Abstract
The effects of static and varying magnetic fields (MF) on shrinkage and sprouting characteristics of stored potatoes were studied. Static MF was produced by the use of direct current (DC), while alternating current (AC) produced varying MF. Intensities of DC MF were set at 0.5, 1.0, 1.5, and 2.0 mT and those of AC MF set at 0.2, 0.4, 0.6 and 0.8 mT. The exposure time of DC MF on potatoes was held at 10, 20, 40, 60, and 120 s, while the exposure time of AC MF was 10, 20, 40, 80, and 160 s. Potatoes were then stored in a dark room, with light intensity and temperature of 0 lm/m2 and 14 ± 2 °C, respectively, for 8 weeks after which they were examined. Weight reduction was significantly (P < 0.05) lower in potatoes that were subjected to both DC and AC MF than in the untreated tubers. However, it is the DC MF of 2.0 mT that resulted in low acceptable weight loss of less than 10%. Fewer numbers of sprouts per tuber were observed in potatoes that were exposed to DC MF of 0.5, 1.0, and 2.0 mT for 60 s or AC MF of 0.4, 0.6, and 0.8 mT for 80 s, respectively. Our results provide important insights on the applicability of static and varying magnetic fields on reduction of postharvest losses in potatoes.
PUBLIC INTEREST STATEMENT
Ware potato value chain actors often face losses that are associated with sprouting. Lack of effective storage facilities for potato farmers in developing countries forces them to sell at a loss. This is because they cannot hold their produce until times of scarcity when they can attract good prices. Commercial processors do not prefer sprouted potatoes because when they sprout, potato reduce their mass, become shriveled, and this hinders their effective processability. When potato sprouts, they develop toxins known as glycoalkaloids, and this poses a health risk to consumers. Sprouted and shriveled potatoes are also not attractive. A novel environmentally friendly technology was presented in this paper to help reduce the chances of sprouting and shrinkage of potatoes while under storage. Use of magnetic fields (MF) has shown great potential in the reduction of potato postharvest losses. The information from this study will inform all actors in the value chain of potatoes on how best to improve the quality of potatoes between harvesting and consumption through effective storage mechanisms.
1. Introduction
Potato (Solanum tuberosum L) is the fourth most important and widely consumed food crop after wheat, rice, and maize worldwide (Zaheer & Akhtar, Citation2016). It is a staple crop in more than 130 countries globally. The majority of urban and rural families in East Africa consider the crop as a staple food (Kaguongo et al., Citation2008). In Kenya, it is the second most important staple crop after maize (Muthoni et al., Citation2010) and has a greater potential of taking position one since it is fast maturing and least affected by climate change unlike maize (Kaguongo et al., Citation2014). Its production in Kenya has been put at 2,000,000 tonnes, contributes to 31.82 kg/capita/year of food, and supplies 62 kcal, 1.31 g and 0.09 g/capita/day of energy, protein, and fat, respectively (FAOSTAT, Citation2019). Industrially, potatoes are a major source of starch that is used in the manufacture of paper and cardboards, pastes, gums, glues, pharmaceuticals, and detergents, and they can also be used to make vodka, salad, and livestock feed (Bond, Citation2014). In addition, potato farming is a source of employment and income to many farmers in the sub-Saharan Africa (Kaguongo et al., Citation2008; Muthoni et al., Citation2010). It is considered a critically important crop in developing world in assuring food security due to its good nutritional value, fast maturity, and being less affected by increasing world food prices. The less volatility of its price is because it is a globally under-traded commodity that does not trade on major international commodities exchanges (Cromme et al., Citation2010; Furrer et al., Citation2018). Thus, it plays a key role in food and nutritional security worldwide.
During storage, potato suffers many postharvest losses, where up to 30% of fresh produce is lost (Ndaka et al., Citation2012). The main causes of the losses are excessive loss of moisture, decay because of infection by micro-organisms, and sprouting (Lysakov et al., Citation2018; Sonnewald & Sonnewald, Citation2014). The commercial value of sprouted potatoes decreases drastically due to undesirable changes in their morphological characteristics, such as loss of dry matter, decrease in potato turgor, and increased concentrations of sugars as starch are hydrolyzed to support growth of sprouts (Daniels-Lake et al., Citation2005; Kirkman, Citation2007). It has also been reported that toxins α-solanine and α-chaconine develop in potato sprouts in considerable high quantities (Romanucci et al., Citation2016). In addition, consumption of potatoes that have sprouted predisposes humans to toxic food that leads to vomiting, diarrhea, fever, and other diseases such as dyspnea, emesis, and convulsion (Koffi et al., Citation2017; Zhang et al., Citation2018). Sprouting of potatoes while they are still in storage forces farmers in Kenya to sell at low prices. Because sprouting is one of the main obstacles to the sustainable development of the potato processing industry, technologies to inhibit sprouting of potatoes while in storage are paramount. (Lysakov et al., Citation2018; Sonnewald & Sonnewald, Citation2014; Zhang et al., Citation2018).
Several biological, physical, and chemical methods of inhibiting sprouting in potatoes have been in use in different parts of the world, but each one of them has had shortcomings. Biological inhibitors only delay sprouting for a short time and are limited to specific varieties (Zhang et al., Citation2018). Physical inhibitors include irradiation and cold storage, both of which have been effective in delaying sprouting of tubers to some extent. However, potatoes treated to these conditions are often compromised on process quality due to high accumulation of reducing sugars, which in turn causes darkening colour of potatoes, that becomes even more pronounced during frying with the formation of acrylamide (Bradshaw & Ramsay, Citation2009; Daniels-Lake et al., Citation2005; Frazier et al., Citation2006; Mehta et al., Citation2014; Zhang et al., Citation2018). In addition, it is expensive to store potatoes in cold temperatures and thus many smallholder farmers in the sub Saharan Africa and other developing countries do not afford to install such a facility. The most effective inhibitor has been the synthetic chlorpropham (isopropyl N-chloro-phenylcarbamate, CIPC), though it has raised several consumer and environmental issues and as a result, it has been banned in many countries (Daniels-Lake et al., Citation2005; Sonnewald & Sonnewald, Citation2014; Zhang et al., Citation2018). There is thus the need to identify an effective method of inhibiting sprouting in potatoes intended for processing. Use of magnetic flux density presents that potential.
MF have been used to suppress undesirable traits in food crops. A review by Belyavskaya (Citation2004) showed that germination of various plants’ seedlings was suppressed by weak magnetic field (WMF), either by suppressing proliferative activity in meristem of plant roots, cell division, growth processes and cell reproductive cycle or by altering metabolic processes, activity of enzymes, and balance of calcium ions. Maffei (Citation2014) documented that WMF delayed organ development in barley by delaying formation of shoots and roots in barley seedlings. Studies on the effects of MF on seed potato reported increased yield (Cramariuc et al., Citation2005; Pittman, Citation1972) and effective control of late blight (Statsyuk et al., Citation2015). Stepura and Talanov (Citation2015), however, have shown that the behaviour and characteristics of potato tuber as well as sprouts, in response to MF, are dependent mainly on intensity and pre-treatment time with magnetic fields. Lysakov et al. (Citation2018) studied the effects of DC- and AC-induced MF on storage loss of ware potatoes, where tubers were exposed to 1.27 and 0.3 mT for the DC- and AC-activated MF, respectively, with exposure time being varied for 10 to 60 s. They reported varied mass loss of between 18 and 72% depending on the MF intensity and exposure time. However, they did not account for the loss in mass, which could be caused by evaporation, respiration, or sprouting. Hence, there is need to study the potential of MF in inhibiting sprouting of potatoes during storage to reduce their postharvest losses, which, to the best of our knowledge, has not been studied.
2. Materials and methods
2.1. Materials
Clean and healthy potatoes (Shangi variety—chosen due to its high preference by Kenyan potato farmers) were collected from a certified potato farmer in Nakuru county, Kenya. Due diligence was done to ensure that good agricultural practices were performed during the growth and harvesting of the potatoes. The potatoes were sorted according to size and tubers of 50 mm in diameter or more were selected. These were cured for 5 days at room temperature (14 ± 2 °C). The tubers were then subjected to varying strengths of magnetic fields and thereafter stored and monitored for shrinkage and sprouting.
2.2. Generation and application of magnetic fields
Magnetic fields were generated by the use of double Helmholtz coils (06960–00, PHYWE Systems GmbH & Co, Gottingen, Germany; 154 turns, 20 cm in radius) that were placed at a distance equal to their radii (). The coils were connected to a variable power supply (13,530–93, PHYWE Systems GmbH & Co, Gottingen, Germany. Input: 230 V, 50–60 Hz; DC output: 0–12 V, 5 A; AC output: 0–15 V, 5 A) that supplied either direct or alternating current. Varying magnetic intensities of 0.5, 1.0, 1.5, and 2.0 mT and 0.2, 0.4, 0.6, and 0.8 mT were obtained by varying both the direct and alternating currents, respectively, as shown in . The power supply could not generate more than 1.0 mT through the alternating current, and thus the reason why magnetic intensities were low in AC than in DC. A multimeter (2010 Dmm, PeakTech, Ahrensburg, Germany) was used to measure amount of current and voltage, while a tesla meter (13,610.93, PHYWE Systems GmbH & Co, Gottingen, Germany), fitted with a Hall effect sensor, was used to measure the amount of magnetic flux that was established.
Figure 1. Schematic representation of treatment of potato tubers with magnetic fields generated by double Helmholtz coils.
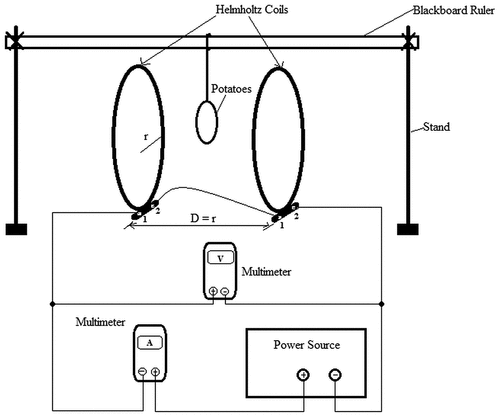
Table 1. Magnetic field (MF) intensities generated through direct currents (DC) and alternating currents (AC)
Six tubers were weighed using an electronic weighing scale (TXB6201L, Shimadzu, Rosario, Cavite, Philippines) and put in a mesh net fabric with holes of 12.5 mm in diameter. The weight of the tubers varied between 330.5 and 493.2 g. The net with tubers was placed at the centre of the Helmholtz coils by hanging it using a thread that was tied to a wooden blackboard ruler which was held tight by two stands that stood on far ends of the centre of coils. Tubers were then subjected to varying magnetic intensities for 10, 20, 40, 60, and 120 s for direct current and 10, 20, 40, 80, and 160 s for alternating current, respectively. Holding times for AC-induced MF were slightly increased than those of DC to compensate for the low intensities in AC MF. Treated potatoes were then stored in a dark room with a light intensity of 0 lm/m2 and a temperature of 14 ± 2 °C for 8 weeks, after which they were sampled for shrinkage and sprouting characteristics.
2.3. Evaluation of sprouting and shrinkage properties of tubers
Sprouting characteristics and shrinkage were determined as outlined by Gachango et al. (Citation2008). The number of sprouted tubers from each lot were counted and expressed as a percentage of the total number of tubers in the sample. A tuber was considered as having sprouted if it had at least one visible sprout of at least 3 mm in length. For evaluation of the number of sprouts per tuber (NST), all sprouted tubers were picked from each lot, and sprouts in each tuber were counted then averaged per number of sprouted tubers. The length of the longest sprout per potato and thickness of the sprout base were measured using a digital vernier caliper (Mars Edpal Instruments Pvt. Ltd, Ambala, India). All tubers from each sample were de-sprouted and weight of the sprouts was recorded. Sprouting capacity was then calculated as a percentage of the fraction of weight of sprouts to the weight of tubers in each sample. For shrinkage evaluation, the final weight of the tubers was expressed as a percentage of the initial weight at the beginning of storage.
2.4. Statistical analyses
Data were analysed for variance using the Generalized Linear Model (PROC GLM) procedure of the Statistical Analysis System (SAS 9.1; SAS Institute, Inc., Cary, North Carolina). Differences between the treatment means were determined using Tukey’s HSD (honestly significant difference) test at P < 0.05) significance level.
3. Results
3.1. Effects of DC- and AC-activated magnetic intensities on shrinkage and sprouting characteristics
The effects of MF intensity on weight reduction and sprouting behaviour of potatoes are given in . The highest weight reduction was recorded in the control sample (0 mT) for both the DC- and AC-activated MF. This reduction was significantly (P < 0.05) high than all other potatoes that were treated with MF. The least weight reduction was observed with the DC-activated MF for the 2.0 mT. Generally, MF activated by AC resulted in higher weight reduction than fields that were generated through DC. The control sample had all its potatoes sprouting after the 2 months. There also seemed to be a reduction in the number of sprouted potatoes as AC MF were increased linearly. On the other hand, high DC magnetic intensities (1.5 and 2.0 mT) resulted in increased number of potatoes that had sprouted. Numbers of sprouts per tuber were recorded in significant (P < 0.05) high numbers in the control tubers. There was no significant (P < 0.05) difference in the number of sprouts per tuber among various magnetic intensities (0.5, 1.0, 1.5 and 2.0 mT) for the DC generated fields. However, for the AC generated fields, intensity of 0.6 mT resulted in low numbers of sprouts per tuber that significantly (P < 0.05) differed with intensities of 0.2 and 0.8 mT. The length of the longest sprout and thickness of the sprout base were least observed when DC and AC MF intensities were set at 0.5 and 0.8 mT, respectively. The optimum intensities that gave maximum length of the longest sprout and sprout base thickness were 1.5 and 0.4 mT for the DC- and AC-activated fields. Increasing magnetic intensities reduced the sprouting capacity of tubers where 2.0 and 0.8 mT of DC and AC magnetic intensities recorded significant (P < 0.05) low levels of sprouting capacity than all other intensities, respectively.
Table 2. Effects of magnetic field (MF) intensities on shrinkage and sprouting characteristics of potatoes
3.2. Effects of varied holding times of magnetic intensities on shrinkage and sprouting characteristics
Influence of different holding times of MF on sprouting behaviour of potatoes is given in . Increasing holding time from 10 to 120 s reduced shrinkage in tubers that were treated with DC-activated MF. For both DC and AC magnetic fields, 10 s of holding time resulted into higher weight reduction that was significant (P < 0.05) from other holding times. The optimum amount of holding time for sprouting appeared to be 40 s, where number of sprouted potatoes was highest for both currents. This time was significant (P < 0.05) for the DC but not for AC-induced fields. The number of sprouts per tuber were least observed at 60 and 80 s of holding time for the DC- and AC- activated fields, respectively, and was maximum with the 10 s for both cases. An interesting trend was observed for the length of the longest sprout, where for both currents, elongation was higher for each consecutive increase in amount of time until an optimum holding time was achieved; 40 and 80 s for the DC- and AC-induced fields, respectively. Just like sprouting, the sprout base thickness had an optimum holding time of 40 s in both cases, after which the thickness reduced with subsequent addition of holding time. Capacity of potatoes to sprout was at maximum with minimum amount of holding time (10s), while 60 and 160s resulted into least capacity of sprouting in the DC- and AC-activated MF, respectively.
Table 3. Shrinkage and sprouting characteristics as influenced by varied holding times of magnetic intensity on potatoes
3.3. Effects of interaction between magnetic field intensities and holding time on shrinkage and sprouting characteristics of potatoes
Shrinkage and sprouting characteristics of potatoes as influenced by DC-activated MF intensities under various holding times are given in . At 10 and 20 s of holding time, tubers that were exposed to 2 mT recorded significant (P < 0.05) lower weight reduction than those that were subjected to 0.5, 1.0, and 1.5 mT. Apart from the 40 s of holding time, subjecting tubers to 1.5 mT for 10, 20, 60, and 120 s resulted into significant (P < 0.05) high weight reduction. The least occurrence of sprouting was observed when tubers were treated with 0.5 mT for 60 s, while 40 s resulted into high numbers of sprouted potatoes for all the intensities. Subjecting potatoes to 0.5, 1.0, and 2.0 mT for 60 s caused minimal numbers of sprouts per tuber, while intensity of 1.5 mT for 60s resulted into longest sprout whose length was significant (P < 0.05). Tubers with minimal sprout base thickness were obtained when potatoes were exposed to 0.5 mT for 10, 20, and 60 s and also, when intensity of 1.0 mT was applied for 10s. An interesting scenario was observed for sprouting capacity where increasing magnetic intensity from 0.5 to 2.0 mT for 40 and 120 s resulted into subsequent reduction of sprouting capacity.
Figure 2. Effects of interaction between DC-activated magnetic field intensities and holding time on shrinkage and sprouting characteristics of potatoes. Error bars represent standard error of individual means.
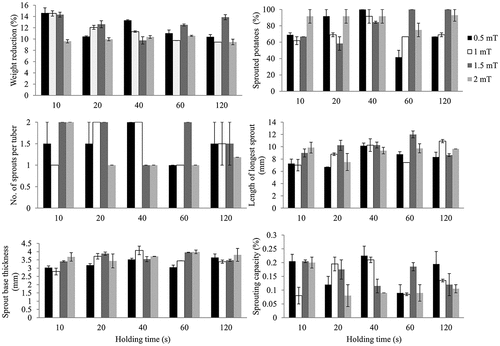
The effects of AC-activated MF intensities under various holding times on shrinkage and sprouting characteristics are shown in . All magnetic intensities (0.2, 0.4, 0.6, and 0.8 mT) resulted into significant (P < 0.05) higher weight reduction when held for 10s than under 20, 40, 80, and 160 s. An increase in magnetic field intensity from 0.4 to 0.8 mT led to a corresponding decrease in the number of sprouted potatoes when the intensities were held for 20 and 40 s, but holding them for 80 and 160 s resulted into an increase in the number of sprouted tubers for each subsequent increase of magnetic intensity. Holding magnetic intensities for 80 s majorly reduced the number of sprouts per tuber where potatoes that were exposed to 0.4, 0.6, and 0.8 mT gave minimum number of sprouts. The length of the longest tuber was highest recorded when potatoes were exposed to an intensity of 0.4 mT for 80 s but was least observed with 0.8 mT under 40 and 160 s of holding time. Significant (P < 0.05) lower base thickness of sprout was recorded with exposure of tubers to 0.8 mT for 160 s. It was also noted that an increase in magnetic field intensity at 160 s of holding time resulted into subsequent decrease in the sprout base thickness. An increase in intensity from 0.2 to 0.6 mT had a linear increase in sprouting capacity of tubers when the magnetic fields were held for 10 and 40 s. On the other hand, there was a linear decrease in the capacity of tubers to sprout when magnetic intensities were increased from 0.4 to 0.8 mT and used to treat potatoes for 20 and 80s.
3.4. Correlation of response variables
Correlation matrix plots for response variables as influenced by DC-activated MF are shown in . The strength and significance of relationships were ascertained by performing a Pearson’s correlation analysis at a confidence level of 95%. The moderate positive correlation between weight reduction and number of sprouts per tuber, as well as weight reduction versus sprouting capacity indicated that the more sprouts there were per tuber and the higher the capacity of tubers to sprouts, the more weight was lost from potatoes, r = 0.48, p = 0.012 and r = 0.45, p = 0.003, respectively. A strong positive correlation was observed between sprouting capacity and the number of sprouts per tuber, r = 0.80, p < 0.001. A moderate positive correlation between length of the longest sprout and sprout base thickness (r = 0.57, p < 0.001) as well as the length of the longest sprout and sprouting capacity (r = 0.40, p = 0.010) showed that the thicker the base of sprout and the higher the sprouting capacity of tubers, the longer the sprout. There was also a moderate positive relationship between length of the tuber and number of sprouted potatoes, r = 0.33, p = 0.036.
Figure 4. Correlation matrix plots for the response variables as affected by DC-activated magnetic fields. WR; weight reduction, SP; sprouted potatoes, NST; number of sprouts per tuber, LLS; length of the longest sprout, SBT; sprout base thickness, SC; sprouting capacity, LT; length of tuber.
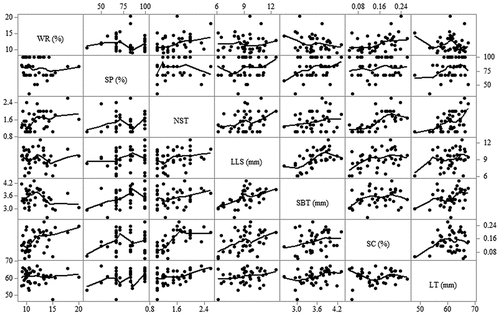
Matrix plots for correlation among response variables as affected by AC-induced MF is as shown in . Unlike in DC MF, the negative correlation between weight reduction and sprout base thickness was significant, r = —0.32, p = 0.037. Interestingly, the moderate correlation between number of sprouts per tuber and length of the longest sprout was negative, r = —0.44, p = 0.013, while the same was positive and non-significant in DC MF. Just like in DC-induced fields, there was a strong positive correlation between the length of the longest sprout and sprout base thickness (r = 0.61, p < 0.001) and a moderate positive correlation between the length of the longest sprout and sprouting capacity (r = 0.44, p = 0.003). Sprouting capacity and sprout base thickness showed a moderate correlation, r = 0.40, p = 0.010.
4. Discussion
Magnetic fields either initiated or suppressed sprouting based on the intensity and amount of holding time applied. Sprouting of potatoes start to occur when stored potatoes break their dormancy. The dormancy period is determined by the cultivar of the potato, maturity of the tuber before harvesting, weather and soil conditions, and the type of treatment that is applied before storage (Pinhero et al., Citation2009; Sonnewald & Sonnewald, Citation2014). In this study, the same variety of potato, which was grown under the same climatic conditions and harvested at the same stage of maturity, was used. In addition, the treated potatoes were stored under the same storage conditions, and this ensured that sprouting was only influenced by the MF. It was evident that potatoes treated with MF recorded low weight reduction than the control (untreated potatoes), at the end of the storage period. We argue that MF slowed down the rates of respiration and transpiration in the tubers under storage, and thus, reducing the weight that was lost. During respiration, potatoes break down glucose and produce carbon dioxide, water, and heat. The liberated heat favours moisture loss from tubers by reducing the relative humidity of the surrounding air thereby increasing its moisture holding capacity (Singh et al., Citation2020). Therefore, MF could have lowered the physiological process of tubers by hindering synthesis of starch to glucose thereby discouraging pronounced respiration.
Weight loss can also can be caused by excessive sprouting of tubers. This explains why the more sprouts there were per tuber, the more the weight reduction was recorded. A tuber with multiple sprouts causes more shrinkage than one with few or one sprout (Struik, Citation2007). Typically, shangi variety has a short dormancy period of less than 1 month (NPCK, Citation2017), indicating that the potato start to sprout within a few days of storage. This causes many losses especially if the potato was intended for processing due to reduction in commercial value (Singh et al., Citation2020). Both the DC and AC MF recorded lower number of sprouted potatoes and low number of sprouts per potato than the control and this shows that MF is promising in prolonging the dormancy of shangi and in effect improving its marketability. This is a breakthrough given that sprouting and evaporation account for 90% of the total weight loss of stored potatoes (Gachango et al., Citation2008). Our findings that DC-induced MF resulted in lower weight reduction than the AC magnetic fields agrees with the findings of Lysakov et al. (Citation2018); however, our study reported lower weight losses than Lysakov et al. (Citation2018), which could probably be attributed to differences in potato variety and storage conditions. A DC-induced MF of 2.0 mT resulted into high numbers of sprouted potatoes, although the number of sprouts per sprouted potato was low and weight reduction was low. This could be due to the dominance of the apical bud that suppresses growth of lateral buds.
Phytohormones play a vital role in the sprouting of potatoes. Of primary concern is abscisic acid (ABA) which is vital in initiation as well as the sustenance of dormancy (Sonnewald & Sonnewald, Citation2014). Subjecting potatoes to DC MF for 60 s and AC MF for 80 s may have activated the synthesis of increased ABA which favoured prolonged dormancy resulting into fewer number of sprouted potatoes as well as number of sprouts per potato. Treating tubers to DC MF for 40 and 120 s may have induced production of cytokinins that aided in breaking of dormancy resulting into higher numbers of sprouted potatoes while on the other hand inhibiting synthesis of gibberellins, which promotes growth of many sprouts, and thus the low number of sprouts per tuber (Bishop et al., Citation2012; Mani & Hannachi, Citation2015). It is plausible that all MF intensities suppressed auxin hormone that is known to promote vascular development for support of growing sprouts (Mani & Hannachi, Citation2015), thus the low number of sprouted potatoes as well as number of sprouts per tuber. It is also likely that application of MF encouraged production of phenolic compounds by the potato periderm and other growth inhibitors such as volatiles, contributing to continued dormancy (Suttle, Citation2004).
Physiological ageing of potato is monitored through sprouting capacity where sprouting capacity increases as the ageing progresses (Jeong et al., Citation2008). It can thus be said that exposing potatoes to DC and AC magnetic intensities of 2.0 and 0.8 mT, respectively, for all exposure periods apart from the 10 s favoured ageing of tubers thereby giving higher sprouting capacity at the end of storage period. When potato age physiologically, sprouts develop, respiration and evaporation increases and consequently, shrinkage increases. This is evident by the scenario that the treatment combinations of magnetic intensities and holding time that resulted into higher sprouting capacity also contributed to high weight loss. Length of the longest sprout is a good indicator of the general sprout development within a tuber (Wurr, Citation1978). It can therefore be argued that subjecting potatoes to DC and AC MF of 1.5 and 0.4 mT for 60 and 80 s, respectively, favours tuber metabolism resulting into well-developed sprouts. Sprout base thickness is a parameter that informs on the vigour of sprouts, and is particularly important when tubers are to be used as seed material, where high values are desired (Gachango et al., Citation2008). In this study, storage was done for future processing of potatoes and therefore subjecting potatoes to 1.0 and 0.8 mT for 10 and 160 s would be recommended for low sprout base thickness when MF are induced by DC and AC, respectively.
Correlation analyses have confirmed that sprouting of tubers has a direct negative impact on the quality of potatoes by reducing their weight. When sprouts develop, they promote moisture loss from the tuber because their epidermis is 100 times more water permeable than the periderm of the tuber (Paul & Ezekiel, Citation2006), thus the reduction in weight of the tuber. Our finding that the number of sprouts per tuber had a positive significant correlation with weight loss has also been reported by Nyankanga et al. (Citation2018) and Pande et al. (Citation2007). Weight loss of above 10% is considered unacceptable because such tubers are predominantly shrivelled and this lead to their reduced marketability (Ezekiel et al., Citation2004). Findings from this study show that DC-induced MF of 2.0 mT has the potential of reducing weight loss to below 10%. In addition, AC-induced MF of 0.6 mT has the same potential although exposure of tubers to this intensity must be held for 40 s. A moderate negative correlation between the number of sprouts per tuber and the length of longest sprout has also been reported by other studies (Singh and Ezekiel, Citation2003; Pande et al., Citation2007). The positive correlation between sprout base thickness and length of the longest sprout could be due to remobilization of sugars and proteins within the tuber, localizing them within the base of the sprout that eventually supported growth of the sprout.
5. Conclusion
It is desired that ware potatoes do not sprout while under storage, rest their marketability reduce. This study was conducted to assess the applicability of magnetic fields in reducing sprouting and weight reduction of potatoes. Acceptable weight reduction of less than 10% was observed in tubers that were exposed to DC MF of 2.0 mT and AC MF of 0.6 mT with holding time of 40s. Tubers that were treated with DC- or AC-induced MF recorded a low number of sprouts per tuber than the untreated tubers, and thus magnetic fields have the potential of reducing post-harvest losses in potatoes. Further research should be done to investigate how MF affect the expression of phytohormones that are involved in sprouting of potatoes including abscisic acid, cytokinins, gibberellins, and auxin.
Abbreviations
DC: Direct current; AC: Alternating current; MF: Magnetic fields; NPCK: National Potato Council of Kenya
Authors’ contributions
Conceptualization: FGI, CMM, FGN, and SMM; Data collection: FGI and SGM; Data Analysis and manuscript preparation: FGI; All authors contributed to the improvement of the manuscript, read and approved the final manuscript.
Data availability
All the necessary data that support this study are included in the manuscript. Further information maybe obtained from the corresponding author upon request
Acknowledgements
This paper is part of Ph.D. research work for the corresponding author.
Disclosure statement
No potential conflict of interest was reported by the author(s).
Additional information
Funding
Notes on contributors
Francis Gichuho Irungu
Francis Gichuho Irungu is an Assistant Lecturer of Food Science and Technology at the Department of Food Technology, Chuka University, Kenya. This work is part of his Ph.D. research work on the use of Magnetic Fields to reduce Postharvest Losses in Potatoes. He is a bona fide Ph.D student at the Department of Dairy, Food Science and Technology, Egerton University, Kenya. At the time of preparation of this article, he was a Research Fellow at the International Institute of Tropical Agriculture (IITA), Dar es Salaam, Tanzania. His research interest includes use of Novel Technologies to reduce Postharvest Losses, Food Biosystems Engineering and Use of Insects for Food and Feed.
Francis G. Ndiritu
Francis G. Ndiritu is an Associate Professor of Condensed Matter Physics at the Department of Physics, Egerton University, Kenya. His research interests are based on Electromagnetic Fields and their applications, Biophysics and Material Science.
Christopher M. Mutungi
Christopher M. Mutungi is a Ph.D Scholar at International Institute of Tropical Agriculture (IITA), Dar es Salaam, Tanzania. His areas of interest are based on Agricultural Research and Development, Food Processing and Engineering, Entomophagy and Food Security.
Simon G. Mathenge
Simon G. Mathenge is a Technologist and Research Fellow at the Department of Physics, Egerton University, Kenya. He has a background in Electrical Technology. His research interests are in Biophysics, Renewable Energy and Material Science.
Symon M. Mahungu
Symon M. Mahungu is a Professor of Food Chemistry at the Department of Dairy, Food Science and Technology, Egerton University, Kenya. He is also the University Industry Liaison Officer. His latest research interest has been on Utilization of Gum Arabica in Food Processing.
References
- Belyavskaya, N. A. (2004). Biological effects due to weak magnetic field on plants. Advances in Space Research, 34(7), 1566–13. https://doi.org/10.1016/j.asr.2004.01.021
- Bishop, C., Rees, D., Cheema, M. U. A., Harper, G., & Stroud, G. (2012). Potatoes. In D. Rees, G. Farrell, & J. Orchard (Eds.), Crop post-harvest; science and technology first edition (pp. 334–359). Blackwell Publishing.
- Bond, J. K. (2014). Potato utilization and markets. In R. Navarre & M. J. Pavek (Eds.), The potato: Botany, production and uses (pp. 29–44). CABI.
- Bradshaw, J. E., & Ramsay, G. (2009). Potato origin and production. In J. Singh & L. Kaur (Eds.), Advances in potato chemistry and technology (pp. 1–26). Academic Press.
- Cramariuc, R., Donescu, V., Popa, M., & Cramariuc, B. (2005). The biological effect of the electrical field treatment on the potato seed: Agronomic evaluation. Journal of Electrostatics, 63(6–10), 837–846. https://doi.org/10.1016/j.elstat.2005.03.082
- Cromme, N., Prakash, A. B., Lutaladio, N., & Ezeta, F. (2010). Strengthening potato value chains: Technical and policy options for developing countries. Food and Agriculture Organization of the United Nations (FAO).
- Daniels-Lake, B. J., Prange, R. K., Nowak, J., Asiedu, S. K., & Walsh, J. R. (2005). Sprout development and processing quality changes in potato tubers stored under ethylene: 1. Effects of ethylene concentration. American Journal of Potato Research, 82(5), 389–397. https://doi.org/10.1007/BF02871969
- Ezekiel, R., Singh, B., Sharma, M. L., Garg, I. D., & Khurana, S. P. (2004). Relationship between weight loss and periderm thickness in potatoes stored at different temperatures. Potato Journal, 31, 135–140.
- FAOSTAT. (2019). New food balances. Rome: Food and Agriculture Organization of the United Nations. Available from: http://www.fao.org/faostat/en/#data/FBS (28 January 2021)
- Frazier, M. J., Kleinkopf, G. E., Brey, R. R., & Olsen, N. L. (2006). Potato sprout inhibition and tuber quality after treatment with high-energy ionizing radiation. American Journal of Potato Research, 83(1), 31–39. https://doi.org/10.1007/BF02869607
- Furrer, A. N., Chegeni, M., & Ferruzzi, M. G. (2018). Impact of potato processing on nutrients, phytochemicals, and human health. Critical Reviews in Food Science and Nutrition, 58(1), 146–168. https://doi.org/10.1080/10408398.2016.1139542
- Gachango, E., Shibairo, S. I., Kabira, J. N., Chemining’wa, G. N., & Demo, P. (2008). Effects of light intensity on quality of potato seed tubers. African Journal of Agricultural Research, 3(10), 732–739.
- Jeong, J. C., Ok, H. C., Hur, O. S., & Kim, C. G. (2008). Prediction of sprouting capacity using near-infrared spectroscopy in potato tubers. American Journal of Potato Research, 85(5), 309–314. https://doi.org/10.1007/s12230-008-9010-x
- Kaguongo, W., Gildemacher, P., Demo, P., Wagoire, W., Kinyae, P., Andrade, J., Forbes, G., Fuglie, K., & Thiele, G. (2008). Farmer practices and adoption of improved potato varieties in Kenya and Uganda. International Potato Centre (CIP).
- Kaguongo, W., Maingi, G., & Giencke, S. (2014). Post-harvest losses in potato value chains in Kenya: Analysis and recommendations for reduction strategies. Deutsche Gesellschaft für Internationale Zusammenarbeit (GIZ) GmbH.
- Kirkman, M. A. (2007). Global markets for processed potato products. In D. Vreugdenhil, J. Bradshaw, C. Gebhardt, F. Govers, M. A. Taylor, D. K. MacKerron, & H. A. Ross (Eds.), Potato biology and biotechnology (pp. 27–44). Elsevier.
- Koffi, G. Y., Remaud-Simeon, M., Due, A. E., & Combes, D. (2017). Isolation and chemoenzymatic treatment of glycoalkaloids from green, sprouting and rotting Solanum tuberosum potatoes for solanidine recovery. Food Chemistry, 220, 257–265. https://doi.org/10.1016/j.foodchem.2016.10.014
- Lysakov, A., Nikitenko, G., Konoplev, E., & Tarasov, Y. (2018). Advanced methods of potato loss reduction in storage. Engineering for Rural Development, 17, 560–565. https://doi.org/10.22616/ERDev2018.17.N194
- Maffei, M. E. (2014). Magnetic field effects on plant growth, development, and evolution. Frontiers in Plant Science, 5 (445) , 1–15. https://doi.org/10.3389/fpls.2014.00445
- Mani, F., & Hannachi, C. (2015). Physiology of potato sprouting. Journal of New Sciences, 17, 1–12.
- Mehta, A., Singh, B., Ezekiel, R., & Minhas, J. S. (2014). Processing quality comparisons in potatoes stored under refrigerated and non-refrigerated conditions. Indian Journal of Plant Physiology, 19(2), 149–155. https://doi.org/10.1007/s40502-014-0089-4
- Muthoni, J., Mbiyu, M. W., & Nyamongo, D. O. (2010). A review of potato seed systems and germplasm conservation in Kenya. Journal of Agricultural & Food Information, 11(2), 157–167. https://doi.org/10.1080/10496501003680565
- Ndaka, D., Macharia, I., Mutungi, C., & Affognon, H. (2012). Postharvest losses in Africa–analytical review and synthesis: The case of Kenya. International Centre for Insect Physiology and Ecology.
- NPCK. (2017). Potato variety catalogue. National Potato Council of Kenya.
- Nyankanga, R. O., Murigi, W. W., Shibairo, S. I., Olanya, O. M., & Larkin, R. P. (2018). Effects of foliar and tuber sprout suppressants on storage of ware potatoes under tropical conditions. American Journal of Potato Research, 95(5), 539–548. https://doi.org/10.1007/s12230-018-9662-0
- Pande, P. C., Singh, S. V., Pandey, S. K., & Singh, B. (2007). Dormancy, sprouting behaviour and weight loss in Indian potato (Solanum tuberosum) varieties. Indian Journal of Agricultural Sciences, 77, 715–720.
- Paul, V., & Ezekiel, R. (2006). Sprout suppression of potato tubers stored at 18° C by pre-and post-harvest application of sub-lethal doses of glyphosate. Indian Journal of Plant Physiology, 11(3), 300–305.
- Pinhero, R. G., Coffin, R., & Yada, R. Y. (2009). Post-harvest storage of potatoes. In J. Singh & L. Kaur (Eds.), Advances in potato chemistry and technology (pp. 339–370). Academic Press.
- Pittman, U. J. (1972). Biomagnetic responses in potatoes. Canadian Journal of Plant Science, 52(5), 727–733. https://doi.org/10.4141/cjps72-119
- Romanucci, V., Pisanti, A., Di Fabio, G., Davinelli, S., Scapagnini, G., Guaragna, A., & Zarrelli, A. (2016). Toxin levels in different variety of potatoes: Alarming contents of α-chaconine. Phytochemistry Letters, 16, 103–107. https://doi.org/10.1016/j.phytol.2016.03.013
- Singh, B., & Ezekiel, R. (2003). Studies on dormancy, sprout growth and weight loss in some old and new potato varieties under controlled temperature conditions. Seed Research, 31(1), 47–52.
- Singh, B., Raigond, P., Jaiswal, A. K., & Kumar, D. (2020). Potato post-harvest management strategies. In A. K. Singh, S. K. Chakrabarti, B. Singh, J. Sharma, & D. V.k (Eds.), Potato science and technology for sub-Tropics (pp. 343–359). New India Publishing.
- Sonnewald, S., & Sonnewald, U. (2014). Regulation of potato tuber sprouting. Planta, 239(1), 27–38. https://doi.org/10.1007/s00425-013-1968-z
- Statsyuk, N. V., Kuznetsova, M. A., Rogozhin, A. N., & Filippov, A. V. (2015). Combination of a pre-planting treatment of tubers with low-frequency pulse electric field and foliar treatments with Agat-25K microbial preparation to control the late blight of potato. PPO-Special Report, 17, 145–152.
- Stepura, A. V., & Talanov, P. I. (2015). Study of the effect of electromagnetic radiation of extremely high frequency wavelength on potato growth rate. 2015 International Conference of Antenna Theory & Tech, 1–3. https://doi.org/10.1109/ICATT.2015.7136897.
- Struik, P. C. (2007). Above-ground and below-ground plant development. In D. Vreugdenhil, J. Bradshaw, C. Gebhardt, F. Govers, M. A. Taylor, D. K. MacKerron, & H. A. Ross (Eds.), Potato biology and biotechnology (pp. 219–236). Elsevier.
- Suttle, J. C. (2004). Physiological regulation of potato tuber dormancy. American Journal of Potato Research, 81(4), 253–262. https://doi.org/10.1007/BF02871767
- Wurr, D. C. E. (1978). Studies of the measurement and interpretation of potato sprout growth. The Journal of Agricultural Science, 90(2), 335–340. https://doi.org/10.1017/S002185960005543X
- Zaheer, K., & Akhtar, M. H. (2016). Potato production, usage, and nutrition—a review. Critical Reviews in Food Science and Nutrition, 56(5), 711–721. https://doi.org/10.1080/10408398.2012.724479
- Zhang, L., Li, M., Zhang, G., Wu, L., Cai, D., & Wu, Z. (2018). Inhibiting sprouting and decreasing α-solanine amount of stored potatoes using hydrophobic nanosilica. ACS Sustainable Chemistry & Engineering, 6(8), 10517–10525. https://doi.org/10.1021/acssuschemeng.8b01860