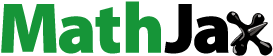
Abstract
Woody plants are important sources of edible oil, and the tea plant (Camellia sinensis) is a new option. In this study, the contents and composition of fatty acids in seed kernels of 10 conventional cultivars and 4 albino cultivars were analyzed and compared by GC-MS. A total of 22 fatty acids were identified. The main fatty acids were oleic acid (C18:1Δ9) (9.1051–15.0670%), linoleic acid (C18:2Δ9,12) (3.6386–7.7231%), and palmitic acid (C16:0) (2.3660–3.9445%), respectively. The ratio of saturated fatty acids, monounsaturated fatty acids (MUFAs) and polyunsaturated fatty acids of albino cultivar “Zhonghuang 2” (ZH No.2) was 1.00:2.67:1.85, of which the proportion of MUFAs was significantly lower than those of most conventional cultivars and albino cultivar “Suyuhuang”. Therefore, it is necessary to determine the chemical constituents before the development and utilization of tea seeds, especially for albino cultivars. Furthermore, ZH No.2 could be chosen as parent for improving the fatty acid composition of tea seeds in the breeding project.
1. Introduction
Tea (Camellia sinensis (L.) O. Kuntze) is a kind of important economic crop that has not been fully exploited. Tea leaf is rich in various antioxidant components and has been consumed as a beverage material by more than two-thirds of the world population (Hibasami et al., Citation1998; Wei et al., Citation2019). Tea seeds, which collected from conventional cultivars, normally consist of black shells and yellow kernels: the dried kernels contain about 15–35% oil (Chang et al., Citation2019; Wang et al., Citation2017). C. sinensis seed oils (CSSOs) have been accepted as edible oil in several countries, such as Iran, India, Sri Lanka, Java Island (Indonesia), Japan and China (Chang et al., Citation2019; Sahari et al., Citation2004; Sengupta et al., Citation1976; Wang et al., Citation2017). The major fatty acids in CSSOs were oleic acid (C18:1Δ9), linoleic acid (C18:2Δ9,12), and palmitic acid (C16:0), respectively. The ratio of saturated fatty acids (SFAs), monounsaturated fatty acids (MUFAs), and polyunsaturated fatty acids (PUFAs) in the CSSO was 1:2.79:1.31, closer to the peanut oil (Rodrigues et al., Citation2011; Wang et al., Citation2017). The Chinese Nutrition Society recommended the optimum ratio of SFAs, MUFAs and PUFAs in edible oil is 1:1:1, which could be beneficial for preventing and suppressing chronic diseases (Chen, Citation2018). Comparatively with olive oil (1:4:1), the fatty acid composition of CSSOs were closer to the recommended value (1:1:1) (Rodrigues et al., Citation2021). It have already proved that CSSOs may have the potential for alleviating the cognitive dysfunction and nerve damage (Kim et al., Citation2019). Furthermore, due to the reasonable fatty acid composition, CSSOs can be used as high-quality raw materials for biodiesel (Aydin & Caliskan, Citation2020; Demirbas & Kinsara, Citation2017). Recently, CSSOs have attracted more and more attention from consumers and scholars (Xu et al., Citation2021; Liu et al., Citation2022; Mei et al., Citation2022; Xiang et al., Citation2022).
The fatty acid composition and content of vegetable oil is greatly influenced by crop species and cultivar (Aranda et al., Citation2004; Sabzalian et al., Citation2008; Kaplan et al., Citation2019, Zeng and Endo, Citation2019; Nkoi et al., Citation2021). For example, the contents of oleic acid (C18:1Δ9c) in the oil of safflower (Carthamus tinctorius) conventional cultivar “Rancho” was around 16–20%, while it reached to 87–89% for special cultivar “UC-1” (Fernandez-Martinez et al., Citation1993; Sabzalian et al., Citation2008). For tea oil tree (C. oleifera Abel), the content of oleic acid (C18:1Δ9c) in the oil of “Ganzhouyou-1” cultivar was 76.3%, significantly lower than that in “Changlin-40” cultivar (80.3%) (Zeng and Endo, Citation2019). There are plenty of fine tea cultivars in China. However, in order to meet the changing demands of market, new cultivars have been continuously bred, including albino cultivars (Li et al., Citation2020; Wang et al., Citation2019). Leaves of albino cultivars become bleaching compared to conventional green leaves, such as “Anji Baicha”, “Zhonghuang 1”, “Zhonghuang 2”, “Suyuhuang” and so on (Wang et al., Citation2020). We wonder, are there significant differences in the composition and contents of fatty acids in seeds of different albino cultivars, or compared to conventional cultivars? In this study, the fatty acid composition and contents in seeds of several conventional cultivars and albino cultivars were determined and compared, the relative activity of two important fatty acid desaturases was evaluated as well. Our work will be useful for future commercial oil production and tea plant breeding.
2. Material and methods
2.1. Plant materials
In the present study, seeds of 14 tea cultivars (C. sinensis var sinensis) were collected. Among them, 4 albino cultivars “Zhonghuang 1” (ZH No.1), “Zhonghuang 2” (ZH No.2), “Suyuhuang” (SYH) and “Anjibaicha” (AJBC) were with yellow or white tender shoots in early spring, respectively; while 10 conventional cultivars including “Longjing Changye” (LJCY), “Jingfeng” (JF), “Baihaozao” (BHZ), “Wuniuzao” (WNZ), “Fuding Dabaicha” (FD DBC), “TRI-19”, “Niaowang 106” (NW 106), “Yixuan 1” (YX No.1), “Laoshan 3” (LS No.3) and “Yuezaoxiang” (YZX) were with regular green leaves (Kaplan et al., Citation2019; Li et al., Citation2020; Shen et al., Citation2012; Yang et al., Citation2014, Citation2017; Wang et al., Citation2019, Citation2020) (Table ). All tea cultivars were planted in the garden of Xinyang Academy of Agricultural Sciences/Henan Provincial Tea Engineering Researching Center in Henan province in China (114°18’N, 32°9’ E). The soil of this experiment field was sandy clay loam with a pH value of 5.42. The contents of soil nitrogen and soil alkali-hydrolyzable nitrogen were 0.88 g/kg and 80.23 mg/kg, respectively. The content of soil available phosphorus and soil available potassium were 10.94 mg/kg and 84.21 mg/kg, respectively. The content of soil organic matter was 15.90 g/kg. This experimental field was suitable for tea plant cultivation. Fertilizer was applied to tea plant in early March, late May and early September, and base fertilizer was applied around November every year. We also need to timely weeding, irrigation and the prevention and control of pests and diseases. In addition, tea (C. sinensis var. assamica) is another important variety of Sect. Thea, widely cultivated in Tea Area of Southwest China. We wondered whether there were significant differences in the fatty acid composition in seeds between tea plant (C. sinensis) and tea-oil tree (C. oleifera Abel), especially the latter one is considered as an important oil crop of Camellia. Therefore, “Menghai Dayezhong” (MH DYZ) (C. sinensis var. assamica), “Changlin 4” (CL No.4) (C. oleifera Abel) and “Changlin 27” (CL No.27) (C. oleifera Abel) were chosen for comparison. Seeds of 10 individuals from each cultivar were collected in early November 2020 and mixed as one treatment. One cultivar was carried out in triplicates. After being dried completely in a cool and ventilated place, seeds were broken with a hammer and peeled. Then, seed kernels were adequately pulverized and stored at −20℃ in airtight containers for further analysis.
Table 1. Samples information
2.2. Chemicals and reagents
The standard mixture of 37 fatty acid methyl esters and methyl nonadecanoate (internal standard) were purchased from Sigma-Aldrich (St. Louis, MO. USA). Hydrochloric acid, methanol, dichloromethane and petroleum ether were purchased from Sinopharm (Shanghai, China). All chemicals belong to analytical reagent grade.
2.3. Kernel rate of seed
The kernel rate was mainly determined according to GB/T 5499–2008, which is a Chinese national standard and usually used for the determination of kernel rate of crop seed. Briefly, weighed a certain amount of tea seeds (m0, g). After broke their shells with a hammer carefully, weighed seed kernels (m1, g). And the kernel rate of tea seeds (X, %) was calculated according to the following formula:
2.4. Crude fat content of seed
The crude fat content of tea seed was extracted using a Soxhlet extractor according to GB/T5009.6–2016, which is a Chinese national standard and normally applied for the determination of oil content in fat. Briefly, a certain amount of powder (m2, g) was weighed and transferred to a filter paper bag; the bag was placed into the extraction cylinder of a Soxhlet extractor, and connected with a receiving bottle (m0, g); after adding moderate anhydrous ether into the bottle, the extractor was heated on a water bath for 8 h, to make anhydrous ether continuous reflux extraction; removed this bottle and recovered anhydrous ether until about 2 mL of solvent left, subsequently, evaporated the bottle on a water bath; dried the bottle at 100 °C for 1 h, then weighed after cooling down, repeated the operation until constant weight (m1, g). The crude fat contents of tea seed kernels (X, %) were calculated according to the following formula:
2.5. Preparation of Fatty Acid Methyl Ester (FAME)
The main steps were described as follows: 0.2 g powder was accurately weighed and added into a sampling bottle; 5 mL of hydrochloric acid-methanol solution (1:20, v/v) and 10 mL dichloromethane-methanol solution (1:1, v/v) were added orderly; this bottle was heated at 80°C for one hour; after cooling down to room temperature, 5 mL methanol was added; 1 mL of treatment solution, internal standard solution, and 5% ammonia solution were added into a new centrifuge tube; after mixing and centrifuging, the dichloromethane phase was transferred to a new sample vial for further study. The appropriate amount of test solution was filtered by a 0.22 μm membrane before GC-MS analysis.
2.6. Determination of fatty acid composition by GC-MS
The analysis was performed on an Trace1310 ISQ gas chromatography-mass spectrometer (Thermo Fisher Technologies, USA) with a TG-MAX MS column (30 m × 0.25 mm, i.d. 0.25 μm). Nitrogen was used as the carrier gas at a flow rate of 1.0 mL/min. The test solution (1 μL) was introduced via split injection (split ratio of 1:50) with an injector temperature of 250℃. The column temperature was firstly set at 50℃; subsequently increased to 160℃ at a rate of 10℃/min; then increased to 250℃ at a rate of 2℃/min, where it was held for 1 min. The ion source was EI source with 70 eV. The temperatures of ion source and transmission line were 200℃ and 250℃, respectively. The scan range was 30–500 amu. The fatty acid content in the sample (%) was calculated based on the following formula:
Xi, content of fatty acid; Ai, peak area of fatty acid; Cc19, concentration (g/mL) of internal standard solution; Vc19, volume (mL) of internal standard solution; Fi , response factor of each FAME (each FAME responded to a constant); Ac19, peak area of internal standard; m, mass (g) of sample; FFAMEi-Fai, the conversion factor to convert a FAME into certain fatty acid.
2.7. Evaluation of the relative activity of fatty acid desaturases
According to Chen et al. (Citation2021), the relative activity of fatty acid desaturase 2 (FAD2) can be evaluated by the ratio of oleic acid (C18:1Δ9) to linoleic acid (C18:2Δ9,12), while the relative activity of fatty acid desaturase 3 (FAD3) can be determined by the ratio of linoleic acid (C18:2Δ9,12) to linolenic acid (C18:3Δ9,12,15), respectively.
2.8. Statistical analysis
Values were expressed as the mean ± SE. Statistical significance was examined through one-way analysis of variance (ANOVA) and Tukey’s honest significant difference (HSD) post-hoc test at P < 0.05 using the Statistical Package for the Social Sciences (SPSS 20.0) software program. Principal component analysis (PCA) using the Python package was applied to identify the main factors controlling the composition of fatty acids, and hierarchical clustering analysis (HCA) was employed to classify all of the samples according to the composition of fatty acids.
3. Results and discussion
3.1. Kernel rate and oil content
Based on production practices, the kernel rate and oil content of crop seeds are two important indicators for evaluating their development and utilization value (Huang et al., Citation2013). The kernel rates of C. sinensis seeds varied from 40.89% to 62.87% (Table ), which was within the scope of previous reports (33.53–71.60%) (Chang et al., Citation2020; Chen et al., Citation2021). The kernel rates in seeds of JF (60.35%), BHZ (62.65%), WNZ (62.87%), AJBC (61.21%), ZH No.1 (58.91%) and YZX (58.09%) were significantly higher than that of FD DBC (40.89%). Consistent with previous studies (Wang et al., Citation2017), oil contents of C. sinensis seeds were significantly lower than those of C. Oleifera samples. However, the tea tree has a wide planting area in the world and about 750 kg of C. sinensis seeds can be obtained per hectare (Jiang, Citation2021), therefore, a large number of seeds can be harvested every year (Chang et al., Citation2019). The oil contents in seed kernels of 14 tea cultivars ranged from 20.63% to 33.40%. Although there was no significant difference among tea cultivars, WNZ (33.40%), BHZ (30.73%), JF (32.13%) and ZH No.1 (31.03%) shared comparatively higher values. It could be noticed that seeds of conventional cultivars WNZ, BHZ and JF, together with an albino cultivar ZH No.1, were higher not only in the kernel rates but also in the oil contents.
Table 2. The kernel rates of seeds and oil contents of seed kernels from 17 Camellia cultivars
3.2. Fatty acid composition
The fatty acid content and composition in seed kernels of 14 tea cultivars was presented at Table . A total of 22 kinds of fatty acids were identified, which was more than previous studies (Chen et al., Citation2021; Xiang et al., Citation2022). The major fatty acids in Camellia seeds were oleic acid (C18:1Δ9), linoleic acid (C18:2Δ9,12), and palmitic acid (C16:0), respectively (supplementary material 1). The oleic acid (C18:1Δ9) is considered as an important monounsaturated fatty acid (MUFA) in edible oil, which was reported to effectively prevent the atherosclerosis and cancer, promote the development of bone and nervous systems (Kris-Etherto et al., Citation1999). The content of oleic acid (C18:1Δ9) in WNZ seeds was 15.0670%, significantly greater than those in LJCY (9.5315%), LS No.3 (9.1051%) and MHDYZ (9.8160%). The linoleic acid (C18:2Δ9,12) is known as an essential fatty acid for human, which can reduce blood cholesterol content and prevent atherosclerosis (Kinsella et al., Citation1999). As shown in Table , linoleic acid (C18:2Δ9,12) is the most abundant polyunsaturated fatty acid (PUFA) in Camellia seeds. The contents of linoleic acid (C18:2Δ9,12) of LJCY and LS No.3 were 4.9364% and 4.2174%, significantly lower than those of WNZ (7.8203%), ZH No.1 (7.7231%) and ZH No.2 (7.2834%). In this study, we found that the contents of oleic acid (C18:1Δ9) in C. sinensis seeds were significantly lower than those in C. oleifera seeds, while the content of linoleic acid (C18:2Δ9,12) were significantly higher. The trend was consistent with previous studies on C. oleifera seed oils (COSOs) and CSSOs (Wang et al., Citation2017). The palmitic acid (C16:0) is the highest content of saturated fatty acid (SFA) in Camellia seeds. The contents of palmitic acid (C16:0) in WNZ and ZH No.1 seeds were 3.9445% and 3.9400%, respectively, significantly higher than those in LJCY (2.3660%) and LS No.3 (2.4781%). Wang et al. (Citation2017) reported that the contents of palmitic acid (C16:0) of COSOs and CSSOs were around 8% and 15%, respectively. It was speculated that the total amount of fatty acids in C. oleifera seeds was obviously higher (Table ), which resulted in the proportion of palmitic acid (C16:0) in COSOs being significantly lower than that in CSSOs. From these major fatty acids, the contents of SFAs and PUFAs in seeds of ZH No.1 seems to be higher, but there was no clear difference between other albino cultivars and conventional cultivars.
Table 3. The contents and composition of fatty acids in seed kernels of 17 Camellia cultivars
As shown in Table , the contents of MUFAs in seed kernels of tea cultivars were closer to each other, while the contents of SFAs and PUFAs were significantly different. The SFAs contents of WNZ and ZH No.1 were 5.0863% and 4.8818%, respectively, significantly greater than those of LJCY (3.1799%), LS No.3 (2.9848%) and MHDYZ (2.9971%). The PUFAs contents of WNZ, ZH No.1 and ZH No.2 were 7.9572%, 7.8446% and 7.3858%, respectively, significantly higher than those in LJCY (5.0257%) and LS No.3 (4.2854%). As shown in Table , there were three PUFAs in Camellia seeds, including linoleic acid (C18:2Δ9,12), linolenic acid (C18:3Δ9,12,15) and eicosapentaenoic acid (EPA, C20:5Δ5,8,11,14,17). The first one is n-6 PUFAs, the latter two belong to n-3 PUFAs. It is well known that EPA (C20:5Δ5,8,11,14,17) plays an important role in immune and inflammatory responses (Kinsella et al., Citation1999). The contents of n-3 PUFAs in seeds of different tea cultivars varied greatly. The contents of n-3 PUFAs in seeds of YZX, WNZ and ZH No.1 were 0.1367%, 0.1369% and 0.1215%, respectively, significantly greater than that in LS No.3 (0.0679%). In addition, the proportion of n-3 PUFAs to total fatty acids in C. sinensis seeds and C. oleifera seeds were 0.47% and 0.46%, respectively, which were similar to previous studies and higher than olive (0.20%) and sunflower (0.29%) (Sahari et al., Citation2004, Wang et al., Citation2017, Zeng & Endo, Citation2019a).
Table 4. The fatty acid contents in seed kernels of 17 Camellia cultivars
As shown in Table , the proportions of SFAs to the total fatty acids (TFAs) in the seed kernels of tea cultivars were similar to each other, while the proportions of MUFAs and PUFAs was significantly different among different varieties. Among them, the ratio of MUFAs to TFAs in seed kernels of ZH No.2 was significantly lower than almost all conventional cultivars and albino cultivar SYH, conversely, the proportion of PUFAs to TFAs in seeds of this albino cultivar was significantly greater. The fatty acid composition in seeds of ZH No.2 seemed to be quite different from other cultivars. The mean ratio of SFAs, MUFAs and PUFAs in seed kernels of conventional cultivars was 1.00:3.01:1.54, similar to the conclusions of early studies (Chang et al., Citation2020; Chen et al., Citation2021; Xiang et al., Citation2022). However, this ratio showed different characteristics among four albino cultivars. These ratios in seeds of AJBC and SYH were 1.00:2.96:1.65 and 1.00:3.18:1.56, respectively, closer to conventional cultivars (1:3.01:1.54). For ZH No.1 and ZH No.2, these values were 1.00:2.71:1.61 and 1.00:2.69:1.85, respectively. Comparatively, the fatty acid composition in seeds of ZH No.1 and ZH No.2 was closer to the optimal ratio of 1:1:1 in edible oil recommended by the Chinese Nutrition Society (Chen, Citation2018). In addition, it is speculated that the occuring mechanisms of ZH No.1 and ZH No.2 may involve in the synthesis and metabolism of fatty acids. Wang et al. (Citation2016) have already proved that protein genes related with lipids transport and metabolism, which expressed differentially in the tender leaves between ZH No.1 and a conventional cultivar “Longjing 43”.
Table 5. The proportion of specific fatty acid in the total fatty acids in seed kernels of 17 Camellia
The intake ratio of n-6 to n-3 fatty acids in the diet recommended by WHO and FAO should be less than 5, which can boost intellectual growth, improve immunity, protect eyesight, prevent cardiovascular disease and senile dementia (Simopoulos, Citation2008). We noticed that the content of n-6 PUFAs was relatively higher in seeds of Sect. Thea cultivars, which resulted in a significantly higher ratio of n-6 to n-3 PUFAs compared to Sect. Oleifera. The ratios of n-6 to n-3 PUFAs in seeds of MHDYZ and YZX were 33.3690 and 47.6390, respectively, significantly lower than those in ZH No.1 (71.1924) and AJBC (72.6274) (Table ). In order to improve the quality of CSSOs, it is necessary to select tea cultivars with the lower ratio of n-6 to n-3 PUFAs as parents in the breeding project, such as YZX and MHDYZ.
Two of multivariate chemometric methods, PCA and HCA, were applied to reveal the relationships on the basis of fatty acid composition and contents (Supplementary material 2). 17 Camellia cultivars were clustered into three groups over a limited range in PCA, with two principal components accounting for 99.8%: group 1 consisted of C. oleifera cultivars and was far apart from of all tea cultivars, which was consistent with previous studies (Wang et al., Citation2017; Zeng & Endo, Citation2019b); group 2 partially overlapped with group 3, including LJCY, JF, BHZ, WNZ, NW 106, FD DBC, and SYH; group 3 was composed of AJBC, LS No.3, TRI-19, YX No.1, ZH No.2, ZH No.1, YZX, and MHDYZ. The analysis results of HCA were completely consistent with PCA. The Euclidean distance was used to measure the similarity; Ward’s linkage (clustering to minimize the sum of squares of any two clusters) was the clustering algorithm. Two cultivars of Sect. Oleifera were in a small branch. All tea cultivars were divided into two branches: One branch mainly consisted of conventional cultivars, which corresponded to the group 2 in PCA, the other branch mainly included newly-bred tea cultivars that was consistent with group 3 in PCA.
3.3. Analysis of the relative activity of two important fatty acid desaturases
The conversion of oleic acid (C18:1Δ9) to linoleic acid (C18:2Δ9,12) in the plastid of oil crop is catalyzed by FAD2, while the conversion of linoleic acid (C18:2Δ9,12) to linolenic acid (C18:3Δ9,12,15) is catalyzed by FAD3 (Liu et al., Citation2018; Zhao et al., Citation2015). According to Chen et al. (Citation2021), the ratio of oleic acid (C18:1Δ9) to linoleic acid (C18:2Δ9,12) could evaluated the relative activity of FAD2. The higher the ratio, the lower the activity of FAD2. The ratios of oleic acid (C18:1Δ9) to linoleic acid (C18:2Δ9,12) in C. sinensis samples ranged from 1.44 to 2.58 (Table ). MHDYZ (2.68) and LS No.3 (2.19) owned comparatively higher values. Similarly, the ratio of linoleic acid (C18:2Δ9,12) to linolenic acid (C18:3Δ9,12,15) reflects the relative enzyme activity of FAD3. The smaller the ratio, the greater the activity of FAD3. The ratios of linoleic acid (C18:2Δ9,12) to linolenic acid (C18:3Δ9,12,15) in seeds of tea cultivars varied from 35.52 to 84.42. MHDYZ (35.52) and YZX (52.55) possessed relatively lower values. It is important to breed cultivar with high yield and good quality of tea seeds for developing the industry of tea seed oil. Recently, Jiang et al. (Citation2022) bred a newly-bred cultivar “Jincha 1”, of which the content of n-3 PUFAs in the oil was 1.7%, significantly greater than that of WNZ (0.53%). In this study, we found that YZX and MHDYZ possessed both the lower activity of FAD2 and the higher activity of FAD3, which may be used as ideal parents for breeding projects, to obtain tea cultivar with improved fatty acid composition.
Table 6. The relative activity of FAD2 and FAD3 in seed kernels of 17 Camellia cultivars
4. Conclusion
In our study, the contents and composition of fatty acids in seed kernels of different tea cultivars were compared by GC-MS. A total of 22 fatty acids were identified. The contents of several fatty acids varied significantly among different cultivars. There were three major fatty acids, including oleic acid (C18:1Δ9), linoleic acid (C18:2Δ9,12) and palmitic acid (C16:0). For them, a conventional cultivar WNZ and an albino cultivar ZH No.1 owned comparatively higher values. The ratio of SFAs, MUFAs and PUFAs in seeds of albino cultivar ZH No.2 was 1.00:2.67:1.85, of which the proportion of MUFAs to TFAs was obviously lower than those in conventional cultivars (1:3.01:1.54). It is necessary to analyze the seed chemical components before development and utilization, especially for albino cultivars. In addition, in order to breed new cultivar suitable for processing tea seed oil, appropriate tea cultivars should be selected as parents for improving the fatty acid composition in the breeding project, such as ZH No.2.
Author contributions
Conceptualization, Yali Chang; Methodology, Shuangjie Huang and Jinlei Luo; Software, Zhu Yao; Validation, Mufang Sun and Yi Chen; Investigation, Shuangjie Huang and Yao Zhu; Resources, Shuangfeng Jiang; Data Curation, Yuewei Li; Writing-Original Draft Preparation, Yuewei Li; Writing-Review & Editing, Yali Chang; Supervision, Mufang Sun; Project Administration, Yi Chen; Funding Acquisition, Guiyi Guo and Mufang Sun.
Supplemental Material
Download Zip (122 KB)Disclosure statement
No potential conflict of interest was reported by the author(s).
Supplementary data
Supplemental data for this article can be accessed online at https://doi.org/10.1080/23311932.2023.2272464
Additional information
Funding
References
- Aranda, F., Gómez-Alonso, S., Riversa Del Álamo, R. M., Salvador, M. D., & Fregapane, G. (2004). Triglyceride, total and 2-position fatty acid composition of cornicabra virgin olive oil: Comparison with other Spanish cultivars. Food Chemistry, 86(4), 485–15. https://doi.org/10.1016/j.foodchem.2003.09.021
- Aydin, F., & Caliskan, S. (2020). Evaluation of biodiesel produced from tea seed oil in terms of fatty acid components. International Journal of Energy Applications and Technologies, 7(1), 13–19. https://doi.org/10.31593/ijeat.699439
- Chang, Y. L., Huang, X. B., Jiang, S. F., Huang, S. J., Sun, M. F., Liu, W., & Guo, G. Y. (2020). Analysis of fat content and fatty acid composition in seeds of southern Henan tea germplasms. Journal of Food Safety and Quality, 40, 352–362. https://doi.org/10.13305/j.cnki.jts.2020.03.006
- Chang, Y. L., Huang, S. J., Liu, W., Chen, Y., Zhang, Y. R., & Guo, G. Y. (2019). Research progress of tea camellia seed oil. Journal Chinese Cereal Oil Ass, 34, 138–146. https://doi.org/10.3969/j.issn.1003-0174.2019.12.022
- Chen, C. (2018). The imbalance of fatty acids leads to a health crisis - global nutrition experts suggested that it is a convenient way to choose the blended oil with balanced fatty acids. China Oils & Fats, 43, 3–4. https://doi.org/CNKI:SUN:ZYZZ.0.2018-06-002
- Chen, M. J., Du, Z. H., & Qin, J. H. (2021). Analysis of characteristics of lipid metabolism of different germplasm tea seeds. Journal Tea Science, 41, 350–360. https://doi.org/10.13305/j.cnki.jts.2021.03.004
- Demirbas, A., & Kinsara, R. A. (2017). Cost analysis of biodiesel from kernel oil of tea seed. Energy Sources, Part B: Economics, Planning, & Policy, 12(5), 480–486. https://doi.org/10.1080/15567249.2016.1198846
- Fernandez-Martinez, J. M., Rio, M. D., & Haro, A. D. (1993). Survey of safflower (carthamus tinctorius L.) germplasm for variants in fatty acid composition and other seed characters. Euphytica, 69(1), 115–122. https://doi.org/10.1007/BF00021734
- Hibasami, H., Komiya, T., Achiwa, Y., Ohnishi, K., Kojima, T., Nakanishi, K., Akashi, K., & Hara, Y. (1998). Induction of apoptosis in human stomach cancer cells by green tea catechins. Oncology Reports, 5, 527–536. https://doi.org/10.3892/or.5.2.527
- Huang, J., Ahrends, A., He, J., Gui, H., Xu, J., & Mortimer, P. E. (2013). An evaluation of the factors influencing seed oil production in Camellia reticulata L. plants. Industrial Crops & Products, 50, 797–02. https://doi.org/10.1016/j.indcrop.2013.08.019
- Jiang, C. J. (2021). Breeding science of tea plant (3rd ed.). China Agriculture Press.
- Jiang, L., Li, D. J., Yuan, M. A., Hu, X. R., Zhao, D. X., Kong, X. J., Weng, X. X., Chen, B., Fu, H. W., & Zheng, Z. S. (2022). Comparative physiological and metabolomic analyses reveal the different biological characteristics between two tea cultivars. Russian Journal of Plant Physiology, 69(1), 16. https://doi.org/10.1134/S1021443722010071
- Kaplan, M., Tutar, H., Kardes, Y. M., Das, A., & Kokten, K. (2019). Fatty acid compositions of the seeds of different sanguisorba minor genotypes. Progress in Nutrition, 21, 868–870. https://doi.org/10.23751/pn.v21i4.6986
- Kim, J. M., Park, S. K., Kang, J. Y., Park, S. B., Yoo, S. K., Han, H. J., Cho, K. H., Kim, J. C., & Heo, H. J. (2019). Green tea seed oil suppressed Aβ1–42-induced behavioral and cognitive deficit via the Aβ-Related akt pathway. International Journal of Molecular Sciences, 20(8), 1865. https://doi.org/10.3390/ijms20081865
- Kinsella, J. E., Lokesh, B., & Stone, R. A. (1999). Dietary n-3 polyunsaturated fatty acids and amelioration of cardiovascular disease: Possible mechanisms. The American Journal of Clinical Nutrition, 52(1), 1–28. https://doi.org/10.1093/ajcn/52.1.1
- Kris-Etherto, P. M., Pearson, T. A., Wan, Y., Hargrove, R. L., Moriarty, K., Fishell, V., & Etherton, T. D. (1999). High–monounsaturated fatty acid diets lower both plasma cholesterol and triacylglycerol concentrations. The American Journal of Clinical Nutrition, 70(6), 1009–1015. https://doi.org/10.1093/ajcn/70.6.1009
- Liu, H., Li, H. F., Gu, J. Z., Deng, L., Ren, L., Hong, Y. B., Lu, Q., Chen, X. P., & Liang, X. Q. (2018). Identification of the candidate proteins related to oleic acid accumulation during peanut (Arachis hypogaea L.) seed development through comparative proteome analysis. International Journal of Molecular Sciences, 19(4), 1235. https://doi.org/10.3390/ijms19041235
- Liu, G. Y., Zhu, W. Q., Li, S., Zhou, W. L., Zhang, H. J., Wang, J., Liu, X. F., Zhang, J. X., Liang, L., & Xu, X. (2022). Antioxidant capacity and interaction of endogenous phenolic compounds from tea seed oil. Food Chemistry, 376, 131940. https://doi.org/10.1016/j.foodchem.2021.131940
- Li, Q., Xiang, J., Zheng, G. Q., & Wei, K. (2020). Advance of the breeding, extension and industrialization of tea cultivars with special colors in China. Chi Tea, 42, 52–57. https://doi.org/10.3969/j.issn.1000-3150.2020.09.015
- Mei, L., Ji, Q. Y., Jin, Z., Guo, T. T., Yu, K., Ding, W. L., Liu, C. J., Wu, Y. T., & Zhang, N. N. (2022). Nano-microencapsulation of tea seed oil via modified complex coacervation with propolis and phosphatidylcholine for improving antioxidant activity. LWT, 163, 113550. https://doi.org/10.1016/j.lwt.2022.113550
- Nkoi, V., de Wit, M., Fouche, H., Coetzer, G., & Hugo, A. (2021). The effect of nitrogen fertilization on the yield, quality and fatty acid composition of Opuntia ficus-indica seed oil. Sustainability, 13(18), 10123. https://doi.org/10.3390/SU131810123
- Rodrigues, N., Casal, S., Pinho, T., Cruz, R., Peres, A. M., Baptista, P., & Pereira, J. A. (2021). Fatty acid composition from olive oils of Portuguese centenarian trees is highly dependent on olive cultivar and crop year. Foods, 10(3), 496. https://doi.org/10.3390/foods10030496
- Rodrigues, A. C., Ströher, G. L., Freitas, A. R., Visentainer, J. V., Oliveira, C. C., & de Souza, N. E. (2011). The effect of genotype and roasting on the fatty acid composition of peanuts. Food Research International (Ottawa, Ont), 44(1), 187–192. https://doi.org/10.1016/j.foodres.2010.10.042
- Sabzalian, M. R., Saeidi, G., & Mirlohi, A. (2008). Oil content and fatty acid composition in seeds of three safflower species. Journal of the American Oil Chemists’ Society, 85(8), 717–721. https://doi.org/10.1007/s11746-008-1254-6
- Sahari, M. A., Ataii, D., & Hamedi, M. (2004). Characteristics of tea seed oil in comparison with sunflower and olive oils and its effect as a natural antioxidant. Journal of the American Oil Chemists’ Society, 8(6), 585–588. https://doi.org/10.1007/s11746-006-0945-0
- Sengupta, C., Sengupta, A., & Ghosh, A. (1976). Triglyceride composition of tea seed oil. Journal of the Science of Food and Agriculture, 27(12), 1115–1122. https://doi.org/10.1002/jsfa.2740271206
- Shen, Y. H., Tang, C. Q., Zhou, J. F., Xu, D. L., & Mei, J. F. (2012). Study on the breeding of a new tea cultivar ‘Suyuhuang’. Chinese Tea, 34, 21–23. https://doi.org/10.3969/j.issn.1000-3150.2012.06.011
- Simopoulos, A. P. (2008). The importance of the omega-6/omega-3 fatty acid ratio in cardiovascular disease and other chronic disease. Experimental Biology and Medicine, 233(6), 674–688. https://doi.org/10.3181/0711-MR-311
- Wang, L., Cao, H. L., Chen, C. S., Yue, C., Hao, X. Y., Yang, Y. J., & Wang, X. C. (2016). Complementary transcriptomic and proteomic analyses of a chlorophyll-deficient tea plant cultivar reveal multiple metabolic pathway changes. Journal of Proteomics, 130, 160–169. https://doi.org/10.1016/j.jprot.2015.08.019
- Wang, X. C., Wang, L., Hao, X. Y., Zeng, J. M., & Yang, Y. J. (2019). Progress of tea genetic breeding study in 40 years of China. Chinese Tea, 41, 1–6. https://doi.org/10.3969/j.issn.1000-3150.2019.05.001
- Wang, X. Q., Zeng, Q. M., Verardo, V., & Del Mar Contreras, M. (2017). Fatty acid and sterol composition of tea seed oils: Their comparison by the “FancyTiles” approach. Food Chemistry, 233, 302–310. https://doi.org/10.1016/j.foodchem.2017.04.110
- Wang, L. J., Zeng, Q. M., & Wang, X. Q. (2017). Research development of Camellia seed oil from three sections. Journal Chinese Cereal Oil Ass, 32, 165–171. https://doi.org/10.3969/j.issn.1003-0174.2017.11.027
- Wang, L. Y., Zhao, R. B., Cheng, H., Wei, K., Ruan, L., & Wu, L. Y. (2020). Current situation on breeding of tea cultivars with special leaf colors. Chinese Tea, 42, 15–19. https://doi.org/10.3969/j.issn.1000-3150.2020.01.004
- Wei, K., Wang, L. Y., Zhang, Y. Z., Ruan, L., Li, H. L., Wu, L. Y., Xu, L. Y., Zhang, C. C., Zhou, X. G., Cheng, H., & Edwards, R. (2019). A coupled role for CsMYB75 and CsGSTF1 in anthocyanin hyperaccumulation in purple tea. The Plant Journal: For Cell and Molecular Biology, 97(5), 825–840. https://doi.org/10.1111/tpj.14161
- Xiang, J., Liang, Y. R., Zhao, D., Wang, K. R., Lu, J. L., Yuan, M. A., & Zheng, X. Q. (2022). Variation of oil content in tea seed kernel and fatty acid compositions and contents in tea seed oil among different cultivars and regions. Journal Tea Science, 42, 233–248. https://doi.org/10.13305/j.cnki.jts.2022.02.008
- Xu, Z. G., Cao, Z. R., Yao, H. R., Li, C. Y., Zhao, Y. L., Yuan, D. Y., & Yang, G. Y. (2021). The physicochemical properties and fatty acid composition of two new woody oil resources: Camellia hainanica seed oil and Camellia sinensis seed oil. CYTA - Journal of Food, 19(1), 208–211. https://doi.org/10.1080/19476337.2021.1879936
- Yang, Y. J., Chen, M., Wang, X. C., Xi, Y. Z., Yu, F. L., Ye, W. G., Xu, L. M., Jin, X., Chen, J., & Bai, K. Y. (2014). Study on the breeding of a new fine tea cultivar ‘Zhonghuang 1’ with special color. Chinese Tea, 36, 8–9+17. https://doi.org/10.3969/j.issn.1000-3150.2014.09.004
- Yang, Y. J., Hu, X. L., Wang, X. C., Xu, K. X., Yu, F. L., Yang, G. Y., Li, Q., Su, L. Y., Bai, K. Y., & Chen, J. X. (2017). Study on the breeding of a new tea cultivar ‘Zhonghuang 2’ with special color. Chinese Tea, 39, 25–26. https://doi.org/CNKI:SUN:CAYA.0.2017-09-013
- Zeng, W., & Endo, Y. (2019a). Effects of cultivars and geography in China on the lipid characteristics of camellia oleifera seeds. Journal of Oleo Science, 68(11), 1051–1061. https://doi.org/10.5650/jos.ess19154
- Zeng, W., & Endo, Y. (2019b). Lipid characteristics of camellia seed oil. Journal of Oleo Science, 68(7), 649–658. https://doi.org/10.5650/jos.ess18234
- Zhao, N., Zhang, Y., Li, Q. Q., Li, R. F., Xia, X. L., Qin, X. W., & Guo, H. H. (2015). Identification and expression of a stearoyl-ACP desaturase gene responsible for oleic acid accumulation in xanthoceras sorbifolia seeds. Plant Physiology & Biochemistry, 87, 9–16. https://doi.org/10.1016/j.plaphy.2014.12.009