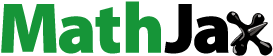
Abstract
Recently, blue/purple maize has gained prominence for its beneficial health properties, however, in Mexico, post-harvest handling of pigmented corn grains reaches losses of up to 80%, where in addition to quantity, nutritional and nutraceutical quality is seriously affected by inadequate storage conditions. The objective of this research was to quantify the nutritional and nutraceutical changes in blue corn grains stored for four months at 6, 25, and 35 °C. The results indicate that, at the fourth month of storage, temperatures affected the nutritional content to a different extent, with decreases of 66.83% in moisture and 57.79% in protein, and with increases of 10.92% in carbohydrates and 49.67% in starch. The concentration of phenols, flavonoids and total anthocyanins decreased by 49.33, 61.09 and 53.33%, after four months of storage at 35 °C, which were reflected in reductions in antioxidant capacity by 17.87, 17.84 and 18.93% for 2, 2− diphenyl − 1−picrylhydrazyl (DPPH), 2,2-azino-bis (3−ethylbenzothiazoline −6−sulfonic acid) (ABTS) and ferric reducing antioxidant power (FRAP), respectively. However, no significant changes in the nutraceutical content were generated during the storage of the grains at 6 °C. High temperatures over a prolonged storage period could seriously affect the concentrations of functional compounds in blue/purple corn kernels before consumption.
1. Introduction
Maize (Zea mays) is a native plant of Mexico and it has 59 races of maize, which represent a significant percentage of the 220 to 300 races existing in America (Rodríguez-Salinas et al., Citation2019). The breeds show different grain pigmentation with colors ranging from black to pale pink, and the most common are red and blue (Arellano-Vázquez et al., Citation2021). Maize with blue/purple pigmentation is characterized by high concentrations of polyphenolic compounds and anthocyanins in the aleurone layer and pericarp (Kapcum & Uriyapongson, Citation2018). Corn rich in polyphenols and anthocyanins has a high antioxidant capacity that helps to counteract chronic-degenerative diseases such as prostate cancer, type 2 diabetes, obesity, visual and cardiovascular problems (Kadiri, Citation2017).
According to the Agricultural and Fisheries Information Service of Mexico (Agricultural and Fisheries Information Service of Mexico AFISM, Citation2022), the production of pigmented maize represents less than 1% compared to white and yellow maize. Pigmented maize is cultivated by small producers in rural communities, who usually store the grain in an artisanal way in rustic constructions (tejabanes), sacks, special baskets made of palm leaves (tenates), among others, for their subsistence and self-consumption (García-Lara & Serna-Saldivar, Citation2019). Unfortunately, postharvest losses in small-scale cultivated maize are high, around 60 to 80% (García-Lara et al., Citation2020), where in addition to volume, the content of nutraceutical and nutritional compounds are seriously affected, given that most of the chemical components that are concentrated in the grains in their different structures (pericarp, aleurone layer, endosperm and germ), are degraded by biotic (pests and phytopathogens) and abiotic factors (temperature, light, pH and oxygen) (Chaaban et al., Citation2017).
In Mexico, the main states where pigmented maize are grown are the State of Mexico, Puebla, Tlaxcala, Oaxaca, Chiapas, and Veracruz, which present a wide climatic variation (temperatures from 5 to 40 °C), this compromises the time in which the corn kernels can preserve their nutritional and nutraceutical properties until they reach the final consumer (Mexican Commission for the Knowledge and Use of Biodiversity (CONABIO, Citation2020; Odjo et al., Citation2022). However, to date there is no study that documents the rate of reduction of chemical components in blue grain corn, that is subjected to different temperatures for long storage times. Therefore, the objective of this study was to evaluate the impact of temperature and storage time on the concentration of nutritional compounds, nutraceuticals, and antioxidant capacity in blue corn grains.
2. Materials and methods
2.1. Genetic material
For the experiment, seeds of genotypes of blue/purple corn (Zea mays) were collected in different Mexican states (Veracruz, Sinaloa, and Puebla). The genotypes were identified and characterized by Dr. Juan Manuel Hernández Casillas (Table ) and the materials are stored in the Seed Technology Training and Development Center (CCDTS), of the Universidad Autónoma Agraria Antonio Narro (UAAAN).
Table 1. Pigmented (blue) maize genotypes selected for the study
2.2. Seed cultivation
The seeds collected from each genotype were cultivated during the spring-summer 2021 agricultural cycle, at the Bajío UAAAN Experimental Field in Saltillo, Coahuila, Mexico (25° 21“29”“N latitude, 101° 02” 21’’ W longitude), at an altitude of 1742 masl, with an average annual rainfall of 350–400 mm and an average annual temperature of 19.8 °C. The genotypes were planted in four-row plots 8 m long, with 80 cm spacing between rows and 20 cm between plants without delimitations, obtaining 120 plants per genotype. Pollination control of each material was carried out by the difference in flowering days of each genotype (62, 106 and 126 days for AV, AS and AP, respectively). For the agronomic management of the crop, fertilizer was applied at a dose of 60 N, 60 P and 30 K kg/ha at the sowing moment, the second application was 30 days after sowing (60 N, 00 P and 00 K kg/ha), together with the first weeding, using drip irrigation during the crop cycle. Of the total harvest, 50% was used for the study.
2.3. Sample collection and storage conditions
Grain samples were obtained by taking 25 cobs from each planted row. The cobs of each genotype were defoliated, shelled, and finally mixed in a Riffle 040 G–020 homogenizer (Hexi District, Tianjin, CHN). From each pool, 200 g samples were weighed with four replicates for each genotype, placed in semi-kraft paper bags (No 1, 8.51 × 20.49 cm, caliber 50–60 g/m2) and polyethylene (27.99 × 35.00 cm, caliber 0.910 g/cm3) to protect them from light and relative humidity. Subsequently, the samples were stored at 6, 25 and 35 °C, in a Torrey R-36 refrigerator (Guadalupe, Nuevo León, MEX) and two Humboldt H-30110 incubators (Lake Forest, Illinois, USA) for 120 days. The samples were free of insect infestation and no chemical was used for their preservation. The analyzes were carried out at 0 (T0), 30 (T30), 60 (T60), 90 (T90) and 120 (T120) days of storage. On each sampling, 50 g were taken from each repetition: 25 g were used for nutritional analyses, and the remaining 25 g for nutraceutical analyses. Maize grains were ground at each sampling date with a Cgoldenwall HC-500 electric pulverizer mill (Xia Cheng, Zhejiang, CHN). Then, the flour was sieved to obtain particles of <0.50 mm (35 standard mesh). The obtained flour was used to carry out the analytical procedures.
2.4. Proximal analysis
The chemical analyzes were carried out with the flour obtained for each genotype on each sampling date (0, 30, 60, 90 and 120 days), using the methods of the Association of Official Analytical Chemists (AOAC, Citation2010), crude protein was quantified by Kjeldahl procedure (AOAC 960.52), moisture using the oven-drying method (AOAC 925.09) and carbohydrates content was determined by difference (subtracting crude protein (%), moisture (%), crude fat (%) (by the Soxhlet extraction (AOAC 923.03)), crude fiber (%) (as the organic residue remaining after digesting with H2SO4 and NaOH (AOAC 920.86)) and crude ash (%) (by incineration in muffle furnace (AOAC 923.03)) contents of the corn sample from 100). % Carbohydrate = 100 – (% protein + % fat + % moisture + % fibre + % ash), the results were reported in percentages (Rodríguez-Salinas et al., Citation2019). Starch content analysis was determined through a colorimetric technique, by detecting the formation of a bluish starch-iodine complex, the results were reported in g/100 g of sample, according to the methodology established by Guzmán et al. (Citation2017).
2.5. Extraction of phenolic compounds (free and bound)
The extraction of free and bound phenolic compounds was carried out according to Rodríguez-Salinas et al. (Citation2019). For the extraction, 200 mg of corn flour were weighed, which were suspended with 4 mL of 80% methanol, purged for 30 s with argon, and stirred for 2 h at 200 rpm in the dark. Afterwards, the samples were centrifuged at 5000 rpm for 5 min and the supernatant was recovered and stored at − 20 °C until analysis. The bound phenolic compounds were obtained from the solid residues of the free phenols, adding 5 mL of 2 M NaOH and purging with argon for 30 s. The mixture was then stirred for 2 h. Subsequently, the pH was adjusted to 2.5 with concentrated HCl and the phenolics were extracted with 5 mL of ethyl acetate twice. The ethyl acetate extracts were combined and placed in a Yamato RE201 rotary evaporator (Yamato, Kanagawa, JPN), to evaporate the ethyl acetate for 30 min. Once evaporated, the sample was resuspended with 4 mL of 80% methanol.
2.6. Quantification of total phenols and flavonoids (free and bound)
The determinations of total phenols and total flavonoids were performed in a Thermo Spectronic BioMate3 spectrophotometer (New York, New York, USA), according to López-Contreras et al. (Citation2015). The determination of the total phenol content was carried out using the Folin-Ciocalteu reagent, using gallic acid as a standard for the calibration curve (0 to 200 mg/L). The absorbance of the samples was measured at 750 nm, and the results were expressed as milligrams of gallic acid equivalent per hundred grams of sample (mg GAE/100 g). The determination of the total flavonoid content was based on the reaction of the complex of aluminum chloride and sodium hydroxide, using (+)-catechin as a reference standard at a concentration of 0 to 200 mg/L. The absorbance of the samples was measured at 510 nm and the result was reported as equivalent milligrams of (+)-catechin per 100 grams of sample (mg EC/100 g).
2.7. Grain chromaticity
Before grinding the grain, on each sampling date, the color characteristics were determined with a Konica Minolta CR-10 reader (Shinjuku, Tokyo, JPN) on the pericarp of the grain, placing 100 g of grain in a Petri dish (4.73 cm × 1.50 cm). The chromatic parameters were obtained using the CIELCH color systems (L*, C*, h) established by the International Commission on Food Illumination (CIE, 2004). L* defines lightness (0 = black, 100 = white), C* (chroma; saturation level of h) and h (hue angle: 0° = red, 90° = yellow, 180° = green, 270° = blue). To graph the color, the Color Hexa online software was used using the values obtained from L*, C* and h (ColorHexa: Color Encyclopedia: Information and Conversion, Citation2019).
2.8. Total anthocyanin content
The total anthocyanin content was evaluated according to Abdel-Aal and Hucl (Citation1999), mixing 200 mg of corn flour with 10 mL of 1 N ethanol-HCl (85:15 v/v, pH 1, 4 °C), purging for 30 s with argon and stirred for 30 min at 200 rpm. Subsequently, the sample was centrifuged at 6,000 rpm (4 °C, 15 min) and the absorbance was measured at 535 nm, the anthocyanin content was expressed as milligrams of cyanidin-3-glucoside equivalents (C3GE) per hundred grams of sample (mg C3GE/100 g): C= (A/ɛ) × (V/1000) × MW × (1/sample weight) × 106, where: C= concentration in mg C3GE/L, A = sample absorbance, ɛ= molar absorptivity (mg C3GE = 26.965 1/cm 1/mol), V= sample volume, MW= molecular weight of C3G (449.2 g/mol). Anthocyanins and antioxidant capacity were determined by espectophotometry too.
2.9. Antioxidant capacity
Antioxidant capacity assays for ABTS (2,2’-azino-bis (3-ethylbenzothiazoline-6-sulfonic acid)), DPPH (2,2-diphenyl-1-picrylhydrazyl) and FRAP (ferric reducing antioxidant power) were performed according to Rodríguez-Salinas et al. (Citation2019). The results were reported in micromoles of Trolox (6-hydroxy-2,5,7,8-tetramethylchroman-2-carboxylic acid) equivalents per hundred grams of sample (µmol TE/100 g), taking as reference the calibration curve of Trolox (0 to 500 µmol/L).
2.10. Compounds retention at the end of storage
The calculation of the apparent retention of moisture, carbohydrates, protein, starch, total phenols, total flavonoids, total anthocyanins, and antioxidant capacity at the end of the storage period (120 days), was carried out according to de Oliveira et al. (Citation2017) with the following equation:
2.11. Experimental design and statistical analysis
The study was established under a randomized experimental design, with a 3 × 5 × 3 factorial arrangement (3 genotypes × 5 storage times × 3 temperatures). The statistical difference between samples was evaluated using an analysis of variance (ANOVA), and the means were compared with Tukey’s test (p ≤ 0.05) using the statistical package SPSSS version 21.0 (SPSS Inc., Chicago, Illinois, USA). Results were reported as mean values of three samples ± standard deviation.
3. Results and discussion
The results indicate significant differences (p ≤0.05) for proximal and nutraceutical determinations when comparing time × temperature interaction, however, the genotype × time × temperature interaction did not show significant differences (Supplementary Table A1). This could be due to the characteristics of the blue/purple corn kernels, such as the type of endosperm and the concentration of polyphenolic compounds in the pericarp and aleurone layer (Luna-Vital et al., Citation2017; Prabakaran et al., Citation2018).
3.1. Proximal analysis
The various temperatures and storage time affected the proximal content to a different extent (Table ). The moisture content decreased drastically at 35 °C after 30 days of storage (49.51%), while at the end of storage (T120) at 25 and 35 °C. grains showed a decrease in moisture of 20.72 and 66.83%. On the other hand, the protein content decreased 54.92, 48.72 and 54.64%, after 30 days of storage at all temperatures (6, 25 and 35 °C), which were maintained without presenting considerable changes at T120. At T30, the carbohydrate content increased 10.01, 9.23 and 15.38% at 6, 25 and 35 °C, and remained constant at T120. For the starch content, average increases of 37.39% at T60 and 49.67% at T120 were observed at the three temperatures.
Table 2. Content of proximal analysis (moisture, protein, carbohydrates, and starch) in genotypes of blue maize stored at different temperatures for 120 days
In white corn grains stored for 180 days at temperatures of 25 and 45 °C, moisture content (24.72 and 37.80%), protein digestibility (5.19 and 9.09%) and starch (9.88 and 15.08%) were reduced; while at temperatures of 10 and 25 °C, the total soluble sugar content is increased at 30 days of storage (1.62%) and continue rising until the end of storage (9.68 and 14.75%) (Ur-Rehman et al., Citation2002). Likewise, studies on stored cereals (180 days) show decreases of 32 and 48.80% at 25 and 45 °C in moisture content (corn) and increases at 25 and 45 ºC from 9.30 to 17.90%, and from 11.90 to 31.80% in total soluble sugars for rice, corn, and wheat, respectively (Ur-Rehman, Citation2006).
Grains are hygroscopic (they can gain or lose moisture), therefore, temperatures above 20 °C during prolonged periods of storage allow greater moisture loss in the external structures of the grain (Prabakaran et al., Citation2018). Likewise, proteins found in the endosperm and pericarp can be negatively affected by Maillard reactions, forming intermediate compounds of amino-protein groups, and reducing sugar carbonyl groups that inhibit the activity of proteolytic and amylolytic enzymes, causing reductions in protein content (Ur-Rehman, Citation2006). Studies have documented protein contents of 8–18% of dry matter in corn, rice, sorghum and wheat for the major proteins (albumins, globulins, prolamins and glutelins) (Cervantes-Pahm et al., Citation2014; Han et al., Citation2019). Vegetable proteins, in addition to their nutritional role for humans and animals, also provide an essential structuring role in the quality and sensory attributes (flavor and texture) of foods, thanks to their functional properties such as solubility, oil/water binding, texturizing, foaming, emulsification, gel formation and fibrillation; however, cereal proteins are restricted by their large molecular weight and limited water solubility under conditions typical of most food products (neutral and slightly acidic) (Poutanen et al., Citation2022). On the other hand, the increase in carbohydrates as storage time passes is related to their metabolism and the regulation of sugars and sugar derivatives, because of the activity of endogenous amylases, which break down starch into simpler sugars, such as glucose and sucrose, among others (Birungi et al., Citation2018; Wang et al., Citation2022).
The increase of 79.54% of glucose and fructose have been reported in corn grains stored for four months, as a result of the gradual degradation of oligosaccharides, which in turn can intervene in the Amadori and Maillard reactions, causing non-enzymatic glycation of the amino groups of different molecules, giving rise to glycated proteins and fructosyl derivatives, which in turn cause changes in the structure of the cytoplasm of the grain cells (de León-Sánchez et al., Citation2006). In addition, the increase in the starch content of blue maize in this study could be related to the type of endosperm that characterizes it, a starchy endosperm (floury with a higher content of amylopectin than amylose), which contains more starch than protein, compared to white maize with vitreous endosperm (Agama-Acevedo et al., Citation2013).
3.2. Concentration of total phenols (free and bound)
Samples of T30 at temperatures of 25 and 35 °C showed an increase of 21.89 and 37.18% for free phenols, and 5.29 to 13.56% for bound phenols, respectively (Table ). On the other hand, after T60 to T120, total phenols presented the greatest reductions depending on the storage time and temperature. The most affected were free phenols with decreases of 50.82 and 68.89%, while bound phenols were reduced to 32.15 and 55.19%, with temperatures ranging from 25 to 35 °C. These decreases were reflected in the final retention percentage. After 120 days of storage, the temperature at 6 °C presented the best retention of free and bound phenols with 80.47 and 87.19%. Most of the cereals that are used for human consumption must be stored for long periods after harvest, to later be transformed from raw material to food through thermal processes that affect the chemical composition of nutritional and nutraceutical components (Colín-Chávez et al., Citation2020). For example, in sorghum grain, storage studies have shown that temperatures of 25 and 40 °C decreased the content of 3-Deoxyanthocyanidins by 26.43 and 29.84%, respectively (de Oliveira et al., Citation2017). It has also been reported that pigmented corn grains that were subjected to nixtamalization thermal processes presented a reduction from 61.46 to 76.53% in total phenolic compounds, this implies that antioxidants during postharvest handling (storage and conditioning) and industrialization, the concentration of antioxidant compounds in the final product is reduced by more than 97% (Žilić et al., Citation2015).
Table 3. Total phenol content (free and bound) in genotypes of blue maize stored for 120 days at different temperatures
Phenolic compounds are not stable and are easily degraded after harvest since the reduction in moisture directly impacts the polyphenol content. In addition, the decrease in the total content of polyphenols during storage could be attributed to different processes such as their degradation by oxidation, giving rise to the formation of new antioxidant polymers, and to the biological activity of the grain (Colombo et al., Citation2021; Zhang et al., Citation2021).
3.3. Total flavonoids (free and bound)
The concentration of total flavonoids was similar to that of total phenols, since at T30, temperatures of 25 and 35 °C favored the soluble flavonoids to increase by 20.32 and 24.75%, and for bound flavonoids, 19.28 and 22.92% (Table ). However, from T30 to T120 at 25 and 35 °C, the decreases were 48.37 and 69.19% for soluble flavonoids, while for bound flavonoids, it was 47.15 and 71.57%. At T120 days of storage, the retention percentage of total flavonoids was 38.91% at 35 °C. Recent studies in cereals show that flavonoids are altered by storage time and temperature, affecting the number and distribution of isoflavone groups (aglycones, glucosides, acetyl glucosides and malonyl glucosides), where the percentage of total malonyl glucosides decreases as storage time is prolonged; at 48 weeks at 4, 20 and 40 °C there were decreases of 77.1, 70.5 and 57.8% (Prabakaran et al., Citation2018). Alterations in the percentage of total malonyl glycosides affect the percentage of total glycosides and total acetyl glycosides, which occurs due to the degradation and decarboxylation of the malonyl group, and with it the acetyl group (Ed Nignpense et al., Citation2021).
Table 4. Total flavonoid content (free and bound) in genotypes of blue maize stored for 120 days at different temperatures
Stored grains rich in carbohydrates show significant differences in the flavonoid content at the metabolic level, as indicated by Wang et al. (Citation2022), in an analysis of rice grains stored at 20 °C for 540 days. They reported that the metabolites most altered during storage are flavonoids, phenolic acids among others (saccharides and alcohols, organic acids, alkaloids, and lipids) with a variation of 11.80% in flavonoids, between fresh and stored samples. The stability of the flavonoids depends on the physicochemical environment where the grains are stored, the factors that are most attributed to the deterioration of these compounds are heat, light, oxygen, and the grain’s own respiration metabolism (Chaaban et al., Citation2017).
3.4. Grain chromaticity and total anthocyanins
The color results show that the values of L* and C* decrease as the days of storage pass at all temperatures, the greatest loss of lightness was observed at 35 °C (Table ). The “h” decreased over the storage period at 25 °C more than 6 °C, but remained stable at 35 °C, indicating that yellow is the most predominant chromatic characteristic. The changes in the color properties are reflected in the anthocyanin content, which show reductions in the retention percentage ranging from 56.74 to 46.67%, for 25 and 35 °C at the end of storage. In corn grains stored for 360 days at 35 °C, color saturation and hue angle were affected by more than 21% (Paraginski et al., Citation2014). In addition, in corn grains, significant changes have been reported in the color parameters L*, a* and b*, with variations ranging from 1.39 to 29.34% after having been stored for 180 days in propylene bags with lime and with micronized lime (Odjo et al., Citation2022). In the pericarp of purple corn, the concentration of anthocyanins decreased as the storage days passed from 15.14 to 40.87% and presented a negative correlation in the decrease in color saturation and in the hue angle, which indicated that the stability of the grain color depends on the degradation of polyphenols (Luna-Vital et al., Citation2017). Color changes in the grain during storage can be attributed to the loss of moisture in its external structures, and as a consequence, a concentration or decrease of water-soluble compounds in the pericarp, which are associated with storage conditions (content of moisture, temperature and duration) and the activity of polyphenol oxidase, which catalyzes reactions of phenols generating other compounds (p-cresol and o-benzoquinone) whose oxidation and polymerization leads to the formation of melanins (Shafiekhani et al., Citation2018). There are factors that can determine the content of easily oxidizable polyphenols, forming polymerized substances that affect the color of the blue/purple maize grain, such as light, the conservation degree, and the stability of anthocyanins, which it decreases considerably at a higher degree of hydroxylation and methoxylation of the aglycone. In addition, the content of polyphenols in food is also influenced by culinary preparation methods, since these substances are often present in high concentrations in the external parts of the food (Eliášová et al., Citation2020; Luna-Vital et al., Citation2017).
Table 5. Total anthocyanin content and chromatic properties in blue maize genotypes stored for 120 days at different temperatures
3.5. Antioxidant properties
The antioxidant capacity in blue corn genotypes at the end of storage (T120) shows retention percentages of 96.03, 83.71 and 76.71% at temperatures of 6, 25 and 35 °C (Table ). DPPH assay showed values from 599.76 to 494.75 µmol TE/100 g (free) and from 797.53 to 604.56 µmol TE/100 g (bound); the ABTS assay showed values in the free antioxidant capacity of 703.78 to 546.34 µmol TE/100 g and bound was 855.63 to 683.14 µmol TE/100 g; by the FRAP method, the free antioxidant capacity was 843. 08 to 662.63 µmol TE/100 g and bound was 968.74 to 683.14 µmol TE/100 g. The least affectations in the antioxidant capacity at T120 were reflected in the proportion of soluble compounds at 6 °C, with 0.22, 3.24 and 0.02% for DPPH, ABTS and FRAP, while the greatest affectations occurred in the proportion linked to 35 °C, with reductions of 24.19, 20.16 and 23.17%, respectively. Similarly, the antioxidant capacity of blue/purple pigmented maize after 60 days at low temperatures (4 °C), did not show significant reductions (1.04%) by the ABTS method (Kapcum & Uriyapongson, Citation2018). However, at 35 °C, pigmented (black) rice grains that were stored for 180 days presented reductions of 24.11 and 45.60% for the ABTS and FRAP assays, respectively (Ito et al., Citation2019). Likewise, in sorghum grains with different pigmentations, the antioxidant capacity by DPPH is affected by time and storage conditions, with a decrease of 29.33% after 56 days of storage (Zhang et al., Citation2021). The content and type of polyphenolic compounds is related to the antioxidant activity, the greater the decrease in these compounds, the antioxidant activity is negatively affected (Tables ) (Yamuangmorn et al., Citation2021). During postharvest handling, temperatures above 20 °C affect the stability of the antioxidant activity, due to the variation in the moisture content in the grains and the possible oxidation of the polyphenol molecules (Antony & Farid, Citation2022; Dar et al., Citation2016). On the other hand, during the storage process, decreases in the content of phenolic acids can be observed, since some polyphenols are degraded, giving rise to new ones such as anthocyanins that can be broken down into derivatives of benzoic acid (syringic acid or 4-hydroxybenzoic), which affects the levels of antioxidant capacity (Hou et al., Citation2022; Zhang et al., Citation2021).
Table 6. Antioxidant capacity DPPH, ABTS and FRAP (free and bound) in genotypes of blue maize stored for 120 days at different temperatures
According to the antioxidant capacity results, the affectations levels in each method was in the following order DPPH<FRAP<ABTS. The antioxidant capacity was less affected in DPPH, since this method only determines the ability to donate hydrogen atoms of the compounds that are in water-soluble form (polyphenols) present in the pericarp and aleurone layer of the blue corn kernel (Duangpapeng et al., Citation2019; Francavilla & Joye, Citation2020). The greater decrease in antioxidant capacity observed in FRAP and ABTS is correlated with the phenolic content, molecules with the capacity to reduce ferric ions, in addition to the presence of compounds of a lipophilic nature (polyunsaturated fatty acids, linoleic acid, carotenoids and vitamins A, D, E, K) (Enaru et al., Citation2021; Martínez-Martínez et al., Citation2019). The differences in the results for each method can be attributed to the differential sensitivity of the phenolics present in the extract, to free radicals, and to the reducing capacities of each method (Minh, Citation2021).
4. Conclusions
In this research, temperatures at 25 and 35 °C were the most affected the moisture content, polyphenols, chromatic properties, and antioxidant capacity of the grains of the blue corn genotypes after four months of storage. In blue corn grains, the protein concentration is lower and decreases considerably after 30 days of storage at all temperatures, while the carbohydrate content increased when the storage temperature increased, and storage time was prolonged. Grains showed minimal decreases in the concentration of polyphenols at 6 °C, therefore, blue corn kernels better preserve bioactive compounds this temperature. High temperatures during a prolonged period of storage could considerably affect the concentrations of functional and nutritional compounds in blue corn kernels, before being consumed. These results are important since the temperatures used represent the wide diversity of climates in which blue corn is grown and consumed by small producers. Based on the results obtained, it is suggested that blue corn grains should not be stored at 25 and 35 °C, to minimize the degradation of their chemical components during postharvest handling.
Supplemental Material
Download MS Excel (19.1 KB)Acknowledgments
Eddaliz García-Reyes thanks the National Council of Science and Technology (CONACyT) for the master’s degree scholarship with CVU 1108488. The authors thank the “Scientific Research Support Program” of the Research Directorate of the Antonio Narro Autonomous Agrarian University (UAAAN), for project 38111-425105001-2446 granted to Josué I. García-López.
Disclosure statement
No potential conflict of interest was reported by the authors.
Supplementary material
Supplemental data for this article can be accessed online at https://doi.org/10.1080/23311932.2023.2218630.
Additional information
Funding
References
- Abdel-Aal, E. S., & Hucl, P. (1999). A rapid method for quantifying total anthocyanins in blue aleurone and purple pericarp wheats. Cereal Chemistry Journal, 76(3), 350–13. https://doi.org/10.1094/CCHEM.1999.76.3.350
- Agama-Acevedo, E., Juárez-García, E., Evangelista-Lozano, S., Rosales-Reynoso, O. L., & Bello-Pérez, L. A. (2013 Retrieved December 28, 2022). Characteristics of maize starch and relationship with the enzymes involved in its biosynthesis. Agrociencia, 47(1), 01–12. ISSN 2521-9766. Available at, http://www.scielo.org.mx/scielo.php?script=sci_arttext&pid=S1405-31952013000100001&lng=es&tlng=es.
- Agricultural and Fisheries Information Service of Mexico (AFISM). 2022. Agricultural production statistical yearbook. https://nube.siap.gob.mx/cierreagricola/
- Antony, A., & Farid, M. (2022). Effect of temperatures on polyphenols during extraction. Applied Sciences, 12(4), 2107. https://doi.org/10.3390/app12042107
- AOAC. (2010). Association of official analytical chemists official methods of analysis of the association of official analytical chemists (18th ed.). AOAC International.
- Arellano-Vázquez, J. L., Herrera-Zamora, A., Gutiérrez-Hernández, G. F., Ceja-Torres, L. F., & Flores Gómez, E. (2021). Color, anthocyanin content and seed dimensions in inbred lines of blue maize and their crosses. Idesia (Arica), 39(3), 75–82. https://doi.org/10.4067/S0718-34292021000300075
- Birungi, C., Nabubuya, A., & Mukisa, I. M. (2018). Effect of sweet potato endogenous amylase activation on in vivo energy bioavailability and acceptability of soy‐enriched orange‐fleshed sweet potato complementary porridges. Food Science and Nutrition, 6(4), 1119–1127. https://doi.org/10.1002/fsn3.653
- Cervantes-Pahm, S. K., Liu, Y., & Stein, H. H. (2014). Digestible indispensable amino acid score and digestible amino acids in eight cereal grains. The British Journal of Nutrition, 111(9), 1663–1672. https://doi.org/10.1017/S0007114513004273
- Chaaban, H., Ioannou, I., Paris, C., Charbonnel, C., & Ghoul, M. (2017). The photostability of flavanones, flavonols and flavones and evolution of their antioxidant activity. Journal of Photochemistry and Photobiology A, Chemistry, 336, 131–139. https://doi.org/10.1016/j.jphotochem.2016.12.027
- Colín-Chávez, C., Virgen-Ortiz, J. J., Serrano-Rubio, L. E., Martínez-Téllez, M. A., & Astier, M. (2020). Comparison of nutritional properties and bioactive compounds between industrial and artisan fresh tortillas from maize landraces. Current Research in Food Science, 3, 189–194. https://doi.org/10.1016/j.crfs.2020.05.004
- Colombo, R., Ferron, L., & Papetti, A. (2021). Colored corn: An up-date on metabolites extraction, health implication, and potential use. Molecules, 26(1), 199. https://doi.org/10.3390/molecules26010199
- ColorHexa: Color Encyclopedia: Information and Conversion. (2019). Computer Software. www.colorhexa.com
- Dar, B. N., Sharma, S., & Nayik, G. A. (2016). Effect of storage period on physiochemical, total phenolic content and antioxidant properties of bran enriched snacks. Journal of Food Measurement and Characterization, 10(4), 755–761. https://doi.org/10.1007/s11694-016-9360-x
- de León-Sánchez, F. D., Vizcaíno, S. C., Carballo, A. C., & Bernal-Lugo, I. (2006 Retrieved December 30, 2022). Sugar content in 4 maize cultivars (Zea mays) and their rate of deterioration in storage. Revista Iberoamericana de Tecnología Postcosecha, 8(1), 38–47. ISSN: 1665-0204. Available at, https://www.redalyc.org/articulo.oa?id=81380106.
- de Oliveira, K. G., Queiroz, V. A. V., de Almeida- Carlos, L., De Morais- Cardoso, L., Pinheiro-Sant’ana, H. M., Anunciação, P. C., De Menezes, C. B., Da Silva, E. C., & Barros, F. (2017). Effect of the storage time and temperature on phenolic compounds of sorghum grain and flour. Food Chem, 216, 390–398. https://doi.org/10.1016/j.foodchem.2016.08.047
- Duangpapeng, P., Lertrat, K., Lomthaisong, K., Paul Scott, M., & Suriharn, B. (2019). Variability in anthocyanins, phenolic compounds and antioxidant capacity in the tassels of collected waxy corn germplasm. Agronomy, 9(3), 158. https://doi.org/10.3390/agronomy9030158
- Ed Nignpense, B., Francis, N., Blanchard, C., & Santhakumar, A. B. (2021). Bioaccessibility and bioactivity of cereal polyphenols: A review. Foods, 10(7), 1595. https://doi.org/10.3390/foods10071595
- Eliášová, M., Kotíková, Z., Lachman, J., Orsák, M., & Martinek, P. (2020). Influence of baking on anthocyanin content in coloured-grain wheat bread. Plant, Soil & Environment, 66(8), 381–386. https://doi.org/10.17221/210/2020-PSE
- Enaru, B., Drețcanu, G., Pop, T. D., Stǎnilǎ, A., & Diaconeasa, Z. (2021). Anthocyanins: Factors affecting their stability and degradation. Antioxidants, 10(12), 1967. https://doi.org/10.3390/antiox10121967
- Francavilla, A., & Joye, I. J. (2020). Anthocyanins in whole grain cereals and their potential effect on health. Nutrients, 12(2922), 2922. https://doi.org/10.3390/nu12102922
- García-Lara, S., García-Jaimes, E., & Ortíz-Islas, S. (2020, 87). Field effectiveness of improved hermetic storage technologies on maize grain quality in central Mexico. Journal of Stored Products Research, 87, 101585. https://doi.org/10.1016/j.jspr.2020.101585
- García-Lara, S., & Serna-Saldivar, S. O. (2019). Corn history and culture. In Serna-Saldivar, S. O. (Ed.), Corn (3rd ed) (pp. 1–18). AACC International Press, Oxford. https://doi.org/10.1016/B978-0-12-811971-6.00001-2
- Guzmán, C., Hurtado, A., Carreño, C., & Casos, I. (2017). Producción de polihidroxialcanoatos por bacterias halófilas nativas utilizando almidón de cáscaras de Solanum tuberosum L. Scientia Agropecuaria, 8(2), 109–118. https://doi.org/10.17268/sci.agropecu.2017.02.03.
- Han, F., Han, F., Wang, Y., Fan, L., Song, G., Chen, X., Jiang, P., Miao, H., & Han, Y. (2019). Digestible indispensable amino acid scores of nine cooked cereal grains. The British Journal of Nutrition, 121(1), 30–41. https://doi.org/10.1017/S0007114518003033
- Hou, C. Y., Hsieh, C. C., Huang, Y. C., Kuo, C. H., Chen, M. H., Hsieh, C. W., & Cheng, K. C. (2022). Development of functional fermented dairy products containing Taiwan Djulis (Chenopodium formosanum Koidz.) in regulating glucose utilization. Fermentation, 8(9), 423. https://doi.org/10.3390/fermentation8090423
- Ito, V. C., Zielinski, A. A. F., Demiate, I. M., Spoto, M., Nogueira, A., & Lacerda, L. G. (2019). Effects of gamma radiation on the stability and degradation kinetics of phenolic compounds and antioxidant activity during storage of (Oryza sativa L.) black rice flour. Brazilian Archives of Biology and Technology, 62. https://doi.org/10.1590/1678-4324-2019180470
- Kadiri, O. (2017). A review on the status of the phenolic compounds and antioxidant capacity of the flour: Effects of cereal processing. International Journal of Food Properties, 20(sup1), S798–S809. https://doi.org/10.1080/10942912.2017.1315130
- Kapcum, C., & Uriyapongson, J. (2018). Effects of storage conditions on phytochemical and stability of purple corn cob extract powder. Food Science & Technology, 38(suppl 1), 301–305. https://doi.org/10.1590/1678-457X.23217
- López-Contreras, J. J., Zavala-García, F., Urías-Orona, V., Martínez-Ávila, G. C. G., Rojas, R., & Niño-Medina, G. (2015). Chromatic, phenolic and antioxidant properties of Sorghum bicolor Genotypes. Notulae Botanicae Horti Agrobotanici Cluj-Napoca, 43(2), 366–370. https://doi.org/10.15835/nbha4329949
- Luna-Vital, D., Li, Q., West, L., West, M., & de Mejia, E. G. (2017). Anthocyanin condensed forms do not affect color or chemical stability of purple corn pericarp extracts stored under different pHs. Food Chemistry, 232, 639–647. https://doi.org/10.1016/j.foodchem.2017.03.169
- Martínez-Martínez, R., Vera-Guzmán, A. M., Chávez-Servia, J. L., Bolaños, E. N. A., Carrillo-Rodríguez, J. C., & Pérez-Herrera, A. (2019 Retrieved January 1, 2023). Bioactive compounds and antioxidant activities in pigmented maize landraces. Interciencia, 44(9), 549–556. ISSN: 0378-1844. Available at, https://www.redalyc.org/articulo.oa?id=33961096008.
- Mexican Commission for the Knowledge and Use of Biodiversity (CONABIO). (2020). Maize races of Mexico. https://www.biodiversidad.gob.mx/diversidad/alimentos/maices/razas-de-maiz
- Minh, N. P. (2021). Influence of thermal treatment on Anthocyanin, total phenolic content and antioxidant capacity of Pigmented Maize (Zea mays L.). Plant Science Today, 8(4), 1075–1078. https://doi.org/10.14719/pst.2021.8.4.1294
- Odjo, S., Palacios-Rojas, N., Burgueno, J., Corrado, M., Ortner, T., & Verhulst, N. (2022). Hermetic storage technologies preserve maize seed quality and minimize grain quality loss in smallholder farming systems in Mexico. Journal of Stored Products Research, 96, 101954. https://doi.org/10.1016/j.jspr.2022.101954
- Paraginski, R. T., Vanier, N. L., Berrios, J. D. J., de Oliveira, M., & Elias, M. C. (2014). Physicochemical and pasting properties of maize as affected by storage temperature. Journal of Stored Products Research, 59, 209–214. https://doi.org/10.1016/j.jspr.2014.02.010
- Poutanen, K. S., Kårlund, A. O., Gómez-Gallego, C., Johansson, D. P., Scheers, N. M., Marklinder, I. M., Eriksen, A. K., Silventoinen, P. C., Nordlund, E., Sozer, N., Hanhineva, K. J., Kolehmainen, M., & Landberg, R. (2022). Grains–a major source of sustainable protein for health. Nutrition Reviews, 80(6), 1648–1663. https://doi.org/10.1093/nutrit/nuab084
- Prabakaran, M., Lee, J. H., Chung, A., Kim, S. H., Woo, K. S., Kim, M. J., Ahmad, I. M., Kim, S.-H., & Chung, I.-M. (2018). Changes in Soybean (Glycine max L.) flour fatty-acid content based on storage temperature and duration. Molecules, 23(9), 2269. https://doi.org/10.3390/molecules23092269
- Rodríguez-Salinas, P. A., Muy-Rangel, D., Urias-Orona, V., Zavala-García, F., Suarez-Jacobo, A., Heredia, J. B., Rubio-Carrasco, W., & Nino-Medina, G. (2019). Thermal processing effects on the microbiological, physicochemical, mineral, and nutracéutical properties of a roasted purple maize beverage. Farmacia, 67(4), 587–595. https://doi.org/10.31925/farmacia.2019.4.5
- Shafiekhani, S., Wilson, S. A., & Atungulu, G. G. (2018). Impacts of storage temperature and rice moisture content on color characteristics of rice from fields with different disease management practices. Journal of Stored Products Research, 78, 89–97. https://doi.org/10.1016/j.jspr.2018.07.001
- Ur-Rehman, Z. (2006). Storage effects on nutritional quality of commonly consumed cereals. Food Chemistry, 95(1), 53–57. https://doi.org/10.1016/j.foodchem.2004.12.017
- Ur-Rehman, Z., Habib, F., & Zafar, S. I. (2002). Nutritional changes in maize (Zea mays) during storage at three temperatures. Food Chemistry, 77(2), 197–201. https://doi.org/10.1016/S0308-81460100337-5
- Wang, Q., Zhang, D., Zhao, L., Liu, J., Shang, B., Yang, W., Duan, X., & Sun, H. (2022). Metabolomic analysis reveals insights into deterioration of rice quality during storage. Foods, 11(12), 1729. https://doi.org/10.3390/foods11121729
- Yamuangmorn, S., Jumrus, S., Jamjod, S., Yimyam, N., & Prom-U-Thai, C. (2021). Stabilizing grain yield and nutrition quality in purple rice varieties by management of planting elevation and storage conditions. Agronomy, 11(1), 83. https://doi.org/10.3390/agronomy11010083
- Zhang, Y., Truzzi, F., D’Amen, E., & Dinelli, G. (2021). Effect of storage conditions and time on the polyphenol content of wheat flours. Processes, 9(2), 248. https://doi.org/10.3390/pr9020248
- Žilić, S., Delić, N., Basić, Z., Ignjatović-Micić, D. R. A. G. A. N. A., Janković, M., & Vančetović, J. (2015). Effects of alkaline cooking and sprouting on bioactive compounds, their bioavailability and relation to antioxidant capacity of maize flour. Journal of Food and Nutrition Research, 54(2). https://doi.org/10.31883/pjfns-2019-105100