doi.org/10.1016/j.optlastec.2022.108262
- Mhareb, M. H. A., Zeama, M., Elsafi, M., Alajerami, Y. S., Sayyed, M. I., Saleh, G., Hamad, R. M., & Hamad, M. K. (2021). Radiation shielding features for various tellurium-based alloys: A comparative study. Journal of Materials Science: Materials in Electronics, 32(23), 26798–26811. https://doi.org/10.1007/s10854-021-07057-0
- Mikla, V. I., Turovci, J. M., Mikla, V. V., & Mehta, N. (2018). Molecular structure of Se-rich amorphous films. Progress in Solid State Chemistry, 49, 1–15. https://doi.org/10.1016/j.progsolidstchem.2017.10.001
- Mishra, M., Chauhan, R., Katiyar, A., & Srivastava, K. K. (2011). Optical properties of amorphous thin film of Se-Te-Ag system prepared by using thermal evaporation technique. Progress in Natural Science: Materials International, 21(1), 36–39. https://doi.org/10.1016/S1002-0071(12)60022-7
- Obaid, S. S., Gaikwad, D. K., & Pawar, P. P. (2018). Determination of gamma ray shielding parameters of rocks and concrete. Radiation Physics and Chemistry, 144, 356–360. https://doi.org/10.1016/j.radphyschem.2017.09.022
- Obaid, S. S., Sayyed, M. I., Gaikwad, D. K., & Pawar, P. P. (2018). Attenuation coefficients and exposure buildup factor of some rocks for gamma ray shielding applications. Radiation Physics and Chemistry, 148, 86–94. https://doi.org/10.1016/j.radphyschem.2018.02.026
- Ogawa, M., Nakajima, Y., Kubota, R., & Endo, Y. (2008). Two cases of acute lead poisoning due to occupational exposure to lead. Clinical Toxicology, 46(4), 332–335. https://doi.org/10.1080/15563650701816448
- Perisanoglu, U., El-Agawany, F. I., Kavaz, E., Al-Buriahi, M., & Rammah, Y. S. (2020). Surveying of Na2O3-BaO-PbO-Nb2O5-SiO2-Al2O3 glass-ceramics system in terms of alpha, proton, neutron and gamma protection features by utilizing GEANT4 simulation codes. Ceramics International, 46(3), 3190–3202. https://doi.org/10.1016/j.ceramint.2019.10.023
- Rammah, Y. S., Askin, A., Abouhaswa, A. S., El-Agawany, F. I., & Sayyed, M. I. (2019). Synthesis, physical, structural and shielding properties of newly developed B2O3–ZnO–PbO–Fe2O3 glasses using Geant4 code and WinXCOM program. Applied Physics A, 125(8), 523. https://doi.org/10.1007/s00339-019-2831-2
- Rammah, Y. S., El-Agawany, F. I., & El-Mesady, I. A. (2019). Evaluation of photon attenuation and optical characterizations of bismuth lead borate glasses modified by TiO2. Applied Physics A, 125(10), 727. https://doi.org/10.1007/s00339-019-3023-9
- Rammah, Y. S., Sayyed, M. I., Ali, A. A., Tekin, H. O., & El-Mallawany, R. (2018). Optical properties and gamma-shielding features of bismuth borate glasses. Applied Physics A, 124(12), 832. https://doi.org/10.1007/s00339-018-2252-7
- Şakar, E., Özpolat, Ö. F., Alım, B., Sayyed, M. I., & Kurudirek, M. (2020). Phy-X/PSD: Development of a user friendly online software for calculation of parameters relevant to radiation shielding and dosimetry. Radiation Physics and Chemistry, 166, 108496. https://doi.org/10.1016/j.radphyschem.2019.108496
- Schindler, C., Valov, I., & Waser, R. (2009). Faradaic currents during electroforming of resistively switching Ag–Ge–Se type electrochemical metallization memory cells. Physical Chemistry Chemical Physics, 11(28), 5974–5979. https://doi.org/10.1039/b901026b
- SCHOTT. Technicalproperties of Radiation Shielding Glasses | SCHOTT. Accessed 21 July 2022. https://www.schott.com/en-us/products/radiation-shielding-glasses-p1000330/technical-details?tab=a1e7a3e14e944b158807b6d8f7547d71
- Tekin, H. O., & Kilicoglu, O. (2020). The influence of gallium (Ga) additive on nuclear radiation shielding effectiveness of Pd/Mn binary alloys. Journal of Alloys and Compounds, 815, 152484. https://doi.org/10.1016/j.jallcom.2019.152484
- Tonguc, B. T., Arslan, H., & Al-Buriahi, M. S. (2018). Studies on mass attenuation coefficients, effective atomic numbers and electron densities for some biomolecules. Radiation Physics and Chemistry, 153, 86–91. https://doi.org/10.1016/j.radphyschem.2018.08.025
- Wood, J. I. (1982). Computational Methods in Reactor Shielding. Pergamon Press.
- Yankov, G., Dimowa, L., Petrova, N., Tarassov, M., Dimitrov, K., Petrov, T., & Shivachev, B. L. (2012). Synthesis, structural and non-linear optical properties of TeO2-GeO2-Li2O glasses. Optical Materials, 35(2), 248–251. https://doi.org/10.1016/j.optmat.2012.08.002