Abstract
United Nation’s sustainable goals place emphasis on renewable energy resources to transform the world into a sustainable one and enable it to have affordable energy. Of late alternative green fuels are becoming promising sources of fuel to address the energy crisis. Waste cooking oil (WCO) is the raw material used for biodiesel preparation, which can be employed as a fuel in compression ignition (CI) engines. This article investigates the dual impact of biodiesel, a green fuel and a renewable green additive (turpentine oil) on the performance and emissions of a CI engine. The studies were performed using a B20 (20% biodiesel + 80% diesel) WCO biodiesel fuel blend with 2.5% and 5% vol. of turpentine oil as an additive. The outcome of this research showed an improvement in brake thermal efficiency for the B20 biodiesel blend with a 5% turpentine oil additive. Turpentine oil additive promoted more complete combustion and produced lower carbon monoxide (CO), hydrocarbon (HC) and smoke emissions. However, the oxides of nitrogen (NOx) emissions increased as the turpentine oil composition increased from 2.5% to 5%, which is 4.5% more than B20. The outcome of this study can be extended to accomplish the objectives of sustainable development goal 7 (affordable and clean energy) and goal 13 (climate action) which are planned to be achieved by 2030.
SUBJECTS:
1. Introduction
The transportation sector operating on compression ignition (CI) engines with conventional fuels occupies a prominent position in the economy of a nation. However, they also contribute significantly on the emission front thereby degrading the environment. This limitation has compelled the researchers to look for alternate options for the functioning of CI engines to conform to the sustainable development goals set by the United Nations (Kalaimurugan et al., Citation2023; Kalyani et al., Citation2023; Mohammed et al., Citation2023; Nikkhah et al., Citation2023; United Nations, Citation2020).
One of the promising options considered by the researchers is the usage of alternative renewable fuels derived from green resources (Gad et al., Citation2023; Sayyed et al., Citation2023; Wong et al., Citation2023). Although considerable studies have been conducted on their usage in CI engines, their availability is the limiting factor in venturing into large scale commercialization. However, the fuel derived from waste cooking oil (WCO) presents a suitable alternative in partially fulfilling the goal of meeting the future needs. Several studies conducted on the usage of WCO in the blend form have revealed superior performance coupled with higher percentage of NOx emissions (Fayad et al., Citation2023). To overcome this limitation, researchers have used diethyl ether (Kumar & Murugesan, Citation2023), alcohols (Rangabashiam et al., Citation2023) and hydrogen peroxide (Mohan & Dinesha, Citation2022) have reported favorable results.
Biodiesel in its neat form exhibits higher viscosity coupled with higher density which cause poor atomization, air-fuel mixture formation and leads to incomplete combustion. Additionally, the engine performs with lower efficiency resulting in higher tailpipe emissions. Blending neat biodiesel with mineral diesel reduces viscosity and density with little improvement in the fuel quality. In addition to lower viscosity and lower density, fuel properties such as volatility, heating content and cetane number play a prominent role in engine combustion. Use of 10% diethyl ether as an additive in WCO B20 biodiesel blend reduced viscosity and improved cetene index. This promoted more complete combustion with 1.26% higher brake thermal efficiency with drastic reduction in tailpipe emissions (Naik et al., Citation2022). Chaudhary conducted tests on a diesel engine with WCO biodiesel and diethyl ether (DEE), investigated the performance and emission characteristics. Higher brake thermal thermal efficiency (BTE) and lower exhaust emissions were noted for the biodiesel blend with 15% DEE. Alcohols are additives that have a tendency to reduce the viscosity of biodiesel fuel. Kukana and Jakhar (Citation2022) reported lower viscosity for a composite biodiesel blend when n-propanol was added as an additive. The authors observed improved and reduced NOx emissions for composite biodiesel blend with 20% n-propanol additive. Similar results were observed by Vergel-Ortega et al. (Citation2021) for ethanol additives. Singh et al. (Citation2020) investigated the effect of n-butanol on the performance and emission characteristics of a diesel engine which was fueled with eucalyptus biodiesel-diesel blends. They reported that the adding n-butanol to biodiesel-diesel blends reduced NOx emissions when compared to diesel.
The use of plant-derived green additives is another way to enhance the combustion quality of CI engines. These additives are ecofriendly, nontoxic, renewable and antioxidant in nature. García et al. (Citation2017) tested the stability of biodiesel by adding bio-oil derived antioxidants. Bio-oil-derived antioxidants showed 175% improvement in oxidation stability of biodiesel. Turpentine oil additives improve lubricity and cold flow properties of biodiesel blends (Ballesteros et al., Citation2020; Zhang et al., Citation2022). Dueso et al. (Citation2018) extracted antioxidants from pine oil and used them as an additive with sunflower biodiesel. The testing of these blends was carried out in a diesel engine and results showed an improvement in BTE coupled with lower NOx and smoke opacity in comparison to neat diesel. Venkatesan and Nallusamy (Citation2020) investigated the combustion and emission characteristics of a twin cylinder tractor engine using pine oil-soapnut oil biodiesel blends. They reported better engine performance with lower emissions when biodiesel was blended with pine oil up to 50%. Saravanan et al. (Citation2020) carried out experimentation on a CRDI engine using 30% pine oil biodiesel blend at different fuel injector opening pressures. The test results showed that higher BTE and lower emissions at 350 bar injection pressure except NOx. Higher blends of turpentine oil additives significantly reduce viscosity and increase calorific value, leading to more complete combustion (Dubey & Gupta, Citation2018). Oxyfunctionalized turpentine can reduce the soot formation tendency due to improved volatility (Ballesteros et al., Citation2020). shows the summary of the literature related to the studies in which turpentine oil has been used as an additive. Although turpentine oil promotes more complete combustion due to its improved volatility, its lower cetane index limits its usage in a higher percentage.
Table 1. Summary of literature.
From the literature review, it can be inferred that researchers have used a higher percentage of turpentine oil which not only has a lower cetane index but also considerably adds to the cost factor. Considering the above factors in this study, a lower percentage of turpentine oil up to 5% by volume has been used as a renewable source additive with B20 WCO and experimentation has been performed to assess the performance and emissions from the engine.
2. Materials and method
2.1. Turpentine oil
Turpentine oil is also known as turpentine spirit, extracted from pine tree resins. It is generally made up of unsaturated isomers and bicyclic hydrocarbons mixtures namely α-pinene, β-pinene and δ-carene (Kadarohman et al., Citation2021). The chemical structure of turpentine oil is shown in . It is a colorless, flammable liquid with a unique odor with a general formula C10H16. Due to its antiseptic nature, turpentine oil is widely used as a cleaning agent. It is also used as a feedstock for organic synthesis. Due to its favorable physicochemical properties, turpentine oil can be used as an additive to CI engine fuels to enhance the combustion process.
Figure 1. Chemical structure of turpentine oil (Kadarohman et al., Citation2021).
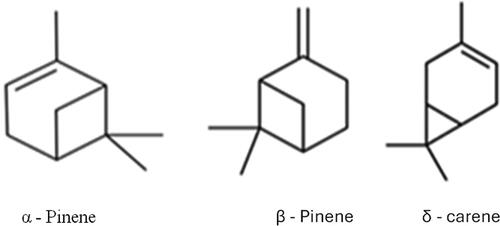
2.2. WCO biodiesel
WCO is one of the promising feedstocks of biodiesel due to its abundant availability which facilitates its efficient waste management. WCO has various kinds of fatty acids, which are suitable for biodiesel preparation. In this current work, readymade WCO biodiesel was purchased from the local Biofuel development center.
2.3. Fuel blend preparation
Literature review suggests that B20 biodiesel blend results in better performance with lower emissions (Dinesha et al., Citation2021). Hence, in this study, a standard test fuel of B20 WCO biodiesel blend has been selected. The fuel blend was prepared by mixing 80% WCO biodiesel with 20% mineral diesel. The turpentine oil additive has been added to B20 blend in proportions of 2.5% and 5% (by vol.). The test fuels have been abbreviated as B20T2.5 and B20T5. The properties of the fuel variants have been given in .
Table 2. Properties of WCO B20 and turpentine oil.
2.4. Experimental setup
The experimental investigation has been performed in a stationary single cylinder CI engine. The engine operates at 1500 rpm constant speed and delivers a peak power output of 5.2 kW. Starting from 25% load, the engine load was increased in increments of 25% up to the full loading condition. represents the test setup, and the specifications are presented in . The engine parameters such as engine speed and fuel consumption have been recorded using the engine control panel. The tailpipe emission concentrations were noted using AVL make five gas analyzer and smoke meter. The test procedure was repeated for all the fuel variants, and the results were compared with the baseline B20 operation. The error analysis of the dependent parameters used in the study was performed and tabulated in . The flow chart of the experimentation is depicted in .
Table 3. Description of the test setup.
Table 4. Values of uncertainties of the noted variables.
3. Results and discussion
A series of experimental trials were performed to assess the influence of turpentine oil additives on the performance and emissions of CI engine fueled with WCO B20. The BTE and tailpipe emissions such as CO, HC, CO2, NOx and smoke opacity were evaluated by comparing with B20 baseline operation.
3.1. Brake thermal efficiency
The effect of turpentine oil additive on BTE has been depicted in . The variation of BTE depends on various factors such as calorific value, volatility, cetane number and oxygen availability which promote more complete combustion (Kumar et al., Citation2018). The higher calorific value and higher volatility nature of turpentine oil enhance the combustion of B20 biodiesel, and the same is visible in the improvement of BTE (Saravanan et al., Citation2020). As the additive composition increased from 2.5% to 5%, the BTE values further increased. The study shows that turpentine oil has a positive impact on fuel combustion, hence, enhanced BTE was obtained. B20T5 blend resulted in 30.5% BTE, which was 2.9% higher as compared to B20.
3.2. Carbon monoxide (CO)
demonstrates the CO emission variation for the fuel variants at varying loads. The main cause of CO emissions is incomplete combustion of fuel. Local stoichiometric air fuel ratio, oxygen availability and auto ignition temperature of the fuel blend decide the combustion quality. Lower CO formation is an indication of improved combustion (Nagappan et al., Citation2022; Selvan et al., Citation2022). It is clear from the results that the use of turpentine oil results in lower CO emissions. When turpentine oil composition was increased to 5%, minimum CO emissions were obtained. Compared to B20, B20T5 has 36.3% lower CO emissions due to the better fuel blend quality with higher volatility and improved local stoichiometric mixture formation (Selvan et al., Citation2022).
3.3. Carbon dioxide (CO2)
Variation of CO2 formation from the engine combustion is illustrated in . As noticed in , a similar trend can be noted which can be attributed to the influence of turpentine oil on CO2 formation thereby enhancing the combustion in a positive manner. Higher formation of CO2 is an indication of superior combustion due to finer fuel atomization and lower viscosity of the fuel blend (Kumar et al., Citation2018; Nagappan et al., Citation2022; Selvan et al., Citation2022). As compared to B20, B20 with turpentine oil blends emit higher CO2 due to their higher volatility and lower auto ignition properties. The reduced carbon to hydrogen ratio of turpentine oil which influences volumetric efficiency might have contributed to the above phenomenon (Jeevanantham et al., Citation2020). From the graph, as the composition of turpentine oil increased from 0 to 5%, the CO2 has increased significantly. B20 with 5% turpentine oil additive has resulted in 12.2% higher CO2 in comparison to B20 fuel.
3.4. Oxides of nitrogen (NOx)
depicts the change of NOx emissions with varying load conditions. NOx emissions raise with the raise in load due to higher operating temperature of the engine (Kumar et al., Citation2018). The oxidation of N2 during combustion may be due to combustion temperature, oxygen availability, and resident time of the gases (Kumar et al., Citation2018; Mirhashemi & Sadrnia, Citation2020). Biodiesel fueled engines produce higher NOx emissions owing to the inbuilt oxygen present in the fuel. The B20T2.5 blend showed 10.5% lower NOx emissions as compared to B20. This may be due to the higher volatility and lower viscosity of the blend which in turn lowers the delay period leading to lower combustion temperature (Mirhashemi & Sadrnia, Citation2020; Selvan et al., Citation2022). When the turpentine oil composition was increased from 2.5% to 5%, the NOx emissions were increased by 6.7%, but it is 4.5% lower than B20. This may be attributed to the increased heat content and higher volatility which lead to higher combustion temperature due to more complete combustion (Mirhashemi & Sadrnia, Citation2020).
3.5. Hydrocarbon (HC)
The HC level in the exhaust gas raises as the engine load is increased for all test fuel variants and maximum concentration was observed at full load as shown in . This may be due to the higher equivalence ratio of the mixture (Kumar et al., Citation2018). B20 produced higher HC emissions as compared to B20T2.5 and B20T5 blends. These fuel blends exhibited lower HC emission characteristics due to the combined effect of lesser viscosity and higher volatility of turpentine oil. As the proportion of turpentine oil increased from 2.5% to 5%, minimum HC emission was obtained which was 50% lower than B20 (García et al., Citation2017).
3.6. Smoke opacity
depicts the smoke emission pertaining to engine load. Literature report that fuels with higher volatility show lower smoke emissions as compared to neat biodiesel blend (Ballesteros et al., Citation2020). Increasing the composition of turpentine oil in B20 leads to reduction in smoke emissions due to higher volatility of the blend, which has aided the fuel to undergo complete combustion (Kumar et al., Citation2018). Viscosity and volatility play a key role in the formation of smoke. The atomization and dispersion of injected fuel helps to achieve rapid combustion due to lower viscosity and higher volatility. Lower carbon to hydrogen ratio facilitates enhanced mixing of fuel and air due to improved volumetric efficiency (Jeevanantham et al., Citation2020). These phenomena have resulted in lower smoke formation. In this study, lower emission was obtained for B20T5 fuel, which was 14.7% less than B20 fuel.
4. Conclusion
In this research, an effort was made to assess the effect of turpentine oil additive with B20 biodiesel on the operating characteristics of a CI engine. From the study, following inference can be drawn:
The use of turpentine oil with biodiesel combination improves engine performance with significantly lower emissions. The B20 fuel with turpentine oil additive showed higher BTE as compared to B20. As the composition of turpentine oil increased from 0% to 5%, a maximum BTE of 30.5% was obtained for B20T5, which was 2.9% higher than B20. Lower NOx emissions were noted for B20T2.5 blend as compared to B20. When the turpentine oil composition was increased from 2.5% to 5%, NOx emission level was increased by 4.5% as compared to B20. CO, HC emissions and smoke opacity were reduced for the B20 fuel with turpentine oil additive. CO, HC emissions and smoke opacity were 3, respectively, lower by 6.3%, 50% and 14.7% for B20T5 as compared to B20. Emission of CO2 increases with B20T2.5 and B20T5 blends. A maximum reduction of CO2 was obtained for B20T5 blend, which was 12.2% lower than B20.
From the study, it can be suggested that the B20 WCO biodiesel with lower percentage of turpentine oil additive can be employed in a CI engine with the existing engines. As turpentine oil is a plant-based product its usage has the potential to replace the synthetic additives which are being currently used in engines. The outcome of this study can be extended to perform in depth analysis of the turpentine oil and adopt it as an additive in commercial applications. The study also provides a path to meet the objectives of sustainable development goals set by United Nations.
Credit author statement
Augustine B. V. Barboza: Conceptualization, Investigation, Formal Analysis, Writing—original. Sooraj Mohan: Visualization, Writing—review and editing. P. Dinesha: Supervision, Writing—review and editing.
Disclosure statement
The authors report there are no competing interests to declare.
Data availability statement
Data can be made available on a reasonable request.
Additional information
Funding
Notes on contributors
Augustine B. V. Barboza
Augustine B. V. Barboza, a post graduate in Mechanical Engineering is presently serving as Additional Professor in the Dept. of Mechanical and Industrial Engg. at Manipal Institute of Technology, which is affiliated to Manipal Academy of Higher Education, Manipal, India. His research interests are in the areas of Alternate Fuels, I C Engine Combustion and Pollution Control.
Sooraj Mohan
Sooraj Mohan completed his PhD in NOx emission control using Selective Catalytic Reduction from MAHE, Manipal, India. He is presently working as a teaching faculty in the Department of Mechanical Engineering at National Institute of Technology Goa, India. He has published more than 20 papers in critically-acclaimed, high-impact journals. His research areas focus mainly on bioenergy conversion systems, emission control from combustion gases, and CO2 capture and storage.
P. Dinesha
P. Dinesha is a Professor in the Department of Mechanical and Industrial Engineering, at Manipal Institute of Technology, Manipal Academy of Higher Education, Manipal, India. His research interests include waste to energy conversion, bio-lubricants, CO2 capture, Engine combustion, Alternative fuels, and pollution control. Professor Dinesha has published more than 75 papers in reputed international journals and conference proceedings.
References
- Abed, K. A., El Morsi, A. K., Sayed, M. M., El Shaib, A. A., & Gad, M. S. (2018). Effect of waste cooking-oil biodiesel on performance and exhaust emissions of a diesel engine. Egyptian Journal of Petroleum, 27(4), 985–989. https://doi.org/10.1016/j.ejpe.2018.02.008
- Ballesteros, R., García, D., Bustamante, F., Alarcón, E., & Lapuerta, M. (2020). Oxyfunctionalized turpentine: Evaluation of properties as automotive fuel. Renewable Energy, 162, 2210–2219. https://doi.org/10.1016/j.renene.2020.10.026
- Dinesha, P., Kumar, S., & Rosen, M. A. (2021). Effects of particle size of cerium oxide nanoparticles on the combustion behavior and exhaust emissions of a diesel engine powered by biodiesel/diesel blend. Biofuel Research Journal, 8(2), 1374–1383. https://doi.org/10.18331/BRJ2021.8.2.3
- Dubey, P., & Gupta, R. (2018). Influences of dual bio-fuel (Jatropha biodiesel and turpentine oil) on single cylinder variable compression ratio diesel engine. Renewable Energy, 115, 1294–1302. https://doi.org/10.1016/j.renene.2017.09.055
- Dueso, C., Muñoz, M., Moreno, F., Arroyo, J., Gil-Lalaguna, N., Bautista, A., Gonzalo, A., & Sánchez, J. L. (2018). Performance and emissions of a diesel engine using sunflower biodiesel with a renewable antioxidant additive from bio-oil. Fuel, 234, 276–285. https://doi.org/10.1016/j.fuel.2018.07.013
- Fayad, M. A., Sobhi, M., Chaichan, M. T., Badawy, T., Abdul-Lateef, W. E., Dhahad, H. A., Yusaf, T., Isahak, W. N. R. W., Takriff, M. S., & Al-Amiery, A. A. (2023). Reducing soot nanoparticles and NOx emissions in CRDI diesel engine by incorporating TiO2 nano-additives into biodiesel blends and using high rate of EGR. Energies, 16(9), 3921. https://doi.org/10.3390/en16093921
- Gad, M. S., Ağbulut, U., Afzal, A., Panchal, H., Jayaraj, S., Qasem, N. A. A., El-Shafay, A. S., Jayaraj, A., Qasem, N. A. A., & El-Shafay, A. S. (2023). A comprehensive review on the usage of the nano-sized particles along with diesel/biofuel blends and their impacts on engine behaviors. Fuel, 339, 127364. https://doi.org/10.1016/j.fuel.2022.127364
- García, M., Botella, L., Gil-Lalaguna, N., Arauzo, J., Gonzalo, A., & Sánchez, J. L. (2017). Antioxidants for biodiesel: Additives prepared from extracted fractions of bio-oil. Fuel Processing Technology, 156, 407–414. https://doi.org/10.1016/j.fuproc.2016.10.001
- Jeevanantham, A. K., Reddy, D. M., Goyal, N., Bansal, D., Kumar, G., Kumar, A., Nanthagopal, K., & Ashok, B. (2020). Experimental study on the effect of cetane improver with turpentine oil on CI engine characteristics. Fuel, 262, 116551. https://doi.org/10.1016/j.fuel.2019.116551
- Kadarohman, A., Khoerunnisa, F., Sapee, S., Eko Sardjono, R., Izzudin, I., Mamat, R., Yusop, A. F., Yusaf, T., & Hendrawan, E. (2021). The effect of oxygenated turpentine oil additive in diesel fuel on the performance and emission characteristics in one-cylinder DI engines. Designs, 5( 4), 73. https://doi.org/10.3390/designs5040073
- Kalaimurugan, K., Karthikeyan, S., Periyasamy, M., Mahendran, G., & Dharmaprabhakaran, T. (2023). Experimental studies on the influence of copper oxide nanoparticle on biodiesel-diesel fuel blend in CI engine. Energy Sources, Part A: Recovery, Utilization, and Environmental Effects, 45(3), 8997–9012. https://doi.org/10.1080/15567036.2019.1679290
- Kalyani, T., Prasad, L. S. V., & Kolakoti, A. (2023). Effect of triacetin as an oxygenated additive in algae biodiesel fuelled CI engine combustion, performance, and exhaust emission analysis. Fuel, 338, 127366. https://doi.org/10.1016/j.fuel.2022.127366
- Kukana, R., & Jakhar, O. P. (2022). Effect of ternary blends diesel/n-propanol/composite biodiesel on diesel engine operating parameters. Energy, 260, 124970. https://doi.org/10.1016/j.energy.2022.124970
- Kumar, D. S., & Murugesan, S. (2023). Experimental investigation on impact of diethyl ether additives with cashew nut shell liquid biodiesel blends on performance characteristics of engine operating at optimum conditions. Emission Control Science and Technology, 9(1), 66–75. https://doi.org/10.1007/s40825-022-00219-4
- Kumar, S., Dinesha, P., & Rosen, M. A. (2018). Cashew nut shell liquid as a fuel for compression ignition engines: A comprehensive review. Energy & Fuels, 32(7), 7237–7244. https://doi.org/10.1021/acs.energyfuels.8b00579
- Mirhashemi, F. S., & Sadrnia, H. (2020). NOx emissions of compression ignition engines fueled with various biodiesel blends: A review. Journal of the Energy Institute, 93(1), 129–151. https://doi.org/10.1016/j.joei.2019.04.003
- Mohammed, A. S., Atnaw, S. M., Ramaya, A. V., & Alemayehu, G. (2023). A comprehensive review on the effect of ethers, antioxidants, and cetane improver additives on biodiesel-diesel blend in CI engine performance and emission characteristics. Journal of the Energy Institute, 108, 101227. https://doi.org/10.1016/j.joei.2023.101227
- Mohan, S., & Dinesha, P. (2022). Emulsification of waste cooking oil biodiesel blend with hydrogen peroxide to assess tailpipe emissions and performance of a compression ignition engine. Heat Transfer, 51(4), 3721–3735. https://doi.org/10.1002/htj.22518
- Nagappan, M., Devaraj, A., Babu, J. M., Saxena, N. V., Prakash, O., Kumar, P., & Sharma, A. (2022). Impact of additives on combustion, performance and exhaust emission of biodiesel fueled direct injection diesel engine. Materials Today: Proceedings, 62, 2326–2331. https://doi.org/10.1016/j.matpr.2022.04.114
- Naik, D. B., Meivelu, U., Thangarasu, V., Annamalai, S., & Sivasankaralingam, V. (2022). Experimental and empirical analysis of a diesel engine fuelled with ternary blends of diesel, waste cooking sunflower oil biodiesel and diethyl ether. Fuel, 320, 123961. https://doi.org/10.1016/j.fuel.2022.123961
- Nikkhah, A., Bagheri, I., Psomopoulos, C., Payman, S. H., Zareiforoush, H., El Haj Assad, M., Bakhshipour, A., & Ghnimi, S. (2023). Sustainable second-generation biofuel production potential in a developing country case study. Energy Sources, Part A: Recovery, Utilization, and Environmental Effects, 45(3), 7785–7798. https://doi.org/10.1080/15567036.2019.1677805
- Rangabashiam, D., Jayaprakash, V., Ganesan, S., & Christopher, D. (2023). Investigation on the performance, emission and combustion pattern of research diesel engine fueled with higher alcohol and pongamia biodiesel blends. Energy Sources, Part A: Recovery, Utilization, and Environmental Effects, 45(2), 4777–4788. https://doi.org/10.1080/15567036.2019.1670760
- Saravanan, C. G., Kiran, K. R., Vikneswaran, M., Rajakrishnamoorthy, P., & Yadav, S. P. R. (2020). Impact of fuel injection pressure on the engine characteristics of CRDI engine powered by pine oil biodiesel blend. Fuel, 264, 116760. https://doi.org/10.1016/j.fuel.2019.116760
- Sayyed, S., Das, R. K., Kulkarni, K., Alam, T., & Eldin, S. M. (2023). Influence of additive mixed ethanol-biodiesel blends on diesel engine characteristics. Alexandria Engineering Journal, 71, 619–629. https://doi.org/10.1016/j.aej.2023.03.091
- Selvan, B. K., Das, S., Chandrasekar, M., Girija, R., Vennison, S. J., Jaya, N., Saravanan, P., Rajasimman, M., Vasseghian, Y., & Rajamohan, N. (2022). Utilization of biodiesel blended fuel in a diesel engine–Combustion engine performance and emission characteristics study. Fuel, 311, 122621. https://doi.org/10.1016/j.fuel.2021.122621
- Singh, R., Singh, S., & Kumar, M. (2020). Impact of n-butanol as an additive with eucalyptus biodiesel-diesel blends on the performance and emission parameters of the diesel engine. Fuel, 277, 118178. https://doi.org/10.1016/j.fuel.2020.118178
- United Nations. (2020). The sustainable development goals report 2020. https://unstats.un.org/sdgs/report/2020/. (Accessed on 15 May 2023).
- Venkatesan, V., & Nallusamy, N. (2020). Pine oil-soapnut oil methyl ester blends: A hybrid biofuel approach to completely eliminate the use of diesel in a twin cylinder off-road tractor diesel engine. Fuel, 262, 116500. https://doi.org/10.1016/j.fuel.2019.116500
- Vergel-Ortega, M., Valencia-Ochoa, G., & Duarte-Forero, J. (2021). Experimental study of emissions in single-cylinder diesel engine operating with diesel-biodiesel blends of palm oil-sunflower oil and ethanol. Case Studies in Thermal Engineering, 26, 101190. https://doi.org/10.1016/j.csite.2021.101190
- Wong, P. K., Chen, S. H., Ghadikolaei, M. A., Ng, K. W., Lee, S. M. Y., Xu, J. C., Lian, Z. D., Ren, M., Ning, Z., & Gali, N. K. (2023). Physical properties and structural characteristics of particulate matter emitted from a diesel engine fueled with biodiesel blends. Environmental Pollution (Barking, Essex: 1987), 333, 122099. https://doi.org/10.1016/j.envpol.2023.122099
- Zhang, X., Li, N., Han, S., Wei, Z., & Dai, B. (2022). Terpene resin prepared from renewable turpentine oil as a new type of cold flow improver for soybean biodiesel-diesel blends. Fuel, 320, 123844. https://doi.org/10.1016/j.fuel.2022.123844