Abstract
Low fertility and inefficient management of sub-Saharan African soils have been the major challenges facing productivity among smallholder farmers. Unfortunately, inorganic fertiliser used as major soil nutrient management is unsustainable, causing soil degradation and environmental pollution. Therefore, smallholder farmers may only realise their maximum potential if a more sustainable, low-cost and efficient integrated nutrient management system compatible with their socioeconomic status is practised. Currently, the increasing demand for sustainable agriculture is driving the use of biological fertilisers, which are composed of beneficial microorganisms; ranging from bacteria to blue-green algae and fungi. Biofertilisers such as Rhizobium, Azotobacter, Azospirillum, Pseudomonas and Bacillus have invaluable use in sustainable agriculture owing to their environmentally-friendliness, cost-effectiveness and improved productivity benefits. They improve plant nutrition and yield through biological nitrogen fixation, nutrient solubilisation, biocontrol activities and production of plant growth promoting substances. This review specifically focuses on biofertiliser potential as an efficient integrated nutrient management in increasing smallholder farmer productivity and profitability. It also suggests that increasing biofertiliser awareness and use is an impetus for maintaining and improving ecological stability and alleviating poverty, especially among the rural dwellers. Importantly, efficient biofertiliser strategies by stakeholders will improve adoption of this technology among smallholder farmers.
Subjects: Soil science; Microbiology; Biotechnology; Agricultural science
Public Interest Statement
The economic condition of most sub-Saharan Africa countries cannot support the development of commercial agriculture and the use of expensive farm inputs such as inorganic fertilizers, which also damage and render more unproductive, the infertile African soil. This article describes the importance of smallholding and sustainable agriculture as the unlocking strategy in alleviating the food security challenges in sub-Saharan Africa. Importantly, the use of environmentally-friendly and cost-effective biofertiliser was reviewed. Biofertilisers, such as nitrogen-fixing, phosphate solubilizing and plant growth-promoting rhizobacteria play crucial roles in preserving and/or restoring soil nutrient richness. It also protects crops against diseases and pests. Evidently, the use of biofertilizers holds the potential to increase crop productivity. Consequently, it is essential to increase awareness and adoption of biofertilizers among smallholder farmers in sub-Saharan Africa. In addition, government and non-government organisations can help to improve awareness through improved regulatory framework, financial support and quality management strategy development.
Competing Interests
The authors declare no competing interest.
1. Introduction
The world population is estimated to reach about 9 billion by 2050 and Africa, especially sub-Saharan Africa, has been predicted to contribute to bulk of the increase (Godfray et al., Citation2010; United Nations, Department of Economic and Social Affairs, Population Division, [UN DESA], Citation2015). In this context, sub-Saharan Africa needs to increase food production to feed her growing population adequately. This growing population has triggered competition in all forms of resources required for human survival such as land, water, energy and food. Perhaps, the most essential is the food resources that have become insufficient, and consequently, its increased production cannot be compromised (Asenso-Okyere & Jemaneh, Citation2012). Sadly, many African countries lack political will that can drive agricultural policies, and this has caused low agricultural productivity. The situation is further aggravated by the design of the economy that reflects “dual” economies, where labour productivity is lower in agriculture than in other industries (Rattso & Torvik, Citation2003). Lack of attention for improving agricultural productivity has degenerated to a more complex situation of food insecurity, which has caused economic, environmental and financial losses in sub-Saharan Africa (Rosegrant, Cline, Li, Sulser, & Valmonte-Santos, Citation2005; Rukuni, Citation2002). Therefore, sub-Saharan African nations need to combat food security situation with a scientific, economic and technologically based approach. This is an impetus for increasing agricultural productivity at a rate higher than the population growth rate, which will in turn trigger economic growth and development (Muzari, Gatsi, & Muvhunzi, Citation2012). Many economic, scientific and technological measures have been designed to support farmers and increase food production in developed countries. However, it is difficult to find evidence of successful implementation of these approaches in many African countries (Abdullah & Samah, Citation2013). Implementation is usually a problem due to challenges related to financing, technical know-how, policy management, financial misappropriation, as well as climatic and environmental conditions (Poulton, Dorward, & Kydd, Citation2010).
Considering this fact, the sustainability of commercial agricultural systems in sub-Saharan Africa has been severely hindered leaving the cultivation of land to smallholder farmers, who cultivate small plots of land to sustain their immediate family (Kariuki, Citation2011; Weis, Citation2007). The smallholder farmers grow subsistence crops and one or more cash crops, relying almost entirely on family labour. It is apparent that the smallholder farmers have the potential to achieve sustainable food production if supported (Collier & Dercon, Citation2014). However, most sub-Saharan African nations have little or no economic policy to support them. It is high time that all stakeholders should fashioned new ideas and strategies to encourage the development of smallholders (Dioula, Deret, Morel, Vachat, & Kiaya, Citation2013; Karuku, Citation2014). According to Jacobs and Baiphethi (Citation2015), one of the strategies is to consider smallholder farmers as entrepreneurs and significant holders of natural resources (soil, water and plants) with the capability to manage natural assets and contribute to the national and global production grid. In Target 4 of the modern Dutch food security policy, some of the ways to achieve 100% increase in smallholder productivity and income include investing in smallholder agriculture, encouraging and reinvesting in agroecological local production and helping smallholder farmers to increase soil organic matter. Helping the smallholders to combat weak agricultural production through efficient integrated nutrient management and intervening through scientific and ecological methods were also suggested (Figure ) (Evert-Jan & Aniek, Citation2014).
Figure 1. Biofertiliser technology employs plant-microbe interactions in influencing plant growth and development.
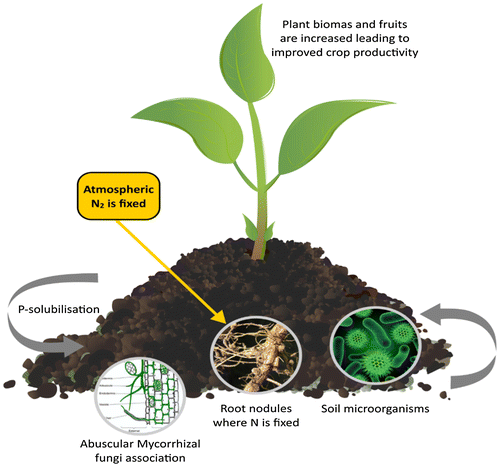
2. Contribution of smallholder farmers to sub-Saharan African economy
Agriculture has been the backbone of African economy and the largest sector on which the economic and social lives of the majority of sub-Saharan African people depend. Interestingly, sub-Saharan African agriculture is primarily driven by smallholder farmers who contribute immensely to the economic growth and development as their roles lead to poverty reduction, rural and social development, as well as food security (Diao, Thurlow, Benin, & Fan, Citation2012; Vanlauwe et al., Citation2014). Smallholder farmers exist in a wide range of locations ranging from the deep rural areas to towns (Ahlers, Kohli, & Sood, Citation2013). It has been estimated that approximately 525 million smallholders exist in the world and support over 2 billion people. About 33 million of these farmers are operating in Africa (International Fund for Agricultural Development [IFAD], Citation2013; Nagayet, Citation2005). Usually, smallholders cultivate below 5–10 acres of land and occupy about 10% of the world’s agricultural land while also accounting for over 20% of the total global food supply. In sub-Saharan Africa, smallholder farms account for over 80% of all the farms and contribute up to 90% of agricultural production (Asenso-Okyere & Jemaneh, Citation2012; Wiggins & Keats, Citation2013). The synergy between economic development and agriculture suggests that if properly managed, agriculture could improve the gross domestic product (GDP) of many sub-Saharan African countries (Cervantes-Godoy & Dewbre, Citation2010). For instance, smallholder farmers cultivate major food crops such as millet, cassava, maize, yam, sorghum, rice, sweet potato, vegetables (tomato, carrot, and pepper) and fruits (mango, pawpaw, apples and orange). Cash crops, which include cotton, cashew nuts, tobacco, tea, coffee and cocoa, are also grown. These contribute to food production and export earnings of sub-Saharan African countries. Many examples of such contributions abound in different African nations. Of recent, Ghana, Zimbabwe, Ethiopia and Kenya have become prominent in export trade as a result of smallholder farmers increasing agricultural production, especially in cash crops in the range of 28–65% that significantly improved their export values (Hu & Lee, Citation2015; Kolavalli & Vigneri, Citation2011; Koskei, Langat, Koskei, & Oyugi, Citation2013; Taffesse, Dorosh, & Asrat, Citation2011). Over 65% of sub-Saharan African labour force is involved in agriculture, generating about 32% of the GDP (Willoughby & Forsythe, Citation2012). In Rwanda, smallholder agriculture employs about 90% of the total labour force and accounts for about 34% of the GDP. Similarly, two third of the entire workforce in Nigeria is employed in agriculture, generating 41% of the GDP. Countries such as Togo, Cote d’ Ivoire, Zimbabwe, Mozambique, Zambia, Tanzania, Kenya and Burundi have within 65–90% of the total labour force employed in agriculture (The World Factbook, Citation2017). Admittedly, harnessing the potentials in smallholder agriculture can lead to social development, sustainable food systems, income equality, decent living conditions and employment opportunities in sub-Saharan Africa, especially among rural dwellers (Dioula et al., Citation2013; Tschirley & Benfica, Citation2001).
However, many of the smallholder farmers are faced with challenges that have hindered their productivity and development (Collier & Dercon, 2014; Karuku, Citation2014). One of such is the inefficient government policies that still encourage the importation of food rather than protecting and improving local production. Also, weak infrastructure, environmental, technical expertise and soil fertility challenges have adversely affected smallholder productivity. It has also been emphasised that there is a need to improve on efficiency, management systems and sustainable practice among smallholders (Francis & van Huis, Citation2016; Tilman, Cassman, Matson, Naylor, & Polasky, Citation2002). Therefore, improved agricultural input subsidies, government financial support and adoption of new technology are critical for greater productivity among smallholder farmers (Denning et al., Citation2009; Muzari et al., Citation2012). One of such essential technologies in integrated nutrient management harnesses the scientific knowledge of the interaction of plant and microorganisms in the rhizosphere (Figure ). This approach is used in biotechnology and microbiological science for products such as phytostimulator, biopesticides and biofertilisers for improving crop nutrient efficiency (Bhattacharyya & Jha, Citation2012; Chandler et al., Citation2011; Rai, Citation2006). The use of these products has potential in increasing smallholder farmer productivity and economic values through soil fertility enhancement and plant growth promoting abilities (Ahmed & El-Araby, Citation2012; Banayo, Cruz, Aguilar, Badayos, & Haefele, Citation2012; Bhardwaj, Ansari, Sahoo, & Tuteja, Citation2014).
In this review, we assess the nutrient challenges of African soil and different integrated nutrient management for its augmentation. In particular, the importance of biofertiliser application in sustainable agronomic practices and in improving the output of smallholder farmers will be discussed in this review.
3. Status of soil fertility in sub-Saharan Africa
Of the over three billion ha of arable land in tropical Africa, only about half a billion ha is free of physical and chemical constraints with 13% having low nutrient content and about 17% having high soil pH (Ugboh & Ulebor, Citation2011). In general, 55% of African land is desert and can only support nomadic grazing (Eswaran, Almaraz, van den Berg, & Reich, Citation1997). Over the years, overgrazing, deforestation and over cultivation without efficient nutrient management have contributed to soil nutrient loss in sub-Saharan Africa (Henao & Baanante, Citation2006). According to Sanchez (Citation2002), an average of 660 kg N, 75 kg P and 450 kg K per ha has been lost in the last 30 years from an estimated 202 million ha of cultivated land in 37 African countries. The estimated nutrient loss for NPK yearly was 800,000 t for humid central Africa, 600,000 t for North Africa, 1.5 million tons for East Africa and 8 million tons in sub-Saharan Africa (Henao & Baanante, Citation2006). In fact, an estimated US$ 4 billion is lost in Africa annually due to nutrient mining and about US$ 42 billion in income and 6 billion ha of valuable land lost annually due to land degradation and reduction in crop productivity (Bationo et al., Citation2012).
In sub-Saharan Africa, the yield gap has continually increased, deteriorating to less than 25% of potential attainable yield while the per capita food production has also continued to decrease over the past 40 years (Sanchez, Citation2002). With the increase in demand for food and social and economic development, the available agricultural land will continue to decrease. Unfortunately, arable land is being damaged by anthropogenic and environmental factors, coupled with the fact that many of the cultivated soils are naturally infertile. Yet, the low nutrient stock will have to be cultivated (Henao & Baanante, Citation2006). This suggests the need for efficient integrated soil fertility management (ISFM) to improve productivity (Rashid et al., Citation2016; Sommer et al., Citation2013).
3.1. Limiting nutrients of sub-Saharan African soil
Unfortunately, some African soils lack essential nutrients. In Uganda, Kenya and Tanzania low yield of crops was attributed mainly to poor soil fertility (Keino et al., Citation2015). For instance, Zn is deficient in most West African soils, especially the lowland areas (Abe, Buri, Issaka, Kiepe, & Wakatsuki, Citation2010) while plant viable P is unavailable in the iron-rich tropical soils of Africa due to low pH and high level of iron and aluminium oxides (de Valença & Bake, Citation2016). In fact, 80% of smallholder farmlands for maize cultivation in Kenya are extremely deficient in P (Tittonell, Shepherd, Vanlauwe, & Giller, Citation2008). Ferrolsol soils, which have low capacity to supply essential nutrients to crops, occupy a sizeable part of central Africa-Angola, Zambia, Burundi, Uganda and Cameroon (Deckers, Citation1993). Low fertile soil mostly characterises West African countries such as Liberia and Sierra Leone and part of Madagascar. The soil lacks Ca, Mg and K, and when acidic, has a high level of free Mn, which is toxic to crops. According to Bühmann, Beukes, and Turner (Citation2006), some South African soils are deficient in K and P, making it unsuitable for cultivation. Similarly, East African soils have up to 90% N, 50% P and 50% K as limiting nutrients (Bekunda, Nkonya, Mugendi, & Msaky, Citation2002).
The average nutrient required by various crops for an increased yield are presented in Table . In sub-Saharan Africa, the total NPK requirement per ha per year range from 24.5 to 176 kg NPK/ha (Henao & Baanante, Citation1999). It is worthy to note that neither crop quality nor adequate nutrient supply will cause an increase in yield where a third factor is limiting.
Table 1. Illustrative information for average nutrients absorbed by crops causing a medium to high crop yield under fertile soil conditions. Soil testing was considered in arriving at the figures and the units are in kg of Nutrient per hectare
3.2. Low fertiliser usage in sub-Saharan Africa
Organic and inorganic fertilisers are the major categories of fertilisers used by smallholder farmers. The inorganic fertilisers are in the form of ammonium nitrate, urea, rock phosphate, potassium chloride and potassium sulphate (Morris, Kelly, Kopicki, & Byerlee, Citation2007). The majority of the inorganic fertilisers in Africa are imported, and the bulk of it is primarily used in commercial agriculture. According to Food and Agricultural Organisation (FAO) (Citation2015), 2013 fertiliser statistics, South Africa and Nigeria had the highest quantity of N fertiliser imported while Uganda and Cameroon had the lowest amount (Figure ). However, Africa has lower fertiliser consumption when compared to other regions of the world. In 2002, sub-Saharan Africa had about 8 kg/ha of fertiliser consumption which increased to 12 kg/ ha in 2010 and 18 kg/ha in 2013 (Sommer et al., Citation2013). This is far below that of other regions of the world such as North America, South Asia, and East Asia and Pacific which were estimated at 127.9 kg/ha, 151.8 and 337.0 kg/ha respectively (World Bank Fertiliser Consumption, Citation2013).
Figure 2. Nitrogen, Phosphorus and Potassium fertiliser importation in some African countries.
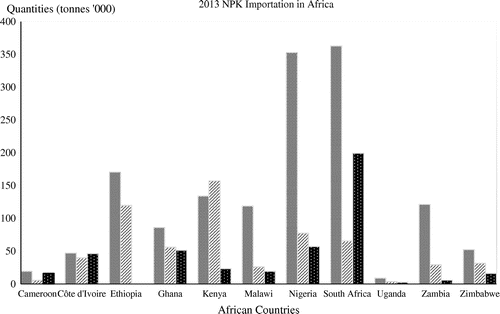
Low fertiliser usage in sub-Saharan Africa is attributed to lack of financial incentives, weak fertiliser policies, high product price and low fertiliser demand and supply (Liverpool-Tasie, Omonona, Sanou, & Ogunleye, Citation2015; Minde, Jayne, Crawford, Ariga, & Govereh, Citation2008). Unfortunately, when demand is low, economies of scale among manufacturers is affected and the consequence is the high cost of operation that is reflected in the product price (Yanggen, Kelly, Reardon, & Naseem, Citation1998). Besides, many African countries do not have favourable business environment; investors are discouraged with exorbitant taxes and fees, unnecessary regulations and high rental costs. These have hindered full commercialisation of fertilisers (Druilhe & Barreiro-Hurlé, Citation2012). Moreover, inconsistency in foreign exchange rate, demand and supply have made fertiliser profitability unpredictable, which often presents a risk and substantial impediments to fertiliser use among smallholders (Yanggen et al., Citation1998).
Sub-Saharan Africa fertiliser market lacks basic infrastructure for sustainability, efficient pricing and competition (Sommer et al., Citation2013). Consequently, the market has been positioned in the hands of monopolists who are in the business only to make profits (Morris et al., Citation2007). A good fertiliser policy would be one of the strategies to resuscitate the collapsing market and poor price incentives (Minde et al., Citation2008). Several policies to encourage the use of inorganic fertiliser in sub-Saharan Africa have either not been formulated or where in place, have been ineffective and inconsistent (Sommer et al., Citation2013). A genuine implementation strategy of effective input subsidy policy would make fertiliser affordable and thus increase usage among the smallholder farmers. The recent introduction of input support program in some African countries such as Malawi, Kenya, Zambia, Ghana and Nigeria has indicated hope for better fertiliser usage among smallholders (Mwangi, Citation1996; Ngetich, Shisanya, Mugwe, Mucheru-Muna, & Mugendi, Citation2012).
Inorganic fertiliser has been suggested to be the short-term solution for increasing crop yield in Africa, and the present fertiliser consumption needs to be increased by 10–18% annually to reach this target (Sommer et al., Citation2013; Wallace & Knausenberger, Citation1997). However, the long-term solution would be an approach that is not only based on improving soil nutrient level and plant management; it must also guarantee risk-free incentives for farmers to invest in. Similarly, the approach should lead to sustainable economic growth in smallholder agriculture, as well as ecological sustainability (Sommer et al., Citation2013). In addition, a very small percentage of sub-Saharan African farmers are also concerned about the adverse environmental impacts of inorganic fertiliser while a larger percentage of farmers consider the benefits and access to other efficient nutrient management system such as organic fertilisers as a good alternative.
3.3. Challenges in the use of organic fertilisers in sub-Saharan Africa
One of the major nutrient management, which has been in use for centuries, is organic fertiliser. It is made from natural materials of either plant or animal matter and includes animal manure (cow dung and poultry manure), household wastes, crop residues and compost (Ngetich et al., Citation2012). Aside from supplying nutrients, organic fertilisers also improve the physical structure and biochemical activity level of the soil. Another advantage of organic fertiliser is that it releases nutrients into the soil through gradual decomposition of the organic matter. This slow decomposition of organic matter regulates the rate at which organic matter is added and builds up in the soil, thereby balancing soil organic matter content (Morris et al., Citation2007). However, the challenge of its availability, management and cost have decreased its use among sub-Saharan African smallholder farmers (Svotwa, Baipai, & Jiyane, Citation2009; Trewavas, Citation2001). The quantity of available organic manure is inadequate to supply the actual nutrient needs of crops in any African region. Unfortunately, the little produced are wasted due to minimal technical expertise (Abbas, Citation2016). In South Africa for example, 3 million tons of animal manure produced annually is enough to supply about 13.3% N, 27.6% P and 9.9% K of the soil nutrient need. However, only 25% is used on the soil while the remaining is unexploited due to management constraints (Harris, Citation2002; Okorogbona & Adebisi, Citation2012). In addition, the nutrient ratio of organic fertilisers varies for the same product from different sources; this has made soil-plant-nutrient need application ratio very difficult (Rowell & Hadad, Citation2004).
Majority of sub-Saharan African countries have poor road networks that increase the cost of transportation and coupled with the extensive labour requirement for truck loading, the cost of organic manure has become increasingly high, making it uneconomical for smallholders (Rosen & Bierman, Citation2005). Furthermore, the pungent smell associated with some of the organic fertilisers (animal manure), has made it difficult to work with. In many instances, cow dung, poultry waste, and compost have been reported as a potential haven for pathogenic microbes (Heinonen-Tanski, Mohaibes, Karinen, & Koivunen, Citation2006) and heavy metal contaminants (Cd, Hg, Pb, and Ni) in agricultural soil. These cause diseases to plants and animals, hence creating more troubles than its benefits (Moreno-Caselles, Moral, Perez-Murcia, Perez-Espinosa, & Rufete, Citation2002). Organic fertilisers have relatively low nutrient content and an uncontrollable decomposition rate due to variability in soil moisture, temperature and biodiversity. This fluctuation in nutrient supply has made organic fertiliser uneconomical for growing plants with urgent and substantial nutrient needs (Pang & Letey, Citation2000).
There is an increasing concern in the inefficient management of organic manure, which causes emissions of gasses (ammonia, nitrous oxide, carbon dioxide and methane) and eutrophication in rivers and lakes (Pacanoski, Citation2009). During the last decade, Africa has witnessed an increase in the emission of greenhouse gasses (GHG), especially CO2 and N2O from the application of manure for crop production (FAO, Citation2015; Hristov et al., Citation2013; Tubiello et al., Citation2013). Emission of GHG remained highest in Eastern Africa followed by Western and Northern Africa while the lowest amount was reported in the Middle and Southern Africa. In 2012, the rate of emission in East Africa increased by 12.8% over the previous year. This may be due to the increased use of organic manure without efficient management (FAO, Citation2015).
The challenges in the use of inorganic and organic fertilisers have caused the need to intensify more on best management practices and efficient integrated nutrient management in order to achieve a more sustainable and economical way of nutrient management. This will not only benefit the smallholders but will also lead to ecological sustainability (Ngetich et al., Citation2012). Such efficient nutrient management has emphasised the practice of sustainable agriculture. Sustainable agriculture ensures resource efficiency by integrating biochemical, economic and physical sciences to develop new practices that are safe, cost-effective and environmentally friendly. It also supports an ecosystem that accommodates and provides for the development of all classes of plants and animals (Gupta, Kalia, & Kapoor, Citation2007; Lichtfouse et al., Citation2009; Vejan, Abdullah, Khadiran, Ismail, & Nasrulhaq Boyce, Citation2016).
4. Biofertiliser
An approach that guarantees biosafety, nutrient-rich yield and efficient nutrient utilisation, as well as increased productivity, will support sustainable agriculture (García-Fraile, Menéndez, & Rivas, Citation2015; Lesueur, Deaker, Herrmann, Bräu, & Jansa, Citation2016). One of such approaches involves the use of microbial technology in the production of biological fertilisers, generally called biofertiliser (Aggani, Citation2013; Ahsan, Ali, & Ahmed, Citation2012).
4.1. What are biofertilisers?
The idea of biofertiliser (“bio” which means life and fertiliser which means substances used to deliver plant nutrients in usable form) was first initiated in 1834 when a French agricultural chemist named J. B. Boussingault reported soil nitrogen build-up through legume cultivation (Bhattacharyya, Citation2014). Hellriegel and Wilfarth (Citation1886) and, Beijerinck (Citation1888), a Dutch scientist, further established the concept of biological nitrogen fixation. Subsequently, Noble F. and Hiltner L. (who produced the first rhizobium biofertiliser, Nitragin in 1896), and other scientists have made various contributions and discoveries that have led to full biofertiliser commercialization and use in sustainable agriculture (Bhattacharjee & Dey, Citation2014; Boraste et al., Citation2009). Many biofertiliser products exist in sub-Saharan Africa with major producers from South Africa and Kenya (Table ).
Table 2. Microbial composition of commercial biofertilisers and their function
Biofertilisers are substances containing live microorganisms, which when applied to plant surfaces, seeds, roots or soil; colonize the rhizosphere or the interior of plants and help to improve soil fertility while also stimulating plant growth by increasing the availability of plant nutrients and growth substances to the host crops (Figueiredo, Seldin, de Araujo, & Mariano, Citation2011; Suyal, Soni, Sai, & Goel, Citation2016; Vessey, Citation2003). The term biofertiliser is sometimes used interchangeably with “microbial inoculant, bioinoculant, inoculum or bioformulation” (Gupta et al., Citation2007; Hassen, Bopape, & Sanger, Citation2016; Suyal et al., Citation2016) or effective microorganisms (Megali, Schlau, & Rasmann, Citation2015). Biofertilisers comprised of bacteria, blue-green algae (BGA) and fungi (separately or in combination), and can be made in solid, powdered, granular carrier materials or liquids. The carrier materials sustain the microbial inoculants and allow the product to be stored for longer period (Boraste et al., Citation2009; Rashid et al., Citation2016). The beneficial microbes may be rhizospheric; colonising the surface or intercellular spaces of the plant roots, or endophytic; where they colonise the tissue or apoplastic space within the host plants (Gupta, Panwar, Akhtar, & Jha, Citation2012; Malusà, Pinzari, & Canfora, Citation2016).
Biofertilisers should not be misunderstood for organic fertilisers such as compost, animal manure and plant manure or extracts (Carvajal-Muñoz & Carmona-Garcia, Citation2012; Malusá, Sas-Paszt, & Ciesielska, Citation2012; Vessey, Citation2003). However, whether the beneficial microbes improve crop accessibility to nutrients (Egamberdiyeva, Citation2007; Mujawar, Citation2014) or replenish soil nutrients (Shridhar, Citation2012; Thamer, Schädler, Bonte, & Ballhorn, Citation2011), if the overall nutrient condition of crop and soil has been improved, such substances containing the beneficial microorganisms are considered as biofertilisers (Vessey, Citation2003).
4.2. Types of biofertiliser
Biofertilisers are classified based on their microbial composition and functional characteristics established during their interactions with plants in the rhizosphere (Lucy, Reed, & Glick, Citation2004; Malusá et al., Citation2012). It has been suggested that the classification of biofertiliser be based on the ability to perform at least two major functions (Gupta et al., Citation2012; Lesueur et al., Citation2016). Major classifications include N-fixing, phosphate and micronutrient solubilising and plant growth promoting rhizobacteria biofertilisers (Figure ).
Figure 3. Types of biofertilisers based on functional attributes.
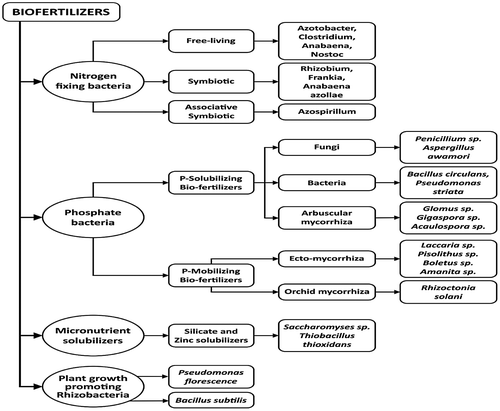
4.2.1. Nitrogen-fixing biofertilisers
Nitrogen is abundant in the atmosphere, making up about 80% (approx. 1015 tonnes) of the atmospheric gasses. It is so stable that it is inaccessible by living organisms except when converted to compounds that can be assimilated by plants (Bernhard, Citation2010; Thamer et al., Citation2011). The inert N2 is recycled through different transformations involving various soil microorganisms (Figure ). In N deficient soil, diazotrophs fix N gas from abiotic to biotic environments through biological nitrogen fixation (BNF) process using the enzyme called nitrogenase. This oxygen-sensitive enzyme complex, which is composed of dinitrogenase reductase and dinitrogenase, is responsible for reducing the atmospheric N2 into reactive forms such as ammonia (Dighe et al., Citation2010). Diazotrophs are usually in symbiotic or non-symbiotic relationships with the host plants. The symbiotic relationship is common in Rhizobium, Bradyrhizobium and Sinorhizobium, which inhabit the root nodules of most leguminous crops such as groundnut, soybean and cowpea (Martínez-Romero, Citation2009; Oldroyd, Murray, Poole, & Downie, Citation2011). The non-symbiotic free-living N-fixers include Azotobacter, Beijerinckia and Clostridium while associative N-fixers include Azospirillum and Enterobacter species (Shridhar, Citation2012; Wagner, Citation2012). Other bacteria that can fix N and as well produce plant growth promoting substances are Azoarcus sp., Klebsiella pneumonia, Rhizobium sp., and Pontoea agglomerans (Riggs, Chelius, Iniguez, Kaeppler, & Triplett, Citation2001; Yanni et al., Citation2001).
Figure 4. Nitrogen cycle.
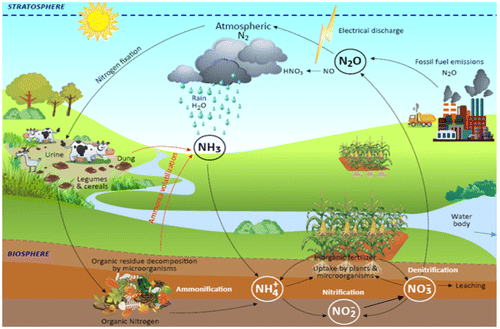
Another important N biofertiliser of considerable economic importance to smallholder farmers is cyanobacteria (Benkeblia & Francis, Citation2014). It is made up of Anabaena (or Nostoc) in association with Azolla and used mainly for rice cultivation. Azolla is a heterosporous water fern and in association with Anabaena, can fix N in the range of 40–100 kg/ha when used for wet-rice cultivation (Paudel, Pradhan, Pant, & Prasad, Citation2012; Wagner, Citation2012).
4.2.2. Solubilising and mobilising biofertiliser
Soil P and K couple with elements like iron, aluminium and calcium to form stable compounds, which are not readily available for plant use (Mohammadi, Citation2012; Richardson, Barea, McNeill, & Prigent-Combaret, Citation2009). This has resulted in limiting nutrients, especially for P. Therefore, solubilisation and mobilisation, which are exhibited by the phosphate-solubilising and mobilising microbes are important aspects of P cycle (Mohammadi, Citation2012; Sundara, Natarajan, & Hari, Citation2002).
P-solubilising microorganisms (PSM) comprise mainly bacteria and fungi (Mohammadi, Citation2012; Sundara et al., Citation2002). According to Pindi and Satyanarayana (Citation2012), most efficient P solubilisers include the following genera: Bacillus, Pseudomonas, Aspergillus, and Penicillium. They mineralize organic P through enzymatic hydrolysis by secreting phosphatase enzymes (Figure ). This can save up to 30–50 kg/ha of P2O5 fertiliser (Richardson et al., Citation2009; Singh, Srivastava, Sharma, & Sharma, Citation2014). PSM also secrete organic acids such as oxalic, citric, gluconic and lactic acids to solubilise soil inorganic P e.g. tricalcium phosphate and rock phosphate to monobasic (H2PO4−) and dibasic (HPO42−) ions (Adeleke, Nwangburuka, & Oboirien, Citation2017; Mohammadi, Citation2012; Rai, Citation2006). Their efficiency is dependent on the soil carbon and nitrogen source, as well as the soil buffering ability. Azotobacter chroococcum, Bacillus circulans, Pseudomonas chlororaphis, P. putida and Rhizobium leguminossarium have been used as phosphate solubilising biofertilisers (PSB) on various crops like potato, tomato, wheat and pulse (Malusà & Ciesielska, Citation2014).
Figure 5. Soil microbes mediate phosphorus (P) availability for plant growth through solubilisation of inorganic P and mineralisation of organic P.
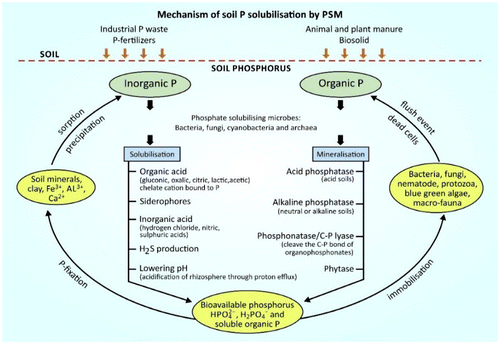
Another technique used by most plants to arrest P limiting situation is the plant-fungi symbiotic relationship (ectomycorrhiza and endomycorrhiza). Plants develop increased root growth by the extension of the existing root systems through mycorrhizal association or hormonal stimulation (phytostimulation) effect (Richardson & Simpson, Citation2011). Here, fungi hyphae are able to mobilise and make P available to the plants (Mujawar, Citation2014; Ramasamy et al., Citation2011). According to Adeleke, Cloete, Bertrand, and Khasa Citation(2010), ectomycorrhizal fungi such as Pisolithus tinctorius, Paxillus involutus, Phialocephala fortini and Suillus tomentosus have potential to mobilise substantial amount of P and K from different minerals under different conditions. The arbuscular mycorrhizal fungi (AMF) increase its exploitation of soil nutrients through specialised structures known as vesicles and arbuscules (Leigh, Hodge, & Fitter, Citation2009). In addition, P is mobilised through the changes in sorption balance of soil solution caused by microbial biomass turnover in the rhizosphere. This leads to an increased mobility and uptake of organic P or orthophosphate ions (Adeleke et al., Citation2017). Microbial metabolic processes can also directly solubilise and mineralise inorganic and organic P through the efflux of protons, organic ions and siderophore production (Figure ) (Richardson & Simpson, Citation2011).
Potassium Solubilising Biofertiliser (KSB) solubilises K from compounds such as mica, muscovite, illite, orthoclase and biotite by producing organic ligands, hydroxyl anions, enzymes and biofilms (Bahadur, Meena, & Kumar, Citation2014; Shanware, Kalkar, & Trivedi, Citation2014). The ability to solubilise potassium effectively depends on the soil type and microbial strain, as well as the form of K compounds (Sangeeth, Bhai, & Srinivasan, Citation2012). KSB include Pseudomonas, Burkholderia, Acidothiobacillus, Bacillus, and Paenibacillus genera (Ahmed & El-Araby, Citation2012; Liu et al., Citation2010; Yasin, Munir, & Faisal, Citation2016). This biofertiliser is important in areas experiencing high rate of potassium loss found in West Africa (Nigeria and Guinea Bissau) and East Africa (Burundi, Malawi, Kenya, Swaziland, Uganda and Rwanda) (Bationo et al., Citation2012).
4.2.3. Micronutrient biofertiliser
Micronutrients (Zn, Fe, Mn, Cu and Mo) form insoluble complexes in the soil, which are not readily accessible by crops. According to Mahdi et al. (Citation2010), 75% of applied Zn forms insoluble complexes while plants use only about 1–4% of total available Zn in the soil. However, Rhizobium, Bradyrhizobium, Pseudomonas, Thiobacillus, Saccharomyces, Penicillum and Bacillus can improve the uptake and availability of micronutrients in the soil (Adeleke, Cloete, & Khasa, Citation2010; Ahsan et al., Citation2012; Esitken et al., Citation2010). In Fe immobilised soils, for example, bacteria siderophores solubilise and chelate Fe into complexes that can be easily absorbed by the plant roots (Mathew, Eberl, & Carlier, Citation2014; Radzki et al., Citation2013). Trichoderma harzianum, a fungal specie, can solubilise minerals such as metallic Zn and MnO2 by chelating and reducing mechanisms (Altomare, Norvell, Björkman, & Harman, Citation1999). Vesicular Arbuscular Mycorrhiza (VAM) are also able to solubilise Zn, Fe, Mn, and Cu in agricultural soil (Martino, Perotto, Parsons, & Gadd, Citation2003; Pal, Singh, Farooqui, & Rakshit, Citation2015).
4.2.4. Plant growth promoting rhizobacteria (PGPR)
This is made up of heterogeneous group of beneficial rhizospheric microbes that can stimulate plant growth and development through one or more mechanisms (Roy, Finck, Blair, & Tandon, Citation2006). The growth promoting substances may increase plant growth directly or indirectly (Hayat, Ali, Amara, Khalid, & Ahmed, Citation2010; Soltani et al., Citation2010). They may indirectly control diseases by producing antimicrobial metabolites such as hydrogen cyanide, phenazines and tensin. PGPR interaction with the plant roots may also elicit plant defense against bacterial, fungal and viral pathogens. This is termed induced systemic resistance (Ahemad & Kibret, Citation2014; Vacheron et al., Citation2013). This group has multiple modes of action, which may include N-fixation, nutrient solubilisation and phytostimulation (Table ) (Beneduzi, Ambrosini, & Passaglia, Citation2012; Bhattacharyya & Jha, Citation2012; Gururani et al., Citation2013; Hassen et al., Citation2016). PGPR include the genera Azotobacter, Bacillus, Azospirillum, Burkholderia, Erwinia, Flavobacterium, Pseudomonas and Serratia. Others include the Mesorhizobium, Bradyrhizobium, Rhizobium, and Azorhizobium, which are intracellular bacteria (Ahemad & Kibret, Citation2014; Bhattacharyya & Jha, Citation2012).
5. Contribution of biofertiliser in smallholder agriculture
The profitability benefit of biofertiliser can be deduced from biofertiliser benefit-cost analysis, which is based on the ratio of the obtainable value of benefit compared to the actual cost of the inoculum at a particular time. An enterprise will be profitable when the benefit to cost ratio exceeds 1 after discounting the gross cost and benefit. According to Mulongoy, Gianinazzi, Roger, and Dommergues (Citation1992), the benefits from legume inoculants is usually based on the N fixed. For instance, the benefit-cost ratio for white clover was found to be 416, fixing 200 kg of N/ha while that of soybean was 17, fixing 100 kg of N/ha from inoculation when the cost of fixed N is considered as 50 cents per kg (Mulongoy et al., Citation1992). Some significant contributions of biofertiliser in smallholder agriculture are discussed below.
5.1. Increased yield and nutrient availability
Legume yield among smallholder farmers can be increased by using N-fixing biofertilisers such as Rhizobium and Bradyrhizobium (Hassen et al., Citation2016). For example, inoculation of soybean causes an increase in yield, improves soil organic matter while also fixing about 80% of soybean N need (Chianu, Nkonya, Mairura, Chianu, & Akinnifesi, Citation2010; Giller, Murwira, Dhliwayo, Mafongoya, & Mpepereki, Citation2011). Rose et al. (Citation2014) reported that biofertiliser could replace about 52% of N-fertiliser and cause an increase in rice yield over the control. Rhizobium biofertiliser alone can supplement about 50% of the fertiliser need of crops in most arid and semiarid marginal lands of Zimbabwe, Tanzania, and Kenya, which are deficient in N (Chianu et al., Citation2010; Mugabe, Citation1994; Nkonya et al., Citation2008). Ronner et al. (Citation2016) also found that crop yield increased by 447 kg/ha over the control due to inoculation in northern Nigeria. Azolla soaked in 50 ppm of superphosphate when inoculated in a paddy field fixed about 40–55 kg N/ha, 15–20 kg P/ha and 20–25 kg K/ha in a month per 1 kg of Azolla applied, bringing the yield of flooded paddy to about 10–20% over the control (Wagner, Citation2012). Different Azolla species- A. pinnata, A. nilotica, A, filiculoides and A. caroliniana found in African countries such as Nigeria, South Africa, Ivory Coast, Togo, Senegal and Kenya can be used on rice plantation to increase yield and soil N content (Diniz, Teixeira, & Carrapico, Citation2015).
The use of biofertilisers leads to separate accumulation of N, P and K in the soil, thereby maintaining soil nutrient balance (Adesemoye, Torbert, & Kloepper, Citation2008; Egamberdiyeva, Citation2007). Sundara et al. (Citation2002) observed an increased sugarcane and sugar yield when the plant was inoculated with PSB, Bacillus magisterium var. Phosphaticum. Similarly, the use of biofertilisers with cheap rock phosphate increased crop yield by 74% over the control, while peanut and sunflower plants inoculated with PSB recorded a significant yield over the control (Ahmed & El-Araby, Citation2012).
KSB such as Pseudomonas and Burkholderia has been reported to cause an increase in plant growth and yield in wheat and pepper (Sangeeth et al., Citation2012; Shanware et al., Citation2014), while Bacillus, a KSB and PSB caused a yield increase in cucumber and pepper (García-Fraile et al., Citation2015). Han and Lee (Citation2005) reported an increase in NPK uptake, photosynthesis and yield of eggplant cultivated on P and K limited soils when KSB and PSB were co-inoculated with direct application of rock P and K. Bradyrhizobium japonicum inoculation causes an increase in yield and micronutrient uptake in soybean plant (Tairo & Ndakidemi, Citation2014). According to Bambara and Ndakidemi (Citation2010), the concentrations of Fe, Cu and Zn in the rhizosphere of Phaseolus Vulgaris when inoculated with Rhizobium increased by 28, 20 and 67% respectively compared to the control. A similar result was obtained in the case of Origanum vulgare L. where AMF inoculum induced a significant increase in shoot nutrient content (Mg, Fe, Cu, Mn, N, P, & K) (Khalil & El-Noemani, Citation2015). Guimarães, Neves, Bonfim-Silva, and Campos (Citation2016) cultivated cowpea plants with four Rhizobium strains. The result showed that biofertiliser treatment gave a better yield with an increase in soil nutrient quantity such as N and P over the control. This could be due to the role of biofertiliser in improving soil nutrient content and nutrient uptake of the plant (Guimarães et al., Citation2016).
5.2. Plant growth promoting rhizobacteria as biofertiliser
Various species of beneficial microorganisms grow in the rhizosphere, participating in nutrient cycling and the production of plant growth promoting substances. Hence, are called plant growth promoting rhizobacteria (PGPR) (Ahemad & Kibret, Citation2014; Beneduzi et al., Citation2012; Bhattacharyya & Jha, Citation2012). Some of the PGPR are considered biofertiliser due to their biofertilization potential (Vessey, Citation2003). Similarly, bacteria such Bacillus and Paenibacillus have been found to cause an increase in plant-mycorrhizal colonisation, therefore, are refered to as mycorhizal helper bacteria (MHB) (Adeleke & Dames, Citation2014). Some biofertilizing-PGPRs produce phytohormones such as indole acetic acid, gibberellins and cytokinins that cause an increase in plant foliage, root elongation, fruit yield and plant-microbe symbiosis (Hassen et al., Citation2016; Vacheron et al., Citation2013). Indole acetic acid (IAA) affects plant root architecture; leading to increased root surface area and root tip elongation (Ahmad, Ahmad, & Khan, Citation2005; Lu et al., Citation2015) while gibberellic acid induces increased flowering, stem and internode elongation, fruit setting and growth in plants (Kumar, Biswas, Singh, & Lal, Citation2014; Zalewska & Antkowiak, Citation2013). Therefore, beneficial microbes that promote root development have an immense impact on nutrient uptake ability (Vessey, Citation2003).
In a trial study by Swain, Naskar, and Ray (Citation2007), Dioscorea rotundata inoculated with Bacillus subtilis, an IAA producing strain, had an increased tuber length and number of sprouts compared to uninoculated plants. Maize and rice cultivated with gibberellic acid producing PGPRs had a significant increase in growth and yield (Vacheron et al., Citation2013). Similarly, root hair, surface area and total biomass increase in tomato plants inoculated with Azospirillum sp. producing IAA-mediated ethylene (Ribaudo et al., Citation2006). Siderophore is another important plant growth promoting substances. According to Egamberdiyeva (Citation2007), siderophore produced by PGPR stimulates the growth of maize under iron-poor soil. More so, increase in yield of many crops (maize, rice, wheat, soybean, and bean) was reported in the work of Pérez-Montaño et al. (Citation2014) when the crops were inoculated with biofertilisers.
5.3. Low cost of nutrient supply
Biological nitrogen fixation (BNF), a natural process of fixing N in the soil, has been put at different values. Galloway (Citation1998) estimated the annual BNF to be about 90–130 Tg N year−1 while Boyer et al. (Citation2004) reported it to be roughly 107 Tg N year−1. Bhattacharyya (Citation2014) estimated BNF on land to be 140 Tg N year−1. Interestingly, the energy bill of this process is fully paid by nature. Similarly, it has been reported that about 48–300 kg N/ha can be fixed by BNF on a grain legume plot in a season (Ngetich et al., Citation2012). This low-cost method of supplying nutrient to the soil has made the use of biofertiliser economical for smallholder farmers. In addition, the quantity of biofertiliser required to achieve the same amount of nutrients supplied by inorganic fertiliser is relatively lower. The cost of peat-based rhizobium inoculants is as low as US$ 0.24 ha−1 for white clover and US$ 6.46 ha−1 for Vicia faba. Rhizobium biofertiliser sufficient for 1 ha cost US$ 5.20 in Zimbabwe and US$ 4.50 in Rwanda (Mulongoy et al., Citation1992). These prices are far below the cost of mineral fertiliser needed for the same quantity of nutrients supplied. In West Africa, NoduMax, like other biofertilisers, is economically profitable costing only $5 per ha in application as opposed to $100 per ha cost of urea fertiliser needed to supply the same quantity of nutrients (N2Africa, Citation2015).
The production process of mineral fertiliser is faced with energy challenges, which makes it more expensive, compared to biofertilisers. In fact, about 60% of smallholder farmers in Africa cannot afford the high priced inorganic fertiliser (Chianu et al., Citation2010). The energy requirement for producing 1 kg of inorganic fertiliser has been estimated to be 80 MJ (11.2 kWh) for N, 12 MJ (1.1 kWh) for P and 8 MJ (1 kWh) for K. This is rather uneconomical and unsustainable, considering the challenges of power generation in many of the sub-Saharan African countries (Bhattacharyya, Citation2014). In addition, phosphorus reserves are likely to be exhausted in the next few decades (Rosemarin, De Bruijne, & Caldwell, Citation2009; Sutton et al., Citation2013). Although this has been discredited, as enough P deposits that can last for the next 400 years have been reported (Cho, Citation2013; Van Kauwenbergh, Stewart, & Mikkelsen, Citation2013). However, the major issue is that P is lost in the soil when applied. Crops take up an estimated 20% of P while about 40% is lost during processing and mining, and over 50% is wasted in the agricultural soil (Cho, Citation2013; Schröder, Cordell, Smit, & Rosemarin, Citation2010). This, if not effectively managed, will cause an increase in the cost of P products (Sutton et al., Citation2013).
5.4. Other beneficial properties of biofertilisers
5.4.1. Prevention of plant pests and diseases
Some biofertilisers prevent plant diseases by directly inhibiting pathogens through their metabolic activities or indirect competition (García-Fraile et al., Citation2015; Rudrappa, Czymmek, Pare, & Bais, Citation2008). The nodule-forming symbiotic association of legumes with Rhizobium has been established to enhance the synthesis of cyanogenic defence substances, which increases plant resistance to herbivore attack (Mazid, Khan, & Mohammad, Citation2011; Megali et al., Citation2015; Thamer et al., Citation2011). Bacterial and fungal attacks are major factors affecting smallholder productivity, especially in sub-Saharan Africa (Strange & Scott, Citation2005). Therefore, using biofertilisers producing antifungal and antibacterial substances such as chitinases and β-glucanases assists in suppressing diseases attack. Fusarium wilt of pigeon pea and soft rot of potato caused by Fusarium udum Butler and Erwinia Carotovora can be controlled by Pseudomonas fluorescens and sinorhizobium, both producing chitinase and β-glucanases (Guo, Rasool, & Li, Citation2013; Kumar, Bajpai, Dubey, Maheshwari, & Kang, Citation2010). Bacillus sp. inhibit important pathogens such as Rhizoctonia solani in tomatoes and Phytophthora capsici in pepper (Akgül & Mirik, Citation2008; Arora, Khare, Oh, Kang, & Maheshwari, Citation2008; Solanki et al., Citation2012).
Some biofertilisers produce siderophore, a Fe-chelating agent, which limits the available Fe in the soil. This indirect competition for nutrients suppresses the pathogen’s ability to cause diseases (Arora, Khare, & Maheshwari, Citation2010; Solanki et al., Citation2014). Siderophores produced by Pseudomonas and Bacillus attack the popular fusarium wilt of potato and maize, thereby increasing potato and maize yield of smallholder farmers (Beneduzi et al., Citation2012). Similarly, Pseudomonas aeruginosa is used against bacterial blight caused by Xanthomonas oryzae pv. oryza, and Rhizoctonia solani, which are major rice diseases in West Africa (Mali, Senegal, Nigeria, Niger etc.) (Nga et al., Citation2013).
5.4.2. Volatile organic compounds (VOCs)
VOCs are part of the normal metabolic activities of microorganisms and play important roles in stimulating plant growth and as signals in plant-microbe interactions (Insam & Seewald, Citation2010). Though plants produce VOCs, soil microbial synthesised VOCs, which include acetone, 3-butanediol, terpenes, jasmonates and isoprene are good source of natural compounds that can increase crop productivity. VOCs from rhizobacteria cause an increase in the biosynthesis of essential oil and growth parameters in Mentha piperita (Peppermint) (Santoro, Zygadlo, Giordano, & Banchio, Citation2011).
5.4.3. Bioremediation
Rhizobacteria in consortium with AMF are now being employed to increase the solubility and clean-up of heavy metals in contaminated agricultural soil, thereby increasing the available arable land for smallholder farmers (Khan, Citation2014; Singh, Pandey, & Singh, Citation2011). El-Kabbany (Citation1998) evaluated the economic importance of beneficial microbes in bioremediation of major pesticides (organophosphate, carbamate, and chlorinated organic compounds) in Egypt. It was reported that PGPRs are potential remediation agent for pesticide-contaminated soil. Similarly, Bello-Akinosho, Adeleke, Swanevelder, and Thantsha (Citation2015) in a laboratory study on degradation of Polycyclic Aromatic Hydrocarbon (PAH) isolated Pseudomonas sp. strain 10–1B from artificially polluted soil. This strain is useful in bioremediation and biofertilization due to its ability to degrade PAH compounds and improve soil fertility.
Bioremediation technology has been successfully used in many sub-Saharan African countries. For instance, in crude oil contaminated soil of Ogoni land, in Delta state, Nigeria (Zabbey, Sam, & Onyebuchi, Citation2017) and in creosote-contaminated soil in South Africa (Atagana, Citation2004). The technology has also been proposed for the clean-up of the pesticide-contaminated soil in Tanzania (Kishimba et al., Citation2004). Biofertilisers such as cyanobacteria (J. Singh, Kumar, Rai, & Singh, Citation2016), Azospirillum and Burkholderia (Mathew et al., Citation2014), Pseudomonas, Bacillus (Adeleke, Cloete, & Khasa, Citation2012), Rhizobium and Enterobacter (Jain & Khichi, Citation2014) and Aspergillus and Penicillium (Abdel-Aziz, Citation2004) have also been found useful in bioremediation. The dual functions of these beneficial organisms in bioremediation and soil fertilisation have made them a significant ISFM technology (Bello-Akinosho et al., Citation2016).
5.4.4. Water stress resistance
Many African countries, especially the arid and semi-arid areas, have long drought season and this has caused limitation to plant growth (Falkenmark & Rockström, Citation2008). In this situation, biofertilisers, which enhance plant water-stress tolerance, is of immeasurable importance (Dimkpa, Weinand, & Asch, Citation2009; Hassen et al., Citation2016). The production of auxins, cytokinins, gibberellins and 1-aminocyclopropane-1-carboxylate (ACC) deaminase by some biofertilisers has been reported to improve plant water stress tolerance (Khalil & El-Noemani, Citation2015; Mayak, Tirosh, & Glick, Citation2004). Gururani et al. (Citation2013) observed an increase in water stress tolerance of potato plants when inoculated with Bacillus sp. that enhanced ACC deaminase activity, phosphate solubilisation, and siderophore production. Similarly, Aroca and Ruiz-Lozano (Citation2009) and Mayak et al. (Citation2004) reported an increase in water resistance of pepper and tomato plant grown on water deficient soil when inoculated with PGPRs. Essentially, under water-stressed conditions, AMF with their hyphae make available substantial amounts of ammonium and nitrate to the host plant (Wu & Xia, Citation2006). Therefore, biofertiliser has a great economic importance in improving the productivity of smallholder farmers in seasons of drought, especially in drought-prone sub-Saharan African countries such as South Africa, Kenya, Uganda, Ethiopia and Somalia (Kaushal & Wani, Citation2016).
6. Conclusion
This review highlighted soil fertility challenges and the huge economic importance associated with the use of biofertilisers in improving crop productivity among smallholder farmers in sub-Saharan Africa. From the different literature reviewed, the use of biofertiliser has been established to increase plant growth and yield, as well as improve soil quality. In addition, biofertiliser could also protect the natural environment and soil biodiversity. Certain biofertilisers produce metabolites that protect plants from pest and disease attack. The environmentally friendly property of biofertiliser, as well as its great potential in sustainable agriculture, have accentuated the need to reduce, if not replace, the use of agrochemical inputs with biofertilisers.
The resource-poor farmers who cultivate on nutrient-poor sub-Saharan African soil need a cost-effective and efficient technology to increase yield and profitability. It is uninteresting that the intensification in the use of chemical fertilisers has mainly focused on productivity with little or no concern about the increasing cost and ecological damage. This review revealed that the cost-benefit ratio in using biofertiliser is higher than any other nutrient management practice especially the inorganic fertilisers. It is, therefore, important to introduce smallholder farmers to biofertiliser technology through extension education and agronomic training such as on-site training. The production process of biofertiliser technology is simple and requires less capital, technology and workforce, unlike inorganic fertiliser production that requires huge energy, high capital base and a significant amount of manpower. Substituting inorganic fertilisers with biofertiliser will not only increase productivity and profitability of smallholder farmers, it will ultimately build a robust agricultural economy in Africa devoid of any known environmental challenges.
In conclusion, there is an urgent need to improve the awareness and use of biofertiliser among sub-Saharan smallholder farmers. Research studies on efficient microbial strain production, optimisation of product design and biofertiliser business management as well as extension programs and product-marketing strategies are essential to achieving these objectives. It is pertinent to emphasise that sub-Saharan African government have crucial roles to play in ensuring biofertiliser technology is fully adopted as the first choice in our quest to address soil fertility challenges. Their support can be in the form of subsidy or materials to farmers. Apart from training the smallholder farmers, the accessibility of the product is also essential.
Funding
This work was supported by the National Research Foundation (NRF) [grant number 98692].
Additional information
Notes on contributors
Adekunle Raimi
Adekunle Raimi is a Masters student in Environmental Science at the University of South Africa (UNISA), South Africa. He is also a research student at ARC-Soil, Climate and Water (ARC-SCW). His research interests are biotechnology and soil nutrient management, specifically the use of biofertilizer in sustainable agriculture.
Rasheed Adeleke
Adeleke Rasheed is a Professor, Senior Researcher and Research Group leader. He obtained his PhD degree from the University of Pretoria, Department of Microbiology and Plant Pathology. His current research interests include microbial ecology, bioremediation, soil biotechnology and plant-microbe interactions. He is a member of the Society for Applied Microbiology and South African Society for Microbiology, and serves on the review board of several journals. He is also an adjunct Senior Lecturer at North-West University, Potchefstroom campus.
Ashira Roopnarain
Ashira Roopnarain is a postdoctoral research fellow at the ARC-SCW. She obtained her PhD from University of Witwatersrand, School of Molecular and Cell Biology. Her research interests include biotechnology and bioenergy, specifically biogas and biofertilizer production from organic waste.
References
- Abbas, I. (2016). The effect of neem leaves and poultry manure in soil amendments on the growth and yield of cucumber in Ohawu. Hamburg: Anchor Academic Publishing.
- Abdel-Aziz, O. (2004). Bioremediation of soil contaminated with some heavy metals using nuclear techniques (Master’s thesis). Al Azhar University, Egypt, INIS. Retrieved from http://inis.iaea.org/search/search.aspx?orig_q=RN:37089663
- Abdullah, F. A., & Samah, B. A. (2013). Factors impinging farmers’ use of agriculture technology. Asian Social Science, 9(3), 120.
- Abe, S. S., Buri, M. M., Issaka, R. N., Kiepe, P., & Wakatsuki, T. (2010). Soil fertility potential for rice production in West African Lowlands. Japan Agricultural Research Quarterly: JARQ, 44(4), 343–355.10.6090/jarq.44.343
- Adeleke, R. A., Cloete, T. E., Bertrand, A., & Khasa, D. P. (2010). Mobilisation of potassium and phosphorus from iron ore by ectomycorrhizal fungi. World Journal of Microbiology and Biotechnology, 26(10), 1901–1913.10.1007/s11274-010-0372-0
- Adeleke, R., Cloete, E., & Khasa, D. (2010). Isolation and identification of iron ore-solubilising fungus. South African Journal of Science, 106(9–10), 1–6.
- Adeleke, R., Cloete, T., & Khasa, D. (2012). Culturable microorganisms associated with Sishen iron ore and their potential roles in biobeneficiation. World Journal of Microbiology and Biotechnology, 28(3), 1057–1070.10.1007/s11274-011-0904-2
- Adeleke, R., & Dames, J. F. (2014). Kalaharituber pfeilii and associated bacterial interactions. South African Journal of Botany, 90, 68–73.10.1016/j.sajb.2013.10.003
- Adeleke, R., Nwangburuka, C., & Oboirien, B. (2017). Origins, roles and fate of organic acids in soils: A review. South African Journal of Botany, 108, 393–406.10.1016/j.sajb.2016.09.002
- Adesemoye, A., Torbert, H., & Kloepper, J. (2008). Enhanced plant nutrient use efficiency with PGPR and AMF in an integrated nutrient management system. Canadian Journal of Microbiology, 54(10), 876–886.10.1139/W08-081
- Aggani, S. L. (2013). Development of biofertilisers and its future perspective. Scholars Academic Journal of Pharmacy, 2(4), 327–332.
- Ahemad, M., & Khan, M. (2010). Influence of selective herbicides on plant growth-promoting traits of phosphate solubilising Enterobacter asburiae strain PS2. Research Journal of Microbiology, 5(9), 849–857.
- Ahemad, M., & Kibret, M. (2014). Mechanisms and applications of plant growth promoting rhizobacteria: Current perspective. Journal of King Saud University-Science, 26(1), 1–20.10.1016/j.jksus.2013.05.001
- Ahlers, T., Kohli, H. S., & Sood, A. (2013). Africa 2050: Realizing the continent’s full potential. Global Journal of Emerging Market Economies, 5(3), 153–213.10.1177/0974910113505790
- Ahmad, F., Ahmad, I., & Khan, M. S. (2005). Indole acetic acid production by the indigenous isolates of Azotobacter and fluorescent Pseudomonas in the presence and absence of tryptophan. Turkish Journal of Biology, 29(1), 29–34.
- Ahmed, H. F., & El-Araby, M. M. (2012). Evaluation of the influence of nitrogen-fixing, phosphate solubilising and potash mobilising biofertilisers on growth, yield, and fatty acid constituents of oil in peanut and sunflower. African Journal of Biotechnology, 11(43), 10079–10088.
- Ahsan, M. L., Ali, A., & Ahmed, I. (2012). Biofertiliser a highly potent alternative to chemical fertilisers: Uses and future prospects. Journal of Chemical Engineering and Biological Science, 6(4), 10–23.
- Akgül, D., & Mirik, M. (2008). Biocontrol of Phytophthora capsici on pepper plants by Bacillus megaterium strains. Journal of Plant Pathology, 90(1), 29–34.
- Altomare, C., Norvell, W., Björkman, T., & Harman, G. (1999). Solubilisation of phosphates and micronutrients by the plant-growth-promoting and biocontrol fungus Trichoderma harzianum Rifai 1295-22. Applied and Environmental Microbiology, 65(7), 2926–2933.
- Antoun, H., Beauchamp, C. J., Goussard, N., Chabot, R., & Lalande, R. (1998). Potential of Rhizobium and Bradyrhizobium species as plant growth promoting rhizobacteria on non-legumes: Effect on radishes (Raphanus sativus L.). Plant and Soil, 204(1), 57–67.10.1023/A:1004326910584
- Aroca, R., & Ruiz-Lozano, J. M. (2009). Induction of plant tolerance to semi-arid environments by beneficial soil microorganisms– A review. In E. Lichtfouse (Eds.), Climate change, intercropping, pest control and beneficial microorganisms. Sustainable agricultural reviews (Vol. 2, pp. 121–135). Dordrecht, Springer10.1007/978-90-481-2716-0
- Arora, N. K., Khare, E., & Maheshwari, D. K. (2010). Plant growth promoting rhizobacteria: Constraints in bioformulation, commercialization, and future strategies. In D. Maheshwari (Ed.), Microbiology Monographs (Vol. 18, pp. 97–116). Berlin Heidelberg: Springer.
- Arora, N. K., Khare, E., Oh, J. H., Kang, S. C., & Maheshwari, D. K. (2008). Diverse mechanisms adopted by fluorescent Pseudomonas PGC2 during the inhibition of Rhizoctonia solani and Phytophthora capsici. World Journal of Microbiology and Biotechnology, 24(4), 581–585.10.1007/s11274-007-9505-5
- Asenso-Okyere, K., & Jemaneh, S. (2012). Increasing agricultural Productivity and enhancing food security in Africa: New challenges and opportunities. Washington, DC: International Food Policy Research Institute.
- Atagana, H. (2004). Bioremediation of creosote-contaminated soil in South Africa by landfarming. Journal of Applied Microbiology, 96(3), 510–520.10.1111/jam.2004.96.issue-3
- Bahadur, I., Meena, V. S., & Kumar, S. (2014). Importance and application of potassic biofertiliser in Indian agriculture. International Research Journal of Biological Sciences, 3(12), 80–85.
- Bambara, S., & Ndakidemi, P. A. (2010). Changes in selected soil chemical properties in the rhizosphere of Phaseolus vulgaris L. supplied with Rhizobium inoculants, molybdenum and lime. Scientific Research and Essays, 5(7), 679–684.
- Banayo, N. P. M., Cruz, P. C., Aguilar, E. A., Badayos, R. B., & Haefele, S. M. (2012). Evaluation of biofertilisers in irrigated rice: Effects on grain yield at different fertiliser rates. Agriculture, 2(4), 73–86.10.3390/agriculture2010073
- Bationo, A., Hartemink, A., Lungu, O., Naimi, M., Okoth, P., Smaling, E., & Thiombiano, L. (2012). African soils: Their productivity and profitability of fertiliser use. In J. Kihara, D. Fatondji, J. W. Jones, G. Hoogenboom, R. Tabo, & Bationo A. (Eds.), Improving soil fertility recommendation in africa using decision support system for agrotechnology transfer (pp. 19–42). New York: Springer.10.1007/978-94-007-2960-5
- Beijerinck, M. (1888). The root-nodule bacteria. Botanische Zeitung, 46, 725–804.
- Bekunda, M., Nkonya, E., Mugendi, D., & Msaky, J. (2002). Soil fertility status, management, and research in East Africa. East African Journal of Rural Development, 20, 94–112.
- Bello-Akinosho, M., Adeleke, R., Swanevelder, D., & Thantsha, M. (2015). Draft genome sequence of Pseudomonas sp. strain 10-1B, a polycyclic aromatic hydrocarbon degrader in contaminated soil. Genome Announcements, 3(3), e00325-15.
- Bello-Akinosho, M., Makofane, R., Adeleke, R., Thantsha, M., Pillay, M., & Chirima, G. J. (2016). Potential of polycyclic aromatic hydrocarbon-degrading bacterial isolates to contribute to soil fertility. BioMed Research International, 2016(2016), 1–10.
- Beneduzi, A., Ambrosini, A., & Passaglia, L. M. (2012). Plant growth-promoting rhizobacteria (PGPR): Their potential as antagonists and biocontrol agents. Genetics and Molecular Biology, 35(4 suppl 1), 1044–1051.10.1590/S1415-47572012000600020
- Benkeblia, N., & Francis, C.A. (2014). Agroecology applications in tropical agriculture systems. In N. Benkeblia (Eds.), Agroecology, Ecosystems, and Sustainability (pp. 201–220). Boca Raton, FL, FL CRC Press, Taylor & Francis Group.
- Bernhard, A. (2010). The nitrogen cycle: Processes, players, and human impact. Nature Education Knowledge, 2(2), 12.
- Berraho, E., Lesueur, D., Diem, H., & Sasson, A. (1997). Iron requirement and siderophore production in Rhizobium ciceri during growth on an iron-deficient medium. World Journal of Microbiology and Biotechnology, 13(5), 501–510.10.1023/A:1018553022960
- Bhardwaj, D., Ansari, M. W., Sahoo, R. K., & Tuteja, N. (2014). Biofertilisers function as key player in sustainable agriculture by improving soil fertility, plant tolerance and crop productivity. Microbial Cell Factories, 13(1), 1.
- Bhattacharjee, R., & Dey, U. (2014). Biofertiliser, a way towards organic agriculture: A review. African Journal of Microbiology Research, 8(24), 2332–2343.
- Bhattacharyya, P. (2014). Biofertiliser use in organic farming: A practical and challenging approach. In P. k. Shetty, C. Alvares, & A.K. Yadav (Eds.), Organic Farming and Sustainability (p. 157). Bangalore: National Institute of Advanced Studies.
- Bhattacharyya, P., & Jha, D. (2012). Plant growth-promoting rhizobacteria (PGPR): Emergence in agriculture. World Journal of Microbiology and Biotechnology, 28(4), 1327–1350.10.1007/s11274-011-0979-9
- Boraste, A., Vamsi, K., Jhadav, A., Khairnar, Y., Gupta, N., Trivedi, S., & Mujapara, A. (2009). Biofertilisers: A novel tool for agriculture. International Journal of Microbiology Research, 1(2), 23–31.
- Boyer, E. W., Howarth, R. W., Galloway, J. N., Dentener, F. J., Cleveland, C., Asner, G. P., & Vörösmarty, C. (2004). Current nitrogen inputs to world regions. In A. R. Mosier, J. K. Syers, & J. R. Freney (Eds.), Agriculture and the nitrogen cycle: Assessing the impacts of fertiliser use on food production and the environment (pp. 221–230). Washington, DC: Island Press.
- Bühmann, C., Beukes, D., & Turner, D. (2006). Plant nutrient status of soils of the Lusikisiki area, Eastern Cape Province. South African Journal of Plant and Soil, 23(2), 93–98.10.1080/02571862.2006.10634737
- Carvajal-Muñoz, J., & Carmona-Garcia, C. (2012). Benefits and limitations of biofertilization in agricultural practices. Livestock Research for Rural Development, 24(3), 1–8.
- Cervantes-Godoy, D., & Dewbre, J. (2010). Economic importance of agriculture for poverty reduction. OECD Food Agriculture and Fisheries (p. 23). Paris: OECD Publishing.
- Chandler, D., Bailey, A. S., Tatchell, G. M., Davidson, G., Greaves, J., & Grant, W. P. (2011). The development, regulation and use of biopesticides for integrated pest management. Philosophical Transactions of the Royal Society B: Biological Sciences, 366(1573), 1987–1998.10.1098/rstb.2010.0390
- Chianu, J. N., Nkonya, E. M., Mairura, F., Chianu, J. N., & Akinnifesi, F. (2010). Biological nitrogen fixation and socioeconomic factors for legume production in sub-Saharan Africa: A review. Agronomy for Sustainable Development, 31(1), 139–154.
- Cho, R. (2013). Phosphorus: Essential to life-are we running out? -State of the planet. Earth Institute, Columbia University. Retrieved from http://blogs.ei.columbia.edu/2013/04/01/phosphorus-essential-to-life-are-we-running-out/
- Collier, P., & Dercon, S. (2014). African agriculture in 50 years: Smallholders in a rapidly changing world? World Development, 63, 92–101.10.1016/j.worlddev.2013.10.001
- Deckers, J. (1993). Soil fertility and environmental problems in different ecological zones of the developing countries in Sub-Saharan Africa. In H. van Reuler & W. H. Prins (Eds.), The role of plant nutrients for sustainable food production in sub-Saharan Africa (pp. 37–52). Wageningen: Posen & Looijen. Retrieved from http://library.wur.nl/WebQuery/file/isric/fulltext/isricu_i14047_001.pdf#page=44
- Denning, G., Kabambe, P., Sanchez, P., Malik, A., Flor, R., Harawa, R., & Magombo, C. (2009). Input subsidies to improve smallholder maize productivity in Malawi: Toward an African Green Revolution. PLoS Biology, 7(1), e1000023.
- de Valença, A. W., & Bake, A. (2016). Micronutrient management for improving harvests, human nutrition, and the environment. Scientific Project, Assigned by Food & Business Knowledge Platform. Wageningen: Wageningen University.
- Diao, X., Thurlow, J., Benin, S., & Fan, S. (2012). Strategies and priorities for African agriculture: Economywide perspectives from country studies. Washinton DC: International Food Policy Research Institute (IFPRI). doi:10.2499/9780896291959
- Dighe, N. S., Shukla, D., Kalkotwar, R. S., Laware, R. B., Bhawar, S. B., & Gaikwad, R. W. (2010). Nitrogenase enzyme: A review. Der Pharmacia Sinica, 1(2), 77–84.
- Dimkpa, C., Weinand, T., & Asch, F. (2009). Plant–rhizobacteria interactions alleviate abiotic stress conditions. Plant, Cell & Environment, 32(12), 1682–1694.10.1111/j.1365-3040.2009.02028.x
- Diniz, M.A., Teixeira, G., & Carrapico, F. (2015). Azolla as a biofertiliser in Africa. A challenge for the future. Revista de Ciências Agrárias, 23(3): 120–138.
- Dioula, B. M., Deret, H., Morel, J., Vachat, E., & Kiaya, V. (2013). enhancing the role of smallholder farmers in achieving sustainable food and nutrition security. Paper presented at the ICN2, Second International Conference on Nutrition, Rome: Food and Agriculture Organization of the United Nations. Retrieved from http://www.fao.org/3/a-as563.pdf
- Druilhe, Z., & Barreiro-Hurlé, J. (2012). Fertiliser subsidies in sub-Saharan Africa. ESA (Working Paper No. 12). Rome, FAO of the United Nations. Retrieved from http://www.fao.org/3/a-ap077e.pdf
- Egamberdiyeva, D. (2007). The effect of plant growth promoting bacteria on growth and nutrient uptake of maize in two different soils. Applied Soil Ecology, 36(2-3), 184–189.10.1016/j.apsoil.2007.02.005
- El-Kabbany, S. (1998, December). Evaluation of four biofertiliser for bioremediation of pesticide-contaminated soil. Paper presented at the International Conference on Hazardous Waste: Sources, Effects and Management. Cairo. Retrieved from http://www.iaea.org/inis/collection/NCLCollectionStore/_Public/31/026/31026700.pdf
- Esitken, A., Yildiz, H. E., Ercisli, S., Figen Donmez, M. F., Turan, M., & Gunes, A. (2010). Effects of plant growth promoting bacteria (PGPB) on yield, growth and nutrient contents of organically grown strawberry. Scientia Horticulturae, 124(1), 62–66.10.1016/j.scienta.2009.12.012
- Eswaran, H., Almaraz, R., van den Berg, E., & Reich, P. (1997). An assessment of the soil resources of Africa in relation to productivity. Geoderma, 77(1), 1–18.10.1016/S0016-7061(97)00007-4
- Evert-Jan, Q., & Aniek, H. (2014). Final Report on Dutch Food Security Policy Consultation. Food and Business knowledge Platform. doi:10.13140/RG.2.2.28107.59680
- Falkenmark, M., & Rockström, J. (2008). Building resilience to drought in desertification-prone savannas in Sub-Saharan Africa: The water perspective. Natural Resources Forum, 32(2), 93–102.10.1111/j.1477-8947.2008.00177.x
- FAO. (2015). FAOSTAT3. 21.09.2016. Food and Agricultural Organisation of the United Nations. Retrieved from http://www.fao.org/faostat/en/#data/
- Figueiredo, M. V. B., Seldin, L., de Araujo, F. F., & Mariano, R. L. R. (2011). Plant growth promoting rhizobacteria: Fundamentals and applications. In D. Maheshwari (Ed.), Plant growth and health promoting bacteria (pp. 21–43). Berlin, Heidelberg: Springer.10.1007/978-3-642-13612-2
- Francis, J. A., & van Huis, A. (2016). Why focus on innovation systems: Implications for research and policy. In J. Francis, L. Mytalka, A. van Huis, & N. Röling, Innovation Systems: Towards effective strategies in support of smallholder farmers (pp. 8–23). Wageningen: CTA. Retrieved from https://publications.cta.int/en/publications/publication/1829/
- Galloway, J. N. (1998). The global nitrogen cycle: Changes and consequences. Environmental Pollution, 102(1), 15–24.10.1016/S0269-7491(98)80010-9
- García-Fraile, P., Menéndez, E., & Rivas, R. (2015). Role of bacterial biofertilizers in agriculture and forestry. AIMS Bioengineering, 2(3), 183–205.10.3934/bioeng.2015.3.183
- Garg, N., & Chandel, S. (2011). Effect of mycorrhizal inoculation on growth, nitrogen fixation, and nutrient uptake in Cicer arietinum (Woomer, Ongoma, & Wafullah) under salt stress. Turkish Journal of Agriculture and Forestry, 35(2), 205–214.
- Ghosh, P. K., Kumar De, T., & Maiti, T. K. (2015). Production and Metabolism of Indole Acetic Acid in Root Nodules and Symbiont (Rhizobium undicola) Isolated from Root Nodule of Aquatic Medicinal Legume Neptunia oleracea Lour. Journal of Botany, 2015, 1–11.
- Giller, K. E., Murwira, M. S., Dhliwayo, D. K., Mafongoya, P. L., & Mpepereki, S. (2011). Soyabeans and sustainable agriculture in southern Africa. International Journal of Agricultural Sustainability, 9(1), 50–58.10.3763/ijas.2010.0548
- Godfray, H. C. J., Beddington, J. R., Crute, I. R., Haddad, L., Lawrence, D., Muir, J. F., … Toulmin, C. (2010). Food security: The challenge of feeding 9 billion people. Science, 327(5967), 812–818.10.1126/science.1185383
- Grady, E. N., MacDonald, J., Liu, L., Richman, A., & Yuan, Z.-C. (2016). Current knowledge and perspectives of Paenibacillus: A review. Microbial Cell Factories, 15(1), 203.10.1186/s12934-016-0603-7
- Guimarães, S. L., Neves, L. C. R. D., Bonfim-Silva, E. D. N. A., & Campos, D. T. D. S. (2016). Development of pigeon pea inoculated with rhizobium isolated from cowpea trap host plants. Revista Caatinga, 29(4), 789–795.10.1590/1983-21252016v29n402rc
- Guo, L., Rasool, A., & Li, C. (2013). Antifungal substances of bacterial origin and plant disease management. In D. Maheshwari (Ed.), Bacteria in Agrobiology: Disease Management (pp. 473–485). Berlin, Heidelberg: Springer.10.1007/978-3-642-33639-3
- Gupta, G., Panwar, J., Akhtar, M. S., & Jha, P. N. (2012). Endophytic nitrogen-fixing bacteria as biofertiliser. In E. Lichtfouse (Ed.), Sustainable Agriculture Reviews (pp. 183–221). Dordrecht: Springer.10.1007/978-94-007-5449-2
- Gupta, R. P., Kalia, A., & Kapoor, S. (2007). Bioinoculants: A step towards sustainable agriculture. New Delhi: New India Publishing.
- Gururani, M. A., Upadhyaya, C. P., Baskar, V., Venkatesh, J., Nookaraju, A., & Park, S. W. (2013). Plant growth-promoting rhizobacteria enhance abiotic stress tolerance in Solanum tuberosum Through inducing changes in the expression of ROS-scavenging enzymes and improved photosynthetic performance. Journal of Plant Growth Regulation, 32(2), 245–258.10.1007/s00344-012-9292-6
- Han, H., & Lee, K. (2005). Phosphate and potassium solubilising bacteria effect on mineral uptake, soil availability and growth of eggplant. Research Journal of Agriculture and Biological Sciences, 1(2), 176–180.
- Harris, F. (2002). Management of manure in farming systems in semi-arid West Africa. Experimental Agriculture, 38(02), 131–148.
- Hassen, A. I., Bopape, F. L., & Sanger, L. K. (2016). Microbial inoculants as agents of growth promotion and abiotic stress tolerance in plants. In D. Singh, H. Singh, & R. Prabha (Eds.), Microbial inoculants in sustainable agricultural productivity (pp. 23–36). New Delhi: Springer.10.1007/978-81-322-2647-5
- Hayat, R., Ali, S., Amara, U., Khalid, R., & Ahmed, I. (2010). Soil beneficial bacteria and their role in plant growth promotion: A review. Annals of Microbiology, 60(4), 579–598.10.1007/s13213-010-0117-1
- Heinonen-Tanski, H., Mohaibes, M., Karinen, P., & Koivunen, J. (2006). Methods to reduce pathogen microorganisms in manure. Livestock Science, 102(3), 248–255.10.1016/j.livsci.2006.03.024
- Hellriegal, H., & Wilfarth, H. (1886). Unter suchungenurnber die stickst off - Nahrung der Graminum and leguminoseu. Beilagcheft. Z. vers. Rubensur lerine, 1–234 (Cited from Biological fixation of atmospheric nitrogen by Mishustin, E.N. and Shilnikova, London, UK, Macmillan Press Limited)
- Henao, J., & Baanante, C. A. (1999). Estimating rates of nutrient depletion in soils of agricultural lands of Africa, International Fertiliser Development Center (IFDC). Technical Bulletin. Retrieved from http://pdf.usaid.gov/pdf_docs/pnacf868.pdf
- Henao, J., & Baanante, C. (2006). Agricultural production and soil nutrient mining in Africa: Implications for resource conservation and policy development: International Center for Soil Fertility and Agricultural Development. Muscle Shoals, AL: IFDC. Retrieved from https://ifdcorg.files.wordpress.com/2015/01/t-72-agricultural_production_and_soil_nutrient.pdf
- Herrmann, L., Atieno, M., Brau, L., & Lesueur, D. (2015). Microbial quality of commercial inoculants to increase BNF and nutrient use efficiency. In F. J. de Bruijn (Ed.), Biological Nitrogen Fixation (pp. 1031–1040). Hoboken, NJ: Wiley.10.1002/9781119053095
- Hristov, A., Oh, J., Lee, C., Meinen, R., Montes, F., Ott, T., … Adesogan, A. (2013). Mitigation of greenhouse gas emissions in livestock production: A review of technical options for non-CO2 emissions. FAO Animal Production and Health Paper No, 177, 1–206.
- Hu, T.-W., & Lee, A. H. (2015). Commentary: Tobacco control and tobacco farming in African countries. Journal of Public Health Policy, 36(1), 41–51.10.1057/jphp.2014.47
- Insam, H., & Seewald, M. S. (2010). Volatile organic compounds (VOCs) in soils. Biology and Fertility of Soils, 46(3), 199–213.10.1007/s00374-010-0442-3
- International Fund for Agricultural Development. (2013). Smallholders, food security and the environment. Rome: International Fund for Agricultural Development, United Nation Environmental Program UNEP. Retrieved from http://www.polity.org.za/attachment.php?aa_id=44788.
- Jacobs, P., & Baiphethi, M. (2015). The contribution of subsistence farming to food security in South Africa. Agricultural Economic Research, Policy and Practice in South Africa, 48(4), 459–482.
- Jain, P., & Khichi, D. S. (2014). Phosphate solubilising microorganism (PSM): An eco-friendly biofertiliser and pollution manager. Journal of Dynamics in Agricultural Research, 1(4), 23–28.
- Karadeniz, A., Topcuoğlu, Ş., & İnan, S. (2006). Auxin, gibberellin, cytokinin and abscisic acid production in some bacteria. World Journal of Microbiology and Biotechnology, 22(10), 1061–1064.10.1007/s11274-005-4561-1
- Kariuki, J. G. (2011). The future of agriculture in Africa (The Pardee papers/No. 15). Massachusetts, Boston: Boston University: The Fredrick S. Pardee Center for the Study Longer-Range. Retrieved from http://www.bu.edu/pardee/files/2011/11/15-PP.pdf?PDF=pardee-papers-15-africa
- Karuku, J. (2014, June 20). Smallholder farming the surest route to Africa growth. Mail & Guardian. Retrieved from https://mg.co.za/article/2014-06-19-smallholder-farming-the-surest-route-to-african-growth
- Kaushal, M., & Wani, S. P. (2016). Plant-growth-promoting rhizobacteria: Drought stress alleviators to ameliorate crop production in drylands. Annals of Microbiology, 66(1), 35–42.10.1007/s13213-015-1112-3
- Keino, L., Baijukya, F., Ng’etich, W., Otinga, A. N., Okalebo, J. R., Njoroge, R., & Mukalama, J. (2015). Nutrients Limiting Soybean (glycine max l) growth in acrisols and ferralsols of Western Kenya. PLoS ONE, 10(12), 1–20.
- Khalil, S., & El-Noemani, A. (2015). Effect of biofertilisers on growth, yield, water relations, photosynthetic pigments and carbohydrates contents of Origanum vulgare L. plants grown under water stress conditions. American-Eurasian Journal of Sustainable Agriculture, 9(4), 60–73.
- Khan, M. A. (2014). Microbiological Solution to Environmental Problems - A Review on Bioremediation. International Journal of Pure Applied. BioScience, 2(6), 295–303.
- Kishimba, M., Henry, L., Mwevura, H., Mmochi, A., Mihale, M., & Hellar, H. (2004). The status of pesticide pollution in Tanzania. Talanta, 64(1), 48–53.10.1016/j.talanta.2003.11.047
- Kolavalli, S., & Vigneri, M. (2011). Cocoa in Ghana: Shaping the success of an economy. Yes, Africa can: Success stories from a dynamic continent, 201–218.
- Koskei, R. C., Langat, J. K., Koskei, E. C., & Oyugi, M. A. (2013). Determinants of agricultural information access by smallholder tea farmers in Bureti District. Kenya. Asian Journal of Agricultural Sciences, 5(5), 102–107. Retrieved from http://maxwellsci.com/print/ajas/v5-102-107.pdf
- Kumar, A., Biswas, T., Singh, N., & Lal, E. (2014). Effect of gibberellic acid on growth, quality and yield of tomato (Lycopersicon esculentum). Journal of Agriculture and Veterinary Science, 7(4), 28–30.
- Kumar, H., Bajpai, V. K., Dubey, R., Maheshwari, D., & Kang, S. C. (2010). Wilt disease management and enhancement of growth and yield of Cajanus cajan (L) var. Manak by bacterial combinations amended with chemical fertiliser. Crop Protection, 29(6), 591–598.10.1016/j.cropro.2010.01.002
- Leigh, J., Hodge, A., & Fitter, A. H. (2009). Arbuscular mycorrhizal fungi can transfer substantial amounts of nitrogen to their host plant from organic material. New Phytologist, 181(1), 199–207.10.1111/nph.2009.181.issue-1
- Lesueur, D., Deaker, R., Herrmann, L., Bräu, L., & Jansa, J. (2016). The production and potential of biofertilisers to improve crop yields. Bioformulations: For Sustainable Agriculture, 71–92.
- Lichtfouse, E., Navarrete, M., Debaeke, P., Souchère, V., Alberola, C., & Ménassieu, J. (2009). Agronomy for sustainable agriculture: A review. Agronomy for Sustainable Development, 29, 1–6.10.1051/agro:2008054
- Liu, E., Yan, C., Mei, X., He, W., Bing, S. H., Ding, L., … Fan, T. (2010). Long-term effect of chemical fertilizer, straw, and manure on soil chemical and biological properties in northwest China. Geoderma, 158(3–4), 173–180.10.1016/j.geoderma.2010.04.029
- Liverpool-Tasie, L. S. O., Omonona, B. T., Sanou, A., & Ogunleye, W. (2015). Is increasing inorganic fertiliser use in Sub-Saharan Africa a profitable proposition? Evidence from Nigeria. Evidence from Nigeria (February 1, 2015). World Bank Policy Research Working Paper (7201)
- Lu, G., Coneva, V., Casaretto, J. A., Ying, S., Mahmood, K., Liu, F., … Rothstein, S. J. (2015). OsPIN5b modulates rice (Oryza sativa) plant architecture and yield by changing auxin homeostasis, transport and distribution. The Plant Journal, 83(5), 913–925.10.1111/tpj.2015.83.issue-5
- Lucy, M., Reed, E., & Glick, B. R. (2004). Applications of free living plant growth-promoting rhizobacteria. Antonie van Leeuwenhoek, 86(1), 1–25.10.1023/B:ANTO.0000024903.10757.6e
- Mahdi, S. S., Hassan, G., Samoon, S., Rather, H., Dar, S. A., & Zehra, B. (2010). Biofertilisers in organic agriculture. Journal of Phytology, 2(10), 42–54.
- Malusà, E., & Ciesielska, J. (2014). Biofertilisers: A resource for sustainable plant nutrition. Fertiliser Technology, 1(1), 282–319.
- Malusà, E., Pinzari, F., & Canfora, L. (2016). Efficacy of biofertilisers: Challenges to improve crop production. In D. Singh, H. Singh, & R. Prabha (Eds.), Microbial inoculants in sustainable agricultural productivity (pp. 17–40). Springer: New Delhi.10.1007/978-81-322-2644-4
- Malusá, E., Sas-Paszt, L., & Ciesielska, J. (2012). Technologies for beneficial microorganisms inocula used as biofertilisers. The Scientific World Journal, 2012(2012), 1–12.
- Martínez-Romero, E. (2009). Coevolution in rhizobium -legume symbiosis? DNA and Cell Biology, 28(8), 361–370.10.1089/dna.2009.0863
- Martino, E., Perotto, S., Parsons, R., & Gadd, G. M. (2003). Solubilisation of insoluble inorganic zinc compounds by ericoid mycorrhizal fungi derived from heavy metal polluted sites. Soil Biology and Biochemistry, 35(1), 133–141.10.1016/S0038-0717(02)00247-X
- Mathew, A., Eberl, L., & Carlier, A. L. (2014). A novel siderophore-independent strategy of iron uptake in the genus B urkholderia. Molecular Microbiology, 91(4), 805–820.10.1111/mmi.2014.91.issue-4
- Mayak, S., Tirosh, T., & Glick, B. R. (2004). Plant growth-promoting bacteria that confer resistance to water stress in tomatoes and peppers. Plant Science, 166(2), 525–530.10.1016/j.plantsci.2003.10.025
- Mazid, M., Khan, T., & Mohammad, F. (2011). Role of secondary metabolites in defense mechanisms of plants. Biology and Medicine, 3(2), 232–249.
- Megali, L., Schlau, B., & Rasmann, S. (2015). Soil microbial inoculation increases corn yield and insect attack. Agronomy for Sustainable Development, 35(4), 1511–1519.10.1007/s13593-015-0323-0
- Minde, I., Jayne, T. S., Crawford, E., Ariga, J., & Govereh, J. (2008). Promoting fertiliser use in Africa: Current issues and empirical evidence from Malawi, Zambia, and Kenya. Food Security International Development Policy Syntheses, 54509. Retrieved from http://pdf.usaid.gov/pdf_docs/PNADS615.pdf
- Mohammadi, K. (2012). Phosphorus solubilising bacteria: Occurrence, mechanisms and their role in crop production. Resources and Environment, 2(1), 80–85.
- Mohammadi, K., & Sohrabi, Y. (2012). Bacterial biofertilisers for sustainable crop production: A review. Journal of Agricultural and Biological Science, 7(5), 307–316.
- Moreno-Caselles, J., Moral, R., Perez-Murcia, M., Perez-Espinosa, A., & Rufete, B. (2002). Nutrient value of animal manures in front of environmental hazards. Communications in Soil Science and Plant Analysis, 33(15–18), 3023–3032.10.1081/CSS-120014499
- Morris, M. L., Kelly, V. A., Kopicki, R. J., & Byerlee, D. (2007). Fertiliser use in African agriculture: Lessons learned and good practice guidelines. Washington, DC: The World Bank.10.1596/978-0-8213-6880-0
- Mugabe, J. (1994). Research on biofertilisers: Kenya, Zimbabwe and Tanzania. Biotechnology and Development Monitor, 18, 9–10.
- Mujawar, M. I. (2014). Bacteria and fungi can contribute to nutrients. Journal of King Saud University, 26(1), 1–20.
- Mukhongo, R., Tumuhairwe, J., Ebanyat, P., AbdelgGadi, A., Thuita, M., & Masso, C. (2016). Production and use of arbuscular mycorrhizal fungi inoculum in sub-Saharan Africa: Challenges and ways of improving. International Journal of Soil Science, 11(3), 108–122.10.3923/ijss.2016.108.122
- Mulongoy, K., Gianinazzi, S., Roger, P.-A., & Dommergues, Y. (1992). Biofertilisers: Agronomic and environmental impacts and economics. In E. J. DaSilva, C. Ratledge, & A. Sasson (Eds.), Biotechnology: Economic and social aspects: issues for developing countries (pp. 55–69). Cambridge: Cambridge University Press.10.1017/CBO9780511760075
- Muzari, W., Gatsi, W., & Muvhunzi, S. (2012). The impacts of technology adoption on smallholder agricultural productivity in sub-Saharan Africa: A review. Journal of Sustainable Development, 5(8), 69.
- Mwangi, W. M. (1996). Low use of fertilizers and low productivity in sub-Saharan Africa. Nutrient Cycling in Agroecosystems, 47(2), 135–147.10.1007/BF01991545
- N2Africa. (2015). N2Africa revitalizes legume production in Nigeria. IITA, Research to Nourish Africa. Retrieved from http://www.iita.org/news-item/n2africa-revitalizes-legume-production-nigeria/
- Nagayet, O. (2005). Small farms: Current status and key trends. In IFPRI (International Food Policy Research Institute): The future of small farms: Proceedings of a research workshop, UK, June 26-29 (pp. 355–367). Washington, DC: Wye. Retrieved from http://citeseerx.ist.psu.edu/viewdoc/download?doi=10.1.1.144.1658&rep=rep1&type=pdf
- Nga, N. T., Tien, D. T., Linh, V. T., Nhung, N. T., Tam, N. T., Neergaard, E. D., & Jørgensen, H. (2013). Control of plant diseases by the endophytic rhizobacterial strain Pseudomonas aeruginosa 23 1-1. In M. S. Reddy, O. I. Ilao, P. S. Faylon, W. D. Dar, W. D. Batchelor, R. Sayyed, H. K. Sudini, V. K. Kumar, A. B. Armanda, & S. Gopalkrishnan (Eds.), Recent advances in biofertilisers and biofungicides (PGPR) for sustainable agriculture (pp. 8–18). Newcastle: Cambridge Scholar.
- Ngetich, F. K., Shisanya, C. A., Mugwe, J., Mucheru-Muna, M., Mugendi, D. N. (2012). The potential of organic and inorganic nutrient sources in sub-Saharan African crop farming systems. In J.K. Whalen, (Ed.), Soil fertility improvement and integrated nutrient management–A global perspective (pp. 135–156). Rijeka: INTECH.
- Nkonya, E., Pender, J., Kaizzi, K. C., Kato, E., Mugarura, S., Ssali, H., & Muwonge, J. (2008). Linkages between land management, land degradation, and poverty in sub-Saharan Africa: The case of Uganda (Report No159). Washington, DC: International Food Policy Research Institute.
- Okorogbona, A. O. M., & Adebisi, L. O. (2012). Animal manure for smallholder agriculture in South Africa. In E. Lichtfouse (Ed.), Farming for food and water security, sustainable agricultural reviews (pp. 201–242). Dordrecht: Springer.10.1007/978-94-007-4500-1
- Oldroyd, G. E., Murray, J. D., Poole, P. S., & Downie, J. A. (2011). The rules of engagement in the legume-rhizobial symbiosis. Annual Review of Genetics, 45, 119–144.10.1146/annurev-genet-110410-132549
- Pacanoski, Z. (2009). The myth of organic agriculture. Plant Protection Science, 45(2), 39–48. Retrieved from http://www.agriculturejournals.cz/publicFiles/08127.pdf
- Pal, S., Singh, H., Farooqui, A., & Rakshit, A. (2015). Fungal biofertilisers in Indian agriculture: Perception, demand and promotion. Journal of Eco-friendly Agriculture, 10(2), 101–113.
- Pang, X., & Letey, J. (2000). Organic farming challenge of timing nitrogen availability to crop nitrogen requirements. Soil Science Society of America Journal, 64(1), 247–253.10.2136/sssaj2000.641247x
- Parani, K., & Saha, B. (2012). Prospects of using phosphate solubilising Pseudomonas as biofertiliser. European Journal of Biological Science, 4(2), 40–44.
- Parmar, P., & Sindhu, S. (2013). Potassium solubilisation by rhizosphere bacteria: Influence of nutritional and environmental conditions. Journal of Microbiology Research, 3(1), 25–31.
- Paudel, Y., Pradhan, S., Pant, B., & Prasad, B. (2012). Role of blue-green algae in rice productivity. Agriculture and Biology Journal of North America, 3(8), 332–335.10.5251/abjna.2012.3.8.332.335
- Pérez-Montaño, F., Alías-Villegas, C., Bellogín, R., del Cerro, P., Espuny, M., Jiménez-Guerrero, I., … Cubo, T. (2014). Plant growth promotion in cereal and leguminous agricultural important plants: From microorganism capacities to crop production. Microbiological Research, 169(5–6), 325–336.10.1016/j.micres.2013.09.011
- Pindi, P. K., & Satyanarayana, S. D. V. (2012). Liquid microbial consortium-a potential tool for sustainable soil health. Journal of Biofertilisers & Biopesticides, 3(4), 124.
- Poulton, C., Dorward, A., & Kydd, J. (2010). The future of small farms: New directions for services, institutions, and intermediation. World Development, 38(10), 1413–1428.10.1016/j.worlddev.2009.06.009
- Radzki, W., Gutierrez Mañero, F. G., Algar, E., Lucas García, J. L., García-Villaraco, A., & Ramos Solano, B. R. (2013). Bacterial siderophores efficiently provide iron to iron-starved tomato plants in hydroponics culture. Antonie van Leeuwenhoek, 104(3), 321–330.10.1007/s10482-013-9954-9
- Rai, M. K. (Ed.). (2006). Handbook of microbial biofertilisers. Binghamton, NY: Haworth Press.
- Ramasamy, K., Joe, M. M., Kim, K.-Y., Lee, S.-M., Shagol, C., Rangasamy, A., … Sa, T.-M. (2011). Synergistic effects of arbuscular mycorrhizal fungi and plant growth promoting rhizobacteria for sustainable agricultural production. Korean Journal of Soil Science and Fertilizer, 44(4), 637–649.10.7745/KJSSF.2011.44.4.637
- Rashid, M. I., Mujawar, L. H., Shahzad, T., Almeelbi, T., Ismail, I. M., & Oves, M. (2016). Bacteria and fungi can contribute to nutrients bioavailability and aggregate formation in degraded soils. Microbiological Research, 183, 26–41.10.1016/j.micres.2015.11.007
- Rattso, J., & Torvik, R. (2003). Interactions between agriculture and industry: Theoretical analysis of the consequences of discriminating agriculture in sub-Saharan Africa. Review of Development Economics, 7(1), 138–151.10.1111/rode.2003.7.issue-1
- Reinhold-Hurek, B., Hurek, T., Gillis, M., Hoste, B., Vancanneyt, M., Kersters, K., & De Ley, J. (1993). Azoarcus gen. nov., nitrogen-fixing proteobacteria associated with roots of kallar grass (Leptochloa fusca (L.) Kunth), and description of two species, Azoarcus indigens sp. nov. and Azoarcus communis sp. nov. International Journal of Systematic and Evolutionary Microbiology, 43(3), 574–584.
- Ribaudo, C. M., Krumpholz, E. M., Cassán, F. D., Bottini, R., Cantore, M. L., & Curá, J. A. (2006). Azospirillum sp. promotes root hair development in tomato plants through a mechanism that involves ethylene. Journal of Plant Growth Regulation, 25(2), 175–185.10.1007/s00344-005-0128-5
- Richardson, A. E., Barea, J.-M., McNeill, A. M., & Prigent-Combaret, C. (2009). Acquisition of phosphorus and nitrogen in the rhizosphere and plant growth promotion by microorganisms. Plant and Soil, 321(1–2), 305–339.10.1007/s11104-009-9895-2
- Richardson, A. E., & Simpson, R. J. (2011). Soil microorganisms mediating phosphorus availability update on microbial phosphorus. Plant Physiology, 156(3), 989–996.10.1104/pp.111.175448
- Riggs, P. J., Chelius, M. K., Iniguez, A. L., Kaeppler, S. M., & Triplett, E. W. (2001). Enhanced maize productivity by inoculation with diazotrophic bacteria. Functional Plant Biology, 28(9), 829–836.10.1071/PP01045
- Rodrigues, E. P., Rodrigues, L. S., de Oliveira, A. L. M., Baldani, V. L. D., Dos Santos Teixeira, K. R., Urquiaga, S., & Reis, V. M. (2008). Azospirillum amazonense inoculation: Effects on growth, yield and N2 fixation of rice (Oryza sativa L.). Plant and Soil, 302(1–2), 249–261.10.1007/s11104-007-9476-1
- Ronner, E., Franke, A., Vanlauwe, B., Dianda, M., Edeh, E., Ukem, B., … Giller, K. (2016). Understanding variability in soybean yield and response to P-fertilizer and rhizobium inoculants on farmers’ fields in northern Nigeria. Field Crops Research, 186, 133–145.10.1016/j.fcr.2015.10.023
- Rose, M. T., Phuong, T. L., Nhan, D. K., Cong, P. T., Hien, N. T., & Kennedy, I. R. (2014). Up to 52 % N fertilizer replaced by biofertilizer in lowland rice via farmer participatory research. Agronomy for Sustainable Development, 34(4), 857–868.10.1007/s13593-014-0210-0
- Rosegrant, M. W., Cline, S. A., Li, W., Sulser, T. B., & Valmonte-Santos, R. (2005). Looking ahead: Long-term prospects for Africa’s agricultural development and food security (Vol. 41). Washinton, DC: International Food Policy Research Institute. Retrieved from https://ideas.repec.org/p/fpr/2020dp/41.html
- Rosemarin, A., De Bruijne, G., & Caldwell, I. (2009). Peak phosphorus: The next inconvenient truth. The Broker, 15, 6–9.
- Rosen, C. J., & Bierman, P. M. (Eds.) (2005). Using manure and compost as nutrient sources for fruit and vegetable crops. (Commercial fruit and vegetable production). Minneapolis: University of Minnesota Extension. Retrieved from https://www.extension.umn.edu/garden/fruit-vegetable/using-manure-and-compost/
- Rowell, B., & Hadad, R. (2004). Organic manures and fertilisers for vegetable crops. Lexington: The University of Kentucky, Department of Horticulture. Retrieved from http://www.uky.edu/hort/node/424.
- Roy, R. N., Finck, A., Blair, G. J., & Tandon, H. L. S. (2006). Plant nutrition for food security. A guide for integrated nutrient management (p. 16). Rome: FAO, Fertiliser and Plant Nutrition Bulletin. Retrieved from http://www.fao.org/3/a-a0443e.pdf
- Rudrappa, T., Czymmek, K. J., Pare, P. W., & Bais, H. P. (2008). Root-secreted malic acid recruits beneficial soil bacteria. Plant Physiology, 148(3), 1547–1556.10.1104/pp.108.127613
- Rukuni, M. (2002). Africa: Addressing growing threats to food security. The Journal of nutrition, 132(11), 3443–3448.
- Sanchez, P. A. (2002). Ecology: soil fertility and hunger in Africa. Science, 295(5562), 2019–2020.10.1126/science.1065256
- Sangeeth, K., Bhai, R. S., & Srinivasan, V. (2012). Paenibacillus glucanolyticus, a promising potassium solubilising bacterium isolated from black pepper (Piper nigrum L.) rhizosphere. Journal of Spices and Aromatic. Crops, 21(2), 118–124.
- Santoro, M. V., Zygadlo, J., Giordano, W., & Banchio, E. (2011). Volatile organic compounds from rhizobacteria increase biosynthesis of essential oils and growth parameters in peppermint (Mentha piperita). Plant Physiology and Biochemistry, 49(10), 1177–1182.10.1016/j.plaphy.2011.07.016
- Schröder, J., Cordell, D., Smit, A., & Rosemarin, A. (Eds.). (2010). Sustainable use of phosphorus (EU tender ENV. B1/ETU/2009/0025). Wageningen: Wageningen University and Research Centre: Plant Research International.
- Shaharoona, B., Naveed, M., Arshad, M., & Zahir, Z. A. (2008). Fertiliser-dependent efficiency of Pseudomonas for improving growth, yield, and nutrient use efficiency of wheat (Triticum aestivum L.). Applied Microbiology and Biotechnology, 79(1), 147–155.10.1007/s00253-008-1419-0
- Shanware, A. S., Kalkar, S. A., & Trivedi, M. M. (2014). Potassium Solubilisers: Occurrence, mechanism and their role as competent biofertilisers. International Journal of Current Microbiological Applied Science, 3(9), 622–629.
- Shridhar, B. S. (2012). Review: Nitrogen fixing microorganisms. International Journal of Microbiological Research, 3(1), 46–52.
- Singh, J. S., Kumar, A., Rai, A. N., & Singh, D. P. (2016). Cyanobacteria: A precious bio-resource in agriculture, ecosystem, and environmental sustainability. Frontiers in Microbiology, 7, 529.
- Singh, J. S., Pandey, V. C., & Singh, D. (2011). Efficient soil microorganisms: A new dimension for sustainable agriculture and environmental development. Agriculture, Ecosystems & Environment, 140(3-4), 339–353.10.1016/j.agee.2011.01.017
- Singh, S., Srivastava, K., Sharma, S., & Sharma, A. (2014). Mycorrhizal inoculum production. In Z. Solaiman, L. Abbott, & A. Varma (Eds.), Mycorrhizal Fungi: Use in sustainable agriculture and land restoration (pp. 67–79). Heidelberg, Berlin: Springer.
- Solanki, M. K., Kumar, S., Pandey, A. K., Srivastava, S., Singh, R. K., Kashyap, P. L., … Arora, D. K. (2012). Diversity and antagonistic potential of Bacillus spp. associated to the rhizosphere of tomato for the management of Rhizoctonia solani. Biocontrol Science and Technology, 22(2), 203–217.10.1080/09583157.2011.649713
- Solanki, M. K., Singh, R. K., Srivastava, S., Kumar, S., Kashyap, P. L., Srivastava, A. K., & Arora, D. K. (2014). Isolation and characterization of siderophore producing antagonistic rhizobacteria against Rhizoctonia solani. Journal of Basic Microbiology, 54(6), 585–597.10.1002/jobm.v54.6
- Soltani, A.-A., Khavazi, K., Asadi-Rahmani, H., Omidvari, M., Dahaji, P. A., & Mirhoseyni, H. (2010). Plant growth promoting characteristics in some Flavobacterium spp. isolated from soils of Iran. Journal of Agricultural Science, 2(4), 106–115.
- Sommer, R., Bossio, D., Desta, L., Dimes, J., Kihara, J., Koala, S., … Winowiecki, L. (2013). Profitable and sustainable nutrient management systems for East and Southern African smallholder farming systems- challenges and opportunities: A synthesis of the Eastern and Southern Africa situation in terms of past experiences, present and future opportunities in promoting nutrients use in Africa. Cali Colombia: CIAT, The University of Queensland. QAAFI & CIMMYT. Retrieved from http://hdl.handle.net/10883/4035
- Strange, R. N., & Scott, P. R. (2005). Plant disease: A threat to global food security. Annual. Review of Phytopathology, 43, 83–116.10.1146/annurev.phyto.43.113004.133839
- Sundara, B., Natarajan, V., & Hari, K. (2002). Influence of phosphorus solubilizing bacteria on the changes in soil available phosphorus and sugarcane and sugar yields. Field Crops Research, 77(1), 43–49.10.1016/S0378-4290(02)00048-5
- Sutton, M. A., Bleeker, A., Howard, C., Bekunda, M., Grizzetti, B., De Vries, W., Davidson, E. A. (2013). Our nutrient world: The challenge to produce more food and energy with less pollution. Edinburgh: Centre for Ecology and Hydrology. Retrieved from http://nora.nerc.ac.uk/id/eprint/500700
- Suyal, D. C., Soni, R., Sai, S., & Goel, R. (2016). Microbial inoculants as biofertiliser. In D. Singh & R. Prabha (Eds.), Microbial Inoculants in Sustainable Agricultural Productivity (pp. 311–318). New Delhi: Springer.10.1007/978-81-322-2647-5
- Svotwa, E., Baipai, R., & Jiyane, J. (2009). Organic farming in the smallholder farming sector of Zimbabwe. Electronic Journal of Environmental Agricultural and Food Chemistry, 6(2), 1820–1827.
- Swain, M. R., Naskar, S. K., & Ray, R. C. (2007). Indole-3-acetic acid production and effect on sprouting of yam (Dioscorea rotundata L.) minisetts by Bacillus subtilis isolated from culturable cowdung microflora. Polish Journal of Microbiology, 56(2), 103.
- Taffesse, A.S., Dorosh, P., & Asrat, S. (2011). Crop production in Ethiopia: Regional patterns and trends (Ethiopia Strategy Support Program ESSP II Working Paper 16). Addis Ababa: International Food Policy Research Institute. Retrieved from http://reliefweb.int/sites/reliefweb.int/files/resources/essprn11.pdf
- Tairo, E. V., & Ndakidemi, P. A. (2014). Micronutrients uptake in soybean (Glycine max L.) as affected by Bradyrhizobium japonicum inoculation and phosphorus (p) supplements. World Journal of Soil and Crop Sciences Research, 1(1), 1–9.
- Tamil Nadu Agricultural University (2014). Biofertiliser: In organic farming- Organic inputs and techniques. Coimbatore: TNAU Agritech Portal. Retrieved from http://agritech.tnau.ac.in/org_farm/orgfarm_biofertilisertechnology.html.
- Tawaraya, K., Naito, M., & Wagatsuma, T. (2006). Solubilization of insoluble inorganic phosphate by hyphal exudates of arbuscular mycorrhizal fungi. Journal of Plant Nutrition, 29(4), 657–665.10.1080/01904160600564428
- Thamer, S., Schädler, M., Bonte, D., & Ballhorn, D. J. (2011). Dual benefit from a belowground symbiosis: Nitrogen-fixing rhizobia promote growth and defense against a specialist herbivore in a cyanogenic plant. Plant and Soil, 341(1–2), 209–219.10.1007/s11104-010-0635-4
- Tilman, D., Cassman, K. G., Matson, P. A., Naylor, R., & Polasky, S. (2002). Agricultural sustainability and intensive production practices. Nature, 418(6898), 671–677.10.1038/nature01014
- Tittonell, P., Shepherd, K. D., Vanlauwe, B., & Giller, K. E. (2008). Unravelling the effects of soil and crop management on maize productivity in smallholder agricultural systems of western Kenya – An application of classification and regression tree analysis. Agriculture, Ecosystems & Environment, 123(1–3), 137–150.10.1016/j.agee.2007.05.005
- Trewavas, A. (2001). Urban myths of organic farming. Nature, 410(6827), 409–410.10.1038/35068639
- Tschirley, D. L., & Benfica, R. (2001). Smallholder agriculture, wage labour and rural poverty alleviation in land-abundant areas of Africa: Evidence from Mozambique. The Journal of Modern African Studies, 39(2), 333–358.
- Tubiello, F. N., Salvatore, M., Rossi, S., Ferrara, A., Fitton, N., & Smith, P. (2013). The FAOSTAT database of greenhouse gas emissions from agriculture. Environmental Research Letters, 8(1), 015009.10.1088/1748-9326/8/1/015009
- Ugboh, O., & Ulebor, J. U. (2011). Application of integrated soil fertility approach in the improvement of soil fertility in semi-arid ecology. Journal of Agriculture and Social Research, 11(2), 81–86.
- United Nations, Department of Economic and Social Affairs, Population Division, (2015). World population prospects: The 2015 revision, key findings and advance tables. (Working Paper No. ESA/P/WP.241).
- Vacheron, J., Desbrosses, G., Bouffaud, M.-L., Touraine, B., Moënne-Loccoz, Y., Muller, D., … Prigent-Combaret, C. (2013). Plant growth-promoting rhizobacteria and root system functioning. Frontiers in Plant Science, 4, 356.
- Van Kauwenbergh, S. J., Stewart, M., & Mikkelsen, R. (2013). World reserves of phosphate rock… a dynamic and unfolding story. Better Crops, 97(3), 18–20.
- Vanlauwe, B., Coyne, D., Gockowski, J., Hauser, S., Huising, J., Masso, C., … Van Asten, P. (2014). Sustainable intensification and the African smallholder farmer. Current Opinion in Environmental Sustainability., 8, 15–22.10.1016/j.cosust.2014.06.001
- Vejan, P., Abdullah, R., Khadiran, T., Ismail, S., & Nasrulhaq Boyce, A. (2016). Role of plant growth promoting rhizobacteria in agricultural sustainability – A review. Molecules, 21(5), 573.10.3390/molecules21050573
- Verma, A., Kukreja, K., Pathak, D., Suneja, S., & Narula, N. (2001). In vitro production of plant growth regulators (PGRs) by Azotobacter chroococcum. Indian Journal of Microbiology, 41(4), 305–307.
- Vessey, J. K. (2003). Plant growth promoting rhizobacteria as biofertilisers. Plant and Soil, 255(2), 571–586.10.1023/A:1026037216893
- Villegas, M. D. C., Rome, S., Mauré, L., Domergue, O., Gardan, L., Bailly, X., … Brunel, B. (2006). Nitrogen-fixing sinorhizobia with Medicago laciniata constitute a novel biovar (bv. medicaginis) of S. Systematic and Applied Microbiology, 29(7), 526–538.10.1016/j.syapm.2005.12.008
- Wagner, S. C. (2012). Biological nitrogen fixation. Nature Education Knowledge, 3(10), 15.
- Wallace, M. B., & Knausenberger, W. I. (1997). Inorganic fertiliser use in Africa: Environmental and economic dimensions. Washington, DC: USAID. Retrieved from https://vtechworks.lib.vt.edu/handle/10919/68427
- Weis, T. (2007). The global food economy: The battle for the future of farming: London: Fernwood. Retrieved from http://idsa012013.pbworks.com/w/file/fetch/70717114/Weis_GlobalFoodEconomy.PDF
- Wiggins, S., & Keats, S. (2013). Leaping and Learning: Linking smallholders to markets in Africa. London: Agriculture for Impact, Imperial College and Overseas Development Institute (ODI). Retrieved from http://hdl.handle.net/10568/35246
- Willoughby, R., & Forsythe, L. (2012). Farming for impact-A case study of smallholder agriculture in Rwanda (Technical Report). Concern worldwide. Retrieved from http://gala.gre.ac.uk/12560/
- World Bank. (2013). World Fertiliser Consumption. The World Bank DataBank. Retrieved from http://data.worldbank.org/indicator/AG.CON.FERT.ZS?view=chart
- The World Factbook. (2017). Washington, DC: Central Intelligence Agency. Retrieved from https://www.cia.gov/library/publications/the-world-factbook/index.html
- Wu, Q.-S., & Xia, R.-X. (2006). Arbuscular mycorrhizal fungi influence growth, osmotic adjustment and photosynthesis of citrus under well-watered and water stress conditions. Journal of Plant Physiology, 163(4), 417–425.10.1016/j.jplph.2005.04.024
- Yanggen, D., Kelly, V. A., Reardon, T., & Naseem, A. (1998). Incentives for fertiliser use in sub-Saharan Africa: A review of empirical evidence on fertiliser response and profitability. International Development (Working Paper No. 70). East Lansing: Michigan State University, Department of Agricultural Economics and Department of Economics. Retrieve from http://purl.umn.edu/54677
- Yanni, Y. G., Rizk, R. Y., El-Fattah, F. K. A., Squartini, A., Corich, V., Giacomini, A., … Dazzo, F. B. (2001). The beneficial plant growth-promoting association of Rhizobium leguminosarum bv. trifolii with rice roots. Australian Journal of Plant Physiology, 28(9), 845–870.
- Yasin, M., Munir, I., & Faisal, M. (2016). Can Bacillus spp. Enhance K+ uptake in crop species. In V. S. Meena, B. R. Maurya, J. P. Verma & R. S. Meena (Eds.), Potassium solubilising microorganisms for sustainable agriculture. India: Springer.
- Zabbey, N., Sam, K., & Onyebuchi, A. T. (2017). Remediation of contaminated lands in the Niger Delta, Nigeria: Prospects and challenges. Science of the Total Environment, 586, 952–965.10.1016/j.scitotenv.2017.02.075
- Zalewska, M., & Antkowiak, M. (2013). Gibberellic acid effect on growth and flowering of Ajania pacifica/Nakai/Bremer et Humphries. Journal of Horticultural Research, 21(1), 21–27.