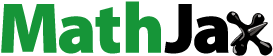
Abstract
The aim of current study was to isolate and evaluate the starch from Chenopodium album (LP) and Chenopodium album (LS) varieties for its physicochemical, particle size analysis, and textural properties. The starch isolated from C. album (LP) and C. album (LS) showed yield values of 37.59 and 47.30%, while amylose content of the mentioned varieties was found to be 16.75 and 19.11%, respectively. The starch from C. album (LS) showed higher values of water and oil binding capacities (178 and 225%, respectively), while as the corresponding values for C. album (LP) were found to be 169.5 and 222%. Laser diffraction particle size analysis showed unimodal particle size distribution profile with mean particle diameters of 10.52 and 10.64 μm for C. album (LP) and C. album (LS) starches, respectively. The granular size and shape of starches measured by Scanning Electron Microscope showed polygonal and angular shaped granules with respective average granule diameter of 1.021 μm and 1.033 μm in C. album (LP) and C. album (LS). Furthermore, starch from both the varieties showed firmer gel texture with hardness values of 35.57 and 46.73 gs. The analyzed properties of two varieties of C. album show its applicability in various food stuffs where desired consistency and firmness are vital requirements. Overall, it is concluded, C. album starches could be a non-conventional viable source with ample intrinsic properties, encouraging its suitability in food and non-food applications.
Public Interest Statement
Chenopodium album is an important valuable underutilized pseudocereal rich in mealy material. Botanically C. album belongs to the class Dicotyledoneae, genus Chenopodium, and family Chenopodiaceae. It is well known as pigweed in English. Its common name in Hindi is Bathua, and is locally notorious by diverse names like lambs quarter, fat–hen, white goosefoot, melde, and frost blite. Starch content of pseudo cereals varies from 51 to 61% and consists of uniform small granules less than 3 in diameters. Starch from pseudocereals displays A-type crystalline packing arrangement and annular polygonal granules. The main constituent of Chenopodium grain is the small-sized granule (< 1 pm) starch, having about 11% amylose. Amylose content affects the functional and physicochemical properties of starch, including its pasting, gelatinization, retrogradation, and swelling characteristics (Bao, Cai, & Corke, Citation2001). Such small-sized starch granules have unique applications such as dusting starches in candy dusting, and as flavor carriers.
Competing interests
The authors declare no competing interest.
1. Introduction
Chenopodium album is an important valuable underutilized pseudocereal rich in mealy material. Botanically C. album belongs to the class Dicotyledoneae, genus Chenopodium, and family Chenopodiaceae. It is well known as pigweed in English. Its common name in Hindi is Bathua, and is locally notorious by diverse names like lambs quarter, fat–hen, white goosefoot, melde, and frost blite. It is most widely distributed weeds in the world, cultivated throughout India particularly Western Rajasthan, Kulu valley, Shimla, and widely distributed in Europe, North America, Asia, and in different parts of Iran. Starch is composed of glucose residues, which are linked together by α-1, 4 bonds and branched via α-1, 6 bonds to form amylose and amylopectin. Amylose is chiefly linear with very few branches, while amylopectin is highly branched. In broad-spectrum, the branches in amylopectin are arranged in clusters rather than randomly thereby allowing the formation of double helices. This organization of amylopectin and amylose is the basis for the semi-crystalline structure of the starch granule (Ball et al., Citation1996). The ratio of amylose to amylopectin is one of the key factors determining industrially important properties. Starch content of pseudo cereals varies from 51 to 61% and consists of uniform small granules less than 3 in diameters (Atwell, Patrick, Johnson, & Glass, Citation1983). Starch from pseudocereals displays A-type crystalline packing arrangement and annular polygonal granules (Wright, Huber, Fairbanks, & Huber, Citation2002). Moreover, the starch gelatinizes at about 56–58°C and shows a single-stage swelling in the temperature range of 65–95°C, which along with its opaque nature makes it highly suitable for emulsion food products. The main constituent of Chenopodium grain is the small-sized granule (< 1 pm) starch, having about 11% amylose (Lorenz, Citation1990). Amylose content affects the functional and physicochemical properties of starch, including its pasting, gelatinization, retrogradation, and swelling characteristics (Bao et al., Citation2001). Such small-sized starch granules have unique applications such as dusting starches in candy dusting, and as flavor carriers (Zhao & Whistler, Citation1994). There are imperative investigations on the properties of starch contained in pseudocereals plants like buckwheat (Fagopyrum esculentum), quinoa (Chenopodium quinoa), and amaranth (Amaranthus spp.). Though there is no single exploration found till date on the separation and characterization of starch from pseudocereal C. album. Therefore, current study is an effort to carry out and investigate the various physicochemical, functional, particle size analysis, and gel textural properties of the starch extracted from C. Album (LP) and compared with the starch isolated from C. Album (LS) grains.
2. Materials and methods
2.1. Raw material
C. album grains are not usually available in market; the grains C. album (LP) were collected from local farmers of Sangrur, Punjab and C. album (LS) grains were brought from local farmers of Shimla, Himachal Pradesh, India.
2.2. Preparation of flour
The grains of C. album (LP) and C. album (LS) cultivars were manually cleaned to remove foreign objects, dried in oven at 40°C for 24 h, and milled in grinder (Sujata Mixing Grinder, India) to obtain flour. The samples were analyzed for carbohydrate, protein, fat, moisture, fiber, and ash content by employing standard methods of Association of Official Analytical Chemists (Citation1995). The analysis was conducted in triplicates.
2.3. Isolation of starch
The pre-standardized alkali-based process was followed for the extraction of starch with some modifications. Grains (100 g) were steeped in 100 ml of NaOH (0.25% w/v) at 4°C for 24 h. Fresh volume of sodium hydroxide was added to the solid part and stirred for another 1 h at ambient temperature. The steeped grains were ground in a blender (wet milling) for 3 min. The paste obtained was mixed with (1:5 ratio) of water to form a slurry. The slurry was filtered through a 200, 300, and 400 mesh sieve, respectively. The filtrate was centrifuged at 5,000 rpm for 10 min. The process of centrifugation and washing were repeated several times until the white starchy layer was obtained. The starch was dried for 6 h at 40°C and passed through a mesh 100 (149 μm).
2.4. Physicochemical properties
2.4.1. Color determination
The color value of starch were examined using Hunter colorimeter (Model I5 Green Macbeth, USA) in terms of lightness (L*) color (+a: red, a: green; +b: yellow, b: blue) were expressed by hunter values.
2.4.2. Amylose content
Amylose content of the samples was determined by the method of Morrison and Laignelet (Citation1983). Starch sample of 70 mg was mixed with 10 ml of urea and DMSO (Dimethyl Sulfoxide) solution in 1:9 ratio and heated at 100°C for 10 min with continuous stirring. The mixed samples were incubated for 1 h at 100°C and then cooled to room temperature. Addition of 0.5 ml solution of above mixed incubated sample was taken with subsequent addition of 25 ml distilled water and 1 ml solution of Iodine (I) and potassium Iodide (KI). Blank sample was also prepared with no addition of starch sample and absorbance was taken at 635 nm.(1)
(1)
(2)
(2)
2.4.3. Water/oil binding capacity (WBC/OBC)
Water binding capacity (WBC) and oil binding capacity (OBC) of the starches were determined using the method described by Yamazaki (Citation1953) as modified by Medcalf and Gilles (Citation1965). A suspension of 5 g starch (dry weight) in 75-ml distilled water was agitated for 1 h and centrifuged at 3,000 g for 10 min. The free water was removed from the wet starch, and the wet starch was then weighed. Similarly, oil binding capacity of the starch was estimated by the same procedure.(3)
(3)
2.4.4. Turbidity
The turbidity of suspensions of the starch samples was measured as described by Perera and Hoover (Citation1999). One percent aqueous starch suspension was heated in a boiling water bath for 1 h with constant stirring and then cooled for 1 h at 30°C. The samples were stored for 5 days at 4°C in a refrigerator under covered conditions to prevent loss/gain of moisture and turbidity was determined every 24 h by measuring absorbance at 640 nm (UV Spectrophotometer, Electronics Corp. of India Limited, Hyderabad, India) against water blank.
2.4.5. Sediment volume
Sediment volume of starches was determined as described by Singh, McCarthy, and Singh (Citation2006). One gram of starch on dry basis was weighed into beaker and 95 ml of distilled water was then added. The pH of the starch slurry was adjusted to 7.0 using 5% NaOH or 5% HCl, following which the slurry was cooked in a boiling water bath for 15 min. The total weight was made to 100 g with distilled water. The mixture was then stirred thoroughly and transferred to a 100-ml graduated cylinder. The cylinder was sealed with aluminum foil and the starch slurry was kept at room temperature for 24 h. The volume of the sediment, consisting of starch granules, was then measured.
3. Morphological properties
The granule shape as a major morphological characteristic of the sample was absorbed at a moisture content of 5–6%. Scanning electron micrographs (SEMs) were taken with a JEOL, Tokyo, Japan, Model No.JSM 6610LV. The starch samples were mounted on aluminum stub using a double-backed cellophane tape, coated in auto finer coater, JEOLJFC1600, with gold palladium (60:40, W/W). The starch samples were examined at magnifications of 5,000×.
4. Particle size distribution
The particle size distribution in term of percentage volume of the equivalent spheres was determined by Shimadzu SALD2300, laser diffraction particle size analyzer. The starch samples were dispersed in cuvette and fixed to the sample port of equipment. The starch sample was added to sample port drop wise till the obscuration to 20%. The percentage number of granules with different sizes was evaluated and the mean granule diameter of the particles was calculated as given by the software.
5. Textural properties of starch gels
The starch prepared in the RVA was poured into small aluminum canisters and stored at 4°C to allow gelation to occur. The gel formed in the canisters was evaluated for their textural properties by texture profile analysis (TPA) using the TA/XT2 (Stable MicroSystems, Surrey, England) texture analyzer as per the procedure followed by Sandhu and Singh (Citation2007) in corn starch. Each canister was placed upright on the metal plate and the gel was compressed at a speed of 0.5 mm/s to a distance of 10 mm with a cylindrical plunger (diameter = 5 mm). The compression was repeated twice to generate a force–time curve from which hardness (height of first peak) was determined. The negative area of the curve during retraction of the probe was termed adhesiveness. Three repeated measurements were performed for each sample and their average was taken.
6. Statistical evaluation
All the analysis were determined in the triplicates and statistical analysis was performed using Statistica-log software package version 7 (M/s. StatSoft Inc., OK, USA). The significant differences were obtained by a one-way analysis of variance (ANOVA) followed by Duncan’s multiple range test (DMRT) (p < 0.05). The mean value and standard deviation were reported for the statistical analysis.
7. Results and discussion
7.1. Chemical composition
The chemical composition of C. album (LP) and C. album (LS) flours is presented in Table . Carbohydrate content was found to be 54.61 and 52.15% in C. album (LS) and C. album (LP), respectively. The observed carbohydrate content was found to be lesser than the values reported by Repo-Carrasco, Espinoza, and Jacobsen (Citation2003) and Weber, Gentry, Kohlhepp, and McCrohan (Citation1991) for other pseudocereals. Protein, fat, and ash content of C. album (LP) were 13.12, 6.50, and 3.25%, while as the corresponding values for C. album (LS) were found to be 13.83, 7.40, and 3.50%, respectively. The results are in close conformity with the findings of Steffolani, León, and Pérez (Citation2013) in their study on flour characteristics of quinoa and kaniwa varieties.
Table 1. Chemical composition of C. album grains
7.2. Starch yield, purity, and color values
The yield of starch from C. album (LP) and C. album (LS) was 37.59 ± 3.60 and 47.30 ± 3.60% with purity of 98.66 ± 0.03 and 98.69 ± 0.03%, respectively. This variation in yield and purity values may be due to varietal difference and the method used for extraction of starch (Nwokocha & Williams, Citation2009). Moreover, the differences in purity might be due to the changes in composition of the starches. C. album (LP) starch showed lightness (L* value) of 96.2, whilst C. album (LS) starch showed (L* value) of 95.95. L* values greater than 90 gives a satisfactory whiteness for starch purity as reported earlier by Boudries et al. (Citation2009). Quality is affected by the color of starch which is due to the presence of polyphenolic compounds, ascorbic acid, and carotene. So any pigmentation in the starch reduces the quality and acceptability of starch product (Galvez & Resurreccion, Citation1993).
7.3. Physicochemical properties of starch
7.3.1. Amylose content and sediment volume
The amylose from C. album (LS) and C. album (LP) was found to be 16.75 ± 0.03% and 19.11 ± 3.60%, respectively as shown in Table . Amylose content was determined to reveal the internal quality of the starch and its functionality. This variation in amylose content is due to the factors such as botanical sources, climatic conditions, soil types, as well as harvest time affecting the variability in amylose/amylopectin ratio between samples and species (Noda, Tsuda, Mori, Takigawa, & Matsuura-Endo, Citation2004). Sediment volume is the ratio of the sediment gel to the dry weight of starch. Non-significant diversity in sediment volume was observed in C. album (LS) and C. album (LP) starches with the former showing higher values than the latter as depicted in Table . However, the values observed were found to be lesser than the findings reported on potato starch. The lower sediment value could be attributed to the smaller size of its polygonal and angular starch granules.
Table 2. Physicochemical properties of starches
7.3.2. Water/oil binding capacity (WBC/OBC)
Significant variation (p ≤ 0.05) in water binding capacity was observed among two varieties of C. album as shown in Table . The differences in WBC values may be due to the increased hydrophilicity/water binding sites among the starches (Wotton & Bamunuarachchi, Citation1978), and smaller particle size as observed by diffraction particle size analysis. The lower water binding capacity of C. album (LP) as compared to C. album (LS) is attributed due to tight organization of starch granules as reported by Soni, Sharma, Srivastava, and Gharia (Citation1990), while as high water binding capacity is indicative of loose association of native starch polymers or low lipid content (Nwokocha & Ogunmola, Citation2005). Similar findings were reported by Lorenz (5) in quinoa and Amaranthus starch. Oil binding capacity of C. album (LP) and C. album (LS) starches varied from 222 and 225%. The affinity of starch toward oil was found to be more than that for the water. These differences in OBC values of the starches might be due to the accessibility of hydrophobic sites within the starch molecules.
7.3.3. Turbidity
The turbidity values of starch suspensions are depicted in Figure . The turbidity value was found lower in C. album (LP) than C. album (LS) starch. The turbidity of C. album (LP) and C. album (LS) starches increased gradually during storage of starch gels at 4°C and thereafter tends to remain constant till late storage period. The differences in turbidity values of both the starch suspensions could be due to the varying amounts of amylose content. Turbidity development in starches during storage has been ascribed to the interaction of various factors, such as swelling of granules, granule remnants, leached amylose, and amylopectin, that lead to the development of junction zones which reflect or scatter a significant amount of light (Yu, Ma, Menager, & Sun, Citation2012).
8. Morphological characteristics
The granular shape, size, and morphology of the starch granules are shown in Figure . The starch granules were polygonal and angular in shape upon observation. Morphological examinations showed that size of starch granules was 1.021 μm and 1.033 μm in C. album (LP) and C. album (LS), respectively. Similar morphological observations of starch granules from quinoa seeds have been reported (Lindeboom, Citation2005; Ruales & Nair, Citation1994).
9. Particle size distribution
Particle size distribution profile has shown unimodal granular size for C. album (LP) and C. album (LS) starches. The results revealed the granule diameter of the C. album (LP) starch showed widest size distribution from 0.60–182.42 μm. The mean and median diameters of C. album (LP) starch were found to be 10.52 and 13.15 μm, respectively, whereas the same dimensions for C. album (LS) starch were 10.64 and 13.33 μm, respectively. Varrian-Marston and DeFrancisco (Citation1984) observed aggregates 18–20 μm in diameter in quinoa starch and reported that the perceptible anomalies in granule size may be due to aggregation. Moreover, Lorenz (Citation1990) reported that aggregates are typical of most starches that consist of small granules, such as quinoa, amaranth, and cow cockle.
10. Gel textural properties
Textural properties of starch gels with their subsequent values were determined using the texture analyzer presented in Table . Textural properties of the gel are indicative of how the starch would perform under various cooking applications. From the texture profile analysis, hardness and adhesiveness were chosen to represent the starch properties. The textural parameters of starch gels varied significantly at p ≤ 0.05 (Table ). Hardness is allied to the firmness of the starch gel, and adhesiveness to its competency to stick with other objects. Hardness of starch gels was found to be lower than earlier reports on buckwheat starch gel (Wende, Lin, & Corke, Citation1997) and higher than the reported values for wheat starch gel. The adhesiveness value of starch gel from C. album (LP) as shown in Figure was found to be higher; however, C. album (LS) starch adhesiveness values were similar to buckwheat starch and wheat starch gels (Wende et al., Citation1997). This difference in hardness and adhesiveness may be due to differences in the content of amylose and biological origin of starches. Mua and Jackson (Citation1997) observed starches that exhibit harder gels tend to have higher amylose content and longer amylopectin chains. Also the mechanical properties of starch gels depend upon various factors, including the rheological characteristics of the amylose matrix, the volume fraction, and the rigidity of the gelatinized starch granules, as well as the interactions between dispersed and continuous phases of the gel as reported by Biliaderis (Citation1998).
Table 3. Textural properties of starch gels
11. Conclusion
The physicochemical, particle size analysis, and textural properties of the starches were studied in C. album (LP) & C. album (LS) varieties. Starch was extracted from C. album grains with adequate yield and acceptable purity. The extracted starch from C. album (LP) & C. album (LS) varieties has shown higher water/oil binding capacity and formation of firmer starch gels with higher hardness and adhesiveness. High amylose may be responsible for increased hardness and adhesiveness of C. album starch gels. The starch granules were polygonal and angular in shape with smooth surface. Hence, the seeds of C. album besides having high amount of starch presently go as waste and can have a great potential to be used in food industry either for the purpose of formulating newer products or can be used for replacement of conventional starches in food products. Furthermore, the properties could be commercially tapped and have a great potential in both food and non-food applications.
Additional information
Funding
Notes on contributors
Romee Jan
Romee Jan is a doctoral research fellow at Department of Food Engineering & Technology, Sant Longowal Institute of Engineering and Technology, Longowal, Punjab, India, specializing in extruded products and processing of pseudocereals. She has three research papers to her credit.
D.C. Saxena
D.C. Saxena is presently working as a professor and head at Department of Food Engineering & Technology, Sant Longowal Institute of Engineering and Technology, Longowal, Punjab, India. He has more than 30 International research papers to his credit.
Sukhcharn Singh
Sukhcharn Singh is presently working as a professor at Department of Food Engineering & Technology, Sant Longowal Institute of Engineering and Technology, Longowal, Punjab, India. He has more than 20 International research papers to his credit.
References
- Association of Official Analytical Chemists . (1995). Official methods of analysis . Washington, DC: Author.
- Atwell, W. A. , Patrick, B. M. , Johnson, L. A. , & Glass, R. W. (1983). Characterization of quinoa starch. Cereal Chemistry , 60 , 9–11.
- Ball, S. , Guan, H. P. , James, M. , Myers, A. , Keeling, P. , Mouille, G. , … Preiss, J. (1996). From glycogen to amylopectin: A model for the biogenesis of the plant starch granule. Cell , 86 , 349–352.10.1016/S0092-8674(00)80107-5
- Bao, J. S. , Cai, Y. , & Corke, H. (2001). Prediction of rice starch quality parameters by near infrared reflectance spectroscopy. Journal of Food Science and Technology , 66 , 936–939.
- Biliaderis, C. G. (1998). Structures and phase transitions of starch polymers. In R. H. Walker (Ed.), Polysaccharide association structures in food (pp. 57–168). New York, NY: Marcel Dekker.
- Boudries, N. , Belhaneche, N. , Nadjemi, B. , Deroanne, C. , Mathlouthi, M. , Roger, B. , & Sindic, M. (2009). Physicochemical and functional properties of starches from sorghum cultivated in the Sahara of Algeria. Carbohydrate Polymers , 78 , 475–480.10.1016/j.carbpol.2009.05.010
- Galvez, F. C. F. , & Resurreccion, A. V. A. (1993). The effects of decortication and method of extraction on the physical and chemical properties of starch from mungbean (Vigna radiata (L.) wilczec). Journal of Food Processing and Preservation , 17 , 93–107.10.1111/jfpp.1993.17.issue-2
- Lindeboom, N. (2005). Studies on the characterization, biosynthesis and isolation of starch and protein from quinoa (Chenopodium quinoa Willd.) . Saskatoon: University of Saskatchewan.
- Lorenz, K. (1990). Quinoa (Chenopodium quinoa) starch—Physico-chemical properties and functional characteristics. Starch - Stärke , 42 , 81–86.10.1002/(ISSN)1521-379X
- Medcalf, D. G. , & Gilles, K. A. (1965). Wheat starches. I. Comparison of physicochemical properties. Cereal Chemistry , 42 , 558–568.
- Morrison, W. R. , & Laignelet, B. (1983). An improved colorimetric procedure for determining apparent and total amylose in cereal and other starches. Journal of Cereal Science , 1 , 9–20.10.1016/S0733-5210(83)80004-6
- Mua, J. P. , & Jackson, D. S. (1997). Relationships between functional attributes and molecular structures of amylose and amylopectin fractions from corn starch. Journal of Agricultural and Food Chemistry , 45 , 3848–3854.10.1021/jf9608783
- Noda, T. S. , Tsuda, S. , Mori, M. , Takigawa, S. , & Matsuura-Endo, C. (2004). The effect of harvest dates on the starch properties of various potato cultivars. Food Chemistry , 86 , 119–125.10.1016/j.foodchem.2003.09.035
- Nwokocha, L. M. , & Ogunmola, G. B. (2005). Isolation and characterization of starch from Treculia Africana Dcne (African breadfruit) seeds. Nigerian Journal of Science , 39 , 73–79.
- Nwokocha, L. M. , & Williams, P. A. (2009). Some properties of white and yellow plantain (Musa paradisiaca, Normalis) starches. Carbohydrate Polymers , 76 , 133–138.10.1016/j.carbpol.2008.10.012
- Perera, C. , & Hoover, R. (1999). Influence of hydroxypropylation on retrogradation properties of native, defatted and heat-moisture treated potato starches. Food Chemistry , 64 , 361–375.10.1016/S0308-8146(98)00130-7
- Repo-Carrasco, R. , Espinoza, C. , & Jacobsen, S. E. (2003). Nutritional value and use of the Andean crops quinoa (Chenopodium quinoa) and kañiwa (Chenopodium pallidicaule). Food Reviews International , 19 , 179–189.10.1081/FRI-120018884
- Ruales, J. , & Nair, B. M. (1994). Effect of processing on in vitro digestibility of protein and starch in quinoa seeds. International Journal of Food Science and Technology , 29 , 449–456.
- Sandhu, K. S. , & Singh, N. (2007). Some properties of corn starches II: Physicochemical, gelatinization, retrogradation, pasting and gel textural properties. Food Chemistry , 101 , 1499–1507.10.1016/j.foodchem.2006.01.060
- Singh, J. , McCarthy, O. , & Singh, H. (2006). Physico-chemical and morphological characteristics of New Zealand Taewa (Maori potato) starches. Carbohydrate Polymers , 64 , 569–581.10.1016/j.carbpol.2005.11.013
- Soni, P. L. , Sharma, H. W. , Srivastava, H. C. , & Gharia, M. M. A. (1990). Physicochemical properties of Canna edulis starch—Comparison with maize starch. Starch - Stärke , 42 , 460–464.10.1002/(ISSN)1521-379X
- Steffolani, M. E. , León, A. E. , & Pérez, G. T. (2013). Study of the physicochemical and functional characterization of quinoa and kañiwa starches. Starch - Stärke , 65 , 976–983.10.1002/star.v65.11-12
- Varriano-Marston, E. , & DeFrancisco, A. (1984). Ultrastructure of quinoa fruit (Chenopodium quinoa Willd.). Food Microstructure , 3 , 165–173.
- Weber, C. W. , Gentry, H. S. , Kohlhepp, E. A. , & McCrohan, P. R. (1991). The nutritional and chemical evaluation of Chia seeds. Ecology of Food and Nutrition , 26 , 119–125.10.1080/03670244.1991.9991195
- Wende, L. , Lin, R. , & Corke, H. (1997). Physicochemical properties of common and tartary buckwheat starch. Cereal Chemistry , 74 , 79–82.
- Wotton, M. , & Bamunuarachchi, A. (1978). Water binding capacity of commercial produced native and modified starches. Starch/Starke , 33 , 159–161.
- Wright, K. H. , Huber, K. C. , Fairbanks, D. J. , & Huber, C. S. (2002). Isolation and characterization of Atriplex hortensis and sweet Chenopodium quinoa starches. Cereal Chemistry , 79 , 715–719.10.1094/CCHEM.2002.79.5.715
- Yamazaki, W. T. (1953). An alkaline water retention capacity test for the evaluation of cookies in baking potentialities of soft winter wheat flours. Cereal Chemistry , 30 , 242–246.
- Yu, S. , Ma, Y. , Menager, L. , & Sun, D. (2012). Physicochemical properties of starch and flour from different rice cultivars. Food Bioprocess Technology , 5 , 626–637.10.1007/s11947-010-0330-8
- Zhao, J. , & Whistler, R. L. (1994). Spherical aggregates of starch granules as flavor carriers. Food Technology , 48 , 104–108.