Abstract
A growing number of farmers’ cooperatives cultivate crops without chemical pesticides, but also without organic certification. How does this intermediate form of agriculture between conventional and organic production function? What are the outcomes of this production form on cropland biodiversity? How does this model contribute to the transition toward more sustainable forms of agriculture? We address these research questions using the original data collected in the AgroBioDiv project from the farmers’ cooperative “KraichgauKorn” based in the German Federal State of Baden-Württemberg. This cooperative is a collection of conventional farmers, who refrain from pesticide use during the growing seasons for KraichgauKorn cereal grains. Our study finds higher levels of weed species biodiversity on KGK cereal fields compared to conventional fields, but lower levels of biodiversity than on organic fields. In addition, more endangered wild species monitored by the State of Baden-Württemberg were found on KGK fields than conventional fields, with organic fields exhibiting the highest presence of endangered flora. We conclude that such kinds of enterprises may indeed contribute substantially to a successful transformation towards sustainable agricultural systems.
1. Introduction and background
Pesticides are used by farmers to protect crops from pests and comprise various chemicals or biological substances. Depending on the agent targeted, pesticides may be referred to as herbicides, insecticides, fungicides, or bactericides (see, e.g., Cullen et al., Citation2019). While pesticide sales are not directly representative of actual pesticide use, these figures provide a rough idea of their significance in agricultural production. Eurostat reports 333,418 tons of pesticides were sold in 2019 in the European Union (EU). Among EU member states, Germany, Spain, France, and Italy comprised over two-thirds of these pesticide sales. However, Eurostat data show pesticide sales decreased between 2011 and 2019, with the lowest sales in 2019 since the start of data collection (Eurostat, Citation2021). Although sales continue, a trend toward pesticide reduction is noticeable given decreasing sales, which, inter alia, reflects a response to societal demands and public policies. Concerning the latter, we can expect that the implementation of the EU’s Farm to Fork Strategy will provide a strong incentive for farmers to change their farming practices, including the use of pesticides.
As the German Environment Agency states, addressing pesticide use is important for nature protection due to both potential and real negative environmental impacts. Pesticides can contaminate nearby biotopes, groundwater, and local waterways through unintentional further transport known as pesticide drift (see, e.g., Rosic et al., Citation2020). Furthermore, the use of broad-spectrum herbicides and insecticides results in fauna impoverishment and habitat loss for birds and other animals living on farmlands. The German Environment Agency identifies the loss of insect pollinators as an adverse impact of pesticides on biodiversity given the reduced occurrence of flowers on and near farmlands with pesticide application (UBA, Citation2021). However, the pollination of crops requiring insect-mediated pollen transfer is also heavily affected, resulting in severe decreases in crop yield (Millard et al., Citation2021).
In view of the various environmental impacts of pesticides, the growing public demand for the formulation and implementation of strategies for reducing or phasing-out pesticide use entirely in many advanced democracies is not surprising. However, the total amount of pesticides used in agriculture worldwide is still increasing (Sharma et al., Citation2019), while biodiversity is decreasing (Marja et al., Citation2022). One of the countries in which pesticides have become politicized is Germany, which is also the largest economy in the EU. There have been several citizens’ initiatives both at the EU-level and subnational-level in Germany calling on policy-makers to phase-out or reduce pesticide use in agriculture (Tosun & Koch, Citation2022; Tosun & Varone, Citation2020). While these bottom-up processes are important and worth studying in detail, in this study, we adopt a different focus. We are interested in an initiative that has existed for three decades and is characterized by a farming approach between conventional farming (permits pesticide use) and organic farming (abstains from synthetic pesticides).
KraichgauKorn (KGK) comprises a group of 45 local farmers based in the German Federal State of Baden-Württemberg who in certain years produce crops conventionally, and in other years produce wheat and other cereals without applying pesticides. In other words, they rotate between organic and conventional farming practices. Their grain products are not certified as organic but are marketed under the KGK cooperative label, which stands for regional production without pesticide application during the KGK cereal grain production season. The cooperative includes farmers, millers, and bakers working exclusively with KGK cereal grains to produce breads and baked goods. Farmers are motivated to participate in the cooperative as a way to reduce their impact on the environment and support wild flora biodiversity by abstaining from pesticide applications during KGK-production years. Finding farmers to participate in the model is not challenging, but the model is limited in its growth by the availability of bakers able to process KGK cereal grains. Thus, supporting the establishment of the full value chain is necessary for expanding the KGK production model and similar farmers’ cooperatives.
How does KGK function as an intermediary production model? What are the outcomes of this production form for cropland biodiversity? How does this model contribute to the transition toward more sustainable forms of agriculture? These three research questions guide our analysis, which brings together expertise in plant biology and public policy and contributes to the discussion on transitionary forms of production for enhancing biodiversity on agricultural fields (Böcker et al., Citation2019; Finger & El Benni, Citation2013; Geiger et al., Citation2010; e.g. Marja et al., Citation2022; Möhring & Finger, Citation2022; Perović et al., Citation2021; Tscharntke et al., Citation2021). Our research addresses a gap in the scientific literature on the biodiversity impacts of pesticide-free production models by documenting and comparing the agrobiodiversity of KGK cereal fields with that of organic and conventional cereal fields. We compile and share an original dataset collected in the framework of the AgroBioDiv project to demonstrate the significance of such alternative production systems for wild flora biodiversity. Interestingly, we find that the KGK farmers’ cooperative provides a production model for initiating and facilitating the transition toward more sustainable agriculture production (Pretty, Citation2008; Zollet & Maharjan, Citation2021).
The remainder of this study unfolds as follows. First, to set the stage for this analysis, we provide background information on the salience of pesticide use in Germany. Then, we describe the KGK cooperative’s farming approach in detail. Subsequently, we present and discuss our expectations on the consequences of intermediate farming practices for agricultural biodiversity.
1.1. The growing salience of pesticide use in agriculture
Pesticides began to rise in salience (i.e., the public’s attention directed at a given issue) in 2015, when the World Health Organization’s International Agency for Research on Cancer classified glyphosate as a probable human carcinogen. Glyphosate is an active substance in numerous herbicides and the majority of pesticides used in Germany. Despite this assessment by the World Health Organization, the European Commission continued to issue an application for renewing the authorization of glyphosate in the EU (Tosun & Varone, Citation2020; Tosun et al., Citation2019). The EU Commission supported glyphosate’s license renewal in the EU since the risk assessment conducted by the European Food Safety Authority (EFSA) at the time did not classify glyphosate as carcinogenic and thus did not allow the substance to be banned (Tosun & Varone, Citation2020).
The renewal requests induced citizens from several EU member states to launch a European Citizens’ Initiative (ECI) in which organizers asked the Commission to ban glyphosate, reform the pesticide approval procedure, and set EU-wide mandatory reduction targets for pesticide use. It should be noted that ECIs do not have direct legislative effect but should rather be seen as agenda-setting tools (Tosun et al., Citation2022). If an ECI reaches the minimum number of signatures, the Commission decides whether to act. If it abstains from taking action, the Commission has to provide reasons for its decision in a communication (Kandyla & Gherghina, Citation2018). Despite organizers’ success in gathering the necessary number of signatures in 2017, and a resolution adopted by the European Parliament in favor of banning glyphosate, the authorization of glyphosate was renewed for a period of 5 years. This decision resulted in bans announced or adopted in several EU member states that disagreed with the Commission’s decision, such as Luxembourg (Tosun & Varone, Citation2020).
Two years later, a similar group of organizers registered the ECI “Save Bees and Farmers” with the Commission. The group of promoters is headed by the Germany-based Environment Institute Munich, and this initiative is in general strongly supported by German organizations. “Save Bees and Farmers” is closely related to the previous ECI on glyphosate, but targets pesticides more broadly. More precisely, this ECI asks the Commission, inter alia, to phase out 80% of synthetic pesticides in EU agriculture by 2030, starting with the most hazardous and to become free of synthetic pesticides by 2035. This ECI has also reached the minimum number of signatures to be considered by the EU Commission. At the time of writing, the ECI had stopped collecting signatures, and was in the process of being checked and evaluated by the Commission. Thus, at the EU-level, pesticides have been the subject of two ECIs: “Stop Glyphosate” and “Save Bees and Farmers”.
While the EU Commission has not directly taken up the demands of “Stop Glyphosate,” it has committed itself to a 50% reduction in pesticide use by 2030 as part of its Farm to Fork Strategy (Beckman et al., Citation2021). This commitment appears well-advised given EU citizens’ concerns about the adverse effects of pesticides on human health and the environment have increased over the past decade. Conversely, satisfaction with the EU and national regulatory approaches has declined (Zeitlin et al., Citation2022). This suggests that, in the near future, alternatives to pesticide use (see, e.g., Aulagnier & Goulet, Citation2017; Vialatte et al., Citation2021) will receive considerable attention.
Turning to Germany, the protection of bees by reducing and phasing out pesticide use was subject to five citizens’ initiatives at the federal state-level. The first initiative was based in Bavaria in 2018. This was followed by initiatives based in Baden-Württemberg and Brandenburg (both launched in 2019) as well as Lower Saxony and North Rhine-Westphalia (both launched in 2020) (Tosun & Koch, Citation2022). Of these, the initiatives based in Baden-Württemberg and Lower Saxony triggered changes in federal state biodiversity laws. The initiative in Baden-Württemberg was successful even without the necessary number of signatures. In fact, the federal state government asked initiative promoters not to even collect signatures. Instead, the state launched a policy dialogue, which led to the revision of the nature protection and agriculture law in July 2020 and incorporated numerous elements of the citizen initiative’s policy proposal. Similarly, the government of Lower Saxony asked initiative promoters to stop collecting signatures, and developed a joint legislative proposal through dialogue. The “Lower Saxonian Way,” which aims to improve nature protection while compensating farmers for their additional efforts in protecting biodiversity, was adopted by the parliament of Lower Saxony in November 2020. The Brandenburg-based initiative succeeded in reaching the necessary number of signatures, but the state parliament rejected it based on formal grounds. The initiative in North Rhine-Westphalia has also succeeded in collecting the necessary number of signatures to be formally considered by the state parliament (Tosun & Koch, Citation2022).
In 2021, the ECI on banning glyphosate and the state-level citizen initiatives in Germany induced the Federal Minister of Agriculture of the Christian Democratic Party to revise her previously supportive position on glyphosate and to accept a phase-out plan proposed by the Federal Minister for Environment of the Social Democratic Party. However, a phase-out of glyphosate is not the only potential policy action for greening agriculture. To facilitate a transition toward more sustainable agriculture, a mix of policy instruments is required, which not only targets the supply side (i.e., farmers and their farming practices) but also the demand side, for example, by shortening food supply chains (Le Velly et al., Citation2021). The change in the composition of the federal German government and the fact that the Federal Minister for Environment is now headed by the Green Party suggests that pesticides and strategies for reducing their use in agriculture will receive even more political attention in the coming years. The policy changes that took place across various levels of Germany’s political system are a consequence of public demand for reducing or phasing out pesticides (Tosun et al., Citation2022). A survey with ~1,000 individuals carried out by the polling company forsa in November 2020 revealed that 77% of respondents would support a ban on pesticides in agriculture, with support for a pesticide ban higher among women and residents of larger cities (forsa, Citation2020). However, policy change has occurred only recently, and therefore it will take some time until results can be seen in cropland biodiversity. The KGK farmers’ cooperative adopted agricultural practices for reducing pesticide use already in 1990. Consequently, we consider this cooperative to be a useful case for studying how the policy measures recently adopted can positively impact cropland biodiversity.
1.2. The KraichgauKorn approach
Thirty years ago, the Agricultural Minister Gerhard Weiser of the Christian Democratic Union (CDU) declared the entire State of Baden-Württemberg to be a protected area due to persistent nitrate pollution—an issue with which not only this state but all of Germany has been struggling (Schaub, Citation2019, Citation2021). At that time, the Evangelical church of North Baden had a working group, which recognized the adverse effect of agriculture on the environment and that farming practices not supporting the environment would eventually pose a threat for food production. The KGK cooperative emerged from the idea that by closely following existing nature protection laws in farm production, crops would be especially high-quality. From the very beginning, the cooperative brought together not only farmers but also a miller, a baker, and a priest—the latter was important for demonstrating that the aims of the cooperative were rooted in local rural culture.
According to interviews carried out with KGK directors (see 5.1), it was unimaginable at that time for conventional farmers to completely switch to organic agriculture. The cooperative developed its own production standards with unplanted buffer zones on field perimeters as reserved habitat for wild flora and insects, a ban on synthetic plant protection materials during KGK-crop seasons, and permanent, routine field samplings to verify the absence of synthetic chemicals in harvested cereal grains. KGK farmers began implementing their model by slowly switching from sugar beet to cereal grain production, which at the time was the easiest way to transition their production from purely conventional practices.
Today, the KGK cooperative is comprised of 45 farmers in northern Baden-Württemberg. In addition, 2 millers and 40 bakers participate in the cooperative by processing only KGK or organic cereal grains into bread and baked goods. As a cooperative, farmers, millers, and bakers are business partners and colleagues in designing and implementing their production model. Seeds are bought collectively, with farmers following shared production rules. KGK is not an organic farming cooperative, but they ban pesticide use during cereal grain production under their market label. Tilling is practiced to control pests and weeds in the fields during KGK cereal grain seasons, and crop rotation is practiced during the off-season to control plant diseases and pests. A crop rotation refers to a planned temporal sequence of crops on a field over several years. A diverse and balanced crop rotation design is an important factor for the sustainable production of food. However, in recent decades, there has been a progressive tendency towards tighter crop rotations with a significant decrease in crop diversity and dominance of a few species, such as wheat, barley, and maize. The reasons for this are of an economic and production-technical nature. Nevertheless, crop rotations offer the possibility to break up peak workloads, to optimally utilize production capacities throughout the year, to spread the cultivation risk, and to flexibly react to changing conditions compared to focusing on just a few crops. In addition, optimized and adapted crop rotations can have a variety of other positive individual and cumulative effects, such as positive effects from previous crops, regulation of harmful organisms and weeds, as well as improvement of soil fertility through the increase in humus, nutrient fixation, and mobilization. The most common crop rotation cycle implemented by KGK farmers is a 4–5-year cycle, starting with a winter cereal grains, such as winter wheat, followed, e.g. by corn in the second year. In the third year, cereal grains are grown again, followed by non-cereal crops, such as legumes, rape seed, and finally wheat or other cereals. However, there are numerous variations in the sequence of cultivated crops depending on various factors as mentioned above. In summary, within a 3- to 7-year cycle, 2–3 cereal grain crops are cultivated. While KGK farmers do not use pesticides during cereal grain production, they are allowed to use pesticides when cultivating non-KGK crops, such as maize, strawberries, sunflowers, rapeseed, potatoes, and sugar beet. The pesticides used for these crops during non-KGK seasons include the widely distributed products: Dual gold, Axial, Biathlon, Ariane C, Axial 50, Switch, Karate Zeon, Revus, Karate, Biskaya, Bandur, Adengo, and Elumis.
In addition to the pesticide ban during KGK cereal grain production, farmers may not use growth regulators or genetically modified organisms, and they sow flower patches along field perimeters to support pollinator and plant biodiversity. During KGK cereal grain production, random soil and grain samples are taken directly from farmers’ fields to ensure zero contamination and perfect control as per cooperative standards. When cereal grains are inspected and confirmed to meet these standards, they are transported to the millers for processing and storage before reaching the cooperative’s bakeries. If products are found to be inconsistent with KGK standards, the grains must be sold to a conventional miller, inflicting profit losses on the entire cooperative. Hence, it is in all members’ best interest to comply fully with the cooperative’s regulations if they choose to participate in this production model.
Regional production and consumption are further cornerstones of the KGK model, with many cooperative members arguing that organic is often not necessarily better. Retailers supplying organic products often import their products from abroad, raising questions about the stability of the national food supply, as well as climate implications when food production is moved abroad to meet organic standards. Regional supply chains have also become increasingly salient both in Germany and the EU, with the ECI “Eat ORIGINal! Unmask your food” calling for mandatory declaration of origin on all food products. At the establishment of the KGK cooperative, it was unimaginable that consumers would be willing to pay 50–60% more for sustainable products, as some organic consumers do today. Given its approach to land management and a 10–20% higher price margin with 5–10% lower crop yield than conventional cereal grain, KGK exhibits a middle path for agriculture development, which can assist Baden-Württemberg in reaching its target for 30–40% organic agriculture by 2030 (see Tosun & Koch, Citation2022). The extra costs of the cooperative’s flour, breads and baked goods are covered by end-consumer prices. Despite the price mark-up, cooperative members do not necessarily earn more by participating in the KGK cooperative. According to KGK directors, it is simply an alternative production model, and farmers participate because they want to contribute to environmental protection efforts.
Based on the crop rotation practices of KGK farmers, we can conservatively calculate a 33–60% reduction in total pesticide use. Framework conditions, such as crop rotation and flower patches on field perimeters, are very similar between organic farming and the KGK model. In our study, we focus exclusively on cereal fields, mainly winter wheat, to achieve maximum comparability between organic and KGK farmers. Plant biodiversity in crop fields (wild arable flora) is evolutionarily adapted to and heavily dependent on respective cultivated crops and cultivation methods (e.g. Koch et al., Citation2016), making it an appropriate indicator to measure the impact and effects of pesticides, and in particular herbicides, on arable plant diversity (Pierny, Citation1994; Brühl and Zaller, Citation2019).
On average, one or two farmers are able to join the cooperative each year. However, this is dependent on the capacity of KGK bakers to process the cereal grains harvested, and the cooperative is thus currently limited in its growth potential by access to bakeries willing to work exclusively with KGK cereal grains or mixed KGK and organic grains. Hence, the cooperative has an informal waiting list for interested farmers once additional bakers are found, or other farmers leave the cooperative due to changing production practices or a change in profession/retirement. Oftentimes, farmers do not want to switch directly from conventional to organic production but have a strong interest to protect the environment and support the diversity of wild flora in their fields. This is the main motivation for farmers to participate in the KGK cooperative. Transitioning from conventional to KGK production practices does require some field and business adjustments, but new farmers are supported by the cooperative directors and their colleagues to make appropriate changes. So far, switching from conventional practices to the KGK model has not been an issue for new members given their self-motivation for joining and the cooperative’s support system.
Over the cooperative’s lifetime, five members have transitioned entirely to certified organic production, with two farmers switching to organic production in the last 5 years. Therefore, KGK can be understood as an intermediate model with permanent rotation of production techniques, or even a gradual shift from conventional to organic farming, if provided with an adequate, supportive political framework. Hence, there are many lessons we can draw from this particular case for sustainability transitions in agriculture. KGK serves as a bottom-up model for the pragmatic reduction of pesticides in agriculture and a sustainable business model that emphasizes the establishment of local value chains. In the case of KGK, it is particularly the local value chain that matters, and which we contend to play a decisive role in the success of similar initiatives that can help reduce pesticide use and make agriculture more sustainable.
1.3. Expectations
In this section, we put forth three expectations regarding different types of potential impacts of the KGK approach on wild arable flora and sustainable agriculture transitions. Given the state of current research, our analytical goal is not to formulate and test generalizable hypotheses, but to understand the implications of the KGK approach. Therefore, we present expectations rather than hypotheses as guiding our analysis to reflect that we investigate a specific farming approach.
1.3.1. Expectation 1: the KGK approach has a positive effect on overall arable field wild flora
We test this expectation by studying KGK production measures, and comparing KGK field biodiversity with reference fields from organic farming (no pesticide application) and conventional farming. If this expectation holds true, we should observe a significantly higher number of arable wild species, often not endangered and (in terms of both occurrence and abundance), on KGK cereal fields compared to conventionally farmed fields, since the soil seed bank of wild arable flora re-builds over time and serves as a long-term reservoir for plant population survival. However, pesticide use during non-KGK crop seasons on the same fields prevents the establishment of wild arable flora to a larger extent, and thus endangered and rare species may not benefit substantially. Therefore, we expect to observe less arable field plant diversity on KGK fields than organic fields, and KGK field species diversity should fall between those observed in conventional and organic fields.
1.3.2. Expectation 2: the KGK approach increases endangered wild flora diversity over time, thereby preventing critical biodiversity loss
We test this expectation by studying the occurrence and abundance of Baden-Württemberg state-wide endangered plant species in KGK cereal fields and compare this to organic and conventional fields. If this expectation is true, rare and endangered wild arable flora may have a chance to colonize, and slowly build a new soil seed bank over time.
1.3.3. Expectation 3: the KGK approach contributes to transitions toward more sustainable agriculture production
KGK was established over 30 years ago. The cooperative’s total field area and number of participating farmers should follow a constant positive trend in transition towards organic farming. If this expectation holds true, we should observe even higher average performance rates as documented for organic farming during the past three decades, indicating the overall attractiveness of the KGK approach to an increasing number of farmers. Furthermore, KGK farmers may have switched to organic farming and left the cooperative, indicating the KGK approach also contributes toward a sustainable transition of agricultural systems over time.
2. Material and methods
2.1. Interdisciplinary approach
This study brings together expertise from biology and the social sciences, which is reflected in the data and methods used. The social scientists were in the lead for developing a questionnaire to conduct a structured interview with KGK directors to learn about the establishment of the cooperative, its business practices, and its activities supporting the diversity of wild flora in cereal fields. Semi-structured interviews were carried out with KGK farmers by the team’s biologists and university students supporting field mapping to understand individual farmers’ cultivation practices and baseline field characteristics, which may influence the biodiversity of wild flora on their cereal fields. A total of eight interviews were carried out between February and August 2021 with six KGK farmers. Data on cereal field plant diversity, and thus the performance of the KGK approach were produced by the project team’s biologists.
2.2. Study area and natural setup
KGK defines itself as a regional farmers’ cooperative. Thus, the geographic context is defined as the broader Kraichgau region in the German Federal State of Baden-Württemberg (Figure ). The individual cereal fields studied are distributed throughout Zabergäu, Leintal, Enzkreis, Kraichgau, Kurpfalz, Hardt, and Taubertal. Kraichgau is a sloped region in northwestern Baden-Württemberg and is bordered by the Odenwald mountains and the Neckar River in the North (city of Heidelberg), the Black Forest in the South (city of Pforzheim), and the Upper Rhine Valley in the West (city of Karlsruhe). The eastern boundary is formed by the edge of a slightly more mountainous region between the cities of Pforzheim and Heilbronn. In total, the Kraichgau region comprises approximately 1,630 km2. The most defining feature of agricultural lands in Kraichgau is its fertile Loess deposits. These create the gently rolling landscape of the valleys between the Odenwald and the Black Forest, giving this area its nickname as the “Tuscany of Germany” and making the region important for agriculture. Fertile soils especially support the cultivation of cereal grains, which is the focus of KGK production. Therefore, we investigated KraichgauKorn cereal fields producing wheat, rye, barley, and old cereal species (e.g. emmer wheat, spelt, and einkorn wheat), which are not treated with pesticides during the growing season. About 24 of the total 45 farmers in the KGK cooperative participated in our study. Each farmer was asked to provide up to five fields for wild arable flora mapping. Due to the limited geographical area of the Kraichgau region, and the small total number of KGK members, the potential sample of cereal fields was limited. Nonetheless, our sample size for the KGK cereal fields was relatively large (n = 139), and participating KGK farms covered the entire Kraichgau region.
Figure 1. Map showing the location of the study area in Europe, Germany in white and the Federal State of Baden-Württemberg in southwest Germany in dark blue.
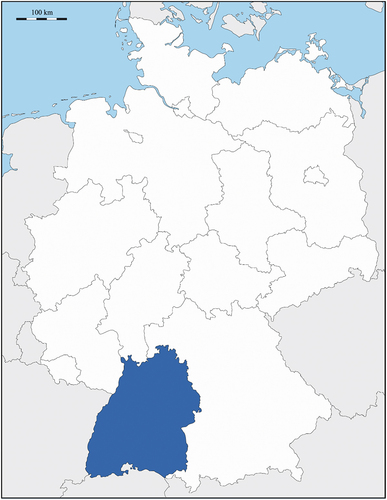
The organic study plots for our comparison group were on 31 farms distributed over the entire Federal State of Baden-Württemberg (n = 84) and comprised a representative set of natural features in terms of regional climate, soil type, and landscape, so that almost all relevant bioclimatic regions and natural areas in Baden-Württemberg could be included (Meynen et al., Citation1953-Citation1962; Ssymank, Citation1994; Pierny, Citation1994). All organic farms were certified, and thus did not apply pesticides or easily soluble mineral fertilizer on any of their fields. On these farms, a total of 84 fields for winter wheat, barley, and rye were studied for their wild arable flora. The locations of the evaluated field plots are shown in Figure .
Figure 2. Experimental setup and location of KraichgauKorn farmland and organic farm site areas studied in southwest Germany. Agrobiodiversity (selected 80 wild arable species from state-wide biodiversity monitoring) and potential vulnerability of wild plant species from arable fields on landscape scale (resolution of 25 km2) is shown as background information (grey-scale). Boxplots indicate that landscape-level diversity measures are not different, hence, allowing direct comparisons of monitoring results on local fields (see Figure ).
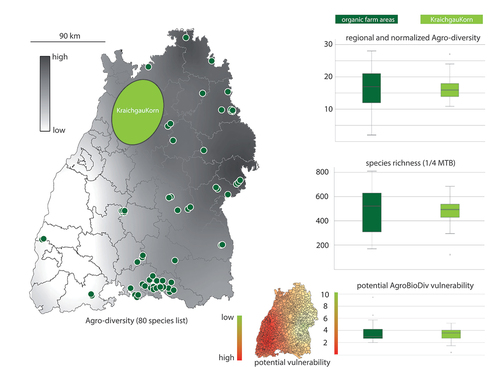
Crop fields of KGK farmers (n = 139) and organic farmers (n = 84) were evaluated and compared for their arable wild flora diversity as a proxy for reduced pesticide use. In addition, 17 conventionally farmed fields (non-KGK production) were analyzed for their arable wild flora diversity. As far as possible, winter wheat fields were investigated to keep the results across farming systems as comparable as possible.
2.3. Exploring state-wide plant biodiversity
Since composition and richness of arable wild flora depends on various factors, such as bioclimatic conditions, soil type, and landscape effects in general (e.g., Tscharntke et al., Citation2012, Tscharntke et al., Citation2021), we analyzed the floristic database of the Natural History Museum in Stuttgart to explore the overall vascular plant biodiversity of Baden-Württemberg while accounting for these factors and testing potential sampling biases in our study design. We also determined the baseline diversity of arable wild flora in Baden-Württemberg to monitor the potential effect of regional agriculture on our study design. Furthermore, this baseline diversity of arable fields was used to compare the mapped species diversity with respective regional total vascular plant biodiversity in Baden-Württemberg to establish a general indicator for the actual vulnerability of wild arable flora on a regional scale. Although there is a Red List of wild vascular plants characteristic for arable fields (Württemberg, Citation2020), there is no systematic monitoring of flora biodiversity in arable fields, and the most recent floristic survey including a critical evaluation was nearly 30 years old at the time of our study (Pierny, Citation1994). With this approach, we aimed to present a proxy to allow for a direct comparison between our studied agriculture systems (the KGK model, organic farming, and conventional farming). Of course, there are numerous factors on individual study plots, which impact arable wild flora diversity (e.g. soil type, mechanical weed control practices, cultivar selection, etc.). However, the analysis of these factors and their impacts on wild flora diversity goes beyond the aims and scope of this study. The continuously updated floristic database of the State of Baden-Württemberg comprises over 2.5 million records from the past century and is freely accessible at http://www.flora.naturkundemuseum-bw.de/start2.htm. Floristic data can be inspected and exported directly at http://www.florabw.recorder-d.de.
The geographic accuracy of documented occurrence data varies. Thus, we aggregated the accuracy (resolution) on a scale of ¼ of the ordnance survey map (MTB 1:25,000, 10 × 10 km), which is equivalent to an area of roughly 25 km2. We restricted the final data set after database screening to occurrence data from the past 20 years to obtain a realistic and contemporary overview of floristic richness, generating 475,814 data points. In the final dataset, we replaced all ¼ MTB with total species numbers smaller than a cutoff value of 100 (considering the total dataset of ¼ MTBs) by the arithmetic mean of its 8 direct neighbors to minimize the impact of erroneous reports or potential lack of mapping data. For all ¼ MTB, geographic coordinates of the centroids were calculated and exported together with species richness data into a MariaDB database (open source: https://mariadb.org/).
A list of 80 representative wild plant species for arable fields was compiled from this data by (i) selecting characteristic flora species of arable fields (Pierny, Citation1994), (ii) excluding very locally distributed species, and (iii) excluding taxa that were not yet taxonomically well defined (Suppl. Material Table S1; DRYAD https://doi.org/10.5061/dryad.s1rn8pkcc). For species selection, we also considered our own results from the field surveys to guarantee a sufficiently large overlap in species occurrence data. From our reference list of 80 species, 42 species (52.5%) occur in our vegetation recordings. The majority of those (75%) occur with a frequency larger than 25% in our vegetation recordings, thereby covering a representative fraction of arable plant species diversity in the Federal State of Baden-Württemberg.
The final three state-level datasets (A: total vascular plant diversity; B: arable field species richness; C: B/A) were imported into QGIS (QGIS Development Team 2021, open source: http://qgis.osgeo.org). We applied the IDW (inverse distance weighting) algorithm (Bartier & Keller, Citation1996) as implemented in QGIS, and used species richness scores and coordinates of the original ¼ MTB to calculate respective raster layers. A high-resolution polygon shapefile indicating local political boundaries is used as the background layer (Natural Earth 2021, open source: https://www.naturalearthdata.com/features/). A color code was adjusted to highlight respective differences in species richness. The respective datasets A and B are provided in Supplementary Material Table S2 (DRYAD https://doi.org/10.5061/dryad.s1rn8pkcc; a detailed description of those tables is given with the respective README.txt file).
A map-based visualization of dataset C measures the putative vulnerability of wild arable flora by showing the fraction of documented wild arable flora to the total potential vascular plant biodiversity in Baden-Württemberg. Lower percentages represent a higher vulnerability and thus an increased risk of species’ decline.
We further investigated at the landscape-level (¼ MTB, 25 km2 resolution) whether arable field plant diversity (80 species list) and the total number of vascular plants differs between regions, with KGK fields and organic fields studied in detail. At best, there is no difference, which allows direct comparison with our collected field data (see following chapter). For this test, we compared the total potential number of vascular plant species following the floristic database of Baden-Württemberg with our total number of recorded arable flora species for all ¼ MTBs of KGK farm sites (45 ¼ MTBs) and organic farm sites (84 ¼ MTBs).
2.4. Monitoring wild plant diversity of arable fields
The selected 31 organic farms in Baden-Württemberg provided access to organic cereal fields during the 2020/2021 growing period. In total, 84 fields were assessed, covering a total area of 299.66 ha. One sectional (3 × 30 m) was defined within each sampled field representing a typical section of the field (Hofmeister and Garve, Citation1998). Sectionals were placed parallel to field borders and located 2–3 m from the nearest field margin to avoid floristic biases caused by marginal vegetation and environmental influence (nitrogen, permanent vegetation, and disturbance) and to maximize consistency among field vegetation recordings. Vegetation monitoring was conducted from April to July 2021. Plant species were identified on-site in the field and documented via photographs and herbarium specimens, which have been deposited at the Heidelberg Botanical Garden and Herbarium (HEID) for documentation. Individually recorded plots were visited 2–3 times, and the Braun-Blanquet vegetation cover-abundance scale was applied (Braun-Blanquet, Citation2012). This method requires only one-third to one-fifth of the field time necessary for determining species density, and allows also for documentation and reliable identification of arable plant species communities (Hofmeister and Garve, Citation1998; Nezadal, Citation2014). Furthermore, cover-abundance ratings are better suited than density values for studying species–environment relationships. In extensive surveys, the method provides sufficiently accurate baseline data to conduct environmental impact assessments as required, e.g. by regulatory agencies. Furthermore, arable fields were inspected for the occurrence of additional plant species that were not present within the 3 × 30 m monitoring sectional, and these species were recorded as present when found (symbol x). These species were also considered for species richness comparisons.
In 2021, five KGK farm sites were monitored representing 30 cereal fields and covering a total area of 99.84 ha. Additional vegetation monitoring data from KGK fields were obtained from earlier monitoring programs conducted in 2016–2019 (Schraml, Citation2018, Citation2019) (2016–2018: n = 82; 2019: n = 27) and contributed additional 109 fields with approximately 250 ha. The same species and vegetation monitoring methods were applied in these studies as in our study and have been described above. Additional 17 conventional fields (non-KGK production) were monitored to conduct a negative control representing floral biodiversity in the context of pesticide application. The entire dataset, including geographic coordinates, is provided in Suppl. Material Table S3 (DRYAD https://doi.org/10.5061/dryad.s1rn8pkcc).
2.5. Biodiversity data analyses
Biodiversity monitoring data were analyzed separately for KGK and organic farmers. As a measure of species richness (S) the mean number of species per study plot was calculated. Floristic alpha diversity of crop fields was estimated applying the widely used Shannon index (H) (Shannon, Citation1948; Spellerberg & Fedor, Citation2003), which considers not only species richness but also their respective abundances. The Shannon index reaches its maximum when all species are occupied with equal frequency, which means different species in the monitoring plot and the community are nearly equally abundant. The Shannon index is one of the most used measures for determining plant species diversity. The Braun-Blanquet abundance classes were transformed into the following frequencies for subsequent calculation of the Shannon index: r = 0.01, + = 0.02, 1 = 0.04, 2 = 0.15, 3 = 0.375, 4 = 0.625, 5 = 0.875 (Güngör, Citation2011).
We also calculated the species evenness index (D), which considers both the number of species and the equilibrium among them. The value of D falls within the interval [0 … 1]. If there is only one species, D is zero. As the number of species increases, and their contribution to the overall abundance is equalized, D approaches the Shannon index. For D, the Shannon index H is divided by ln(S), the natural logarithm of species richness.
To study the positive long-term effects of agricultural practices, the occurrence of endangered plant species on cereal fields was evaluated. We compared the total number of observed endangered plant species (KGK, organic farming) and local contribution (average number per field) of endangered plant species (Red List Data Book of Endangered Plants in Baden-Württemberg) to the observed cereal field plant diversity. In general, there should be an increase in the fraction of state-wide endangered species over time in the absence of pesticide use, thereby indicating an increase in ecological habitat quality and allowing a permanent and rich soil seed bank to build up over a period of several decades. Descriptive statistical analyses (Boxplots and Student´s t-test) were performed using SPSS version 27 before evaluating the normality of data distribution (IBM Corp., Armonk, NY, USA).
We extracted values for total vascular plant species richness, richness of arable flora, and “vulnerability of arable flora” from regions with monitoring sites on the resolution scale shown in Figure (¼ MTB) to elaborate on the relative comparability of KGK biodiversity data with data collected across organic farms in Baden-Württemberg. The respective biodiversity numbers from KGK cereal fields were compared to organic and conventional farming, with testing for significant regional floristic differences, which could explain differences in richness of arable flora not attributed to pesticide reductions (such as soil type, climate, and landscape).
3. Findings
The distribution of organic farms (dark green circles) within the KGK region (light green area) is indicated in Figure . The Baden-Württemberg state-wide occurrence of 80 species in arable fields, as reported by the floristic inventory gathered during the last 20 years, shows a decrease in plant species diversity from east to west. This decrease is well explained by geomorphological features, elevation, climate, vegetation types, and geology (Wörz & Thiv, Citation2015). However, the overall comparison of landscape-level species diversity (¼ MTB, resolution of 25 km2) shows that local sites (KGK versus organic farms) do not differ significantly regarding agrobiodiversity based on our list of 80 species nor in total vascular plant biodiversity (Figure ). The potential vulnerability of wild arable flora is expressed as the percentage of wild arable flora among total plant biodiversity in a regional landscape and does not differ between the regions of organic and KGK farms in our study (Figure , boxplots). Therefore, our study design is not affected by landscape-level biases, and may offer firm general conclusions on the local effects of pesticide reduction.
3.1. Finding 1: the KGK approach has a positive effect on overall arable field weed biodiversity
The evaluation of plant biodiversity in cereal fields shows significantly (p-value 2.6e-09) higher species diversity (wild floral species number per arable field) in KGK fields (n = 139, 350 ha) compared to conventionally farmed fields (n = 17, 51 ha). However, the fields under organic management (n = 84, 300 ha) show significantly (p-value 2.7e-09) higher species diversity than both KGK and conventional fields. Similar results are obtained when species diversity and abundance are considered using the Shannon-Wiener index, thereby providing an ecologically more meaningful parameter. Evenness, a measure of the equal frequency distribution of individuals of a sample among species, is not significantly different among all three treatments (organic, KGK, conventional), which also shows that there is no potential bias of unevenly distributed plant diversity among our compared cereal fields (Figure ). The empirical data demonstrate that expectation 1 (The KGK approach has a positive effect on overall arable wild flora biodiversity) is true since we observed significantly higher wild species numbers and diversity (occurrence and abundance) on KGK fields compared to conventional fields. This indicates a positive effect of pesticide reduction to increase the capacity of the soil seed bank and allow seeds to accumulate over time in cereal fields.
Figure 3. Field monitoring results comparing arable field plant biodiversity from organically managed sites, KGK farmland and conventional agriculture. State-wide vascular plant diversity and agrobiodiversity (80 species list) is provided as maps on ¼ MTB resolution (grey scale). Study sites are indicated with dark green circles (organic farming) and pale green area (KGK).
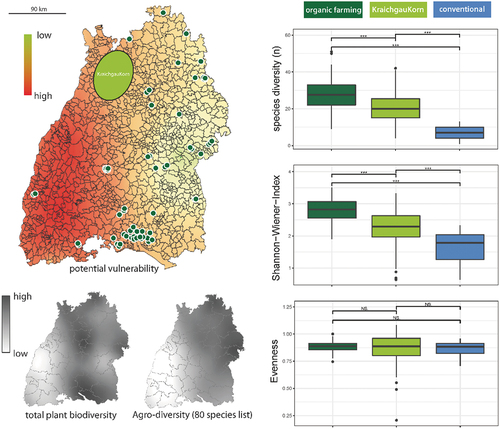
3.2. Finding 2: KGK production contributes to the preservation of critical floral biodiversity based on the presence of endangered species in KGK fields compared to conventional fields
Comparing the occurrence of Baden-Württemberg state-wide endangered plant species on crop fields, KGK cereal fields showed in total 13 endangered species out of 193 species monitored (6.7%), and organic fields showed in total 16 endangered species out of 193 species monitored (7.4%). Conventionally farmed fields did not exhibit any endangered plant species (0 endangered species out of 193 species monitored, 0%).
3.3. Finding 3: despite higher species diversity and presence of endangered species in both KGK and organic fields, neither production form is currently adequate to prevent biodiversity loss
Despite the higher occurrence of endangered species in both KGK and organic fields compared to conventional fields, the mean number of endangered species per field was below one for both KGK and organic fields, and zero at conventional fields. Unfortunately, this result demonstrates severe biodiversity loss at all sites, with even our studied organic field sites not serving as adequate biodiversity reservoirs of vulnerable species within a depleted landscape. Hence, KGK fields are contributing just as well as our studied organic fields to plant biodiversity reservoirs. The relevance of expectation 2 (The KGK approach increases endangered wild flora diversity over time, thereby preventing critical biodiversity loss) is less clear, because we do not observe sufficient endangered species on any of our sampled fields to make a firm conclusion. We have to tentatively conclude that neither KGK nor organic farming, in our study, serves as a reservoir for endangered crop field plant biodiversity. However, KGK fields showed similar biodiversity values compared to organic fields, indicating a similar positive effect, at least on less specialized wild arable flora. Therefore, we tentatively conclude that the KGK concept may contribute to the prevention of ongoing local and regional biodiversity loss but is not sufficient for preserving plant species, which are already highly threatened.
3.4. Finding 4: the KGK cooperative supports the transition toward more sustainable agriculture production
The KGK approach enables sustainable agricultural transition given a continuous increase in KGK cultivated fields contributing to the local product chain and regional market since the cooperative’s establishment. The total field size increased from 60 ha in 1990 to 1,600 ha in 2017, equal to an average annual expansion of 13% in cultivation area. The number of contributing farmers also increased steadily from 7 farmers in 1990 to 51 farmers at the end of 2021, resulting in a yearly average increase of 7%. The respective increase in organic farming over the last 25 years (1994–2019) and average increase in total field size was 7% per year, which is only half the increase of the KGK cooperative.
These numbers indicate that KGK is strongly contributing, with over-proportionally increasing area size compared to organic farming, to reducing pesticide use and has thus established a successful long-term model. Interestingly, over the last three decades, five KGK farmers switched to organic agriculture and left the KGK cooperative. This equals approximately 8.5% of KGK members within the entire period and is a lower value compared to the increase in organic farms in Germany from 1995 to 2020 from 2% to 10.5% with on average 7% per year over this same 25-year time-frame (out of the total number of agricultural enterprises) (Federal Ministry of Food and Agriculture—BMEL, Citation2021).
In this context, our third expectation (The KGK approach contributes to transitions toward more sustainable agriculture production) appears to hold true, since some KGK farmers have transitioned entirely to organic production after participating in the cooperative. However, it could also be hypothesized that the increase in farmers participating in the KGK cooperative (7% per year) is directly competing with a direct transition from conventional to organic farming.
Under the given economic circumstances, and due to the success of the KGK approach, this concept seems to hinder an even higher transition to organic farming. This is an interesting, and also unexpected finding, which entails the need for reflecting on what is more desirable from a societal and political perspective. If priority is given to starting the transition process, the KGK approach appears ideal and should be supported. However, if a permanent phase-out of pesticides is preferred, the KGK approach appears less desirable, at least on the basis of the data analyzed in this study. Regardless of the outcome of this reflection process, policy-makers should be aware of such models, their effects on wild arable flora, and the economic incentive structures they offer to farmers.
4. Discussion
For a long time, agriculture policy was shielded from public pressure and focused on securing farmer income and food supply. However, this has changed in the last few years, and agriculture policy has had to open up to new actors and ideas (Daugbjerg & Feindt, Citation2017), resulting in a “greening” of this policy sector (Alons, Citation2017; Persson & Runhaar, Citation2018; Persson et al., Citation2018). While agriculture policy has been slow in changing, we could witness that farmers took matters into their own hands and decided to establish a model in which they abstain from using pesticides during certain seasons. While the KGK cooperative still uses pesticides in the off-season, it provides a useful model for learning about the positive effects of reduced pesticide use for wild flora diversity on cereal fields. Furthermore, it is an intriguing model for initiating a transition toward sustainable agriculture, which would ideally eliminate pesticides entirely.
For this model to function, it is important to develop a regional value chain that brings together relevant actors, and attracts customers by advertising local products rather than organic. This is an important point. Many consumers tend to set organic food equal to local food, but this is not accurate. Choice experiments fielded in Germany have revealed that consumers prefer locally produced food to organic food, if they can choose (Hempel & Hamm, Citation2016). Considering that some KGK farmers have switched completely to organic production, from the viewpoint of facilitating a transition toward more sustainable agriculture, it appears promising to support locally produced food. The higher the visibility and trust in such a brand, the more farmers may be willing to join the KGK cooperative or consider setting up cooperatives that follow a similar approach. KGK is not the only cooperative with an intermediate production model between conventional and organic farming. A similar cooperative, also located in the Federal State of Baden-Württemberg by the name of LinzgauKorn, and KGK members have been approached by farmers from other German states as well to learn about their production model and its transferability.
At the same time, we have also alluded to the possibility that the KGK model is so attractive to farmers that they would prefer producing for the KGK cooperative than shifting entirely to organic farming. In other words, the KGK approach has value in facilitating the beginning of the transition process with reduced pesticide use, but it may also hinder a full transition to organic farming. To use the positive transition-related effects, and overcome the negative effects, a “capable” policy design process that brings together the right types of policy tools is required (Howlett & Mukherjee, Citation2014).
If policy-makers want to promote the KGK model, one policy tool for facilitating the long-term phase-out of pesticides could be a label certifying the local origin of food products. The state government of Baden-Württemberg supports such a quality label (Qualitätszeichen Baden-Württemberg), as well as the establishment of short food supply chains, where mass catering facilities must purchase foods from local producers. To ensure that farmers who are willing to shift to organic agriculture are not prevented from doing so due to an attractive alternative model, policy-makers must provide financial incentives.
Based on our study, we recommend policy-makers recognize and support intermediary production models between conventional and organic as an additional mechanism for reducing pesticide inputs to increase biodiversity, since policy outputs directly targeting pesticide reduction remain limited in effectiveness (Möhring et al., Citation2020). Farmers have developed their own models for meeting demands from society and public policy for sustainable food production, which has produced a spectrum of different farming approaches. The positive impacts of these approaches can motivate farmers to adopt similar models (Möhring & Finger, Citation2022) or to further increase the ambition of existing models. Therefore, it is worth assessing the impacts of these models on the future research and replicating similar studies across different regions within Germany and the EU. Communication of the respective findings to both farmers and policy-makers would stimulate mutual learning and foster transformation processes for reaching higher political goals, such as the Farm to Fork Strategy.
Correction
This article has been corrected with minor changes. These changes do not impact the academic content of the article.
Supplemental Material
Download MS Word (12.6 KB)Acknowledgments
We are grateful to Volker Kaltschmitt and Roland Waldi (KraichgauKorn) for sharing their knowledge, detailed information, and initiating further contact with KGK farmers, and Dr Markus Sonnberger for contributing to field data collection at organic farms. We thank Dr Peter Sack at our Herbarium (HEID) for curating vouchers and collecting plant material, and numerous students who contributed to fieldwork (Eva Holzberg, Lukas Meysen, Raphael Pörsel, Jan Schäfer, Charlotte Lauel, Frithjof Pölzing), as well as interview transcription and KGK farmer surveys (Anna Markus, Marie Pröll). Cornelia Wiethaler assisted with contacting organic farmers. For the publication fee we acknowledge financial support by Deutsche Forschungsgemeinschaft within the funding programme „Open Access Publikationskosten“ as well as by Heidelberg University.
Disclosure statement
No potential conflict of interest was reported by the authors.
Supplementary material
Supplemental data for this article can be accessed online at https://doi.org/10.1080/23311932.2023.2202892
Additional information
Funding
References
- Federal Ministry of Food and Agriculture - BMEL. (2021). Ökologischer Landbau in Deutschland. BMEL, Berlin.
- Agency for the Environment Baden-Württemberg, 2020. Rote Listen und Artenverzeichnisse. https://www.lubw.baden-wuerttemberg.de/natur-und-landschaft/rote-listen.
- Alons, G. (2017). Environmental policy integration in the EU’s common agricultural policy: Greening or greenwashing? Journal of European Public Policy, 24(11), 1604–18. https://doi.org/10.1080/13501763.2017.1334085
- Aulagnier, A., & Goulet, F. (2017). Des technologies controversées et de leurs alternatives. Le cas des pesticides agricoles en France, 59(3), sdt. https://doi.org/10.4000/sdt.840.
- Bartier, P. M., & Keller, C. P. (1996). Multivariate interpolation to incorporate thematic surface data using inverse distance weighting (IDW). Computers & Geosciences, 22(7), 795–799. https://doi.org/10.1016/0098-3004(96)00021-0
- Beckman, J., Ivanic, M., & Jelliffe, J. (2021). Market impacts of farm to fork: Reducing agricultural input usage. Applied Economic Perspectives and Policy, 44(4), 1995–2013. https://doi.org/10.1002/aepp.13176
- Böcker, T., Möhring, N., & Finger, R. (2019). Herbicide free agriculture? A bio-economic modelling application to Swiss wheat production. Agricultural Systems, 173, 378–392. https://doi.org/10.1016/j.agsy.2019.03.001
- Braun-Blanquet, J. (2012). Pflanzensoziologie: Grundzüge der Vegetationskunde, 3., neubearb. und wesentl. vermehrte Aufl. 1964 (Softcover reprint ed.). Springer, Wien.
- Brühl C.A., & Zaller J.G. (2019) Biodiversity decline as a consequence of an inappropriate environmental risk assessment of pesticides. Frontiers in Environmental Science, 7, 177. https://doi.org/10.3389/fenvs.2019.00177
- Cullen, M. G., Thompson, L. J., Carolan, J. C., Stout, J. C., Stanley, D. A., & Nieh, J. C. (2019). Fungicides, herbicides and bees: A systematic review of existing research and methods. PLoS One, 14(12), e0225743. https://doi.org/10.1371/journal.pone.0225743
- Daugbjerg, C., & Feindt, P. H. (2017). Post-exceptionalism in public policy: Transforming food and agricultural policy. Journal of European Public Policy, 24(11), 1565–1584. https://doi.org/10.1080/13501763.2017.1334081
- Eurostat, 2021. Agri-environmental indicator - consumption of pesticides. https://ec.europa.eu/eurostat/statistics-explained/index.php?title=Agri-environmental_indicator_-_consumption_of_pesticides.
- Federal Environment Agency - UBA. 2021. Pesticides. https://www.umweltbundesamt.de/en/topics/soil-agriculture/ecological-impact-of-farming/pesticides
- Finger, R., & El Benni, N. (2013). Farmers’ adoption of extensive wheat production – Determinants and implications. Land Use Policy, 30(1), 206–213. https://doi.org/10.1016/j.landusepol.2012.03.014
- forsa, 2020. Pestizide in der Landwirtschaft. http://www.umweltinstitut.org/fileadmin/Mediapool/Downloads/01_Themen/05_Landwirtschaft/Pestizide/Messprojekt_Pestizide_Luft/forsa_Ergebnisbericht_Einsatz_von_Pestiziden-2-1.pdf.
- Geiger, F., Bengtsson, J., Berendse, F., Weisser, W. W., Emmerson, M., Morales, M. B., Ceryngier, P., Liira, J., Tscharntke, T., Winqvist, C., Eggers, S., Bommarco, R., Pärt, T., Bretagnolle, V., Plantegenest, M., Clement, L. W., Dennis, C., Palmer, C., Oñate, J. J. … Hawro, V. (2010). Persistent negative effects of pesticides on biodiversity and biological control potential on European farmland. Basic and Applied Ecology, 11(2), 97–105. https://doi.org/10.1016/j.baae.2009.12.001
- Güngör, Ş. (2011). Measuring plant species diversity in alpine zones: A case study at the Kazdaği National Park, in Turkey. Arch Biol Sci (Beogr), 63(4), 1147–1156. https://doi.org/10.2298/ABS1104147G
- Hempel, C., & Hamm, U. (2016). Local and/or organic: A study on consumer preferences for organic food and food from different origins. International Journal of Consumer Studies, 40(6), 732–741. https://doi.org/10.1111/ijcs.12288
- Hofmeister, H., & Garve, E. (1998). Lebensraum Acker (2nd ed., pp. 1–322). Parey Verlag.
- Howlett, M., & Mukherjee, I. (2014). Policy design and non-design: Towards a spectrum of policy formulation types. Politics and Governance, 2(2), 57. https://doi.org/10.17645/pag.v2i2.149
- Kandyla, A., & Gherghina, S. (2018). What triggers the intention to use the European citizens’ initiative? the role of benefits, values and efficacy. JCMS: Journal of Common Market Studies, 56(6), 1223–1239. https://doi.org/10.1111/jcms.12737
- Koch, M. A., Meyer, N., Engelhardt, M., Thiv, M., Bernhardt, K. -G., & Michling, F. (2016). Morphological and genetic variation of highly endangered Bromus species and the status of these neolithic weeds in central Europe. Plant Systematics and Evolution, 302(5), 515–525. https://doi.org/10.1007/s00606-016-1279-5
- Le Velly, R., Goulet, F., & Vinck, D. (2021). Allowing for detachment processes in market innovation. The case of short food supply chains. Consumption Markets & Culture, 24(4), 313–328. https://doi.org/10.1080/10253866.2020.1807342
- Marja, R., Tscharntke, T., & Batáry, P. (2022). Increasing landscape complexity enhances species richness of farmland arthropods, agri-environment schemes also abundance – a meta-analysis. Agriculture, Ecosystems & Environment, 326(1971), 107822. https://doi.org/10.1016/j.agee.2021.107822
- Meynen, E., Schmithüsen, J., Gellert, J., Neef, E., Müller-Miny, H., & Schultze, J. H. (1962). Handbuch der naturräumlichen Gliederung Deutschlands. In Bd. (Vol. 2). Bad Godesberg.
- Millard, J., Outhwaite, C. L., Kinnersley, R., Freeman, R., Gregory, R. D., Adedoja, O., Gavini, S., Kioko, E., Kuhlmann, M., Ollerton, J., Ren, Z. -X., & Newbold, T. (2021). Global effects of land-use intensity on local pollinator biodiversity. Nature Communications, 12(1), 2902. https://doi.org/10.1038/s41467-021-23228-3
- Möhring, N., & Finger, R. (2022). Pesticide-free but not organic: Adoption of a large-scale wheat production standard in Switzerland. Food Policy, 106, 102188. https://doi.org/10.1016/j.foodpol.2021.102188
- Möhring, N., Ingold, K., Kudsk, P., Martin Laurent, F., Niggli, U., Siegrist, M., Studer, B., Walter, A., & Finger, R. (2020). Pathways for advancing pesticide policies. Nature Food, 1(9), 535–540. https://doi.org/10.1038/s43016-020-00141-4
- Nezadal, W. (2014). Pflanzengesellschaften der Acker und Ackerbrachen. In Hampicke, U., Böcker, R., & Konold, W. (Eds.), Handbuch Naturschutz und Landschaftspflege (pp. 1–7). https://doi.org/10.1002/9783527678471.hbnl2000010
- Perović, D. J., Gámez-Virués, S., Landis, D. A., Tscharntke, T., Zalucki, M. P., Saura, S., Furlong, M. J., Desneux, N., Sciarretta, A., Balkenhol, N., Schmidt, J. M., Trematerra, P., & Westphal, C. (2021). Broadening the scope of empirical studies to answer persistent questions in landscape-moderated effects on biodiversity and ecosystem functioning. In D. Bohan (Ed.), The future of agricultural landscapes, Part III (Vol. 65, pp. 109–131). Issn Ser. Elsevier Science & Technology.
- Persson, Å., & Runhaar, H. (2018). Conclusion: Drawing lessons for environmental policy integration and prospects for future research. Environmental Science & Policy, 85, 141–145. https://doi.org/10.1016/j.envsci.2018.04.008
- Persson, Å., Runhaar, H., Karlsson-Vinkhuyzen, S., Mullally, G., Russel, D., & Widmer, A. (2018). Environmental policy integration: Taking stock of policy practice in different contexts. Environmental Science & Policy, 85, 113–115. https://doi.org/10.1016/j.envsci.2018.03.029
- Pierny, M., 1994. Bestandsaufnahme zum aktuellen Entwicklungszustand der Ackerbegleitflora und ihre räumliche Differenzierung in Baden-Württemberg. https://lel.landwirtschaft-bw.de/pb/MLR.LEL-SG,Lde/Startseite/Unsere+Themen/Ackerwildkrautaecker.
- Pretty, J. (2008). Agricultural sustainability: Concepts, principles and evidence. Philosophical Transactions of the Royal Society B: Biological Sciences, 363(1491), 447–465. https://doi.org/10.1098/rstb.2007.2163
- Rosic, N., Bradbury, J., Lee, M., Baltrotsky, K., & Grace, S. (2020). The impact of pesticides on local waterways: A scoping review and method for identifying pesticides in local usage. Environmental Science & Policy, 106, 12–21. https://doi.org/10.1016/j.envsci.2019.12.005
- Schaub, S. (2019). Salient to whom? The positioning of German political parties on agricultural pollutants in water bodies. Water, 11(11), 2278. https://doi.org/10.3390/w11112278
- Schaub, S. (2021). Public contestation over agricultural pollution: A discourse network analysis on narrative strategies in the policy process. Policy Sciences, 54(4), 783–821. https://doi.org/10.1007/s11077-021-09439-x
- Schraml, A. (2018). Mehr Biodiversität im Getreidebau mit KraichgauKorn© und LinzgauKorn©. Ergebnisbericht zu den Schlagbegehungen 2018. Free Press.
- Schraml, A., 2019. Mehr Biodiversität im Getreidebau mit KraichgauKorn© und LinzgauKorn©. Ergebnisbericht zu den Schlagbegehungen 2019. ifab,
- Shannon, C. E. (1948). A mathematical theory of communication. Bell System Technical Journal, 27(3), 379–423. https://doi.org/10.1002/j.1538-7305.1948.tb01338.x
- Sharma, A., Kumar, V., Shahzad, B., Tanveer, M., Sidhu, G. P. S., Handa, N., Kohli, S. K., Yadav, P., Bali, A. S., & Parihar, R. D. (2019). Worldwide pesticide usage and its impacts on ecosystem. SN Applied Sciences, 1(11), 1–16. https://doi.org/10.1007/s42452-019-1485-1
- Spellerberg, I. F., & Fedor, P. J. (2003). A tribute to Claude Shannon (1916-2001) and a plea for more rigorous use of species richness, species diversity and the ‘Shannon-Wiener’ Index. Global Ecology and Biogeography, 12(3), 177–179. https://doi.org/10.1046/j.1466-822X.2003.00015.x
- Ssymank, A. (1994). Neue Anforderungen im europäischen Naturschutz: das Schutzgebietssystem Natura 2000 und die FFH-Richtlinie der EU. Natur und Landschaft, 69(9), 395–406.
- Tosun, J., Béland, D., & Papadopoulos, Y. (2022). The impact of direct democracy on policy change: Insights from European citizens’ initiatives. Policy & Politics, 50(3), 323–340. https://doi.org/10.1332/030557321X16476244758073
- Tosun, J., & Koch, M. A. (2022). Policy mixes for biodiversity: A diffusion analysis of state-level citizens’ initiatives in Germany. Journal of Environmental Policy & Planning, 24(5), 513–525. https://doi.org/10.1080/1523908X.2021.1992265
- Tosun, J., Lelieveldt, H., & Wing, T. S. (2019). A case of ‘muddling through’? The politics of renewing glyphosate authorization in the European Union. Sustainability, 11(2), 440. https://doi.org/10.3390/su11020440
- Tosun, J., & Varone, F. (2020). Politicizing the use of glyphosate in Europe: Comparing policy issue linkage across advocacy organizations and Countries. Journal of Comparative Policy Analysis: Research and Practice, 50(2), 1–18.
- Tscharntke, T., Grass, I., Wanger, T. C., Westphal, C., & Batáry, P. (2021). Beyond organic farming - harnessing biodiversity-friendly landscapes. Trends in Ecology & Evolution, 36(10), 919–930. https://doi.org/10.1016/j.tree.2021.06.010
- Tscharntke, T., Tylianakis, J. M., Rand, T. A., Didham, R. K., Fahrig, L., Batáry, P., Bengtsson, J., Clough, Y., Crist, T. O., Dormann, C. F., Ewers, R. M., Fründ, J., Holt, R. D., Holzschuh, A., Klein, A. M., Kleijn, D., Kremen, C., Landis, D. A., Laurance, W. … Westphal, C. (2012). Landscape moderation of biodiversity patterns and processes - eight hypotheses. Biological Reviews, 87, 661–685.
- Vialatte, A., Tibi, A., Alignier, A., Angeon, V., Bedoussac, L., Bohan, D. A., Bougherara, D., Carpentier, A., Castagneyrol, B., Cordeau, S., Courtois, P., Deguine, J. -P., Enjalbert, J., Fabre, F., Féménia, F., Fréville, H., Goulet, F., Grateau, R., Grimonprez, B. … Hannachi, M. (2021). Promoting crop pest control by plant diversification in agricultural landscapes: A conceptual framework for analysing feedback loops between agro-ecological and socio-economic effects. In D. Bohan (Ed.), The future of agricultural landscapes, part III (Vol. 65, pp. 133–165). Issn Ser. Elsevier Science & Technology.
- Wörz, A., & Thiv, M. (2015). The temporal dynamics of a regional flora—The effects of global and local impacts. Flora-Morphology, Distribution, Functional Ecology of Plants, 217, 99–108. https://doi.org/10.1016/j.flora.2015.09.013
- Zeitlin, J., Weimer, M., van der Duin, D., Kuhn, T., & Jensen, M. D. (2022). Governance reforms and public acceptance of regulatory decisions: Cross-national evidence from linked survey experiments on pesticides authorization in the European Union. Regulation & Governance.
- Zollet, S., & Maharjan, K. L. (2021). Resisting the vineyard invasion: Anti-pesticide movements as a vehicle for territorial food democracy and just sustainability transitions. Journal of Rural Studies, 86, 318–329. https://doi.org/10.1016/j.jrurstud.2021.06.020