Abstract
The fruits of citrus family are rich in flavonoids with superior antioxidant properties; citrus bioactive components are potentially beneficial without adverse effects or cytotoxicity in healthy individuals. Citrus fruit peel contains ample amount of hesperidin which is flavanone glycoside and has showed effective role in biological systems as an antioxidant and anti-inflammatory bioactive compound. Different studies have investigated such roles of hesperidin. Due to superior antioxidant potential of hesperidin and its derivatives, these compounds have been intensively studied for their anti-cancer and cardio protective perspective. This review article is a collection of updated data covering different aspects of hesperidin and its derivatives, like occurrence, physical and chemical composition, pharmacokinetics and safety of these compounds. Moreover, effective role of these compounds against different lifestyle-related metabolic syndromes in mechanistic perspective has also been extensively discussed in this article.
PUBLIC INTEREST STATEMENT
Hesperidin is often found in citrus fruits including lemons, limes, mandarins, oranges, and grapefruits. Hesperidin works best when taken alongside vitamin C, like other bioflavonoids. Comparatively speaking, hesperidin from orange juice is more readily available than hesperidin from whole oranges. Hesperidin may offer a variety of advantages. They include reducing blood pressure, treating diabetes and its consequences, depression, decreasing blood cholesterol, and improving skin health. They also include easing the symptoms of vascular disorders including chronic venous insufficiency and hemorrhoids. Other advantages of hesperidin include immune system support, possible cancer prevention, and menopausal symptom management. Depending on the problem it is being used to treat, hesperidin may also be mixed with other antioxidants, herbs, or substances. If you are using certain medications, such as anticoagulants, blood thinners, blood pressure medicines, or calcium channel blockers, you should not take this supplement.
1. Introduction
Numerous bioactive plant chemicals have indeed been studied for their potential to reduce inflammation and other pathogenic disorders notably heart dysfunction (Corrêa et al., Citation2019). Polyphenolic compounds fall into two main categories: flavonoids and nonflavonoids. Flavonoids encompass flavanones like citrus, flavanols like black tea, and flavonols like coffee and garlic. But at the other side, nonflavonoids contain anthocyanins, which are found in cherry, and hydroxycinnamic acids, which are found in coffee (Williamson, Citation2017). The primary molecules of all polyphenols, flavanones like hesperidin and naringin, are regarded as the major category (Del Rio et al., Citation2013).
Lebreton, a French scientist, originally extracted hesperidin (C28H34O15) from orange peel (Roohbakhsh et al., Citation2015). The hesperidin comprises flavonoid glycoside. Genus Rutaceae, the banana fruit, citrus fruits, the lemon peel, and other plants are among the several sources of hesperidin. Additionally, it may be found in the uppercase sections of the Rubiceae genus as well as in the roots and entire grasslands of cruciferous plants. Hesperetin or methyl eriodictyol, an aglycon, is linked to rutinose [6-O-(l-Rhamnopyranosyl)-D-glucopyranose] and/or [6-O-(l-Rhamnosyl)-D-glucose], a disaccharide, in the composition of hesperidin (Hajialyani et al., Citation2019).
In Europe, oranges particularly associated fruit drinks, that possess considerable amounts of hesperidin and naringin, remain arguably the most popular citrus fruit commodities (Zamora-Ros et al., Citation2010). And over 90% of the flavonoids inside the delicious orange are hesperidin and naringin (Erlund, Citation2004). Despite citrus liquids have sizable amounts of hesperidin, the dense tissues of citrus fruits have the greatest quantities of hesperidin (Tomás‐Barberán & Clifford, Citation2000). Because it doesn’t accumulate, hesperidin is regarded as safe when used as a supplement or nutritional additive, with nearly no negative impacts (Hajialyani et al., Citation2019).
The micronutrients present in a variety of botanical commodities, particularly the organic polyphenols, have an impact on a number of biological and metabolic processes within the organism. The advantages of flavonoids in treating numerous neuronal and behavioural illnesses have been demonstrated in several researches. Inside the brains of depressed experimental animals, flavonoids modulate the monoaminergic neurotransmitters, acting like antidepressant (Mahmoud et al., Citation2019).
Numerous polyphenols produced from plants have significant antioxidant capacity in several test techniques. Ultimate scavenging predicted values constants for a wide range of oxidizing radicals could be determined through pulse radiolysis experimentations, allowing for more framework commonalities and, when combined with EPR spectroscopy, providing a thorough understanding of the insight into the mechanisms underlying these antioxidant responses (Musumeci et al., Citation2020; Rauf et al., Citation2022; Y. Wang et al., Citation2022).
Significant pharmaceutical properties of hesperidin, that is especially abundant in the peels of satsuma mandarin, have indeed been demonstrated. Nevertheless, there is no reliable method for detecting or identifying hesperidin particles in Citrus unshia. Hesperidin does, nevertheless, produce crystals in a few tissues of the Rutaceae & Umbelliferae, according to histology investigations. Hesperidin’s health benefits and absorption can be influenced by a variety of variables. Some of these have to do with how the substance is prepared and stored, its content, the features of the recipient, the dietary environment, the physical shape it takes when consumed (such as juices, aqueous extraction, or pills), and the makeup of the intestinal flora (Barreca et al., Citation2017).
Great antioxidant property and potential function in the prevention of persistent disorders including malignancy and cardiac attack, polyphenols have acquired popularity over the past several decades. Hesperidin is an affiliate of flavanone set of flavonoids and having linked to a number of medical advantages, including being an antioxidant, reducing inflammation, fighting cancer, and preventing bone resorption (Abdulaziz Ahmeedah Rabee & Bennasir, Citation2018; El-Kersh et al., Citation2021; Koolaji et al., Citation2020; Liskova et al., Citation2021; Pandey & Khan, Citation2021). Citrus fruits and drinks could influence significantly to hesperetin absorption in the meal; for instance, in Finland, hesperetin accounted for 50% of all flavonoid ingestion (Adan & Baran, Citation2016; Dhingra et al., Citation2022).
Optimizing the bioavailability of the hesperitin is essential for reaping all of its advantages. Intake of 0.5–1 liters of orange juice causes human volunteers to have very low plasma concentrations of hesperitinagycone (<2 micro mol per liter). Plants frequently produce glycoside-conjugated flavonoids. As hesperetin-7-O-rutinoside (hesperidin), which is an aglycone related to glucose & rhamnose sugars, hesperetin is found in citrus fruits. In contrast, glycosides containing rhamnose are weakly assimilated contrasted to their aglycones and glucoside counterparts, with the glucose constituent of flavonoids being hypothesised to be the primary predictor of their administration in humans (Adan & Baran, Citation2016).
2. Variables influencing the bioavailability of hesperidin
2.1. α-L-Rhamnosidase performance and microbiota concentration
Hesperitin and a disaccharide component are conjugated to form hesperidin. This disaccharide compound prevents hesperitin from being absorbed in the intestinal tract, thus lowers the amount of hesperitin that is bioavailable (Nielsen et al., Citation2006). The gastrointestinal micro-flora’s enzymes, alpha rhamnosidase and beta glucosidase, metabolize a large portion of hesperidin by removing sugar and forming hesperitin, and that is an aglycone version of hesperidin (Borges et al., Citation2013). The gut microbiome influences alpha rhamnosidase performance, and when it is inactive, the large intestine stops producing hesperitin (Amaretti et al., Citation2015).
As a result, it may be inferred that differences in gut microbes have a major impact upon that bioavailability of hesperidin since they change the efficiency of the alpha rhamnosidase enzyme. It seems to be worth noting that there is a strong negative correlation among the excretion of hesperidin metabolites and the efflux of hesperetin catabolites produced by the gut microbiome, like HMPPA (Aschoff et al., Citation2016). This suggests that the microbes of individuals who excrete hesperidin at distinct rates possess varying hesperidin metabolism-related actions. There aren’t any investigations that explicitly show such impacts, despite the possibility that rhamnosidase activities affect the bioavailability of hesperidin as well as its biologic consequences. Consequently, more human in vivo investigations are required to determine how rhamnosidase efficiency affects the bioavailability of hesperidin (Aschoff et al., Citation2016).
2.2. Food manufacturing and food matrix
The food matrix wherein hesperidin is ingested might affect how bioavailable it really is. The phrase “bio accessibility” through this context relates to portion of a substance which is liberated from its prevailing conditions in intestinal system and subsequently made accessible for intestinal amalgamation. The effect of complete fat yoghurt on permeability of orange juice flavanones that being examined in a clinical trial (Mullen et al., Citation2008). Following the ingestion of 250 mL of orange juice both by means of & devoid of 150 mL of complete fat yoghurt, hesperidin concentrations in the urine and serum were assessed. The findings showed that whereas yoghurt considerably decreased the proportion of flavanone metabolites voided 0–4 hours following orange juice administration, it had no effect on levels excreted for the whole 0–24 hour urine sampling interval. The scientists came to the conclusion that the low level of fats in the yoghurt presumably contributed to its lack of impact on bioavailability of orange juice flavanones (Mullen et al., Citation2008).
The dissolving of particular metabolite is a prerequisite for it to reach total circulation & have a physiological impact, which is linked to foodstuff atmosphere component. Poor dissolving of hesperidin, particularly in aqueous environments, has been reported (Majumdar & Srirangam, Citation2009). In a study it is examined the impact of hesperidin quantity and solubility on its bioavailability in a crossover clinical investigation including ten volunteers. Respondents received five distinct drinks with varying amounts of hesperidin. The findings demonstrated a link between hesperidin elimination and maximal concentration (Cmax) in serum and the concentration of soluble hesperidin in juice, but not with total hesperidin consumption. The scientists came to the conclusion that hesperidin’s bioavailability was significantly influenced by its solubility in the juice (Vallejo et al., Citation2010).
When juice excavation was used instead of orange slices in a research to assess the in-vitro bio availability of hesperidin in various matrix resources, it was shown that hesperidin’s bio permeability rose dramatically (Aschoff et al., Citation2015). The researchers came to the wrapping up that while poor flavonoid solubility might be the restricting reason, the reduced flavonoid values in orange juice contrasted to orange slices may not be as significant in terms of their intestine uptake (Aschoff et al., Citation2015).
In a medical trial comparing the bioavailability of hesperidin from citrus fruit and orange juice, renal hesperetin discharge did not distinguish from that seen after consuming orange juice, despite higher hesperidin dosages conveyed with the orange fruit. This finding suggests that discharge, solubility, and metabolic activity of dietary flavanones are overloaded when consumption surpasses. Hesperidin being trapped inside the fiber-rich matrix of orange fruit was additional theory that could be true (Aschoff et al., Citation2016). This fallout affords credence to hypothesis that orange fruits’ greater hesperidin content than orange juice provides just a modest nutritional advantage. However, no variations in bioavailability were found in similar medical trial by Brett et al., (Citation2008), depending on total urine hesperetin excretion of human participants following ingestion of orange fruit & Orange Juice matrices (Brett et al., Citation2008).
2.3. How nanotechnology affects the accessibility of hesperidin
Because they are more effective and have less adverse consequences than synthetic medications, organic antioxidants like hesperidin have showed promise in the management of malignant proliferation and other similar disorders (Majumdar & Srirangam, Citation2009; S. Wang et al., Citation2013). However, owing to decreased water mobility and poor absorption, hesperidin’s therapeutic application appears to have been severely constrained (Duranoğlu et al., Citation2018). To make the most use of this substance, it is important to resolve these difficulties (Duranoğlu et al., Citation2018). Studies in the multidisciplinary field of nanotechnology have several possibilities, including customized medication delivering, bio-imaging, and molecular diagnostics (Nie et al., Citation2007).
Additionally, this technique has the potential to resolve medication solubility and bioavailability problems, which show a substantial influence on the management of cancer (Gu et al., Citation2019; Merisko-Liversidge et al., Citation2003). In order to augment permeability, uptake, & bio-distribution of such flavonoids, numerous investigations were started on the development of hesperidin-based nanoparticle. To boost water solubilization, antioxidant properties, & oral uptake of hesperetin Gu et al., (Citation2019) designed two nano-based regimens, namely hesperetin-TPGS (D-a-tocopheryl polyethylene glycol 1000 succinate) micelles & hesperetin-phosphatidylcholine (PC) clusters. Such preparations increased hesperetin’s in-vitro antioxidant potential by 16 and 18 fold (Gu et al., Citation2019).
3. Hesperidin’s stereochemistry
The cyclic carbon in the hesperidin structure can produce the diastereoisomers -R and -S, though in nature, -S diastereoisomer is more common, as illustrated in . According to Lévèques et al. (Citation2012), hesperidin is found in fresh fruit items like orange juice in an S: R ratio of minimum 92:8 in support of the 2S-epimer.
Figure 1. (A); Hesperidin’s chemical composition and complete stereochemistry (3, 5, 7-trihydroxy-4-methoxyflavanone-7-rhamnoglucoside), (B); Hesperitin’s chemical composition and complete stereochemistry (3, 5, 7-trihydroxy-4-methoxyflavanone).
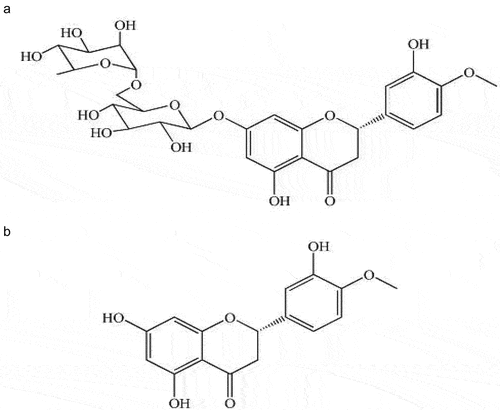
It has been suggested that flavonoids’ stereochemical characteristics affect its permeability (Donovan et al., Citation2006). The implications of hesperidin’s stereo-chemical characteristics on serum and urinary kinetics of hesperetin () have indeed been documented in detail (Yáñez et al., Citation2005), and might therefore exert an impact on the physiological activities of hesperetin as well as its gastrointestinal metabolism and transportation (Brand et al., Citation2010). Racemic hesperetin was administered to mice in an in vivo investigation, and it was shown that R-hesperetin significantly outperformed S-hesperetin in terms of area under the serum concentration-time curve (AUC), & accumulated urine elimination (Yáñez et al., Citation2008).
Figure 2. Hesperidin’s impact on lipid metabolism. (Source: Xiong et al., Citation2019)
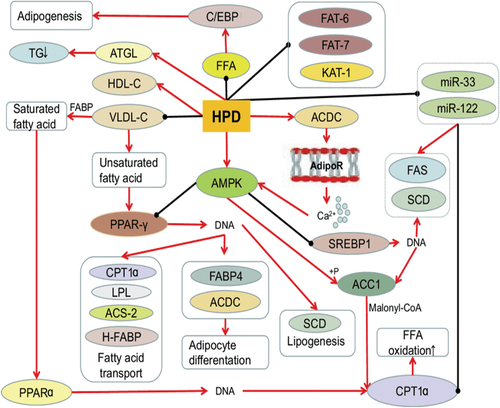
Additionally, when racemic hesperidin was given to a solitary rat orally, the amount of R-hesperetin that was excreted in the urine over the course of 24 hours was somewhat (by about 15%) higher than that of S-hesperetin. A greater sensitivity and ability for S-hesperetin was found in an in vitro investigation using human small intestine microsomes, which led to an estimated 5.2-fold enhanced catalytic effectiveness for synthesis of S-hesperetin glucuronides as opposed to R-hesperetin glucuronides (Brand et al., Citation2010). This is significant since, despite the fact that hesperidin is primarily found in nature as the 2S-epimer that is then converted into S-hesperetin upon consumption almost all investigations on hesperidin & hesperetin by means of “pure” substances is conducted on complex mixtures. That’s because most of hesperidin commodities are readily accessible in the market as a combination of both diastereoisomers. Owing of these conditions, several businesses are looking into creating hesperidin goods which are 100% S-hesperidin.
Hesperidin, a flavonone mostly found in citrus fruits, has newly come to light as a novel possible therapeutic treatment capable of modulating a number of susceptibility markers for cardiovascular diseases (CVDs). Hesperidin as well as its derivative chemicals has positive impacts on CVD risk variables in animal and in vitro investigations.
Hesperidin has therefore demonstrated glucose-lowering and anti-inflammatory actions in diabetic mice, dyslipidemia, obesity, and CVDs prevention benefits in obese mice, and antihypertensive and antioxidant properties in hypertensive models. Hesperidin’s potential to improve hypertension, obesity, cholesterol profiles, and glucose tolerance in humans is still up for debate, as shown by multiple experimental investigations that found no impact of its or orange juice-based therapies on key cardiovascular indicators. The positive benefits of hesperidin on CVD risk variables are the main topic of this review. We pay particular attention to the substantial interindividual variation in responding to hesperidin-based acute and chronic therapies that is at least partially explained by variations in gut flora. According to the available data, we hypothesise that a few of hesperidin’s paradoxical impacts in human studies are related to the variations in its bioavailability that is strongly reliant on the functioning of -rhamnosidase and the makeup of the gut flora (Capdevill et al, 2020).
The aglycone of hesperidin, main flavonoid found in delicious oranges, is hesperetin (()-4′-methoxy-3′, 5, 7-trihydroxyflavanone). Hesperetin may appear as both an S- and R-enantiomer due to its symmetrical C-atom, nevertheless in environment, 2S-hesperidin & its S-hesperetinaglycone are more common (Brand et al., Citation2010).
The heteropolycyclic, aromatic bioflavonoids hesperidin and naringenin have a variety of physiological and medicinal properties. Naringenin is an aglycon, whereas hesperidin is a flavanone glycoside. Citrus fruits, grapefruits, tomato, and certain other plants all contain large amounts of both chemicals. The most significant characteristics of such molecules are their antioxidant and free radical scavenging capacities, which enhance their preventive properties against a wide range of illnesses like melanoma, heart disease, hypercholesterolemia, high blood pressure, neurodegenerative disorders, metabolic syndrome, renal diseases, and skin problems.
4. Hesperidin’s effects on health
4.1. Impact of hesperidin on overweight or obesity
Hesperidin offers a broad assortment of beneficial possessions, counting antioxidant & anti-cancer potential, anti-inflammatory properties, the ability to dwindle blood pressure & blood lipids, and anti-obesity properties (Lu & Yip, Citation2023). Hesperidin and hesperitin are employed to alleviate obesity by reducing hunger because they possess the ability to control the CCK, an endocrine hormone that regulates hunger within the STC-1 cells (Kim et al., Citation2013). Hesperidin lowers cholesterol and triglyceride contents in chest muscle and poultry serum, and it improves fatty acid & lipid metabolism in poultry breast in a dose-dependent manner (Kamboh & Zhu, Citation2013). Glucaronide binding, Sulfate binding, hydrolysis, and N-acetylcysteine binding are a few of several forms of hesperidin that are mostly found in mice (Jiao et al., Citation2020). Table showed the specifics of how hesperidin influences weight loss.
Table 1. The specifics of how hesperidin influences weight loss
Blood urea nitrogen (BUN), alanine aminotransferase (ALT), aspartate amino transferase, and creatinine fibroblast growth factor may all be considerably lowered in renal tissue and plasma by hesperidin. On the other hand, diabetic mice that consume hesperidin have higher levels of x-Kl in their blood, liver, and renal tissues (Dokumacioglu et al., Citation2019). In rats with an impairment of glycolipid metabolism brought on by a high-fat way of eating, hesperidin at elevated doses increases the expression of stimulated protein kinase, adenosine 5-monophosphate, and mRNA. This has an impact on the insulin receptor substrate, other insulin signalling pathways, and genes like sterol regulatory element binding protein 1c (SREBP1C), FAS, & Acetylcol particularly pertaining to lipid metabolism (Pu, Citation2016).
4.2. Hesperidin’s impact on lipid metabolism
Obesity is the outcome of a body’s fat content rising over a specific threshold (Feng & Li, Citation2006). Therefore, alterations in fat metabolism lead to obesity. Hesperidin has the capacity to enhance lipid metabolism (Amiot et al., Citation2016). Triglycerides release and control a large number of adipokines and cytokines. Lipids are stored in the adipose tissues as triglycerides. Adipose tissue expands as a response of excess fat intake. This is done by adipocytes to offset the lipid burden (Assini et al., Citation2013). Hesperidin (0.08%) decreases adipose tissue, hepatic lipids, and aids in hepatic steatosis management. In meals heavy in lipid, hesperidin also lowers blood cholesterol levels and retinal binding protein (RBP) 4 levels (X. Wang et al., Citation2011). As spotted in , fatty acid metabolism is thought to be primarily regulated by two proteins, cutaneous fatty acid-binding protein (C-FABP) and heart fatty acid-binding protein (H-FABP).
Hesperidin controls cholesterol production, uptake, and heart fatty acid binding protein (H-FABR) and cutaneous fatty acid-binding protein (C-FABP) mRNA expression to improve fatty liver and hyperlipidemia (X. Wang et al., Citation2011). In obese hypertensive rats, hesperidin has indeed been demonstrated to lower serum total cholesterol, serum triglycerides, and systolic blood pressure via reducing serum fatty acid synthase (FFA), demonstrating that it possesses lipolytic action. Aside from that, this even performs a position in the fluctuations of very low density lipoprotein cholesterol (VLDL-C), greatly raises high density lipoprotein cholesterol (HDL-C), and significantly lowers plasma low density lipoprotein cholesterol (LDL-C) (Selvaraj & Pugalendi, Citation2012).
The three key genes engaged in the three phases of adipogenesis—perilipin, PPAR-r, and C/EBPBSREBP1C—are inhibited by hesperidin (Gómez-Zorita et al., Citation2017). Hesperidin has been proven to reduce SREBP1, a crucial gene for Stearoyl-CoA desaturase (SCD), in mice. Additionally, it restored the PKLR gene’s normal expression (Mosqueda-Solís et al., Citation2018a). Hesperidin has particular inhibitory effect in the early stages of differentiation in 3T3-L1 preadipocytes (Chambers et al., Citation2019). Hesperidin increases messenger RNA expression and accelerates the breakdown of adipocytes by working with hormone sensitive lipases (Jack et al., Citation2018). Hesperidin inhibits the expression of lipid metabolism-related genes such mediator subunit-15 (MDT-15), acetyl-CoA carboxylase-2 (PoD-2), 3-ketoacyl-CoA thiolase-1 (KAT-1), and acyl CoA synthetase-2 (AoS-2) to minimize fat storage (Peng et al., Citation2016). Hesperidin can enhance metabolism of fatty acids & lipids in the chicken breast (Kamboh & Zhu, Citation2013).
4.3. Hesperidin’s impact on glucose metabolism
Obesity-modifying variables, particularly in relation to glucose resistance, are expected to interplay alongside genetic polymorphisms associated with poor glucose metabolism (S. Y. Jung et al., Citation2016). Due to the carbon atoms it supplies, glucose is necessary for the constriction of compounds like amino acids, fatty acids, and nucleotides (Yang et al., Citation2013). Hesperidin exhibits inhibitory outcome on alpha-glucosidase inside hepaticG2 cells, which prevents the breakdown of amylose & amylopectin (Shen et al., Citation2012). By preventing entrance of the enzyme further into circuit and so slowing the formation of pyruvate, Alpha ketoglutarate, and oxaloacetate, hesperidin slows the progression of diabetes by reducing hepatic gluconeogenesis (S. Zareei, Boojar, et al., Citation2017).
Hesperidin has also demonstrated a diminution in blood sugar in diabetic mice caused by porcine streptozotocin (Franke et al., Citation2018). In accumulation, hesperidin initiates glycolysis & glycogenolysis in mice hepatocytes that have been separated and ruptured (Do Nascimento et al., Citation2018a). As per the proportion of sugar and hesperidin, hesperidin can be utilized to control or inhibit postprandial hyperglycemia by residing in the intestine glucose receptor (Shen et al., Citation2012). Hesperidin modulates PPAR-C activity, a nuclear protein transcription factor which controls lipid & glucose metabolism, to sustain glucose metabolism (Shin et al., Citation2013).
In newborn Goto-kakizaki rats, hesperidin & cyclodextrin-clathratehesperitin regulate blood sugar via changing performance of glucose-regulating enzymes & reducing blood and liver lipid profile. The hypoglycemia & hypolipidemic consequences of type-2 diabetes affect several genes that are crucial for producing specific proteins, including PPAR, 3-hydroy-3-methyl-glutatyl coenzyme A (HMG-CoA) reductase, & LDL receptors. RBP4 has indeed been acknowledged as an adipokine that regulates metabolism of glucose (Qian et al., Citation2016a).
The protein known as GLUT4 facilitates glucose absorption and raises intracellular glucose concentrations that are accessible for metabolic transformation, which encourages increased cell proliferation (Yang et al., Citation2013). According to X. Wang et al. (Citation2011), hesperidin has the ability to impact GLUT4 and lower RBP4 expression. Lipase activity is inhibited by insulin, which also slows down the breakdown of fat. Additionally, on the other hand, insulin can encourage the liver’s production of fatty acids, which in turn encourages the entrance of glucose into adipose cells and the conversion of that sugar into triglycerides (S. Li et al., Citation2004). Hesperidin enhances glucose homeostasis, insulin sensitivity, & short chain fatty acid (SCFA) synthesis by promoting intestine development. It also indirectly impacts insulin resistance, which controls the performance of adipocytes, muscle fibers, and hepatocytes. Hesperidin improves the relationship amongst problems of glucose metabolism and obesity by regulating the metabolism of insulin and glucose either explicitly or implicitly (Lima et al., Citation2019). Hesperidin’s impact on glucose metabolism was presented in .
Figure 3. Hesperidin’s impact on glucose metabolism. (Source: Xiong et al., Citation2019)
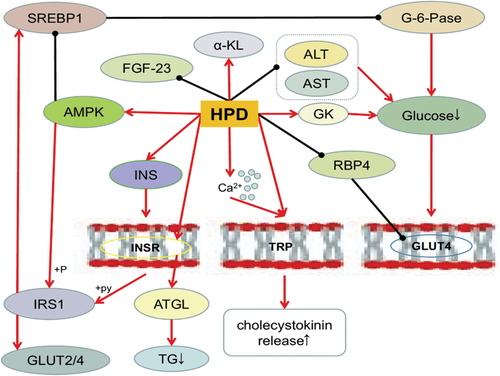
4.4. Hesperidin’s impact on oxidation and inflammation
Obesity and serum oxidative products deposition have been correlated (Colleluori et al., Citation2019). In diabetic mice, hesperidin reduces the level of sugar, glycosylated haemoglobin (HBA1C %), and nitric oxide while increasing serum insulin, glutathione hormone, vitamin E, and vitamin C concentrations. When hyperglycemia causes oxidative stress, hesperidin acts as a preventative agent (Mahmoud et al., Citation2012). Decreased antioxidant activities when hesperidin and alpha Amylase are combined (W. Y. Liu et al., Citation2017). Hesperidin possess capability to prevent the reduction of superoxide dismutase (SOD) & catalase action in the thymus and spleen and also formation of reactive oxygen species (ROS) inside rats after strenuous exercise (Estruel-Amades et al., Citation2019).
By preventing apoptosis, that is brought on through an elevated glucose meal and is a source of oxidative stress, hesperidin protects RGC-5 cells and lowers levels of ROS, MDA, caspase-9, caspase-3, & Bax/Bcl-2 (W. Y. Liu et al., Citation2017). This is seen in . Hesperidin has an adequate impact on the antioxidant levels of many vital nutrients, such as mutton’s high antioxidant activities when kept in the refrigerators (Simitzis et al., Citation2013). Enhance malondialdehyde MDA and superoxide dismutase SOD synthesis as well as overall antioxidant properties. Hesperidin could also increase plasma antioxidant potential (Kamboh & Zhu, Citation2013).
Figure 4. Hesperidin’s impact on oxidation and inflammation. (Source: Xiong et al., Citation2019)
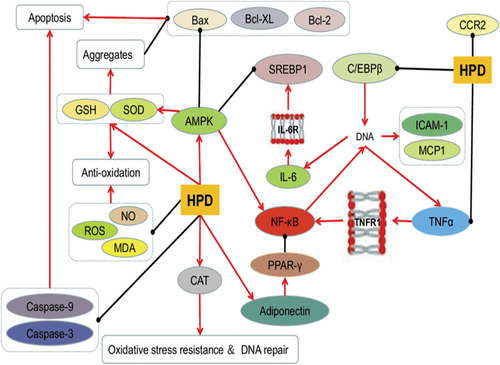
A research showed that various dosages of hesperidin, such as (100 mg/kg) considered to as medium dosages and (200 mg/kg) designated to as high dosages of hesperidin on hypercholesterolemic mice, enhanced the amount of endogenous enzyme GSH which had antioxidant activity (Yuce et al., Citation2009). Hesperidin ingestion affects liver fatty acid oxidation characteristics such as oxygen absorption, ketone generation, and citrate cycling activity (Constantin et al., Citation2013). A combination of curcumin, hesperidin, and rutin reduces the symptoms of hyperglycemia brought on by streptozatocin, including as impaired hepatic functioning, glucose dysregulation, and hepatic oxidative stress (S. Parmar et al., Citation2015).
Inflammation that is chronic and lasting at lower levels has been linked to obesity (Xiong et al., Citation2019). Obesity is thought to have its pathological roots in early inflammatory alterations in adipocytes. Necrosis factor kappa B (NF-KB) commencement & interleukin-6 synthesis are inhibited by the hormone adiponectin, which is produced from adipose tissue during inflammatory processes. Hesperidin can promote adipocyte differentiation and stimulate the secretion of adiponectin, which lowers fat buildup (Akiyama et al., Citation2009). White blood cells, red blood cells, and associated operational markers all greatly strengthen after receiving hesperidin orally intended for one month in type-2 diabetic rat. Hesperidin also increases the expression of adiponectin. Hesperidin affects adipose tissue to treat anemia (associated with diabetes) (Mahmoud, Citation2013).
In rats given a high fat diet, hesperidin has demonstrated the ability to increase blood antioxidant status. The process for lowering hepatic thiobarbituric acid reactive substance (TBARS) levels and spleen mass involves suppression of interleukin-6, macrophage chemo attractant protein (MCP-1), and C-reaction protein (RP). It also inhibits the metabolic alterations linked to cardiovascular disorders by preventing the inflammation and oxidative stress brought on through an elevated fat meal (Ferreira et al., Citation2016).
4.5. Hesperidin’s impact on hepatic diseases
The liver, which functions as the body’s primary digestive organ, is responsible for metabolizing xenobiotics through a number of different metabolic pathways, comprising conjugation, hydrolysis, hydration, elimination, and oxidation (Shirani et al., Citation2020). Any disturbance of these processes causes liver damage or hepatotoxicity, that could be brought on by a variety of factors including an interruption in the mitochondria, DNA destruction and apoptosis in the hepatocytes, and an excessive amount of liver enzymes in the blood (Thompson et al., Citation2017). Numerous xenobiotics have been reported to cause hepatotoxicity in over 50 million persons globally (Thompson et al., Citation2017).
One essential glycolipid established in cell wall of gram-negative bacteria is lipopolysaccharide (Ajuwon et al., Citation2014). In a research, mice were specified an intraperitoneal injection of lipopolysaccharide (1 mg/kg, single administration) to cause hepatotoxicity, that was demonstrated through an increase in concentrations of the enzymes aspartate aminotransferase (AST), alanine aminotransferase (ALT), alkaline phosphatase (ALP), & total bilirubin. While tissue and serum nitrite levels increased as well as the levels of thiobarbituric acid reactive substances (TBARS), glutathione (GSH) content & superoxide dismutase (SOD) activity diminished. Scientists came to conclusion that hesperidin might reduce nitric oxide and reactive oxygen species (ROS) formation & prevented lipopolysaccharide exacerbated hepatotoxicity after hesperidin treatment restored all of these aberrations. Additionally, lipopolysaccharide-induced histological alterations like Kupffer cell hyperplasia, necrosis, and pyknotic nuclei were mitigated by hesperidin (Kaur et al., Citation2006).
In a diverse research, rats were given 0.1 g of lipopolysaccharide intravenously. As a consequence, the mice’s concentrations of the proteins high-mobility group box 1 protein (HMGB1) and tumour necrosis factor alpha (TNF-alpha) elevated. Additionally, the liver’s level of apoptotic cells was increased by lipopolysaccharide. Hesperidin was sufficient to rectify the abnormalities brought on by lipopolysaccharide and displayed hepatoprotective properties in contrast to its anti-inflammatory exploit (Kawaguchi et al., Citation2004).
Animal hepatotoxicity is modelled using the plant lectin “concanavalin A”. As indicators of hepatocyte injury, this lectin causes Kupffer cells to produce TNF-α and interferon gamma (IFNγ) (Ju & Tacke, Citation2016). In a study, mice received concanavalin A (15 mg/kg, intravenously, once), which caused an increment in hepatic malondialdehyde (MDA) substance and a decline in SOD action, and a boost in the production of TNF-α and interferon gamma, as well as the appearance of the increased mobility box 1 protein HMGB1 and the stimulation of T cells. Additionally, increased AST and ALT activity were seen. Surprisingly, hesperidin may correct these abnormalities when given intravenously at a rate of 1000 mg/kg for ten days. As fundamental processes, it has been suggested that blocking hepatic oxidative stress, cytokine and HMGB1 release, & activating T cells are responsible. Hesperidin also lessens the liver necrosis and inflammatory infiltration brought on by concanavalin A (G. Li et al., Citation2014).
Microcystins are a class of cyclic heptapeptide hepatotoxic peptides generated by cyanobacteria. Microcystin-LR increases the production of ROS by inhibiting the actions of protein phosphatases (Shi et al., Citation2015). Mice were injected with microcystin-LR to cause hepatotoxicity in a study at a dosage of 200 g/kg (intraperitoneal, single injection). Gamma glutamyl transferase (GGT), lactate dehydrogenase (LDH), and ALT serum levels all rose. Additionally, it raised the amounts of MDA and methylglyoxal while inhibiting protein phosphatase. However, oral hesperidin treatment (300 mg/kg/d) completely undid all of these changes. Hesperidin, a strong antioxidant, shielded the liver from the damage brought on by microcystinLR (Wahsha, Citation2010).
This has been shown that ingesting ethanol causes hepatic inflammation and pro-inflammatory cytokines, and also ethanol’s initiation of oxidative stress, to cause hepatic cells to get injured and die (Bakhautdin et al., Citation2014). In a research, giving ethanol to mice causes the levels of TNF-, inhibitor of kappa B proteinalpha (IB), interleukin 1 beta (IL-1β), and interleukin 6 (IL-6) to significantly increase in the hepatocytes. Furthermore, ethanol lowers the capabilities of catalase (CAT), glutathione reductase (GR), glutathione-S-transferase (GST), and glutathione peroxidase (GPx), while raising blood concentrations of AST and ALT. This alcohol decreases the level of hepatic GSH while raising the liver’s MDA concentration. Hesperidin has demonstrated to rectify these abnormalities and demonstrate its hepatoprotective benefits through raising antioxidant potential, reducing inflammation & too much lipid production within liver cells. This is encouraging (H.-Y. Park et al., Citation2013).
Hesperidin has been demonstrated to ameliorate the morphological alterations generated by ethanol 350 mM over 32 hours in an alcoholic liver disease condition when administered at doses of 6.25, 12.5 and 25 g/ml for 24 hours. Hesperidin also reduces the expression of genes associated to alcohol & lipid metabolism, such as cyp2y3, cyp3a65, hmgcra, hmgcrb, fasn, and fads2, as well as genes related to endoplasmic reticulum stresses & DNA degradation, such as chop, gadd45 a, and edem1. Hesperidin exhibits its preventive benefits over alcoholic liver injury via plummeting endoplasmic reticulum stress, DNA damage, and alcohol & lipid metabolism (Zhou et al., Citation2017).
Hesperidin at dosages of 200 mg orally administrated for a duration of 7 weeks and diethylcarbamazine at dosages of 50 mg orally administered for phase of 7 weeks have been prescribed to mice in a composite therapy approach of research to assess their potential hepatoprotective activity against ethanol 1 ml/100 g/d two times a week for 7 weeks. The serum concentrations of AST, ALT, ALP, and GGT as well as MDA levels were markedly increased by ethanol. Additionally, it lowered GSH content while increasing hepatic nitric oxide and 4-hydroxyproline levels. Ethanol enhanced the levels of the inflammatory proteins interleukin (IL)-6 and transforming growth factor (TGF) in the blood as well as the expression of alpha smooth muscle actin (−SMA). These imbalances have been found to be normalized by a therapeutic strategy combining hesperidin and diethylcarbamazine. The fundamental processes for their hepatoprotective benefits involve raising the amount of antioxidants in the liver, which inhibits the activation of hepatic lobes cells & prevents nuclear factor kappa-lightchain promoter of activated B cells (NF-κB) from occurring (El et al., Citation2017).
Acetaldehyde seems to be a highly volatile intermediate metabolite of ethanol that has been linked to alcoholic liver disease and causes cell damage when produced in hepatocytes (Cheraghpour et al., Citation2019). HepG2 cells were treated to acetaldehyde (100 M) in an in vitro research setting. The findings showed that acetaldehyde induced cell invasion, matrix metalloproteinase-9 (MMP-9) production and excretion, and an elevation in NF-κB and activator protein 1 (AP-1) activity. The IκBα signaling pathway increased NF-κB translocation into the nucleus. Through the activation of the JNK & P38 kinase signalling pathways, AP-1 function was enhanced. Hesperidin (50, 100, and 200 M) administration of the cells resulted in an inhibition of NF-κB and AP-1 activity via the IκBα, JNK, and P38 signaling routes. Additionally, hesperidin reduced MMP-9 expression, emission, and cellular invasions, demonstrating its hepatoprotective properties (Yeh et al., Citation2009).
Acrylamide, among the most significant environmental pollutants, is frequently utilised to create polyacrylamides (Yeh et al., Citation2009). This substance has harmful consequences on a assortment of organs, counting hepatic, kidneys, and nervous system (LoPachin & Gavin, Citation2012). The blood levels of AST, ALT, and LDH, creatinine and urea, protein carbonyls, MDA concentration, and carcinoembryonic antigen are noticeably elevated following oral administration of acrylamide to mice for a duration of four months at a dosage of 15 mg/kg per day. Additionally, this has been astonishingly demonstrated that acrylamide lowers GSH levels, SOD, CAT, and GPx actions. Histopathologically, injection of acrylamide causes degrading changes in a number of hepatocytes, as well as nuclei & lymphocyte infiltration amongst hepatocytes & close to the central vein. Such findings led scientists to the conclusion that hesperidin’s antioxidant properties shield the liver from acrylamide poisoning by reversing all these abnormalities and reducing histological alterations (Hamdy et al., Citation2017).
A powerful liver toxin, carbon tetrachloride (CCl4) causes inflammation and apoptosis in laboratory animals (Yu et al., Citation2014). Two milliliters per kilogram of carbon tetrachloride given to mice causes a rise in AST and ALT activity as well as a huge diminution in liver GSH content, SOD activity, and GPx activity. However, studies have shown that oral treatment of hesperidin 10 and 100 mg/kg for 28 days prevents the hepatic through its antioxidant action, making it a unique therapeutic option towards hepatotoxicity (S.-Y. Chen et al., Citation2013). In a different animal paradigm, mice given hesperidin 100 and 200 mg/kg for 10 days after receiving a sole dosage of carbon tetrachloride-induced hepatotoxicity (2 ml/kg) showed a noteworthy dwindle in blood values of AST and ALT, TNF-, and MDA concentration. As confirmed by histopathological results, however, the reactivity of SOD and GSH concentration, as well as the overall antioxidant capacity, were noticeably raised. When taken as a whole, hesperidin has dose-dependent hepatoprotective benefits (Abdulaziz Ahmeedah Rabee & Bennasir, Citation2018; Halim et al., Citation2017; Çetin et al., Citation2016).
In a research using a combo of therapies, mice were given intraperitoneal injections of 1 ml/kg of carbon tetrachloride and olive oil for 2 days, which caused the initiation of hepatotoxicity as evidenced by increased AST and ALT levels in the blood and decreased GGT, GPx, and GSH activities. Along with alpha-fetoprotein (AFP), extrinsic tissue proteins, and inflammatory markers counting C-reactive protein and IL-6, it also boosted their levels. When administered orally at a dosage of 100 mg for 14 days, the hesperidin and diosmin combination designated as daflon has demonstrated the ability to protect liver failure via its antioxidant and anti-inflammatory potentials (Timoshin et al., Citation2005). Hesperidin and quercetin demonstrate significant antioxidant activity in rats in a different combination treatment by preventing the generation of nitric oxide radicals caused by carbon tetrachloride (Hassan et al., Citation2018).
A further study found that giving mice carbon tetrachloride at a dosage rate of 2 ml/kg for five weeks causes liver fibrosis. However, oral treatment of hesperidin (200 mg/kg) for 5 weeks causes a considerable decrease in the levels of TGF-1, hydroxyproline, AST, ALT, total bilirubin, MDA, myeloperoxidase, TNF-, LDL, and TG as well as a rise in the concentrations of albumin, GSH, and CAT. The researchers draw the conclusion that hesperidin’s antioxidant and anti-inflammatory qualities construct it a potentially effective treatment for liver fibrosis (Abd Elhakim et al., Citation2020; Abdel-Sttar et al., Citation2017).
4.6. Anti-cancer role of hesperidin
In comparison to untreated cells, hesperidin has been demonstrated to drastically lower the lifespan of pre-B cell line NAML-6 (leukaemia cells that develop into B cells). NAML-6 cells treated with hesperidin showed suppression of AKT, 1KBx, and GSK-3 activation as well as downregulation of IKK expression. Hesperidin’s use as a chemotherapy drug is justified by the inhibition of certain signalling pathways, such as the PI3K3/AKT or IKK signalling pathways (Shahbazi et al., Citation2018). Hesperitin, an aglycon of hesperidin, has considerable cytotoxic action in a concentration- and dose-dependent manner against human breast cancer carcinoma MCF-7 cells, immortalizing average healthy memory epithelial cells despite having no impact on average healthy memory epithelial cells (Madureira et al., Citation2023). Hesperitin exerts cytotoxic activity, which means that it increases the apoptotic activity as shown by the externalization of phosphatidyl-serine, initiation of caspase-7 followed by segmentation, and dissociation of PARP. Likewise, mechanisms linked to hesperidin’s apoptotic action include caspase-9 activation, loss of mitochondrial membrane potential, emission of cytochrome C, and an increase in Bax/Bcl-2 (Palit et al., Citation2015).
Hesperitin, reported to Zhang et al. (Citation2015), has the ability to increase intracellular ROS, ATP, and calcium levels, which activates the mitochondrial pathway and causes apoptosis to occur in hepatocellular carcinoma. Additionally, Hoechst 33,285 staining demonstrates that hesperitin raises the levels of cytosolic Apaf-1 and cytosolic cyt C, caspase-3, caspase-9, and death protein Bcl-2, as well as mitochondrial AIF, Apaf-1, and cyt C (Zhang et al., Citation2015).
A549 and Ncl-H358 cells treated with hesperidin exhibit decreased proliferation and increased apoptosis, as shown by caspase-3, which has a major impact on the signal transduction pathways of FGF and NF-kB (Birsu Cincin et al., Citation2015). Hesperidin inhibits proliferation in A549 cells by inducing cell cycle arrest at G0/G1 stage by upregulating P21 and P53 expression while down-regulating cyclin D1 expression. Hesperidin might thus be considered a possible treatment medication for NSCLC (Xia et al., Citation2018). Hesperidin raises levels of GADD 153/cHop and GRP78 inside Hela cells and causes cell apoptosis that is defined by the accumulation of nuclear and DNA fragmentation (hallmark of endoplasmic reticulum stress). Hesperidin also encourages production of reactive oxygen species, calcium mobilization inside of cells, and degradation of mitochondrial membrane capacity (Y. Wang et al., Citation2015).
Hesperidin is thought to have anticancer properties and may be used to treat gall bladder carcinoma. Hesperidin 200 micrometer treatment for 1 day dramatically inhibits cell growth in an in-vitro study by causing cell cycle arrest at the G2/M phase, the production of ROS, and the stimulation of caspase-3 (Pandey et al., Citation2019). A crucial molecule for promoting cell proliferation, calcium/calmodulin-dependent protein kinase IV (CAMKIV) is linked to the advancement of cancer. In human liver carcinoma HepG2 cells and neuroblastoma SH-SY5Y cancer cells, hesperidin has the ability to suppress CAMKIV. Via the activation of the caspase-3 dependent intrinsic mechanism and the over-expression of the proapoptotic Bax protein, hesperidin causes apoptosis and inhibits proliferation (Naz et al., Citation2019).
Hesperidin drastically affects the azoxymethane-mediated apoptotic situation in animal studies of colon cancer that have been administered with the drug by modifying the Bax/Bcl ratio along with elevated caspase-3,9 activations and improved cytochrome c releasing. Additionally, hesperidin treatment causes a decrease in p-Pbk and p-AKT facilitated by azoxymethane and an increase in tumour suppressor phosphatase & tensin homologue (PTEN). Likewise, the autophagic markers Beclin-1 and LC3-II are stimulated by hesperidin’s suppression of p-mTOR expression (Saiprasad et al., Citation2014). Hesperidin and fistein have demonstrated anti-cancer efficacy in the K562 CML cells, which is supported by their capacity to depolarize the mitochondrial membrane, boost caspase-3 activation, and induce cell cycle arrest at the G2/M and G0/G1 stages. According to the genetic test, hesperidin and fistein therapy affect the JAK/STAT pathway, KIT receptor signaling, and growth hormone receptor signaling, among other signaling pathways. Furthermore, they have a major impact on the expression of genes involved in cell growth, cell death, and cell cycle control (Adan & Baran, Citation2016).
Hesperidin pre-treatment induces caspase-3,9, Bax expression and inhibits the translations of Bcl-2, NF-KB, iNOS, TNF—α, and PCNA towards ferric nitrilotriacetate (Fe-NTA)-induced renal oxidative stress and carcinogenesis in wistar rats, according to (Siddiqi et al., Citation2015). Furthermore, by rebuilding renal shape, histological data support hesperidin’s chemoprotective effectiveness (Siddiqi et al., Citation2015). In human osteosarcoma MG-63 cells and xenografted mouse models, hesperidin has demonstrated anti-tumor actions both in vitro and in vivo by inhibiting cell motility and invasive, arresting the cell cycle in the G2/M phase, and inducing cell-mediated death (Du et al., Citation2018).
Rehabilitation with hesperidin, tamoxifen, and camel exosomes greatly lessens the proliferation of MCF-7. Simultaneous co-treatment provides considerably more potent anti-migratory, anti-invasive, and apoptotic properties. They suppress the EGFR and Er genes, which are linked to melanoma. Such a procedure lessens tamoxifen adverse effects (Mahmoud et al., Citation2017). By boosting the apoptotic process and cell cycle arrest, hesperidin has been found to decrease the survivability of cancer cells in the HEp-2 cell line. As a result, it could be utilized to address oral cancer or head and neck squamous cell carcinoma (HNSCC) (Fernández‐Bedmar et al., Citation2017). The in-vitro anticancer activity of hesperidin on human prostate cancer cells was described by (Zaghloul et al., Citation2017). Owing to the production of cell cycle arrest and necrosis, which is similar to apoptotic cell death, the therapy caused a drop in the development of cancer cells. It has been demonstrated that a decrease in MMP and intracellular buildup of ROS molecules cause necrosis. Hesperidin also prevents the motility and invasion of cancer cells (Zaghloul et al., Citation2017).
By inducing the production of programmed cell death ligand (PD-L1) through the dysregulation of AKT and NF-KB signaling in triple negative breast cancer, hesperidin therapy slows the proliferation of breast cancer cells, particularly in MDA-MB231, the triple negative breast adenocarcinoma cell line (TNBC). Hesperidin also inhibits the migration of MDA-MB231 cells (Kabała-Dzik et al., Citation2018; Siddiqi et al., Citation2018). Gamma radiation at 150 KGy has been shown in in vitro studies to have the ability to slow the development of melanoma B16BL6 cells and lung metastasis in C57BL/6 mice. The large population of double positive cells, enhanced sub-G1 phase cells, depolarization of matrix metalloproteinase, manufacturing of ROS, altered cell morphology, frailty of cell adhesion, and suppression of B16BL6 cell migration are evidence that gamma-irradiated 150 KGy hesperidin stimulates cytotoxicity by exerting apoptosis, according to the framework (He et al., Citation2017). Hesperidin’s ability to dramatically suppress angiopoietin 1 (an angiogenic promoter) secretion and enhance annexin-V (an apoptotic indicator) in Hep-2 Laryngeal cancer cells supports its anticancer function in the spread of laryngeal cancer cells (Kamaraj et al., Citation2010).
Hesperidin exposure to cancer and tumours of all sorts has good effects, and it may one day be utilised as a possible anticancer treatment, according to the results of the research stated above.
5. Role of hesperidin in neurodegenerative disorders
5.1. Parkinson’s disease
Depletion of dopaminergic neurons is a symptom of Parkinson’s disease, a form of neurological ailment. Destruction of dopaminergic neurons and the ongoing active participation of these functionalities lead to emergence of Parkinson’s disease non-motor symptoms like anxiety, emotions and cognitive deficits, sleeplessness, deficient smell, etc (Bal‐Price et al., Citation2002; Mottay & Neergheen-Bhujun, Citation2015; Rasool et al., Citation2014). Oxidative stress is more notable sign in pathophysiology of Reactive oxygen species (ROS) buildup is linked to this kind of neurological illness. The participation in the control of antioxidant enzyme concentrations may provide an explanation for the suppressive strategies for reducing free radical production & oxidative stress (Girdhar et al., Citation2015). The oxidative metabolism of cytosolic dopamine (DA) through association with monoamine oxidase (MAO) enzyme plays a significant position in course of Parkinson’s disease (PD), in complement to oxidative stress (Bansal et al., Citation2023; Kivrak et al., Citation2009).
Through antioxidant and cytosolic dopamine-enhancing pathways, hesperidin, a powerful antioxidant and bio membrane stabiliser, can have a number of preventive benefits in Parkinson’s disease animals. Additionally, hesperidin has demonstrated modulatory effect on serotonergic 5-HT1A receptors and kappa-opioid (−opioid) receptors that diminishes signs of anxiety (Antunes et al., Citation2014; Souza et al., Citation2013). Hesperidin was said to be useful in reducing cognition and behavioral deficiencies because of its capacity to alter neurotransmitter networks. Additionally, by regulating GSH levels, GPx and CAT operations, preventing ROS establishment, and dampening GR action, it may have prevented the exhaustion of dopamine and its metabolites 3,4-dihydroxyphenylacetic acid (DOPAC) and homovanillic acid (HVA), as well as its exhaustion of antioxidant activity (Antunes et al., Citation2014).
Likewise, in Chlorpyrifos-induced designs of Parkinson’s disease, hesperidin improves motion control effectiveness, markedly reduces lipid peroxidation (by lowering malondialdehyde (MDA) message), attenuates hypercholesterolemia (by lowering total cholesterol and triacylglycerols in circulating levels), and improves DNA destruction (by lowering levels of 8 hydroxydeoxyguanosine (8-OHdG)) (Salem et al., Citation2012). Hesperidin’s neuroprotective effects in Parkinson’s disease may possibly be a result of its capacity to control many neurotransmitters, including norepinephrine, serotonin, and epinephrine (Nagappan & Krishnamurthy). By reducing the concentrations of pro-inflammatory cytokines such TNF-, IL-1, IL-6, IL-4, and IL-10 and also glial fibrillary acidic protein (GFAP), iNOS, and COX-2, hesperidin intake could also be useful for Parkinson’s disease animals (Tamilselvam et al., Citation2013).
Hesperidin has been discovered to be far more successful than that of the L-Dopa medication currently being used to cure Parkinson’s disease. Hesperidin is more bio-available when combined with L-Dopa in 6-OHDA rat paradigm of Parkinson’s disease, and it also slows the striatal and midbrain degeneration of cytoplasmic vacuolation driven by 6-hydroxydopamine (6-OHDA) (Hajialyani et al., Citation2019). Synuclein alpha (SNCA), a widely espoused protein that affects the instruction of dopamine discharge, and Leucine-rich repeat kinase 2 (LRRK2), an enzyme with kinase and GTPase function, whose mutation is by far most common heritable component of Parkinson’s disease, are both suppressed by the synergistic interplay of these two Parkinson’s disease medications. Parkin, a protein implicated in the pathophysiology of autosomal recessive juvenile Parkinsonism (AR-JP), and PTEN-induced putative kinase-1, which phosphorylates parkin, were also potentiated by such an interaction (Santos et al., Citation2016). Hesperidin and Sinemet, among the most widely used treatments for Parkinson’s disease, work together to lessen Sinemet’s negative effects in Parkinson’s disease animals brought on by Chlorpyrifos (Salem et al., Citation2012).
5.2. Alzheimer’s disease & dementia
Alzheimer’s disease is a CNS neurodegenerative condition that causes loss of cognitive and gradual intellectual deterioration. Dementia is thought to be most commonly caused by AD (Jiménez-Aliaga et al., Citation2011). Unfortunately, in spite of reality that it is generally known that the pathophysiology of AD is intimately linked to cholinergic impairment, mitochondrial irregularities, and oxidative stress, there really is no known cause for the disease (Qin et al., Citation2012). Hesperidin might be thought of as a possible management for Alzheimer’s disease and dementia because of its high memory-improving and antioxidant properties.
Another of the methods through which hesperidin enhances cognitive performance may be its capacity to increase anti-oxidative defense. Hesperidin supplementation for 16 weeks increased recognition index in the APPswe/PS1dE9 mice model, which aided to promote neurobehavioral performance. It reduced MDA and H2O2 concentrations, and also restored depleted GSH levels and overall antioxidant capability; to rectify the Aβ induced mitochondrial defects (T-AOC). Through increasing the activity of the mitochondrial complex I-IV enzymes, the mitochondrial enzyme actions were likewise recovered (D. Wang et al., Citation2014). Glycogen synthase kinase-3 (GSK-3) is a protein kinase with a significant role in AD and mitochondrial processes. Tau protein hyperphosphorylation and the mitochondrial intention are both significantly impacted. The amount of oxidative destruction increases when this protein kinase is activated (Badalzadeh et al., Citation2015).
Through preventing the repair of such a enzyme, hesperidin displayed a mitochondrial neuroprotective activity and may have recovered cognitive impairments. Hesperidin’s potential method for lowering Aβ1–40 levels was this (DaRocha-Souto et al., Citation2012; D. Wang et al., Citation2014). Hesperidin, which works as an AChE inhibitor, also prevented memory and cognitive deficits brought on by AlCl3-induced AD. In hippocampus & cortex of rat brain, hesperidin reduced the production of the amyloid precursor protein (APP) through an NF-κB-dependent route & lowered concentrations of Aβ1–40, β-, and γ-secretases (that both regulate the breakdown of APP) . Hesperidin’s impact on memory decline in mice with AD brought on by AlCl3 was examined in a different investigation. It was discovered that AD might improve cognitive impairment. Translations were included into neuroprotective process of hesperidin by up—regulation B-cell lymphoma 2 (Bcl2) and inhibits Bcl2-associated X proteins (Bax) (Justin Thenmozhi et al., Citation2017).
In accumulation to cognitive deficiencies, AD is linked to behavioral and non-cognitive problems. The causes of behavioral and cognitive symptoms may include A accumulation, accumulation, and neuroinflammation. Hesperidin was proven to be beneficial in reversing behavioral manifestations linked to AD in additional to intellectual problems. Hesperidin prevented inflammation, restored APP synthesis and A-peptide accumulation inside cortex & hippocampus of animals, & subsequently improved transgenic mouse’s capability to nest and communicate with one another in a research using the APP/PS1 rat model of AD (C. Li et al., Citation2015).
Hesperidin’s ability to reduce levels of converting growth factor β1 (TGF-β1) immune-reactivity and NF-κB, both of which are recognized to contribute to the advancement of Alzheimer’s disease (AD), was shown to be the technique by which this herbaceous chemical reduced inflammation (Ghorbani et al., Citation2012). Involvement of TGF-β1 in promoting synthesis of APP and the depositing of Aβ peptides was previously established by Bansal (Bansal et al., Citation2023).
Additionally, cognition, intelligence, and motor skills gradually deteriorate as a result of dementia. Hesperidin’s neuroprotective impact on the intracerebroventricular streptozotocin (ICV-STZ)-induced animal paradigm of sporadic dementia of the Alzheimer’s type (SDAT) was confirmed by examining how well it affected cholinergic disruptions, cognition, and spatial learning. Hesperidin has been exposed to diminish escape delay and perk up remembrance retention in dose-dependent ways in the Morris water maze escape and probing trials. Hesperidin showed a potential effect in reducing AChE activity and lipid peroxidation (by reducing amount of thiobarbituric acid reactive substances (TBARS)), enhancing ganglioside levels, and preventing the inflammatory reaction (by inhibiting NF-κB, COX-2, and iNOS) following treatment (Javed et al., Citation2015).
Additionally, within mouse model of l-methionine-induced hyperhomocysteinemia (HHcy), hesperidin showed a preventive role against vascular dementia (VD). Hesperidin alleviated HHcy-mediated endothelial impairment in additional to cognitive decline through a dose-dependent mechanism that saw nitrite and serum Hcy levels with MDA levels fall, AChE activity be inhibited, and GSH, SOD, and CAT concentrations rise (Hemanth Kumar et al., Citation2017; B. H. Kumar et al., Citation2017). Similar outcomes were also obtained when hesperidin and donepezil were administered together in the scopolamine-induced amnesia paradigm; supporting significant position that hesperidin plays in reducing dementia & intellectual impairments in AD (Habibyar et al., Citation2016).
5.3. Huntington’s disease
Huntington’s disease is a neurologic condition that progresses and eventually kills its victims. It is characterized by a spacious assortment of malformations, counting dementia, uncontrollable shaking, impaired motor, intellectual and cognition indications, personality modifications, and expressions of cognition and memory (H. Rosas et al., Citation2002; H. D. Rosas et al., Citation2010). 3-Nitropropionic acid (3-NP), a recognized and well-established phenotypic HD activator both in animal and human research, was utilized to examine HD neuro-psychiatric signs. In the striatum, 3-NP poisoning was followed by oxidative stress, body weight loss, & abnormalities of the grip, locomotion, and mitochondria. Resolving these limitations and improving locomotors and grip power are necessary components of hesperidin therapy. It was discovered that hesperidin’s modulatory action on the nitric oxide pathway was responsible for the substance’s augmentation of striatal oxidative defense & its impact on intracellular energy storage (P. Kumar & Kumar, Citation2010).
Researchers evaluated expressions of iNOS before & after hesperidin exposure to then-3-NP-intoxicated animal models of HD to confirm the potential effect of hesperidin in dampening nitric oxide synthase production. Hesperidin’s substantial impact in reducing iNOSlevels in the cortical, striatal, and hippocampus areas (p 0.05) supported its nitric oxide linked method of action on HD animals (Menze et al., Citation2012). Hesperidin’s participation in the lowering of MDA levels, the augmentation of CAT activity, and the avoidance of prepulse inhibition (PPI) of the startle reaction offered compelling evidence that it served a positive impact in the rehabilitation of HD in complement to these benefits (Menze et al., Citation2012).
Preventative action of hesperidin on HD was discovered to probably entail a microglial circuit, which is intriguing (Carlos Filho et al., Citation2013). In rat versions of HD driven by quinolinic acid (QA), co-treatment of minocycline (like a microglial blocker) and hesperidin dramatically increased the impact of hesperidin on neurotoxic effects brought on by QA (Coull et al. (Citation2005). Minocycline and hesperidin prevented the QA-mediated apoptosis (raised amount of caspase-3 activity), diminution of brain-derived neurotrophic factor (BDNF, a signalling molecule released through activated microglia that aids in supporting lifespan of neurons concentration), and augmentation of TNF-α level. These findings collectively imply that hesperidin’s neuroprotective benefits towards HD may be due to its inhibition of microglial cell stimulation and participation in the microglial circuit (A. Kumar et al., Citation2013).
5.4. Multiple sclerosis
Primary etiology of neurological dysfunction is multiple sclerosis, a chronic and complicated neuro-inflammatory demyelinating disorder of CNS (Ontaneda et al., Citation2017). Along with the release of inflammatory cytokines, this kind of neuro-inflammatory illness is frequently characterized with axonal degeneration and glial scarring (Farzaei et al., Citation2017). Incursion and enlargement of CD4+ T-cells, the infiltration of T-cells and macrophages, and the generation of NO in the cerebral spinal fluid (CSF) are all factors in the pathophysiology of these specific CNS illnesses (Minagar et al., Citation2004; Muili et al., Citation2012).
Anti-inflammatory properties of flavonoids, such as hesperidin, as well as associated suppressive effects on pro-inflammatory cytokines, along with their capacity to reduce T-cell proliferation, offer flavonoids an effective treatment for MS. Hesperidin improved the clinical aberrations and reduced demyelination in the CNS in myelin oligodendrocyte glycoprotein (MOG)-induced C57BL/6 mouse variant of MS. Pro-inflammatory cytokines like IL-6, IL-17, IL-23, TNF-α, and Th17 cells transcription factor are excreted, while Treg-related cytokines like IL-10 and TGF-β are decreased, along with transcription factor FoxP3 (Haghmorad et al., Citation2017).
MS models also showed lipid per-oxidation (higher TBARS level) & inhibition of enzymatic and non-enzymatic antioxidants in addition to the above mentioned aberrations. Exploratory allergic encephalomyelitis (EAE) was discovered to generate oxidative destruction and histological abnormalities in the cerebral cortex, which were both restored by hesperidin therapy (Ciftci et al., Citation2015). Hesperidin’s ability to inhibit caspase3-like immune-reactivity on the neurons of a C57BL/J6 mice model further supported its anti-apoptotic effects (Ciftci et al., Citation2015).
6. Role of hesperidin against corona virus
Hesperidin’s physiochemical composition makes it particularly advantageous and suited to connect with important proteins throughout the development of the severe acute respiratory syndrome corona virus 2 (SARS Covid-2) (Joshi et al., Citation2021). The capacity of a molecule, biological or manufactured, to connect with less bonding power has been assessed by investigators who have been concentrating on the thorough understanding of the organization of the protein of viruses. This is because attaching is even more powerful and precise if the necessary energy is reduced. A method known as “in silico” is at present utilized to predict pharmacological behavior & increase classification performance. This method enables the assessment of various medications while reducing the need for costly research activities (C. Wu et al., Citation2020).
About 1060 natural compounds have been studied by several scientists for their prospective antiviral effects, and 77 antiviral medications were examined for their capacity to interact with the SARS Covid-2. Hesperidin has been shown to be the much more advantageous of all owing to its superior knack to connect with spike. Hesperidin might impede with the association of ACE-2 with receptor binding domain because it overlaps with angiotensin converting enzyme-2 interference that superimposes the complex of ACE-2 and receptor binding domain on hesperidin (Dhingra et al., Citation2023).
Additionally, it is hypothesized that hesperidin be able to unite to SARS-Covid 2 protease at a second low-energy location, enabling the conversion of the viral genome proteins PP1a and PPa1ab into functional proteins inside host cells (C. Wu et al., Citation2020). This enzyme is also known as “3Clpro” or “Mpro” by different authors. Numerous chemical antiviral medications have it as their focus. In an evaluation of 1500 possible compounds which possess the ability to interact with the 3CLPro, hesperidin has been shown to be the 2nd highest effective for the attachment to chain A, according to numerous researchers. Hesperidin has a lower free energy at −10.1 Kcal/mol than the benchmark medications lopinavir (−8) and ritonavir (−7.9). Hesperidin may likewise effortlessly attach to chain B with − 8.3 Kcal. Despite having a lesser binding ability than ritonavir (−6.9) and lopinavir (−6.9) (C. Wu et al., Citation2020).
Das et al., (Citation2021) demonstrate how hesperidin and MPro cooperate. The binding energies of naturally occurring and commercially accessible antiviral compounds were compared. Hesperidin is highly affine to THR24, THR25, HIS4, SER46, and CYS145 with hydrogen bonds, among various amino acids. Further study provides more proof that hesperidin is one of the natural elements with aptitude to interrelate with the primary protease of SAR Covid-2 as well as viral receptor angiotensin converting enzyme-2. Hesperidin binds to SAR Covid-2 proteins with an affinity of −9.02 Kcal/mol (Das et al., Citation2021).
There are several publications from various parts of the globe that address the fundamentals of the ability of molecules to attach with viral proteins. Alongside these, important therapeutic plants are Curcuma sp., citrus sp., caesalpiniasappen, and alpinia galangal. Hesperidin has coupling sites with the SAR Covid-2 protease, glycoprotein-RBD spike, & angiotensin conversion enzyme-2 receptor that are sequentially − 13.51, −9.61, and − 9.50, making it a much more potent and powerful interacting molecule with all three of the key viral proteins (Utomo et al., Citation2020).
Hesperidin exhibits a greater capacity to interface with the SAR Covid-2 protease when contrasted to lopinovir (a baseline medication utilized in the clinical studies of covid-19). Due to their comparatively modest binding energies to the three crucial proteins, further orange flavonoids including tangerine, naringenin, and nobiletine may also participate to the inhibitory impact on viral illness. Parallel to this, 26 organic polyphenol compounds were evaluated for their capacity to interact with the crystalline form of the primary protease of SAR Covid-2 in another molecular docking study (Adem et al., Citation2020). With the maximum binding capability, hesperidin was shown to be the greatest of everyone. Hesperidin’s association with the flavanone, which binds with the hydrogen bonds of the protein’s amino acids, is extremely efficacious when contrasted to the benchmark medicine nelfinavir (Adem et al., Citation2020).
Hesperitin and hemodyne have the maximum potential to impede the proteolytic action in a dose-dependent manner out of the seven phenolic substances evaluated, with IC50 values of 365 micromol/liter and 8.2 micromol/liter, correspondingly, as per (Lin et al., Citation2005), an analysis wherein the ability of bioactive components to embody the 3CLPro of the SARS virus is analysed. Moreover, cutting-edge investigation has shown that hesperidin’s ability to block viral protease grows in direct proportion to its plasma levels and vice-versa. Orange juice is an effective way to consume this amount of hesperidin. Consequently, it may be inferred that hesperidin’s inhibitory action may be used to leverage SARS-Covid 2 (Adem et al., Citation2020).
Viral diseases disturb the stability of oxidative interactions, resulting in oxidative stress that ultimately enables the phases of life cycle of SAR-COV-2 (J. Wu, Citation2020) and ultimately results in apoptosis. This is owing to interference of enzymatic framework and various phagocytic compounds that are indispensable for operating of cells at every tissue level. Hesperidin greatly contributes to the antioxidant defense mechanisms and is a powerful reagent versus superoxide and hydroxyl radicals (H.-K. Park et al., Citation2019). A hesperidin precursor called hesperetin prevents LPS-stimulated microglial cells from producing nitric oxide (Jo et al., Citation2019).
Systemic disturbances brought on by extensive COVID-19 disease infections lead to deregulation of iron homeostasis, free heme discharge, and hyperferritinemia. Such circumstances cause an overwhelming production of reactive oxygen species, including the hydroxyl radical (OH), superoxide anion (O2-), and hydrogen peroxide (H2O2) (Cavezzi et al., Citation2020; Wagener et al., Citation2020). The failure of mitochondrial oxidative metabolism causes platelet destruction and the development of thrombus formation throughout the pathogenic phase (Saleh et al., Citation2020). Following Covid-19, free oxygen radicals are produced similarly to how they are produced during ischemia second reperfusion (I/R) (Tian & Ye, Citation2020). They can quicken the healing process by using medications like chloroquine (Mubagwa, Citation2020). Long-term tissue hypoxia has been shown to cause structural failure, particularly in organelles like the mitochondria and endoplasmic reticulum. The biological mechanism, specifically while oxygen delivered via blood comes to identical tissue, causes a monovalent elimination of oxygen, leading to creation of superoxide & other chain radicals. Hesperidin may have a rehabilitative impact on injuries caused by liver ischemia-reperfusion in rats that have experienced this condition repeatedly (H.-K. Park et al., Citation2019).
Even with an enzyme called NADPH Oxidase, phagocytic cells including neutrophils, eosinophils, and basophils create large amounts of hazardous oxygen derivatives with the ability to kill microbes. These actions all take place when the inflammation is happening (Al-Rikabi et al., Citation2020). On occasion, the extracellular surrounding is exposed to hazardous oxygen as a result of increased synthesis and concentration of free radical oxygen. As an outcome, there are negative repercussions and an aggravation of the damage (Manea, Citation2010; Puertollano et al., Citation2011). The intracellular redox state is the target of contemporary natural or synthetic medications. These cutting-edge medicines will combat viral reproduction and virus-induced inflammation (J. Wu, Citation2020). Recent research recommends supplementing with antioxidants such N-acetylcysteine, melatonin, polyphenols, and fat-specific vitamins K, C, D, and E throughout the initial stages of COVID-19 therapy (Beigmohammadi et al., Citation2020).
Hesperidin exhibits strong antioxidant action, which implies that COVID-19 might be treated with it. However, there haven’t been many substantial studies looking at how hesperidin directly affects Covid-19. Nevertheless, it can be ingested to advantage from its antioxidant properties since it aids in the body’s defense mechanism against cytotoxic damage brought on by the virus. Hesperidin is nevertheless entailed in free radical scavenging activity (antioxidant activities), but also strengthens cellular defenses alongside oxidative stress & aids in decline of inflammatory indicators through the ERK/NrfZ signaling route, according to countless novel research findings conducted both in vivo and in vitro (Roohbakhsh et al., Citation2015). By lowering the quantity of reactive oxygen species and stimulating the Nrfz signaling route, hesperidin reduces oxidative stress & apoptosis in cisplatin-treated HK-2 cells. The Nrfz route subsequently controls the oxidative response components (X. Chen et al., Citation2019). When administered in a dose-dependent way, hesperitin can reduce the liver’s oxidative stress caused by an excess of paracetamol (a popular antipyretic and analgesic medicine) (Wan et al., Citation2020).
Older individuals may benefit much from hesperidin since they experience higher oxidative stress. According to research, the severity of the COVID-19 outbreak varies depending on the patient’s age. But it’s not apparent what the process entails. Due to their high antioxidant capability contrasted to older people with low antioxidant potential, it is hypothesized that children have reduced severe infection risks (Delgado-Roche & Mesta, Citation2020; Keles, Citation2020). The expression of angiotensin conversion enzyme 2 (Dalan et al., Citation2020; Hati & Bhattacharyya, Citation2020) and the delivery of antigens are both affected by the intracellular redox environment (Trujillo et al., Citation2014). Age-related reductions of catalase, superoxide dismutase, and glutathione reductase in the liver of old mice are prevented by citrus flavors such naringin and hesperidin (Miler et al., Citation2016). Following an intense training session, hesperidin reduces the release of cytokines from activated macrophages and exhibits antioxidant potential in mice (Estruel-Amades et al., Citation2019). Effective hesperidin administration has been demonstrated to drastically lower levels of myeloperoxidase, malondialdehyde (a hallmark of lipid per-oxidation), and inflammation in animal models of colitis (Polat et al., Citation2018) and liver damage (Duran & Karaboğa, Citation2020). Additionally, hesperidin has significantly corrected the signs, sensations, lipid per-oxidation, and inflammatory indicators in an investigative type of arthritis (Ahmed et al., Citation2018).
7. Conclusion
Citrus fruit has rich nutritional profile and is loaded with promising bioactive compounds of nutraceutical importance and hesperidin is one of them. Hesperidin and its derivatives have strong anti-oxidant and anti-inflammatory potential against wide range of lifestyle linked metabolic syndromes. This review has gathered updated data regarding the efficacy of these compounds in major metabolic pathways involved in hindering the disease proliferation, that can play important role in the epidemiological studies of these diseases. However, this area has immense potential in exploring these bioactive compounds for other novel applications beneficial for human welfare. Therefore, novel scientific interventions are required to extend the scope of these phytochemicals.
Disclosure statement
No potential conflict of interest was reported by the authors.
Data availability statement
The authors confirm that the data supporting the findings of this study are available within the article
References
- Abd Elhakim, Y. M., Ghoneim, M. H., Khairy, M. H., & Eissa, S. A. (2020). Single or combined protective and therapeutic impact of taurine and hesperidin on carbon tetrachloride-induced acute hepatic injury in rat. Environmental Science and Pollution Research, 27(12), 13180–30. https://doi.org/10.1007/s11356-020-07895-1
- Abdel-Sttar, A. R., Khalaf, M. M., Aboyoussef, A. M., & Abosaif, A. (2017). Ameliorative effect of hesperidin on carbon tetrachloride induced liver fibrosis in rats. International Journal of Pharmacy and Pharmaceutical Sciences, 9(7), 45–51. https://doi.org/10.22159/ijpps.2017v9i7.17611
- Abdulaziz Ahmeedah Rabee, A., & Bennasir, H. (2018). Hesperidin an antioxidant flavonoid prevents carbon tetrachloride-induced hepatic toxicity in male albino rats. JIPBS, 5, 127–132. https://jipbs.com/index.php/journal/article/view/350
- Adan, A., & Baran, Y. (2016). Fisetin and hesperetin induced apoptosis and cell cycle arrest in chronic myeloid leukemia cells accompanied by modulation of cellular signaling. Tumor Biology, 37(5), 5781–5795. https://doi.org/10.1007/s13277-015-4118-3
- Adem, S., Eyupoglu, V., Sarfraz, I., Rasul, A., & Ali, M. (2020). Identification of potent COVID-19 main protease (Mpro) inhibitors from natural polyphenols: An in silico strategy unveils a hope against CORONA.
- Ahmed, O., Fahim, H., Mahmoud, A., & Ahmed, E. A. E. (2018). Bee venom and hesperidin effectively mitigate complete Freund’s adjuvant-induced arthritis via immunomodulation and enhancement of antioxidant defense system. Archives of Rheumatology, 33(2), 198. https://doi.org/10.5606/ArchRheumatol.2018.6519
- Ajuwon, O. R., Oguntibeju, O. O., & Marnewick, J. L. (2014). Amelioration of lipopolysaccharide-induced liver injury by aqueous rooibos (Aspalathus linearis) extract via inhibition of pro-inflammatory cytokines and oxidative stress. BMC Complementary and Alternative Medicine, 14(1), 1–12. https://doi.org/10.1186/1472-6882-14-392
- Akiyama, S., Katsumata, S.-I., Suzuki, K., Ishimi, Y., Wu, J., & Uehara, M. (2009). Dietary hesperidin exerts hypoglycemic and hypolipidemic effects in streptozotocin-induced marginal type 1 diabetic rats. Journal of Clinical Biochemistry and Nutrition, 46(1), 87–92. https://doi.org/10.3164/jcbn.09-82
- Al-Rikabi, R., Al-Shmgani, H., Dewir, Y. H., & El-Hendawy, S. (2020). In Vivo and in vitro evaluation of the protective effects of hesperidin in lipopolysaccharide-induced inflammation and cytotoxicity of cell. Molecules, 25(3), 478. https://doi.org/10.3390/molecules25030478
- Amaretti, A., Raimondi, S., Leonardi, A., Quartieri, A., & Rossi, M. (2015). Hydrolysis of the rutinose-conjugates flavonoids rutin and hesperidin by the gut microbiota and bifidobacteria. Nutrients, 7(4), 2788–2800. https://doi.org/10.3390/nu7042788
- Amiot, M., Riva, C., & Vinet, A. (2016). Effects of dietary polyphenols on metabolic syndrome features in humans: A systematic review. Obesity Reviews, 17(7), 573–586. https://doi.org/10.1111/obr.12409
- Antunes, M. S., Goes, A. T., Boeira, S. P., Prigol, M., & Jesse, C. R. (2014). Protective effect of hesperidin in a model of Parkinson’s disease induced by 6-hydroxydopamine in aged mice. Nutrition, 30(11–12), 1415–1422. https://doi.org/10.1016/j.nut.2014.03.024
- Aranaz, P., Navarro-Herrera, D., Zabala, M., Miguéliz, I., Romo-Hualde, A., López-Yoldi, M., Martínez, J., Vizmanos, J., Milagro, F., & González-Navarro, C. (2019). Phenolic compounds inhibit 3T3-L1 adipogenesis depending on the stage of differentiation and their binding affinity to PPARγ. Molecules, 24(6), 1045. https://doi.org/10.3390/molecules24061045
- Aschoff, J. K., Kaufmann, S., Kalkan, O., Neidhart, S., Carle, R., & Schweiggert, R. M. (2015). In vitro bioaccessibility of carotenoids, flavonoids, and vitamin C from differently processed oranges and orange juices [Citrus sinensis (L.) Osbeck]. Journal of Agricultural and Food Chemistry, 63(2), 578–587. https://doi.org/10.1021/jf505297t
- Aschoff, J. K., Riedl, K. M., Cooperstone, J. L., Högel, J., Bosy‐Westphal, A., Schwartz, S. J., Carle, R., & Schweiggert, R. M. (2016). Urinary excretion of citrus flavanones and their major catabolites after consumption of fresh oranges and pasteurized orange juice: A randomized cross‐over study. Molecular Nutrition & Food Research, 60(12), 2602–2610. https://doi.org/10.1002/mnfr.201600315
- Assini, J. M., Mulvihill, E. E., & Huff, M. W. (2013). Citrus flavonoids and lipid metabolism. Current Opinion in Lipidology, 24(1), 34–40. https://doi.org/10.1097/MOL.0b013e32835c07fd
- Badalzadeh, R., Mohammadi, M., Yousefi, B., Farajnia, S., Najafi, M., & Mohammadi, S. (2015). Involvement of glycogen synthase kinase-3β and oxidation status in the loss of cardioprotection by postconditioning in chronic diabetic male rats. Advanced Pharmaceutical Bulletin, 5(3), 321. https://doi.org/10.15171/apb.2015.045
- Bakhautdin, B., Das, D., Mandal, P., Roychowdhury, S., Danner, J., Bush, K., Pollard, K., Kaspar, J. W., Li, W., Salomon, R. G., McMullen, M. R., & Nagy, L. E. (2014). Protective role of HO-1 and carbon monoxide in ethanol-induced hepatocyte cell death and liver injury in mice. Journal of Hepatology, 61(5), 1029–1037. https://doi.org/10.1016/j.jhep.2014.06.007
- Bal‐Price, A., Matthias, A., & Brown, G. C. (2002). Stimulation of the NADPH oxidase in activated rat microglia removes nitric oxide but induces peroxynitrite production. Journal of Neurochemistry, 80(1), 73–80. https://doi.org/10.1046/j.0022-3042.2001.00675.x
- Bansal, K., Singh, V., Singh, S., & Mishra, S. (2023 Mar 20). Neuroprotective potential of hesperidin as therapeutic agent in the treatment of brain disorders: Preclinical evidence-based review. Current Molecular Medicine, 23, https://doi.org/10.2174/1566524023666230320144722
- Barreca, D., Gattuso, G., Bellocco, E., Calderaro, A., Trombetta, D., Smeriglio, A., Laganà, G., Daglia, M., Meneghini, S., & Nabavi, S. M. (2017). Flavanones: Citrus phytochemical with health‐promoting properties. BioFactors, 43(4), 495–506. https://doi.org/10.1002/biof.1363
- Beigmohammadi, M. T., Bitarafan, S., Hoseindokht, A., Abdollahi, A., Amoozadeh, L., Mahmoodi Ali Abadi, M., & Foroumandi, M. (2020). Impact of vitamins A, B, C, D, and E supplementation on improvement and mortality rate in ICU patients with coronavirus-19: A structured summary of a study protocol for a randomized controlled trial. Trials, 21(1), 1–4. https://doi.org/10.1186/s13063-020-04547-0
- Birsu Cincin, Z., Unlu, M., Kiran, B., Sinem Bireller, E., Baran, Y., & Cakmakoglu, B. (2015). Anti-proliferative, apoptotic and signal transduction effects of hesperidin in non-small cell lung cancer cells. Cellular Oncology, 38(3), 195–204. https://doi.org/10.1007/s13402-015-0222-z
- Borges, G., Lean, M. E., Roberts, S. A., & Crozier, A. (2013). Bioavailability of dietary (poly) phenols: A study with ileostomists to discriminate between absorption in small and large intestine. Food & Function, 4(5), 754–762. https://doi.org/10.1039/c3fo60024f
- Brand, W., Shao, J., Hoek Van Den Hil, E. F., Van Elk, K. N., Spenkelink, B., De Haan, L. H., Rein, M. J., Dionisi, F., Williamson, G., Van Bladeren, P. J., & Rietjens, I. M. C. M. (2010). Stereoselective conjugation, transport and bioactivity of S - and R -hesperetin enantiomers in vitro. Journal of Agricultural and Food Chemistry, 58(10), 6119–6125. https://doi.org/10.1021/jf1008617
- Brett, G. M., Hollands, W., Needs, P. W., Teucher, B., Dainty, J. R., Davis, B. D., Brodbelt, J. S., & Kroon, P. A. (2008). Absorption, metabolism and excretion of flavanones from single portions of orange fruit and juice and effects of anthropometric variables and contraceptive pill use on flavanone excretion. British Journal of Nutrition, 101(5), 664–675. https://doi.org/10.1017/S000711450803081X
- Carlos Filho, B., Del Fabbro, L., de Gomes, M. G., Goes, A. T., Souza, L. C., Boeira, S. P., & Jesse, C. R. (2013). Kappa-opioid receptors mediate the antidepressant-like activity of hesperidin in the mouse forced swimming test. European Journal of Pharmacology, 698(1–3), 286–291. https://doi.org/10.1016/j.ejphar.2012.11.003
- Cavezzi, A., Troiani, E., & Corrao, S. (2020). COVID-19: Hemoglobin, iron, and hypoxia beyond inflammation. A narrative review. Clinics and Practice, 10(2), 1271. https://doi.org/10.4081/cp.2020.1271
- Çetin, A., Çiftçi, O., & Otlu, A. (2016). Protective effect of hesperidin on oxidative and histological liver damage following carbon tetrachloride administration in Wistar rats. Archives of Medical Science, 12(3), 486–493. https://doi.org/10.5114/aoms.2015.49484
- Chagwedera, D. N., Ang, Q. Y., Bisanz, J. E., Leong, Y. A., Ganeshan, K., Cai, J., Patterson, A. D., Turnbaugh, P. J., & Chawla, A. (2019). Nutrient sensing in CD11c cells alters the gut microbiota to regulate food intake and body mass. Cell Metabolism, 30(2), 364–373.e7. https://doi.org/10.1016/j.cmet.2019.05.002
- Chambers, C. S., Biedermann, D., Valentová, K., Petrásková, L., Viktorová, J., Kuzma, M., & Křen, V. (2019). Preparation of retinoyl-flavonolignan hybrids and their antioxidant properties. Antioxidants, 8(7), 236. https://doi.org/10.3390/antiox8070236
- Chen, S.-Y., Chyau, C.-C., Chu, C.-C., Chen, Y.-H., Chen, T.-H., & Duh, P.-D. (2013). Hepatoprotection using sweet orange peel and its bioactive compound, hesperidin, for CCl4-induced liver injury in vivo. Journal of Functional Foods, 5(4), 1591–1600. https://doi.org/10.1016/j.jff.2013.07.001
- Chen, X., Wei, W., Li, Y., Huang, J., & Ci, X. (2019). Hesperetin relieves cisplatin-induced acute kidney injury by mitigating oxidative stress, inflammation and apoptosis. Chemico-Biological Interactions, 308, 269–278. https://doi.org/10.1016/j.cbi.2019.05.040
- Cheraghpour, M., Imani, H., Ommi, S., Alavian, S. M., Karimi-Shahrbabak, E., Hedayati, M., Yari, Z., & Hekmatdoost, A. (2019, August). Hesperidin improves hepatic steatosis, hepatic enzymes, and metabolic and inflammatory parameters in patients with nonalcoholic fatty liver disease: A randomized, placebo-controlled, double-blind clinical trial. Phytotherapy Research: PTR, 33(8), 2118–2125. https://doi.org/10.1002/ptr.6406
- Ciftci, O., Ozcan, C., Kamisli, O., Cetin, A., Basak, N., & Aytac, B. (2015). Hesperidin, a citrus flavonoid, has the ameliorative effects against experimental autoimmune encephalomyelitis (EAE) in a C57BL/J6 mouse model. Neurochemical Research, 40(6), 1111–1120. https://doi.org/10.1007/s11064-015-1571-8
- Colleluori, G., Aguirre, L., Phadnis, U., Fowler, K., Armamento-Villareal, R., Sun, Z., Brunetti, L., Park, J. H., Kaipparettu, B. A., Putluri, N., Auetumrongsawat, V., Yarasheski, K., Qualls, C., & Villareal, D. T. (2019). Aerobic plus resistance exercise in obese older adults improves muscle protein synthesis and preserves myocellular quality despite weight loss. Cell Metabolism, 30(2), 261–273. e266. https://doi.org/10.1016/j.cmet.2019.06.008
- Constantin, R. P., Nascimento, G. S. D., Constantin, R. P., Salgueiro, C. L., Bracht, A., Ishii-Iwamoto, E. L., Yamamoto, N. S., & Constantin, J. (2013). Citrus flavanones affect hepatic fatty acid oxidation in rats by acting as prooxidant agents. BioMed Research International, 2013, 1–12. https://doi.org/10.1155/2013/342973
- Corrêa, T. A. F., Rogero, M. M., Hassimotto, N. M. A., & Lajolo, F. M. (2019). The two-way polyphenols-microbiota interactions and their effects on obesity and related metabolic diseases. Frontiers in Nutrition, 6. Frontiers in nutrition, 188. https://doi.org/10.3389/fnut.2019.00188
- Coull, J. A., Beggs, S., Boudreau, D., Boivin, D., Tsuda, M., Inoue, K., Gravel, C., Salter, M. W., & De Koninck, Y. (2005). BDNF from microglia causes the shift in neuronal anion gradient underlying neuropathic pain. Nature, 438(7070), 1017–1021. https://doi.org/10.1038/nature04223
- Dalan, R., Bornstein, S. R., El-Armouche, A., Rodionov, R. N., Markov, A., Wielockx, B., Beuschlein, F., & Boehm, B. O. (2020). The ACE-2 in COVID-19: Foe or friend? Hormone and Metabolic Research, 52(5), 257–263. https://doi.org/10.1055/a-1155-0501
- DaRocha-Souto, B., Coma, M., Perez-Nievas, B., Scotton, T., Siao, M., Sánchez-Ferrer, P., Hashimoto, T., Fan, Z., Hudry, E., Barroeta, I., Serenó, L., Rodríguez, M., Sánchez, M. B., Hyman, B. T., & Gómez-Isla, T. (2012). Activation of glycogen synthase kinase-3 beta mediates β-amyloid induced neuritic damage in Alzheimer’s disease. Neurobiology of Disease, 45(1), 425–437. https://doi.org/10.1016/j.nbd.2011.09.002
- Das, S., Sarmah, S., Lyndem, S., & Singha Roy, A. (2021). An investigation into the identification of potential inhibitors of SARS-CoV-2 main protease using molecular docking study. Journal of Biomolecular Structure and Dynamics, 39(9), 3347–3357. https://doi.org/10.1080/07391102.2020.1763201
- Delgado-Roche, L., & Mesta, F. (2020). Oxidative stress as key player in severe acute respiratory syndrome coronavirus (SARS-CoV) infection. Archives of Medical Research, 51(5), 384–387. https://doi.org/10.1016/j.arcmed.2020.04.019
- Del Rio, D., Rodriguez-Mateos, A., Spencer, J. P., Tognolini, M., Borges, G., & Crozier, A. (2013). Dietary (poly) phenolics in human health: Structures, bioavailability, and evidence of protective effects against chronic diseases. Antioxidants & Redox Signaling, 18(14), 1818–1892. https://doi.org/10.1089/ars.2012.4581
- Dhingra, A. K., Chopra, B., Rathi, V., & Sapra, S. (2022). Hesperidin: A potential therapeutic agent against COVID-19. Current Drug Discovery Technologies, 20(2). https://doi.org/10.2174/1570163820666221017111556/
- Dhingra, A. K., Chopra, B., Rathi, V., & Sapra, S. (2023). Hesperidin: A potential therapeutic agent against COVID-19. Current Drug Discovery Technologies, 20(2), 1–8. https://doi.org/10.2174/1570163820666221017111556
- Dokumacioglu, E., Iskender, H., & Musmul, A. (2019). Effect of hesperidin treatment on α-Klotho/FGF-23 pathway in rats with experimentally-induced diabetes. Biomedicine & Pharmacotherapy, 109, 1206–1210. https://doi.org/10.1016/j.biopha.2018.10.192
- Do Nascimento, G. S., Constantin, R. P., Gilglioni, E. H., de Castro Ghizoni, C. V., Bracht, A., Utsunomiya, K. S., Yamamoto, N. S., Ishii-Iwamoto, E. L., Constantin, J., & Constantin, R. P. (2018a). The acute effects of citrus flavanones on the metabolism of glycogen and monosaccharides in the isolated perfused rat liver. Toxicology Letters, 291, 158–172. https://doi.org/10.1016/j.toxlet.2018.04.001/
- Donovan, J. L., Crespy, V., Oliveira, M., Cooper, K. A., Gibson, B. B., & Williamson, G. (2006). (+)-Catechin is more bioavailable than (−)-catechin: Relevance to the bioavailability of catechin from cocoa. Free Radical Research, 40(10), 1029–1034. https://doi.org/10.1080/10715760600868545
- Du, G. Y., He, S. W., Zhang, L., Sun, C. X., Mi, L. D., & Sun, Z. G. (2018). Hesperidin exhibits in vitro and in vivo antitumor effects in human osteosarcoma MG‑63 cells and xenograft mice models via inhibition of cell migration and invasion, cell cycle arrest and induction of mitochondrial‑mediated apoptosis. Oncology Letters, 16(5), 6299–6306. https://doi.org/10.3892/ol.2018.9439
- Duran, Y., & Karaboğa, İ. (2020). Effect of hesperetin on systemic inflammation and hepatic injury after blunt chest trauma in rats. Biotechnic & Histochemistry, 95(4), 297–304. https://doi.org/10.1080/10520295.2019.1691265
- Duranoğlu, D., Uzunoglu, D., Mansuroglu, B., Arasoglu, T., & Derman, S. (2018). Synthesis of hesperetin-loaded PLGA nanoparticles by two different experimental design methods and biological evaluation of optimized nanoparticles. Nanotechnology, 29(39), 395603. https://doi.org/10.1088/1361-6528/aad111
- El-Kersh, D. M., Ezzat, S. M., Salama, M. M., Mahrous, E. A., Attia, Y. M., Ahmed, M. S., & Elmazar, M. M. (2021). Anti-estrogenic and anti-aromatase activities of citrus peels major compounds in breast cancer. Scientific Reports, 11(1), 7121. https://doi.org/10.1038/s41598-021-86599-z
- El, A. E.-D. E.-S., Sokar, S. S., Shebl, A. M., & Mohamed, D. Z. (2017). Antifibrotic effect of diethylcarbamazine combined with hesperidin against ethanol induced liver fibrosis in rats. Biomedicine & Pharmacotherapy, 89, 1196–1206. https://doi.org/10.1016/j.biopha.2017.03.013
- Erlund, I. (2004). Review of the flavonoids quercetin, hesperetin, and naringenin. Dietary sources, bioactivities, bioavailability, and epidemiology. Nutrition Research, 24(10), 851–874. https://doi.org/10.1016/j.nutres.2004.07.005
- Estruel-Amades, S., Massot-Cladera, M., Garcia-Cerdà, P., Pérez-Cano, F. J., Franch, À., Castell, M., & Camps-Bossacoma, M. (2019). Protective effect of hesperidin on the oxidative stress induced by an exhausting exercise in intensively trained rats. Nutrients, 11(4), 783. https://doi.org/10.3390/nu11040783
- Farzaei, M. H., Shahpiri, Z., Bahramsoltani, R., Rahimi, F., Najafi, R., & Rahimi, R. (2017). Efficacy and tolerability of phytomedicines in multiple sclerosis patients: A review. CNS Drugs, 31(10), 867–889. https://doi.org/10.1007/s40263-017-0466-4
- Feng, Q., & Li, Z. (2006). The causation and correlative disease of obesity and losing weight. Med Philosophy (B), 27(5), 65–69.
- Fernández‐Bedmar, Z., Anter, J., Alonso‐Moraga, A., Martín de las Mulas, J., Millán‐Ruiz, Y., & Guil‐Luna, S. (2017). Demethylating and anti‐hepatocarcinogenic potential of hesperidin, a natural polyphenol of Citrus juices. Molecular Carcinogenesis, 56(6), 1653–1662. https://doi.org/10.1002/mc.22621
- Ferreira, P. S., Spolidorio, L. C., Manthey, J. A., & Cesar, T. B. (2016). Citrus flavanones prevent systemic inflammation and ameliorate oxidative stress in C57BL/6J mice fed high-fat diet. Food & Function, 7(6), 2675–2681. https://doi.org/10.1039/C5FO01541C
- Franke, S. I., Molz, P., Mai, C., Ellwanger, J. H., Zenkner, F. F., Horta, J. A., & Prá, D. (2018). Influence of hesperidin and vitamin C on glycemic parameters, lipid profile, and DNA damage in rats treated with sucrose overload. Anais da Academia Brasileira de Ciências, 90(2 suppl 1), 2203–2210. https://doi.org/10.1590/0001-3765201820170751
- Ghorbani, A., Nazari, M., Jeddi-Tehrani, M., & Zand, H. (2012). The citrus flavonoid hesperidin induces p53 and inhibits NF-κB activation in order to trigger apoptosis in NALM-6 cells: Involvement of PPARγ-dependent mechanism. European Journal of Nutrition, 51(1), 39–46. https://doi.org/10.1007/s00394-011-0187-2
- Girdhar, S., Girdhar, A., Verma, S. K., Lather, V., & Pandita, D. (2015). Plant derived alkaloids in major neurodegenerative diseases: From animal models to clinical trials. Journal of Ayurvedic and Herbal Medicine, 1(3), 91–100. https://doi.org/10.31254/jahm.2015.1307
- Gómez-Zorita, S., Lasa, A., Abendaño, N., Fernández-Quintela, A., Mosqueda-Solís, A., Garcia-Sobreviela, M. P., Arbonés-Mainar, J. M., & Portillo, M. P. (2017). Phenolic compounds apigenin, hesperidin and kaempferol reduce in vitro lipid accumulation in human adipocytes. Journal of Translational Medicine, 15(1), 1–10. https://doi.org/10.1186/s12967-017-1343-0
- Gu, S.-F., Wang, L.-Y., Tian, Y.-J., Zhou, Z.-X., Tang, J.-B., Liu, X.-R., Jiang, H.-P., & Shen, Y.-Q. (2019). Enhanced water solubility, antioxidant activity, and oral absorption of hesperetin by D-α-tocopheryl polyethylene glycol 1000 succinate and phosphatidylcholine. Journal of Zhejiang University-SCIENCE B, 20(3), 273–281. https://doi.org/10.1631/jzus.B1800346
- Habibyar, A. F., Sharma, N., & Khurana, N. (2016). PASS assisted prediction and pharmacological evaluation of hesperidin against scopolamine induced amnesia in mice. European Journal of Pharmacology, 789, 385–394. https://doi.org/10.1016/j.ejphar.2016.07.013
- Haghmorad, D., Mahmoudi, M. B., Salehipour, Z., Jalayer, Z., Kokhaei, M., Rastin, P., Mahmoudi, M., & Mahmoudi, M. (2017). Hesperidin ameliorates immunological outcome and reduces neuroinflammation in the mouse model of multiple sclerosis. Journal of Neuroimmunology, 302, 23–33. https://doi.org/10.1016/j.jneuroim.2016.11.009
- Hajialyani, M., Hosein Farzaei, M., Echeverría, J., Nabavi, S. M., Uriarte, E., & Sobarzo-Sánchez, E. (2019). Hesperidin as a neuroprotective agent: A review of animal and clinical evidence. Molecules, 24(3), 648. https://doi.org/10.3390/molecules24030648
- Halim, A., Nur, N. M., El-Agamy, E.-S., & Ibrahim, A. (2017). Protective effect of hesperidin (HDN) on carbon tetrachloride (CCl4)-induced hepatic toxicity in male albino rats. AIJCR, 8(11), 20328–20338. https://doi.org/10.15520/ijcrr/2017/8/11/349
- Hamdy, S. M., Shabaan, A. M., Latif, A. K. M. A., Abdel-Aziz, A. M., & Amin, A. M. (2017). Protective effect of hesperidin and tiger nut against acrylamide toxicity in female rats. Experimental and Toxicologic Pathology, 69(8), 580–588. https://doi.org/10.1016/j.etp.2017.05.004
- Hassan, A. A., Thabet, N. M., & Abdel-Rafei, M. K. (2018). Hyaluronan as a mediator for the hepatoprotective effect of Diosmin/Hesperidin complex. Pakistan Journal of Pharmaceutical Sciences, 31(4).
- Hati, S., & Bhattacharyya, S. (2020). Impact of thiol–Disulfide balance on the binding of COVID-19 spike protein with angiotensin-converting enzyme 2 receptor. ACS Omega, 5(26), 16292–16298. https://doi.org/10.1021/acsomega.0c02125
- Hemanth Kumar, B., Dinesh Kumar, B., & Diwan, P. V. (2017). Hesperidin, a citrus flavonoid, protects against l-methionine-induced hyperhomocysteinemia by abrogation of oxidative stress, endothelial dysfunction and neurotoxicity in Wistar rats. Pharmaceutical Biology, 55(1), 146–155. https://doi.org/10.1080/13880209.2016.1231695
- He, S., Wang, X., Zhong, Y., Tang, L., Zhang, Y., Ling, Y., Tan, Z., Yang, P., & Chen, A. (2017). Hesperetin post-treatment prevents rat cardiomyocytes from hypoxia/reoxygenation injury in vitro via activating PI3K/Akt signaling pathway. Biomedicine & Pharmacotherapy, 91, 1106–1112. https://doi.org/10.1016/j.biopha.2017.05.003
- Jack, B. U., Malherbe, C. J., Willenburg, E. L., de Beer, D., Huisamen, B., Joubert, E., Muller, C. J., Louw, J., & Pheiffer, C. (2018). Polyphenol-enriched fractions of cyclopia intermedia selectively affect lipogenesis and Lipolysis in 3T3-L1 adipocytes. Planta medica, 84(2), 100–110. https://doi.org/10.1055/s-0043-119463
- Javed, H., Vaibhav, K., Ahmed, M. E., Khan, A., Tabassum, R., Islam, F., Safhi, M. M., & Islam, F. (2015). Effect of hesperidin on neurobehavioral, neuroinflammation, oxidative stress and lipid alteration in intracerebroventricular streptozotocin induced cognitive impairment in mice. Journal of the Neurological Sciences, 348(1–2), 51–59. https://doi.org/10.1016/j.jns.2014.10.044
- Jiao, Q., Xu, L., Jiang, L., Jiang, Y., Zhang, J., & Liu, B. (2020). Metabolism study of hesperetin and hesperidin in rats by UHPLC-LTQ-Orbitrap MS n. Xenobiotica, 50(11), 1311–1322. https://doi.org/10.1080/00498254.2019.1567956
- Jiménez-Aliaga, K., Bermejo-Bescós, P., Benedí, J., & Martín-Aragón, S. (2011). Quercetin and rutin exhibit antiamyloidogenic and fibril-disaggregating effects in vitro and potent antioxidant activity in APPswe cells. Life Sciences, 89(25–26), 939–945. https://doi.org/10.1016/j.lfs.2011.09.023
- Jo, S. H., Kim, M. E., Cho, J. H., Lee, Y., Lee, J., Park, Y.-D., & Lee, J. S. (2019). Hesperetin inhibits neuroinflammation on microglia by suppressing inflammatory cytokines and MAPK pathways. Archives of Pharmacal Research, 42(8), 695–703. https://doi.org/10.1007/s12272-019-01174-5
- Joshi, R. S., Jagdale, S. S., Bansode, S. B., Shankar, S. S., Tellis, M. B., Pandya, V. K., Chugh, A., Giri, A. P., & Kulkarni, M. J. (2021). Discovery of potential multi-target-directed ligands by targeting host-specific SARS-CoV-2 structurally conserved main protease. Journal of Biomolecular Structure and Dynamics, 39(9), 3099–3114.
- Jung, S. Y., Sobel, E. M., Papp, J. C., Crandall, C. J., Fu, A. N., & Zhang, Z. F. (2016). Obesity and associated lifestyles modify the effect of glucose metabolism‐related genetic variants on impaired glucose homeostasis among postmenopausal women. Genetic Epidemiology, 40(6), 520–530. https://doi.org/10.1002/gepi.21991
- Justin Thenmozhi, A., William Raja, T. R., Manivasagam, T., Janakiraman, U., & Essa, M. M. (2017). Hesperidin ameliorates cognitive dysfunction, oxidative stress and apoptosis against aluminium chloride induced rat model of Alzheimer’s disease. Nutritional Neuroscience, 20(6), 360–368. https://doi.org/10.1080/1028415X.2016.1144846
- Ju, C., & Tacke, F. (2016). Hepatic macrophages in homeostasis and liver diseases: From pathogenesis to novel therapeutic strategies. Cellular & Molecular Immunology, 13(3), 316–327. https://doi.org/10.1038/cmi.2015.104
- Kabała-Dzik, A., Rzepecka-Stojko, A., Kubina, R., Iriti, M., Wojtyczka, R. D., Buszman, E., & Stojko, J. F. (2018). Flavonoids, bioactive components of propolis, exhibit cytotoxic activity and induce cell cycle arrest and apoptosis in human breast cancer cells MDA-MB-231 and MCF-7 – a comparative study. Cellular and Molecular Biology, 64(8), 1–10. https://doi.org/10.14715/cmb/2018.64.8.1
- Kamaraj, S., Anandakumar, P., Jagan, S., Ramakrishnan, G., & Devaki, T. (2010). Modulatory effect of hesperidin on benzo (a) pyrene induced experimental lung carcinogenesis with reference to COX-2, MMP-2 and MMP-9. European Journal of Pharmacology, 649(1–3), 320–327. https://doi.org/10.1016/j.ejphar.2010.09.017
- Kamboh, A., & Zhu, W.-Y. (2013). Effect of increasing levels of bioflavonoids in broiler feed on plasma anti-oxidative potential, lipid metabolites, and fatty acid composition of meat. Poultry Science, 92(2), 454–461. https://doi.org/10.3382/ps.2012-02584
- Kaur, G., Tirkey, N., & Chopra, K. (2006). Beneficial effect of hesperidin on lipopolysaccharide-induced hepatotoxicity. Toxicology, 226(2–3), 152–160. https://doi.org/10.1016/j.tox.2006.06.018
- Kawaguchi, K., Kikuchi, S.-I., Hasunuma, R., Maruyama, H., Yoshikawa, T., & Kumazawa, Y. (2004). A citrus flavonoid hesperidin suppresses infection-induced endotoxin shock in mice. Biological and Pharmaceutical Bulletin, 27(5), 679–683. https://doi.org/10.1248/bpb.27.679
- Keles, E. (2020). Mild SARS-CoV-2 infections in children might be based on evolutionary biology and linked with host reactive oxidative stress and antioxidant capabilities. New Microbes and New Infections, 36, 100723. https://doi.org/10.1016/j.nmni.2020.100723
- Kim, H. Y., Park, M., Kim, K., Lee, Y. M., & Rhyu, M. R. (2013). Hesperetin stimulates cholecystokinin secretion in enteroendocrine STC-1 cells. Biomolecules & Therapeutics, 21(2), 121. https://doi.org/10.4062/biomolther.2012.077
- Kivrak, I., Duru, M. E., Öztürk, M., Mercan, N., Harmandar, M., & Topçu, G. (2009). Antioxidant, anticholinesterase and antimicrobial constituents from the essential oil and ethanol extract of Salvia potentillifolia. Food Chemistry, 116(2), 470–479. https://doi.org/10.1016/j.foodchem.2009.02.069
- Koolaji, N., Shammugasamy, B., Schindeler, A., Dong, Q., Dehghani, F., & Valtchev, P. (2020). Citrus peel flavonoids as potential cancer prevention agents. Current Developments in Nutrition, 4(5), nzaa025. https://doi.org/10.1093/cdn/nzaa025
- Kumar, A., Chaudhary, T., & Mishra, J. (2013). Minocycline modulates neuroprotective effect of hesperidin against quinolinic acid induced Huntington’s disease like symptoms in rats: Behavioral, biochemical, cellular and histological evidences. European Journal of Pharmacology, 720(1–3), 16–28. https://doi.org/10.1016/j.ejphar.2013.10.057
- Kumar, P., & Kumar, A. (2010). Protective effect of hesperidin and naringin against 3-nitropropionic acid induced Huntington’s like symptoms in rats: Possible role of nitric oxide. Behavioural Brain Research, 206(1), 38–46. https://doi.org/10.1016/j.bbr.2009.08.028
- Kumar, B. H., Kumar, B. D., & Diwan, P. (2017). Protective effects of natural dietary antioxidants fisetin and hesperidin on chronic mild hyperhomocysteinemia-induced vascular dementia in wistar rats. Journal of the Neurological Sciences, 381, 319. https://doi.org/10.1016/j.jns.2017.08.905
- Lévèques, A., Actis-Goretta, L., Rein, M. J., Williamson, G., Dionisi, F., & Giuffrida, F. (2012). UPLC–MS/MS quantification of total hesperetin and hesperetin enantiomers in biological matrices. Journal of Pharmaceutical and Biomedical Analysis, 57, 1–6. https://doi.org/10.1016/j.jpba.2011.08.031
- Li, G., Chen, M.-J., Wang, C., Nie, H., Huang, W.-J., Yuan, T.-D., Sun, T., Shu, K.-G., Wang, C.-F., Gong, Q., & Tang, S.-Q. (2014). Protective effects of hesperidin on concanavalin A-induced hepatic injury in mice. International Immunopharmacology, 21(2), 406–411. https://doi.org/10.1016/j.intimp.2014.05.018
- Lima, A. C. D., Cecatti, C., Fidélix, M. P., Adorno, M. A. T., Sakamoto, I. K., Cesar, T. B., & Sivieri, K. (2019). Effect of daily consumption of orange juice on the levels of blood glucose, lipids, and gut microbiota metabolites: Controlled clinical trials. Journal of Medicinal Food, 22(2), 202–210. https://doi.org/10.1089/jmf.2018.0080
- Lim, H., Yeo, E., Song, E., Chang, Y.-H., Han, B.-K., Choi, H.-J., & Hwang, J. (2015). Bioconversion of Citrus unshiu peel extracts with cytolase suppresses adipogenic activity in 3T3-L1 cells. Nutrition Research and Practice, 9(6), 599–605. https://doi.org/10.4162/nrp.2015.9.6.599
- Lin, C.-W., Tsai, F.-J., Tsai, C.-H., Lai, C.-C., Wan, L., Ho, T.-Y., Hsieh, C.-C., & Chao, P.-D. L. (2005). Anti-SARS coronavirus 3C-like protease effects of Isatis indigotica root and plant-derived phenolic compounds. Antiviral Research, 68(1), 36–42. https://doi.org/10.1016/j.antiviral.2005.07.002
- Liskova, A., Samec, M., Koklesova, L., Brockmueller, A., Zhai, K., Abdellatif, B., Siddiqui, M., Biringer, K., Kudela, E., Pec, M., Gadanec, L. K., Šudomová, M., Hassan, S. T. S., Zulli, A., Shakibaei, M., Giordano, F. A., Büsselberg, D., Golubnitschaja, O., & Kubatka, P. (2021). Flavonoids as an effective sensitizer for anti-cancer therapy: Insights into multi-faceted mechanisms and applicability towards individualized patient profiles. The EPMA Journal, 12(2), 155–176. https://doi.org/10.1007/s13167-021-00242-5
- Liu, W. Y., Liou, S.-S., Hong, T.-Y., & Liu, I.-M. (2017). Protective effects of hesperidin (citrus flavonone) on high glucose induced oxidative stress and apoptosis in a cellular model for diabetic retinopathy. Nutrients, 9(12), 1312. https://doi.org/10.3390/nu9121312
- Liu, X., Luo, F., Li, P., She, Y., & Gao, W. (2017). Investigation of the interaction for three Citrus flavonoids and α-amylase by surface plasmon resonance. Food Research International, 97, 1–6. https://doi.org/10.1016/j.foodres.2017.03.023
- Li, S., Zhou, X., & Zheng, T. (2004). Progress of studies of obese on mechanism and treatment by traditional Chinese medicine. Chinese Journal of Integrative Medicine, 2(11), 657–659.
- Li, C., Zug, C., Qu, H., Schluesener, H., & Zhang, Z. (2015). Hesperidin ameliorates behavioral impairments and neuropathology of transgenic APP/PS1 mice. Behavioural Brain Research, 281, 32–42. https://doi.org/10.1016/j.bbr.2014.12.012
- LoPachin, R. M., & Gavin, T. (2012). Molecular mechanism of acrylamide neurotoxicity: Lessons learned from organic chemistry. Environmental Health Perspectives, 120(12), 1650–1657. https://doi.org/10.1289/ehp.1205432
- Lu, K., & Yip, Y. M. (2023). Therapeutic potential of bioactive flavonoids from citrus fruit peels toward obesity and diabetes mellitus. Future Pharmacology, 3(1), 14–37. https://doi.org/10.3390/futurepharmacol3010002
- Madureira, M. B., Concato, V. M., Cruz, E. M. S., Bitencourt de Morais, J. M., Inoue, F. S. R., Concimo Santos, N., Gonçalves, M. D., Cremer de Souza, M., Basso Scandolara, T., Fontana Mezoni, M., Galvani, M., Rodrigues Ferreira Seiva, F., Panis, C., Miranda-Sapla, M. M., & Pavanelli, W. R. (2023). Naringenin and hesperidin as promising alternatives for prevention and co-adjuvant therapy for breast cancer. Antioxidants, 12(3), 586. https://doi.org/10.3390/antiox12030586
- Mahmoud, A. M. (2013). Hematological alterations in diabetic rats-role of adipocytokines and effect of citrus flavonoids. Excli Journal, 12, 647. https://www.ncbi.nlm.nih.gov/pmc/articles/PMC4778348/
- Mahmoud, A. M., Ashour, M. B., Abdel-Moneim, A., & Ahmed, O. M. (2012). Hesperidin and naringin attenuate hyperglycemia-mediated oxidative stress and proinflammatory cytokine production in high fat fed/streptozotocin-induced type 2 diabetic rats. Journal of Diabetes and Its Complications, 26(6), 483–490. https://doi.org/10.1016/j.jdiacomp.2012.06.001
- Mahmoud, A. M., Hernández Bautista, R. J., Sandhu, M. A., & Hussein, O. E. (2019). Beneficial effects of citrus flavonoids on cardiovascular and metabolic health. Oxidative Medicine and Cellular Longevity, 2019, 5484138. https://doi.org/10.1155/2019/5484138
- Mahmoud, A. M., Mohammed, H. M., Khadrawy, S. M., & Galaly, S. R. (2017). Hesperidin protects against chemically induced hepatocarcinogenesis via modulation of Nrf2/ARE/HO-1, PPARγ and TGF-β1/Smad3 signaling, and amelioration of oxidative stress and inflammation. Chemico-Biological Interactions, 277, 146–158. https://doi.org/10.1016/j.cbi.2017.09.015
- Majumdar, S., & Srirangam, R. (2009). Solubility, stability, physicochemical characteristics and in vitro ocular tissue permeability of hesperidin: A natural bioflavonoid. Pharmaceutical Research, 26(5), 1217–1225. https://doi.org/10.1007/s11095-008-9729-6
- Manea, A. (2010). NADPH oxidase-derived reactive oxygen species: Involvement in vascular physiology and pathology. Cell and Tissue Research, 342(3), 325–339. https://doi.org/10.1007/s00441-010-1060-y
- Menze, E. T., Tadros, M. G., Abdel-Tawab, A. M., & Khalifa, A. E. (2012). Potential neuroprotective effects of hesperidin on 3-nitropropionic acid-induced neurotoxicity in rats. Neurotoxicology, 33(5), 1265–1275. https://doi.org/10.1016/j.neuro.2012.07.007
- Merisko-Liversidge, E., Liversidge, G. G., & Cooper, E. R. (2003). Nanosizing: A formulation approach for poorly-water-soluble compounds. European Journal of Pharmaceutical Sciences, 18(2), 113–120. https://doi.org/10.1016/S0928-0987(02)00251-8
- Miler, M., Živanović, J., Ajdžanović, V., Oreščanin-Dušić, Z., Milenković, D., Konić-Ristić, A., Blagojević, D., Milošević, V., & Šošić-Jurjević, B. (2016). Citrus flavanones naringenin and hesperetin improve antioxidant status and membrane lipid compositions in the liver of old-aged Wistar rats. Experimental Gerontology, 84, 49–60. https://doi.org/10.1016/j.exger.2016.08.014
- Minagar, A., Shapshak, P., & Alexander, J. S. (2004). Dementia and multiple sclerosis: Role of microglia and astrocytes. In Role Glia Neurotox (pp. 263).
- Mosqueda-Solís, A., Lasa, A., Gómez-Zorita, S., Eseberri, I., Picó, C., & Portillo, M. P. (2017). Screening of potential anti-adipogenic effects of phenolic compounds showing different chemical structure in 3T3-L1 preadipocytes. Food & Function, 8(10), 3576–3586. https://doi.org/10.1039/C7FO00679A
- Mosqueda-Solís, A., Sánchez, J., Reynés, B., Palou, M., Portillo, M. P., Palou, A., & Picó, C. (2018a). Hesperidin and capsaicin, but not the combination, prevent hepatic steatosis and other metabolic syndrome-related alterations in western diet-fed rats. Scientific Reports, 8(1), 1–14. https://doi.org/10.1038/s41598-018-32875-4/
- Mottay, D., & Neergheen-Bhujun, V. S. (2015). Anticholinesterase and antioxidant effects of traditional herbal medicines used in the management of neurodegenerative diseases in mauritius. Archives of Medical and Biomedical Research, 2(4), 114–130. https://doi.org/10.4314/ambr.v2i4.2
- Mubagwa, K. (2020). Cardiac effects and toxicity of chloroquine: A short update. International Journal of Antimicrobial Agents, 56(2), 106057. https://doi.org/10.1016/j.ijantimicag.2020.106057
- Muili, K. A., Gopalakrishnan, S., Meyer, S. L., Eells, J. T., Lyons, J.-A., & Najbauer, J. (2012). Amelioration of experimental autoimmune encephalomyelitis in C57BL/6 mice by photobiomodulation induced by 670 nm light. PloS One, 7(1), e30655. https://doi.org/10.1371/journal.pone.0030655
- Mullen, W., Archeveque, M.-A., Edwards, C. A., Matsumoto, H., & Crozier, A. (2008). Bioavailability and metabolism of orange juice flavanones in humans: Impact of a full-fat yogurt. Journal of Agricultural and Food Chemistry, 56(23), 11157–11164. https://doi.org/10.1021/jf801974v
- Musumeci, L., Maugeri, A., Cirmi, S., Lombardo, G. E., Russo, C., Gangemi, S., Calapai, G., & Navarra, M. (2020). Citrus fruits and their flavonoids in inflammatory bowel disease: An overview. Natural Product Research, 34(1), 122–136. https://doi.org/10.1080/14786419.2019.1601196
- Nakajima, V. M., Moala, T., Caria, C., Moura, C. S., Amaya-Farfan, J., Gambero, A., Macedo, G. A., & Macedo, J. A. (2017). Biotransformed citrus extract as a source of anti-inflammatory polyphenols: Effects in macrophages and adipocytes. Food Research International, (Ottawa, Ont.) 97, 37–44. https://doi.org/10.1016/j.foodres.2017.03.034
- Naz, H., Tarique, M., Ahamad, S., Alajmi, M. F., Hussain, A., Rehman, M. T., Luqman, S., & Hassan, M. I. (2019). Hesperidin‐CAMKIV interaction and its impact on cell proliferation and apoptosis in the human hepatic carcinoma and neuroblastoma cells. Journal of Cellular Biochemistry, 120(9), 15119–15130. https://doi.org/10.1002/jcb.28774
- Nielsen, I. L. F., Chee, W. S., Poulsen, L., Offord-Cavin, E., Rasmussen, S. E., Frederiksen, H., Enslen, M., Barron, D., Horcajada, M.-N., & Williamson, G. (2006). Bioavailability is improved by enzymatic modification of the citrus flavonoid hesperidin in humans: A randomized, double-blind, crossover trial. The Journal of Nutrition, 136(2), 404–408. https://doi.org/10.1093/jn/136.2.404
- Nie, S., Xing, Y., Kim, G. J., & Simons, J. W. (2007). Nanotechnology applications in cancer. Annual Review of Biomedical Engineering, 9(1), 257–288. https://doi.org/10.1146/annurev.bioeng.9.060906.152025
- Ontaneda, D., Thompson, A. J., Fox, R. J., & Cohen, J. A. (2017). Progressive multiple sclerosis: Prospects for disease therapy, repair, and restoration of function. The Lancet, 389(10076), 1357–1366. https://doi.org/10.1016/S0140-67361631320-4
- Palit, S., Kar, S., Sharma, G., & Das, P. K. (2015). Hesperetin induces apoptosis in breast carcinoma by triggering accumulation of ROS and activation of ASK1/JNK pathway. Journal of Cellular Physiology, 230(8), 1729–1739. https://doi.org/10.1002/jcp.24818
- Pandey, P., & Khan, F. (2021). A mechanistic review of the anticancer potential of hesperidin, a natural flavonoid from citrus fruits. Nutrition Research, 92, 21–31. https://doi.org/10.1016/j.nutres.2021.05.011
- Pandey, P., Sayyed, U., Tiwari, R. K., Siddiqui, M. H., Pathak, N., & Bajpai, P. (2019). Hesperidin induces ROS-mediated apoptosis along with cell cycle arrest at G2/M phase in human gall bladder carcinoma. Nutrition and Cancer, 71(4), 676–687. https://doi.org/10.1080/01635581.2018.1508732
- Park, H.-Y., Choi, H.-D., Eom, H., & Choi, I. (2013). Enzymatic modification enhances the protective activity of citrus flavonoids against alcohol-induced liver disease. Food Chemistry, 139(1–4), 231–240. https://doi.org/10.1016/j.foodchem.2013.01.044
- Park, H.-K., Kang, S. W., & Park, M.-S. (2019). Hesperidin ameliorates hepatic ischemia-reperfusion injury in Sprague-Dawley rats. Transplantation Proceedings,
- Parmar, S., Syed, M., Gray, I. P., & Ray, D. S. (2015). Curcumin, hesperidin, and rutin selectively interfere with apoptosis signaling and attenuate streptozotocin-induced oxidative stress-mediated hyperglycemia. Current Neurovascular Research, 12(4), 363–374. https://doi.org/10.2174/1567202612666150812150249
- Peng, H., Wei, Z., Luo, H., Yang, Y., Wu, Z., Gan, L., & Yang, X. (2016). Inhibition of fat accumulation by hesperidin in Caenorhabditis elegans. Journal of Agricultural and Food Chemistry, 64(25), 5207–5214. https://doi.org/10.1021/acs.jafc.6b02183
- Polat, F. R., Karaboğa, I., Polat, M. S., Erboğa, Z. F., Yılmaz, A., & Güzel, S. (2018). Effect of hesperetin on inflammatory and oxidative status in trinitrobenzene sulfonic acid-induced experimental colitis model. Cellular and Molecular Biology, 64(11), 58–65. https://doi.org/10.14715/cmb/2018.64.11.11
- Pu, P. (2016). Protection mechanisms of hesperidin on mouse with insulin resistance. Zhongguo Zhong Yao Za Zhi= Zhongguo Zhongyao Zazhi= China Journal of Chinese Materia Medica, 41(17), 3290–3295. https://doi.org/10.4268/cjcmm20161728
- Puertollano, A., Puertollano, M., Alvarez, E., & de Cienfuegos, G. (2011). Dietary antioxidants: Immunity and host defense. Current Topics in Medicinal Chemistry, 11(14), 1752–1766. https://doi.org/10.2174/156802611796235107
- Qian, W., Hasegawa, J., Cai, X., Yang, J., Ishihara, Y., Ping, B., Tsuno, S., Endo, Y., Matsuda, A., & Miura, N. (2016a). Components of boiogito suppress the progression of hypercholesterolemia and fatty liver induced by high-cholesterol diet in rats. Yonago Acta Medica, 59(1), 67. https://www.ncbi.nlm.nih.gov/pmc/articles/PMC4816751/
- Qin, X.-Y., Cheng, Y., & Yu, L.-C. (2012). Potential protection of green tea polyphenols against intracellular amyloid beta-induced toxicity on primary cultured prefrontal cortical neurons of rats. Neuroscience Letters, 513(2), 170–173. https://doi.org/10.1016/j.neulet.2012.02.029
- Rasool, M., Malik, A., Qureshi, M. S., Manan, A., Pushparaj, P. N., Asif, M., Qazi, M. H., Qazi, A. M., Kamal, M. A., Gan, S. H., & Sheikh, I. A. (2014). Recent updates in the treatment of neurodegenerative disorders using natural compounds. Evidence-Based Complementary and Alternative Medicine, 2014, 1–7. https://doi.org/10.1155/2014/979730
- Rauf, A., Shariati, M. A., Imran, M., Bashir, K., Khan, S. A., Mitra, S., Emran, T. B., Badalova, K., Uddin, M. S., Mubarak, M. S., Aljohani, A. S. M., Alhumaydhi, F. A., Derkho, M., Korpayev, S., & Zengin, G. (2022). Comprehensive review on naringenin and naringin polyphenols as a potent anticancer agent. Environmental Science and Pollution Research International, 29(21), 31025–31041. https://doi.org/10.1007/s11356-022-18754-6
- Roohbakhsh, A., Parhiz, H., Soltani, F., Rezaee, R., & Iranshahi, M. (2015). Molecular mechanisms behind the biological effects of hesperidin and hesperetin for the prevention of cancer and cardiovascular diseases. Life Sciences, 124, 64–74. https://doi.org/10.1016/j.lfs.2014.12.030
- Rosas, H. D., Lee, S. Y., Bender, A. C., Zaleta, A. K., Vangel, M., Yu, P., Fischl, B., Pappu, V., Onorato, C., & Cha, J.-H. (2010). Altered white matter microstructure in the corpus callosum in Huntington’s disease: Implications for cortical “disconnection”. Neuroimage, 49(4), 2995–3004. https://doi.org/10.1016/j.neuroimage.2009.10.015
- Rosas, H., Liu, A., Hersch, S., Glessner, M., Ferrante, R., Salat, D., van Der Kouwe, A., Jenkins, B., Dale, A., & Fischl, B. (2002). Regional and progressive thinning of the cortical ribbon in Huntington’s disease. Neurology, 58(5), 695–701. https://doi.org/10.1212/WNL.58.5.695
- Saiprasad, G., Chitra, P., Manikandan, R., & Sudhandiran, G. (2014). Hesperidin induces apoptosis and triggers autophagic markers through inhibition of Aurora-A mediated phosphoinositide-3-kinase/Akt/mammalian target of rapamycin and glycogen synthase kinase-3 beta signalling cascades in experimental colon carcinogenesis. European Journal of Cancer, 50(14), 2489–2507. https://doi.org/10.1016/j.ejca.2014.06.013
- Saleh, J., Peyssonnaux, C., Singh, K. K., & Edeas, M. (2020). Mitochondria and microbiota dysfunction in COVID-19 pathogenesis. Mitochondrion, 54, 1–7. https://doi.org/10.1016/j.mito.2020.06.008
- Salem, H. R. A., El-Raouf, A., Saleh, E. M., & Shalaby, K. (2012). Influence of hesperidin combined with Sinemet on genetical and biochemical abnormalities in rats suffering from Parkinson’s disease. Life Science Journal, 9(4), 930–945. http://www.lifesciencesite.com/lsj/life0904/145_11564life0904_930_945.pdf
- Santos, G., Giraldez-Alvarez, L. D., Ávila-Rodriguez, M., Capani, F., Galembeck, E., Neto, A. G., Barreto, G. E., & Andrade, B. (2016). SUR1 receptor interaction with hesperidin and linarin predicts possible mechanisms of action of Valeriana officinalis in Parkinson. Frontiers in Aging Neuroscience, 8, 97. https://doi.org/10.3389/fnagi.2016.00097
- Selvaraj, P., & Pugalendi, K. V. (2012). Efficacy of hesperidin on plasma, heart and liver tissue lipids in rats subjected to isoproterenol-induced cardiotoxicity. Experimental and Toxicologic Pathology, 64(5), 449–452. https://doi.org/10.1016/j.etp.2010.10.012
- Shahbazi, R., Cheraghpour, M., Homayounfar, R., Nazari, M., Nasrollahzadeh, J., & Davoodi, S. H. (2018). Hesperidin inhibits insulin-induced phosphoinositide 3–kinase/Akt activation in human pre-B cell line NALM-6. Journal of Cancer Research and Therapeutics, 14(3), 503. https://doi.org/10.4103/0973-1482.157323
- Shen, W., Xu, Y., & Lu, Y.-H. (2012). Inhibitory effects of Citrus flavonoids on starch digestion and antihyperglycemic effects in HepG2 cells. Journal of Agricultural and Food Chemistry, 60(38), 9609–9619. https://doi.org/10.1021/jf3032556
- Shi, Y., Jiang, J., Shan, Z., Bu, Y., Deng, Z., & Cheng, Y. (2015). Oxidative stress and histopathological alterations in liver of Cyprinus carpio L. induced by intraperitoneal injection of microcystin-LR. Ecotoxicology, 24(3), 511–519. https://doi.org/10.1007/s10646-014-1399-z
- Shin, E. J., Hur, H. J., Sung, M. J., Park, J. H., Yang, H. J., Kim, M. S., Kwon, D. Y., & Hwang, J.-T. (2013). Ethanol extract of the Prunus mume fruits stimulates glucose uptake by regulating PPAR-γ in C2C12 myotubes and ameliorates glucose intolerance and fat accumulation in mice fed a high-fat diet. Food Chemistry, 141(4), 4115–4121. https://doi.org/10.1016/j.foodchem.2013.06.059
- Shirani, K., Yousefsani, B. S., Shirani, M., & Karimi, G. (2020). Protective effects of naringin against drugs and chemical toxins induced hepatotoxicity: A review. Phytotherapy Research, 34(8), 1734–1744. https://doi.org/10.1002/ptr.6641
- Siddiqi, A., Hasan, S. K., Nafees, S., Rashid, S., Saidullah, B., & Sultana, S. (2015). Chemopreventive efficacy of hesperidin against chemically induced nephrotoxicity and renal carcinogenesis via amelioration of oxidative stress and modulation of multiple molecular pathways. Experimental and Molecular Pathology, 99(3), 641–653. https://doi.org/10.1016/j.yexmp.2015.11.012
- Siddiqi, A., Saidullah, B., & Sultana, S. (2018). Anti‐carcinogenic effect of hesperidin against renal cell carcinoma by targeting COX‐2/PGE2 pathway in Wistar rats. Environmental Toxicology, 33(10), 1069–1077. https://doi.org/10.1002/tox.22626
- Simitzis, P. E., ILIAS‐DIMOPOULOS, V., Charismiadou, M. A., Biniari, E. E., & Deligeorgis, S. G. (2013). The effects of dietary hesperidin supplementation on lamb performance and meat characteristics. Animal Science Journal, 84(2), 136–143. https://doi.org/10.1111/j.1740-0929.2012.01049.x
- Souza, L. C., de Gomes, M. G., Goes, A. T., Del Fabbro, L., Carlos Filho, B., Boeira, S. P., & Jesse, C. R. (2013). Evidence for the involvement of the serotonergic 5-HT1A receptors in the antidepressant-like effect caused by hesperidin in mice. Progress in Neuro-Psychopharmacology and Biological Psychiatry, 40, 103–109. https://doi.org/10.1016/j.pnpbp.2012.09.003
- Su, D., Liu, H., Qi, X., Dong, L., Zhang, R., & Zhang, J. (2019). Citrus peel flavonoids improve lipid metabolism by inhibiting miR-33 and miR-122 expression in HepG2 cells. Bioscience, Biotechnology, and Biochemistry, 83(9), 1–9. https://doi.org/10.1080/09168451.2019.1608807
- Tamilselvam, K., Nataraj, J., Janakiraman, U., Manivasagam, T., & Essa, M. M. (2013). Antioxidant and anti-inflammatory potential of hesperidin against 1-methyl-4-phenyl-1, 2, 3, 6-tetrahydropyridine-induced experimental Parkinson’s disease in mice. International Journal of Nutrition, Pharmacology, Neurological Diseases, 3(3), 294. https://doi.org/10.4103/2231-0738.114875
- Thompson, M., Jaiswal, Y., Wang, I., & Williams, L. (2017). Hepatotoxicity: Treatment, causes and applications of medicinal plants as therapeutic agents. Journal Phytopharmacol, 6(3), 186–193. https://doi.org/10.31254/phyto.2017.6308
- Tian, D., & Ye, Q. (2020). Hepatic complications of COVID‐19 and its treatment. Journal of Medical Virology, 92(10), 1818–1824. https://doi.org/10.1002/jmv.26036
- Timoshin, A., Dorkina, E., Paukova, E., & Vanin, A. (2005). Quercetin and hesperidin suppress the formation of nitric oxide radicals in rat liver and heart under acute hepatosis conditions. Biophysics, 50(6), 986–989.
- Tomás‐Barberán, F. A., & Clifford, M. N. (2000). Flavanones, chalcones and dihydrochalcones–nature, occurrence and dietary burden. Journal of the Science of Food and Agriculture, 80(7), 1073–1080. https://doi.org/10.1002/(SICI)1097-0010(20000515)80:7<1073:AID-JSFA568>3.0.CO;2-B
- Trujillo, J. A., Croft, N. P., Dudek, N. L., Channappanavar, R., Theodossis, A., Webb, A. I., Dunstone, M. A., Illing, P. T., Butler, N. S., Fett, C., Tscharke, D. C., Rossjohn, J., Perlman, S., & Purcell, A. W. (2014). The cellular redox environment alters antigen presentation. Journal of Biological Chemistry, 289(40), 27979–27991. https://doi.org/10.1074/jbc.M114.573402
- Utomo, R. Y., Ikawati, M., & Meiyanto, E. (2020). Revealing the potency of citrus and galangal constituents to halt SARS-CoV-2 infection.
- Vallejo, F., Larrosa, M., Escudero, E., Zafrilla, M. P., Cerda, B., Boza, J., García-Conesa, M. T., Espín, J. C., & Tomás-Barberán, F. A. (2010). Concentration and solubility of flavanones in orange beverages affect their bioavailability in humans. Journal of Agricultural and Food Chemistry, 58(10), 6516–6524. https://doi.org/10.1021/jf100752j
- Wagener, F. A., Pickkers, P., Peterson, S. J., Immenschuh, S., & Abraham, N. G. (2020). Targeting the heme-heme oxygenase system to prevent severe complications following COVID-19 infections. Antioxidants, 9(6), 540. https://doi.org/10.3390/antiox9060540
- Wahsha, M. (2010). Biochemical screening of hesperidin and naringin against liver damage in Balb/c Mice Exposed to Microcystin-LR* Mohammad Wahsha,“Saad Al-Jassabi,“Mohd Sofian Azirun and’Khaled Abdul-Aziz” Department of Environmental Sciences, Ca’Foscari University, Italy “Institute of Biological Sciences, University of Malaya. Middle-East Journal of Scientific Research, 6(4), 354–359.
- Wang, X., Hasegawa, J., Kitamura, Y., Wang, Z., Matsuda, A., Shinoda, W., Miura, N., & Kimura, K. (2011). Effects of hesperidin on the progression of hypercholesterolemia and fatty liver induced by high-cholesterol diet in rats. Journal of Pharmacological Sciences, 117(3), 129–138. https://doi.org/10.1254/jphs.11097FP
- Wang, Y., Liu, X.-J., Chen, J.-B., Cao, J.-P., Li, X., & Sun, C.-D. (2022). Citrus flavonoids and their antioxidant evaluation. Critical Reviews in Food Science and Nutrition, 62(14), 3833–3854. https://doi.org/10.1080/10408398.2020.1870035
- Wang, D., Liu, L., Zhu, X., Wu, W., & Wang, Y. (2014). Hesperidin alleviates cognitive impairment, mitochondrial dysfunction and oxidative stress in a mouse model of Alzheimer’s disease. Cellular and Molecular Neurobiology, 34(8), 1209–1221. https://doi.org/10.1007/s10571-014-0098-x
- Wang, Y., Yu, H., Zhang, J., Gao, J., Ge, X., & Lou, G. (2015). Hesperidin inhibits HeLa cell proliferation through apoptosis mediated by endoplasmic reticulum stress pathways and cell cycle arrest. BMC Cancer, 15(1), 1–11. https://doi.org/10.1186/s12885-015-1706-y
- Wang, S., Zhang, J., Chen, M., & Wang, Y. (2013). Delivering flavonoids into solid tumors using nanotechnologies. Expert Opinion on Drug Delivery, 10(10), 1411–1428. https://doi.org/10.1517/17425247.2013.807795
- Wan, J., Kuang, G., Zhang, L., Jiang, R., Chen, Y., He, Z., & Ye, D. (2020). Hesperetin attenuated acetaminophen-induced hepatotoxicity by inhibiting hepatocyte necrosis and apoptosis, oxidative stress and inflammatory response via upregulation of heme oxygenase-1 expression. International Immunopharmacology, 83, 106435. https://doi.org/10.1016/j.intimp.2020.106435
- Williamson, G. (2017). The role of polyphenols in modern nutrition. Nutrition Bulletin, 42(3), 226–235. https://doi.org/10.1111/nbu.12278
- Wu, J. (2020). Tackle the free radicals damage in COVID-19. Nitric Oxide: Biology & Chemistry / Official Journal of the Nitric Oxide Society, 102, 39–41. https://doi.org/10.1016/j.niox.2020.06.002
- Wu, C., Liu, Y., Yang, Y., Zhang, P., Zhong, W., Wang, Y., Wang, Q., Xu, Y., Li, M., Li, X., Zheng, M., Chen, L., & Li, H. (2020). Analysis of therapeutic targets for SARS-CoV-2 and discovery of potential drugs by computational methods. Acta Pharmaceutica Sinica B, 10(5), 766–788. https://doi.org/10.1016/j.apsb.2020.02.008
- Xia, R., Sheng, X., Xu, X., Yu, C., & Lu, H. (2018). Hesperidin induces apoptosis and G0/G1 arrest in human non-small cell lung cancer A549 cells. International Journal of Molecular Medicine, 41(1), 464–472. https://doi.org/10.3892/ijmm.2017.3250
- Xiong, H., Wang, J., Ran, Q., Lou, G., Peng, C., Gan, Q., Hu, J., Sun, J., Yao, R., & Huang, Q. (2019). Hesperidin: A therapeutic agent for obesity. Drug Design, Development and Therapy, 13, 3855. https://doi.org/10.2147/DDDT.S227499
- Yáñez, J. A., Remsberg, C. M., Miranda, N. D., Vega‐Villa, K. R., Andrews, P. K., & Davies, N. M. (2008). Pharmacokinetics of selected chiral flavonoids: Hesperetin, naringenin and eriodictyol in rats and their content in fruit juices. Biopharmaceutics & Drug Disposition, 29(2), 63–82. https://doi.org/10.1002/bdd.588
- Yáñez, J. A., Teng, X. W., Roupe, K. A., & Davies, N. M. (2005). Stereospecific high-performance liquid chromatographic analysis of hesperetin in biological matrices. Journal of Pharmaceutical and Biomedical Analysis, 37(3), 591–595. https://doi.org/10.1016/j.jpba.2004.10.028
- Yang, Y., Wolfram, J., Boom, K., Fang, X., Shen, H., & Ferrari, M. (2013). Hesperetin impairs glucose uptake and inhibits proliferation of breast cancer cells. Cell Biochemistry and Function, 31(5), 374–379. https://doi.org/10.1002/cbf.2905
- Yeh, M.-H., Kao, S.-T., Hung, C.-M., Liu, C.-J., Lee, K.-H., & Yeh, C.-C. (2009). Hesperidin inhibited acetaldehyde-induced matrix metalloproteinase-9 gene expression in human hepatocellular carcinoma cells. Toxicology Letters, 184(3), 204–210. https://doi.org/10.1016/j.toxlet.2008.11.018
- Yuce, B., Danis, O., Ogan, A., Sener, G., Bulut, M., & Yarat, A. (2009). Antioxidative and lipid lowering effects of 7, 8-dihydroxy-3-(4-methylphenyl) coumarin in hyperlipidemic rats. Arzneimittelforschung, 59(3), 129–134. https://doi.org/10.1055/s-0031-1296375
- Yu, H., Zheng, L., Yin, L., Xu, L., Qi, Y., Han, X., Xu, Y., Liu, K., & Peng, J. (2014). Protective effects of the total saponins from Dioscorea nipponica Makino against carbon tetrachloride-induced liver injury in mice through suppression of apoptosis and inflammation. International Immunopharmacology, 19(2), 233–244. https://doi.org/10.1016/j.intimp.2014.01.019
- Zaghloul, R. A., Elsherbiny, N. M., Kenawy, H. I., El-Karef, A., Eissa, L. A., & El-Shishtawy, M. M. (2017). Hepatoprotective effect of hesperidin in hepatocellular carcinoma: Involvement of Wnt signaling pathways. Life Sciences, 185, 114–125. https://doi.org/10.1016/j.lfs.2017.07.026
- Zamora-Ros, R., Andres Lacueva, C., Lamuela-Raventós, R. M., Berenguer, T., Jakszyn, P., Barricarte, A., Ardanaz, E., Amiano, P., Dorronsoro, M., Larrañaga, N., Martínez, C., Sánchez, M. J., Navarro, C., Chirlaque, M. D., Tormo, M. J., Quirós, J. R., & González, C. A. (2010). Estimation of dietary sources and flavonoid intake in a Spanish adult population (EPIC-Spain). Journal of the American Dietetic Association, 110(3), 390–398. https://doi.org/10.1016/j.jada.2009.11.024
- Zareei, S., Boojar, M. M., & Amanlou, M. (2017). Inhibition of liver alanine aminotransferase and aspartate aminotransferase by hesperidin and its aglycone hesperetin: An in vitro and in silico study. Life Sciences, 178, 49–55. https://doi.org/10.1016/j.lfs.2017.04.001/
- Zhang, J., Song, J., Wu, D., Wang, J., & Dong, W. (2015). Hesperetin induces the apoptosis of hepatocellular carcinoma cells via mitochondrial pathway mediated by the increased intracellular reactive oxygen species, ATP and calcium. Medical Oncology, 32(4), 1–11. https://doi.org/10.1007/s12032-015-0516-z
- Zhou, Z., Zhong, W., Lin, H., Huang, P., Ma, N., Zhang, Y., Zhou, C., Lai, Y., Huang, S., Huang, S., Gao, L., & Lv, Z. (2017). Hesperidin protects against acute alcoholic injury through improving lipid metabolism and cell damage in zebrafish larvae. Evidence-Based Complementary and Alternative Medicine, 2017, 1–9. https://doi.org/10.1155/2017/7282653