Abstract
Meat consumers are very concerned about the quality and tenderness of meat. Meat tenderness generally depends upon connective tissue, sarcomere length, and the proteolytic potential of muscle. Different physical and chemical methods are used to assess the tenderness of meat. Protease treatment is an efficient method used for meat tenderization. In the food industry, different proteases such as bromelain, papain, ficin, actinidin, and calpain are widely used for proteolytic degradation, to improve meat tenderness. Two structural components determine the toughness of meat, connective tissues composed of structural proteins and post-mortem changes in the sarcomere. Proteases play an important role in degrading the structural proteins in the connective tissues, thus reducing toughness of meat. Bacterial proteases are also used in meat tenderization. Bacterial proteases show effective proteolytic degradation of elastin and collagen, but have negligible or no effect in degrading myofibrillar proteins. The present review highlight the importance of plant and bacterial enzymes with special reference to meat tenderization.
Public Interest Statement
The use of plant and bacterial enzymes are the useful method for the meat tenderization. There are many methods of tenderizing meat, chemically or physically but the popular method is the use of proteolytic enzymes. This review has innovation for the researchers and scientists in the field of enzymes with reference to meat tenderization. The combined use of plant and bacterial proteases was first time present together in a paper.
Competing Interest
The authors declare no competing interest.
1. Introduction
The palatable quality of meat is influenced by several factors, among which meat tenderness is considered the most important determinant of consumer preferences. Meat tenderness generally depends upon connective tissues, sarcomere length, and the extent of proteolytic degradation of muscles (Kemp & Parr, Citation2012). In contrast, meat toughness is an undesirable attribute of palatable meat quality for consumers (Kemp, Sensky, Bardsley, Buttery, & Parr, Citation2010). Toughness in meat primarily occurs because of the actomyosin effect (changes in myofibrillar proteins) or the background effect (because of the amounts of connective tissues or stromal proteins) (Chen, He, Jiao, & Ni, Citation2006). The quality and tenderness of meat and meat products can be improved in several ways using physical and chemical treatments. However, all treatments commonly emphasize on disrupting or degrading the connective tissues and myofibrillar proteins.
Myofibrillar toughness is caused by the onset of rigor mortis in slaughtered meat, and enzymatic breakdown of contractile proteins in post-slaughtered meat muscles causes tenderization of the meat (Naveena et al., Citation2011). In older animals, the formation of stronger and complex collagen cross-links in connective tissue increases; proteolytic enzymes can degrade connective tissue composed of 80% collagen, to tenderize meat (Gelse, Pöschl, & Aigner, Citation2003).
Meat tenderness is the most important attribute that governs consumer acceptability, consumer satisfaction, and recurrent purchasing trends and market value for meat and meat products (Grunert, Bredahl, & Brunsø, Citation2004; Mennecke, Townsend, Hayes, & Lonergan, Citation2007). The factors that influence the toughness of meat also contribute to the tenderness of meat. Meat tenderness depends on the type of muscle, pre- and post-slaughter factors, and postmortem pH and temperature (Anderson et al., Citation2012). The chemical composition, structure and amount of connective tissue, generally depends on the age of the animal and the specific muscle types, also affect meat tenderness (Bolumar, Enneking, Toepfl, & Heinz, Citation2013).
The use of exogenous proteases for meat tenderization is a relatively progressive method to improve meat quality. There are five exogenous proteolytic enzymes, plant proteases (papain, bromelain, and ficin), and proteases from Aspergillus oryzae and Bacillus subtilis, which have been approved as generally regarded as safe (GRAS) for use in the meat industry by the US Department of Agriculture (Ha, Bekhit, Carne, & Hopkins, Citation2012; Ketnawa & Rawdkuen, Citation2011). These proteolytic enzymes are mixed with the meat to breakdown the proteins in muscle and hydrolyze collagen and elastin, which helps in meat tenderization (Rawdkuen, Jaimakreu, & Benjakul, Citation2013). The use of enzymes reduces the amount of connective tissues and does not breakdown myofibrillar proteins. Papain and bromelain are the most commonly used plant enzymes for meat tenderization (Liu, Liao, Qi, & Tang, Citation2008). As meat tenderizers, proteolytic enzymes are best suited for degradation of collagen and elastin in connective tissue at relatively low pH and low temperature (Ryder, Ha, Bekhit, & Carne, Citation2015). The objective of this review was to gather the latest findings regarding the plant and bacterial enzymes which are used for the meat tenderization. Although the concept for the use of proteolytic enzymes is not new but the synergism between plants and bacterial enzymes added the value to this review.
The structure of myosin and actin filaments is affected by the plant proteases, papain, bromelain, and ficin (Wada, Suzuki, Yaguti, & Hasegawa, Citation2002). Ketnawa, Rawdkuen, and Chaiwut (Citation2010) depicted that collagen from beef and giant catfish skin is degraded by bromelain obtained from pineapple peels. The plant proteases are better than bacterial enzymes, because of safety, standard problems; high concentrations of plant proteases can cause meat deformation (Chen et al., Citation2006). Moreover, the tenderness of meat is also assessed by activity of enzymes estimation, myofibrillar fragmentation index, hydroxyproline measurement, and scanning electron microscopic. Plant proteases also used to improve tenderization of meat by biochemical changes as well as micro structural changes (Maiti, Ahlawat, Sharma, & Khanna, Citation2008).
Additionally, tenderization can be achieved by application of electric current, dissipation of energy at the beginning of muscle contraction, and by changing the process of rigor mortis, such that rigor mortis sets in while the muscles are still warm. It shows that tenderization of meat has been started at a definite rate. Thus, temperature conditions and electric stimulation can be used to reduce the cold shortening. The methods employed for meat tenderization play a role in providing good efficiency and high quality of meat and meat products (Breidenstein & Carpenter, Citation1983). The enzymatic mechanism of protein hydrolysis by cysteine proteases is shown in Figure .
Figure 1. Enzymatic mechanism of protein hydrolysis by cysteine proteases (Bekhit et al., Citation2014; Grzonka et al., Citation2007).
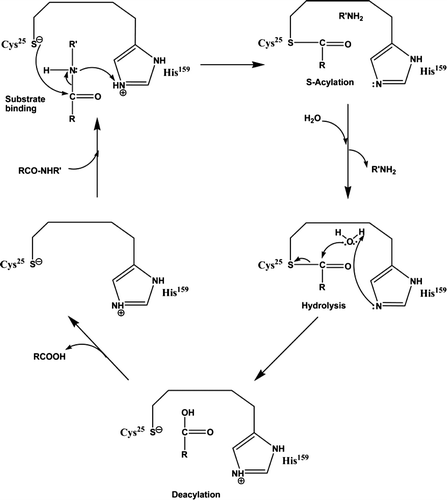
2. Meat tenderization with plant proteases
The process of meat tenderization is essentially an enzymatic degradation, and proteolytic enzymes in meat are responsible for tenderization during aging. However, plant or microbial enzymes can be exogenously added when additional tenderization is necessary (Lantto et al., Citation2009). Commercial meat tenderization is usually achieved using exogenously added proteolytic enzymes from plants, such as papain, bromelain, and ficin, as well as bacterial collagenase (Abdel-Naeem & Mohamed, Citation2016). Garg and Mendiratta (Citation2006) reported that the proteases derived from ginger rhizome and fruits of Cucumis trigonus Roxb plant were also effective in tenderization of meat. The connective tissues and muscle proteins are easily digested by the exogenously added proteases (Abdel-Naeem & Mohamed, Citation2016; Grzonka, Kasprzykowski, & Wiczk, Citation2007).
The myofibrillar breakdown starts after activation of the enzymatic system and includes the proteins troponin-1, troponin-t, desmin, vinculin, meta-vincilin, dystrophin, nebulin, and titin (Koohmaraie, Citation1996). When meat tenderization occurs from z-to z-line attachment-and M-line attachments in sarcolemma with the help of costameric proteins and the elastic protein titin, degradation of three important cytoskeleton structures occurs (Taylor, Geesink, Thompson, Koohmaraie, & Goll, Citation1995). In muscles, these proteolytic enzymes have a significant role in post-mortem proteolysis and meat tenderization (Koohmaraie & Geesink, Citation2006). Cathepsins were the first enzymes used in meat tenderization, after which, calpain was introduced owing to its efficiency in changing the Z-line density seen in post-mortem, although it was not initially related to meat tenderization (Taylor et al., Citation1995).
The three familiar tenderizing enzymes from plants, i.e. papain, bromelain, and ficin are obtained from papaya, pineapple, and fig, respectively. The pH, temperature, and strength of hydrolysis of various enzymes in proteolytic degradation of myofibrillar proteins and collagen are summarized in Table . The activity and application of different plant and bacterial proteases is shown in Table .
Table 1. pH, Temperature (0C) and strength of hydrolysis of myofibrillar proteins and collagen by various enzymesTable Footnote1
Table 2. The activity and application of different plant proteases
3. Papain
Papain is an important plant protease derived from the latex of the papaya fruit. Latex is obtained by scoring, and then allowing the latex to dry on the fruit, to give crude material. Papain is purified by reducing contaminating agents and further extraction. This enzyme is stable at high temperatures and pressure and is inactivated in extreme conditions at 900 mpa, 80°C degree for 22 min. Papain is used in the meat industry as a tenderizer, owing to its proteolytic effect, as well as in beer making as an additive in flour (Starley, Mohammed, Schneider, & Bickler, Citation1999). It has the ability to hydrolyze larger protein molecules into smaller peptides and amino acids. For many years, papain has been used to breakdown tough fibers (Eshamah, Han, Naas, Acton, & Dawson, Citation2014). Moreover, papain can tenderize the meat surface and develop characteristic “mushiness” (Islam & Molinar-Toribio, Citation2013). The physiological role of papain in plants is to protect them from insects (Konno et al., Citation2004).
The three dimensional structure for papain has been determined (Kamphuis, Kalk, Swarte, & Drenth, Citation1984). Broad-spectrum enzymatic activity has been shown by papain in the pH range 5–8 and at 65°C temperature (Smith & Hong-Shum, Citation2003). Berger and Schechter (Citation1970) demonstrated that papain has a specificity for amino acids with aromatic side chains such as Phe and Tyr at the P2 position. The synthetic peptides and inhibitors in mapping are the active sites of papain; within the active site, Cys25 and His159 are two of the essential residues for the protease activity (Bekhit, Hopkins, Geesink, Bekhit, & Franks, Citation2014). The schematic diagram for the effect of papain in either active or inactive form during ante-mortem and post-mortem conditions is shown in Figure .
Figure 2. Schematic diagram for the effect of papain in either active or inactive form in ante-mortem and post-mortem conditions (Bekhit et al., Citation2014; Dransfield & Etherington, Citation1981).
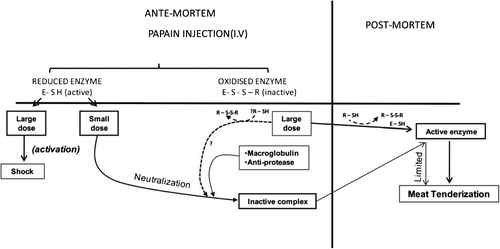
4. Bromelain
Bromelain is a proteolytic enzyme and is obtained from the root of the pineapple plant after harvesting (Fileti, Fischer, & Tambourgi, Citation2010). The juice contains bromelain enzyme in soluble form. The processing involves precipitation of enzymes for further purification. Bromelain breaks down myofibrillar proteins and collagen and causes over-tenderization of meat. The use of bromelain in processing adult beef showed the best results at 10 mg/100 g meat, for 24 h at 4°C, followed by increasing the temperature at the rate of 1°C/min, until it reached 70°C. Bromelain is important for tenderization of meat in industries with controlled environment, and is useful for assurance of the microbiological quality and purity. Bromelain is commercially available in powdered form. It is estimated that 95% of the enzymes used in the United States are obtained from plant proteases like papain and bromelain, whereas microbially derived tenderizers are not used widely (Ionescu, Fillit, Jaffrezic-Renault, & Cosnier, Citation2008).
Reverse micellar extraction is used for separation and purification of bromelain from pineapple. The commercially obtained stem bromelain was also compared with the purified bromelain in meat tenderization. There was high bromelain recovery and purification in the reverse micellar extraction technique. In reverse micellar extraction, the toughness of meat was also reduced as compared to control (Chaurasiya, Sakhare, Bhaskar, & Hebbar, Citation2015).
5. Ficin and actinidin
Ficin is a well-known plant protease used in meat tenderization (Maróstica & Pastore, Citation2010). Ficin is a sulfhydral or cysteine protease commonly obtained from Ficus carica (Fig. tree) that enhances the solubility of muscle proteins (Ramezani, Aminlari, & Fallahi, Citation2003). Ficin is an endoproteolytic enzyme present in the latex of Fig. trees (F. carica and F. glabrata). In 2008, ficin obtained from F. racemosa has a molecular weight of protein has 44.5 KDa, and shows maximum activity in the optimal pH range of 4.5–6.5 at 60°C. These properties make ficins a beneficial class of plant proteases for use in meat tenderization.
Actinidin is also a novel sulfhydryl protease extracted from gooseberry or the kiwi fruit. It has a molecular weight of 32 kDa. It is used commercially in meat industry to tenderize meat (Varughese, Su, Cromwell, Hasnain, & Nguyen Huu Xuong, Citation1992) and enhance the chemical processes related to degradation of the myofibrillar proteins into peptides. It is also involved in the activation of m-calpain throughout postmortem ageing (Ha et al., Citation2012). Actinidin has many applications in the food industry, because of its advantages over other plant proteases such as papain and ficin. Actinidin shows mild tenderizing activity even at high concentrations, preventing surface mushiness. It has a relatively low inactivation temperature (60°C), which makes it easier to control the tenderization process without overcooking (Eshamah et al., Citation2014; Tarté, Citation2009).
6. Calpain
Calpain is an important enzyme that is chiefly used for degradation of myofibrillar proteins. It also aids in meat tenderizing and improves water holding capacity during postmortem aging (Huff-Lonergan & Lonergan, Citation2005; Lonergan, Huff-Lonergan, Wiegand, & Kriese-Anderson, Citation2001). During postmortem refrigerated storage, calpain oxidation may adversely affect its proteolytic activity and negatively influence the quality of fresh meat (Zhang, Xiao, & Ahn, Citation2013).
The calpain system consists of three members—m-calpain, μ-calpain, and calpastatin, which is the calpain-specific endogenous inhibitor (Goll, Thompson, Li, Wei, & Cong, Citation2003; Wendt, Thompson, & Goll, Citation2004). In the presence of calcium, calpains autolyze, and this autolysis is indication of their proteolytic activation during postmortem changes in muscles (Geesink & Koohmaraie, Citation1999). Both m-calpain and μ-calpain are cysteine proteases, and their proteolytic activity is affected by oxidation, which can influence the quality of fresh meat (Zhang et al., Citation2013).
Meat tenderness undergoes changes after slaughtering due to the activity of the endogenous calpains and calpastatin. These calcium-dependent proteases degrade the myofibrillar proteins tropomyosin, roponin T, troponin I, C-protein, connectin, titin, vinculin, and desmin. Calpains are inactivated by calpastastin and decreases myofibrillar breakdown and decreases tenderness of meat (Cheret, Delbarreladrat, Lamballerieanton, & Verrezbagnis, Citation2007; Gerelt, Rusman, Nishiumi, & Suzuki, Citation2005).
The endogenous concentration of these enzymes in meat influences meat quality (Koohmaraie, Shackelford, Muggli-Cockett, & Stone, Citation1991). Calpain is involved in postmortem proteolysis and meat tenderization in domesticated animals (Huff-Lonergan & Lonergan, Citation2005; Koohmaraie, Citation1992).
Although calpains improve the tenderization of meat, increased expression of the CAST gene decreases proteolysis and increases toughness of meat. In contrast, the calpain genes CAPN1 and CAPN2 are involved in the breakdown of myofibrillar proteins (Huff-Lonergan et al., Citation1996). CAPN1 plays a significant role in postmortem muscle proteolysis and in tenderization of meat (Kemp et al., Citation2010). In bovine and ovine muscles, activity of CAPN2 is low (Camou et al., Citation2007; Veiseth, Shackelford, Wheeler, & Koohmaraie, Citation2001). The most important musclespecific calcium-dependent cysteine protease is the CAPN3 which has two structural domains called ISI and IS2 and the muscle specific proteins bind at N2 line region where proteolysis occurs (Geesink, Taylor, & Koohmaraie, Citation2005; Kemp et al., Citation2010).
7. Bacterial enzymes
Bacterial strains with proteolytic activity play an important role in the degradation of proteins in meat and meat products (Bekhit, Citation2010). B. subtilis contains two major proteases, subtilisin and neutral protease. The US Food and Drug Administration (FDA) has approved the GRAS status of these bacterial strains (FDA, Citation2001). The relative specific activity and low inactivation temperature of the bacterial proteases make them suitable for meat tenderization. Naveena, Mendiratta, and Anjaneyulu (Citation2004) reported that the enzyme alkaline elastase, obtained from the alkalophilic Bacillus sp., breaks down the collagen, elastin, and myofibrillar proteins in tenderized meat. Alkaline elastase showed optimal activity at pH range 5.5–6.0 and temperature range 10–50°C. The hydrolytic activity of bacterial proteases in myofibrillar proteins is low as compared to that of the plant proteases; however, the hydrolytic activity of bacterial proteases in mediating collagen degradation was found to be intermediate to the hydrolytic activities of papain and bromelain (Yeh, Yang, & Tsai, Citation2002).
The commercially available bacterial proteases are obtained from A. oryzae. Payne (Citation2009) reported that these proteases are stable over a wide range of pH and below 70°C. Aspartic proteases produced by A. oryzae show optimal activity at pH range 2.5–6.0, and at 75°C, its activity is reduced by 20% (Ashie, Sorensen, & Nielsen, Citation2002). The fungal proteases are usually effective in the proteolytic activity against elastin and collagen, but show negligible or no effect on myofibrillar proteins (Payne, Citation2009). Similarly, aspartic proteases from A. oryzae show negligible or no effect in the degradation of myofibrillar proteins in meat (Ha, Bekhit, Carne, & Hopkins, Citation2013).
8. Conclusions
Meat tenderness is the most important factor associated with meat palatability and consumer satisfaction. Different plant proteases like papain, bromelain, actinidin, and ficin have been used for tenderization of meat and meat products. Meat proteins can also be hydrolyzed by bacterial enzymes, such as proteases from Aspergillus oryzae and Bacillus subtilis. The bacterial proteases exhibit lower hydrolytic activity in myofibrillar proteins as compared to plant proteases. However, hydrolytic activity of bacterial enzymes in the collagen was found to be intermediate to the activities of papain and bromelain. Further, it is recommended that the combined use of plant and bacterial proteases may have synergistic effect on meat tenderization.
Additional information
Funding
Notes on contributors
Muhammad Sajid Arshad
Muhammad Sajid Arshad is Assistant Professor at Department of Food Science, Government College University, Faisalabad, Pakistan. He has published a number of research and review articles related to his research area in high repute Journals.
References
- Abdel-Naeem, H. H., & Mohamed, H. M. (2016). Improving the physico-chemical and sensory characteristics of camel meat burger patties using ginger extract and papain. Meat Science, 118, 52–60.10.1016/j.meatsci.2016.03.021
- Akpan, I. P., & Omojola, A. B. (2015). Quality attributes of crude papain injected beef. Journal of Meat Science and Technology, 3, 42–46.
- Anderson, M. J., Lonergan, S. M., Fedler, C. A., Prusa, K. J., Binning, J. M., & Huff-Lonergan, E. (2012). Profile of biochemical traits influencing tenderness of muscles from the beef round. Meat Science, 91, 247–254.10.1016/j.meatsci.2012.01.022
- Ashie, I. N. A., Sorensen, T. L., & Nielsen, P. M. (2002). Effects of papain and a microbial enzyme on meat proteins and beef tenderness. Journal of Food Science, 67, 2138–2142.10.1111/jfds.2002.67.issue-6
- Bekhit, A. A., Hopkins, D. L., Geesink, G., Bekhit, A. A., & Franks, P. (2014). Exogenous proteases for meat tenderization. Critical Reviews in Food Science and Nutrition, 54, 1012–1031.10.1080/10408398.2011.623247
- Bekhit, A. E. D. (2010). Fermentation of Fish Roe. In D. R. Heldman, D. G. Hoover, & M. B. Wheeler (Eds.), Encyclopedia of biotechnology in agriculture and food (vol. 1, pp. 251–256). Boca Raton, FL: Taylor & Francis Group.10.1081/E-EBAF
- Berger, A., & Schechter, I. (1970). Mapping the active site of papain with the aid of peptide substrates and inhibitors. Philosophical Transactions of the Royal Society B: Biological Sciences, 257, 249–264.10.1098/rstb.1970.0024
- Bolumar, T., Enneking, M., Toepfl, S., & Heinz, V. (2013). New developments in shockwave technology intended for meat tenderization: Opportunities and challenges. A review. Meat Science, 95, 931–939.10.1016/j.meatsci.2013.04.039
- Breidenstein, B. C., & Carpenter, Z. L. (1983). The red meat industry: Product and consumerism. Journal of Animal Science, 57, 119–132.
- Calkin, C. R., & Sullivan, G. (2007). Adding enzymes to improve beef tenderness. Beef fact. Product enhancement. Lincoln, NE: University of Nebraska.
- Camou, J. P., Mares, S. W., Marchello, J. A., Vazquez, R., Taylor, M., Thompson, V. F., & Goll, D. E. (2007). Isolation and characterization of μ-calpain, m-calpain, and calpastatin from postmortem muscle. I. Initial steps. Journal of Animal Science, 85, 3400–3414.10.2527/jas.2007-0356
- Chaurasiya, R. S., Sakhare, P. Z., Bhaskar, N., & Hebbar, H. U. (2015). Efficacy of reverse micellar extracted fruit bromelain in meat tenderization. Journal of Food Science and Technology, 52, 870–880.
- Chen, Q. H., He, G. Q., Jiao, Y. C., & Ni, H. (2006). Effects of elastase from a Bacillus strain on the tenderisation of beef meat. Food Chemistry, 98, 624–629.
- Cheret, R., Delbarreladrat, C., Lamballerieanton, M., & Verrezbagnis, V. (2007). Calpain and cathepsin activities in post mortem fish and meat muscles. Food Chemistry, 101, 1474–1479.10.1016/j.foodchem.2006.04.023
- Dransfield, E., & Etherington, D. (1981). Enzymes in the tenderization of Meat. In G. G. Birch, N. Blakebrough, & K. J. Parker (Eds.), Enzymes and Food Processing (pp. 177–194). London: Applied Science.
- Eshamah, H., Han, I., Naas, H., Acton, J., & Dawson, P. (2014). Antibacterial effects of natural tenderizing enzymes on different strains of Escherichia coli O157: H7 and Listeria monocytogenes on beef. Meat Science, 96, 1494–1500.10.1016/j.meatsci.2013.12.010
- Fileti, A. M. F., Fischer, G. A., & Tambourgi, E. B. (2010). Neural modeling of bromelain extraction by reversed micelles. Brazilian Archives of Biology and Technology, 53, 455–463.10.1590/S1516-89132010000200026
- FDA. (2001). Secondary direct food additives permitted in food for human consumption. Federal Register, 66, 33829–33830.
- Garg, V., & Mendiratta, S. K. (2006). Studies on tenderization and preparation of enrobed pork chunks in microwave oven. Meat Science, 74, 718–726.10.1016/j.meatsci.2006.06.003
- Geesink, G. H., & Koohmaraie, M. (1999). Postmortem proteolysis and calpain/calpastatin activity in callipyge and normal lamb biceps femoris during extended postmortem storage. Journal of Animal Science, 77, 1490–1501.10.2527/1999.7761490x
- Geesink, G. H., Taylor, R. G., & Koohmaraie, M. (2005). Calpain 3/p94 is not involved in postmortem proteolysis. Journal of Animal Science, 83, 1646–1652.10.2527/2005.8371646x
- Gelse, K., Pöschl, E., & Aigner, T. (2003). Collagens-structure, function, and biosynthesis. Advanced Drug Delivery Reviews, 55, 1531–1546.10.1016/j.addr.2003.08.002
- Gerelt, B., Rusman, H., Nishiumi, T., & Suzuki, A. (2005). Changes in calpain and calpastatin activities of osmotically dehydrated bovine muscle during storage after treatment with calcium. Meat Science, 70, 55–61.10.1016/j.meatsci.2004.11.020
- Goll, D. E., Thompson, V. F., Li, H., Wei, W., & Cong, J. (2003). The calpain system. Physiological Reviews, 83, 731–801.10.1152/physrev.00029.2002
- Grunert, K. G., Bredahl, L., & Brunsø, K. (2004). Consumer perception of meat quality and implications for product development in the meat sector—A review. Meat Science, 66, 259–272.10.1016/S0309-1740(03)00130-X
- Grzonka, Z., Kasprzykowski, F., & Wiczk, W. (2007). Cysteine proteases. In J. Polaina & A. P. MacCabe (Eds.), Industrial enzymes structure, Function and Applications (pp. 181–195). Dordrecht: Springer.
- Ha, M., Bekhit, A. E. A., Carne, A., & Hopkins, D. L. (2012). Characterisation of commercial papain, bromelain, actinidin and zingibain protease preparations and their activities toward meat proteins. Food Chemistry, 134, 95–105.10.1016/j.foodchem.2012.02.071
- Ha, M., Bekhit, A. E. D., Carne, A., & Hopkins, D. L. (2013). Comparison of the proteolytic activities of new commercially available bacterial and fungal proteases toward meat proteins. Journal of Food Science, 78, C170–C177.10.1111/jfds.2013.78.issue-2
- Huff-Lonergan, E., & Lonergan, S. M. (2005). Mechanisms of water-holding capacity of meat: The role of postmortem biochemical and structural changes. Meat Science, 71, 194–204.10.1016/j.meatsci.2005.04.022
- Huff-Lonergan, E., Mitsuhashi, T., Beekman, D. D., Parrish, F. C., Olson, D. G., & Robson, R. M. (1996). Proteolysis of specific muscle structural proteins by mu-calpain at low pH and temperature is similar to degradation in postmortem bovine muscle. Journal of Animal Science, 74, 993–1008.10.2527/1996.745993x
- Ionescu, R. E., Fillit, C., Jaffrezic-Renault, N., & Cosnier, S. (2008). Urease–gelatin interdigitated microelectrodes for the conductometric determination of protease activity. Biosensors and Bioelectronics, 24, 489–492.10.1016/j.bios.2008.06.021
- Islam, M. N., & Molinar-Toribio, E. M. (2013). Development of a meat tenderizer based on papaya peel. Observación por Pares Basada en Mapas Conceptuales: Una Estrategia para Fomentar el “Scholarship of Teaching and Learning” en la Universidad Tecnológica de Panamá, 24.
- Kamphuis, I. G., Kalk, K. H., Swarte, M. B. A., & Drenth, J. (1984). Structure of papain refined at 1.65 Å resolution. Journal of Molecular Biology, 179, 233–256.10.1016/0022-2836(84)90467-4
- Kemp, C. M., & Parr, T. (2012). Advances in apoptotic mediated proteolysis in meat tenderisation. Meat Science, 92, 252–259.10.1016/j.meatsci.2012.03.013
- Kemp, C. M., Sensky, P. L., Bardsley, R. G., Buttery, P. J., & Parr, T. (2010). Tenderness—An enzymatic view. Meat Science, 84, 248–256.10.1016/j.meatsci.2009.06.008
- Ketnawa, S., & Rawdkuen, S. (2011). Application of bromelain extract for muscle foods tenderization. Food and Nutrition Sciences, 2, 393–401.10.4236/fns.2011.25055
- Ketnawa, S., Rawdkuen, S., & Chaiwut, P. (2010). Two phase partitioning and collagen hydrolysis of bromelain from pineapple peel Nang Lae cultivar. Biochemical Engineering Journal, 52, 205–211.10.1016/j.bej.2010.08.012
- Konno, K., Hirayama, C., Nakamura, M., Tateishi, K., Tamura, Y., Hattori, M., & Kohno, K. (2004). Papain protects papaya trees from herbivorous insects: role of cysteine proteases in latex. The Plant Journal, 37, 370–378.10.1046/j.1365-313X.2003.01968.x
- Koohmaraie, M. (1992). Role of the neutral proteinases in postmortem muscle protein degradation and meat tenderness. Reciprocal Meat Conference Proceedings, 45, 63–74.
- Koohmaraie, M. (1996). Biochemical factors regulating the toughening and tenderization processes of meat. Meat Science, 43, 193–201.10.1016/0309-1740(96)00065-4
- Koohmaraie, M., & Geesink, G. H. (2006). Contribution of postmortem muscle biochemistry to the delivery of consistent meat quality with particular focus on the calpain system. Meat Science, 74, 34–43.10.1016/j.meatsci.2006.04.025
- Koohmaraie, M., Shackelford, S. D., Muggli-Cockett, N. E., & Stone, R. T. (1991). Effect of the beta-adrenergic agonist L644, 969 on muscle growth, endogenous proteinase activities, and postmortem proteolysis in whether lambs. Journal of Animal Science, 69, 4823–4835.10.2527/1991.69124823x
- Lantto, R., Kruus, K., Puolanne, E., Honkapää, K., Roininen, K., & Buchert, J. (2009). 12 Enzymes in meat processing. In Robert J. Whitehurst, & Maarten van Oort (Eds.), Enzymes in food technology (2nd ed., pp. 264–291). Hoboken, NJ: Blackwell.
- Liu, F. Y., Liao, J. S., Qi, J. R., & Tang, P. F. (2008). The industry development of papain and bromelain. Science and Technology of Food Industry, 7, 091.
- Lonergan, S. M., Huff-Lonergan, E., Wiegand, B. R., & Kriese-Anderson, L. A. (2001). Postmortem proteolysis and tenderization of top loin steaks from brangus cattle. Journal of Muscle Foods, 12, 121–136.10.1111/jmf.2001.12.issue-2
- Maiti, A. K., Ahlawat, S. S., Sharma, D. P., & Khanna, N. (2008). Application of natural tenderizers in meat-a review. Agriculture Review, 29, 226–230.
- Maróstica, M. R., & Pastore, G. M. (2010). Some nutritional, technological and environmental advances in the use of enzymes in meat products. Enzyme Research, 1–8. doi:10.4061/2010/480923
- Mennecke, B. E., Townsend, A. M., Hayes, D. J., & Lonergan, S. M. (2007). A study of the factors that influence consumer attitudes toward beef products using the conjoint market analysis tool. Journal of Animal Science, 85, 2639–2659.10.2527/jas.2006-495
- Naveena, B. M., Kiran, M., Reddy, K. S., Ramakrishna, C., Vaithiyanathan, S., & Devatkal, S. K. (2011). Effect of ammonium hydroxide on ultrastructure and tenderness of buffalo meat. Meat Science, 88, 727–732.10.1016/j.meatsci.2011.03.005
- Naveena, B. M., Mendiratta, S. K., & Anjaneyulu, A. S. R. (2004). Tenderization of buffalo meat using plant proteases from Cucumis trigonus Roxb (Kachri) and Zingiber officinale roscoe (Ginger rhizome). Meat Science, 68, 363–369.10.1016/j.meatsci.2004.04.004
- Payne, C. T. (2009). Enzymes. In R. Tarté (Ed.), Ingredients in meat products (pp. 173–198). New York, NY: Springer Science + Business Media, LLC.10.1007/978-0-387-71327-4
- Ramezani, R., Aminlari, M., & Fallahi, H. (2003). Effect of chemically modified soy proteins and ficin-tenderized meat on the quality attributes of sausage. Journal of Food Science, 68, 85–88.10.1111/jfds.2003.68.issue-1
- Rawdkuen, S., Jaimakreu, M., & Benjakul, S. (2013). Physicochemical properties and tenderness of meat samples using proteolytic extract from Calotropis procera latex. Food Chemistry, 136, 909–916.10.1016/j.foodchem.2012.08.077
- Ryder, K., Ha, M., Bekhit, A. E. D., & Carne, A. (2015). Characterisation of novel fungal and bacterial protease preparations and evaluation of their ability to hydrolyse meat myofibrillar and connective tissue proteins. Food Chemistry, 172, 197–206.10.1016/j.foodchem.2014.09.061
- Smith, J., & Hong-Shum, L. (2003). Gases. Food additives data book (2nd ed., pp. 581–596). Hoboken, NJ: Wiley-Blackwell
- Starley, I. F., Mohammed, P., Schneider, G., & Bickler, S. W. (1999). The treatment of paediatric burns using topical papaya. Burns, 25, 636–639.10.1016/S0305-4179(99)00056-X
- Tarté, R. (Ed.). (2009). Ingredients in meat products. New York, NY: Springer Science & Business Media.10.1007/978-0-387-71327-4
- Taylor, R. G., Geesink, G. H., Thompson, V. F., Koohmaraie, M., & Goll, D. E. (1995). Is Z-disk degradation responsible for postmortem tenderization? Journal of Animal Science, 73, 1351–1367.10.2527/1995.7351351x
- Toohey, E. S., Kerr, M. J., van de Ven, R., & Hopkins, D. L. (2011). The effect of a kiwi fruit based solution on meat traits in beef m. semimembranosus (topside). Meat Science, 88, 468–471.10.1016/j.meatsci.2011.01.028
- Varughese, K. I., Su, Y., Cromwell, D., Hasnain, S., & Nguyen Huu Xuong, N. H. (1992). Crystal structure of an actinidin-E-64 complex. Biochemistry, 31, 5172–5176.10.1021/bi00137a012
- Veiseth, E., Shackelford, S. D., Wheeler, T. L., & Koohmaraie, M. (2001). Effect of postmortem storage on mu-calpain and m-calpain in ovine skeletal muscle. Journal of Animal Science, 79, 1502–1508.10.2527/2001.7961502x
- Wada, M., Suzuki, T., Yaguti, Y., & Hasegawa, T. (2002). The effects of pressure treatments with kiwi fruit protease on adult cattle semitendinosus muscle. Food Chemistry, 78, 167–171.10.1016/S0308-8146(01)00395-8
- Wendt, A., Thompson, V. F., & Goll, D. E. (2004). Interaction of calpastatin with calpain: A review. Biological Chemistry, 385, 465–472.
- Yeh, C.-M., Yang, M.-C., & Tsai, Y.-C. (2002). Application potency of engineered G159 mutants on P1 substrate pocket of subtilisin YaB as improved meat tenderizers. Journal of Agricultural and Food Chemistry, 50, 6199–6204.10.1021/jf0256889
- Zhang, W., Xiao, S., & Ahn, D. U. (2013). Protein oxidation: Basic principles and implications for meat quality. Critical Reviews in Food Science and Nutrition, 53, 1191–1201.10.1080/10408398.2011.577540