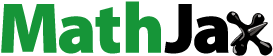
Abstract
This study focused on the nematicidal potential of sea urchin-like zinc oxide nanometrics (SUZN) against the root-knot nematode Meloidogyne incognita. XRD, SEM, UV-vis spectroscopy, TEM, and EDX have been utilized to characterize the SUZN. Various SUZN concentrations (150–750 ppm) were examined for their implications for M. incognita second-stage juveniles (J2s) and egg masses, including mortality as well as hatching inhibition. The largest J2s hatching inhibition (83.07%) was seen at 750 ppm SUZN after 48 hours of incubation, whereas the lowest hatching occurred at 150 ppm when compared to the control. SUZN illustrated increased nematicidal efficiency with increasing concentration and incubation duration. LC50 values for J2s were calculated at various time points. In root-dip trials, all SUZN doses substantially reduced J2s penetration into eggplant roots relative to the untreated control. The study emphasizes SUZN’s strong nematicidal activity against M. incognita, suggesting its promise as a long-term alternative to chemical nematicides for root-knot nematode management while reducing the environmental concerns associated with traditional pesticides.
Reviewing Editor:
1. Introduction
Nanotechnology involves studying development, analysis and implementation of materials with dimensions ranging from 1 to 100 nanometers (Baig et al., Citation2021). The atomic scale of these elements results in a heightened surface area-to-volume ratio, distinct from bulky materials (Khan et al., Citation2019). These nanoscale materials exhibit unique biological, chemical and physical properties, which offer various anomalies and roles (Pandya & Shukla, Citation2018; Titus et al., Citation2019). Due to these exceptional attributes, nanotechnology has emerged as a state-of-the-art technology with vast and practical applications in diverse fields, including agri-businesses, cosmetology functions, forensic areas, electronic sciences, bio-medicine, optics and many more (Mukhopadhyay, Citation2014; Lodha et al., 2016; Nazir, Citation2018).
Zinc oxide nanoparticles (ZnONPs) have acquired significant attraction as a potential alternative to traditional chemical pesticides due to their antimicrobial, antifungal, and insecticidal properties (Hashem et al., Citation2022; Jameel et al., Citation2020). ZnONPs are influential against different pathogens and pests like bacteria, nematodes, and fungi (Afzal & Mukhtar, Citation2024; Kalaba et al., Citation2021). Recently, using ZnONPs has been an emerging interest in the sustainable and eco-friendly manner to control plant parasitic nematodes, which are responsible for significant yield losses in many crops (Zhao et al., Citation2020).
Meliodogyne incognita is a biotrophic parasite that feeds on the roots of the host plant, causing significant damage to the root system and minimizing the plant’s water and nutrient absorption ability (Jatala, Citation2019). A wide range of more than 3000 host species are affected by some of the most damaging nematodes that harm crops, including M. incognita, M. javanica, M. arenaria, and M. hapla (Elkobrosy et al., Citation2020; Ghareeb et al., Citation2020). This genus has approximately 100 distinct species (Subbotin et al., Citation2021), with M. arenaria, M. hapla, M. incognita, and M. javanica standing out owing to their widespread availability and broad range of hosts (Jones et al., Citation2013). M. incognita symptoms include cracking in store roots caused by changes in water content in the soil throughout root growth, which leads to necrotic lesions (Lawrence et al., Citation1986). These consequences cause internal necrosis and outward galling, lowering the market value of damaged roots and making them unfit for sale (Overstreet, Citation2009).
The use of chemical pesticides to control M. incognita is expensive and leads to environmental contamination, and resistance develops against pesticides in the nematode population. Therefore, a need arises to discover alternative agro-compatible materials for managing the harmful impact of M. Incognita (Balesdent et al., Citation2012). In recent years, various studies have investigated the possible applications of ZnONPs as an alternative to chemical pesticides to control M. incognita (El-Ansary et al., Citation2021). These studies exhibited the potent antagonistic properties of ZnONPs against M. incognita (El-Ansary et al., Citation2021; Yazhiniprabha et al., Citation2019). They demonstrated that ZnONPs inhibit the M. incognita egg hatching and reduce the motility and survival of the nematode larvae. In vivo studies have shown that applying ZnONPs with soil significantly reduces the M. incognita population in the root zone of the host plant (Cardozo et al., Citation2019). Firstly, unlike chemical pesticides, ZnONPs are biodegradable and do not persist in the environment. It reduces the risk of environmental contamination and developing pesticide resistance in the nematode population. Secondly, ZnONPs are generally inexpensive and simple to manufacture, which makes them a financially viable option for small-scale agricultural producers. Thirdly, using ZnONPs does not negatively impact non-target organisms, such as beneficial soil microbes and pollinators, making it an eco-friendly approach.
To the best of the authors’ knowledge, the anti-nematode effectiveness of sea urchin-like zinc oxide nanometrics (SUZN) generated using the sol-gel technique has not been investigated. Therefore, the impact of various doses of SUZN prepared by the sol-gel process, against the infective stage of juveniles of M. incognita, was evaluated. This work showed SUZN as a possible sustainable and environmentally friendly alternative to conventional pesticides, addressing their limitations while giving a long-term solution for the control of Meloidogyne incognita in eggplants.
2. Materials and methods
2.1. Materials
The Root-knot nematode, Meloidogyne incognita, was chosen as a pathogen because of its worldwide distribution and eggplant, Solanum melongena L. was used as a test plant. E. Merck Ltd. and Loba Chemie, India provided the zinc acetate dihydrate, capping agent cetyltrimethylammonium bromide (CTAB), and sodium hydroxide. Double-distilled water (DDW) was utilized throughout the study. All remaining chemicals and supplements were purchased from Hi-Media and Sigma-Aldrich.
2.2. Synthesis of of sea urchin-like zinc oxide nanometrics (SUZN)
SUZN was formed under monitored conditions at room temperature with DDW. A 0.15 M solution of zinc acetate dihydrate (18 mL) was blended with DDW (175 mL) using a magnetic stirrer. Cetyltrimethylammonium bromide (CTAB) was then added to the solution as a capping agent, keeping the concentration at 0.0015 moles while swirling constantly. To put an end to the reaction, ∼ 0.9 g of sodium hydroxide were slowly added to the solution. The reaction mixture was then incubated for just over an hour until it turned white and cloudy. The resulting solution was then poured into centrifuge chambers and centrifugation run is carried out at high rpm. The resulting pellet was then stored at room temperature for more than a month. The ensuing product was purified by washing it with DDW and absolute ethanol (99.8%) before being placed in storage with suitable labelling for subsequent characterization.
2.3. Characterization of SUZN
2.3.1. Experimental Analysis techniques
The X-ray diffraction analysis of synthesized sea urchins like Zinc oxide nanometrics (SUZN) was conducted using an AXD D8 ADVANCE BRUKER X-ray diffractometer manufactured in Germany. The instrument utilized nickel-filtered copper Kα radiation with a wavelength of 1.54 Å. The scanning process involved step dimensions of 0.01° and a scanning speed of 0.02 steps per second. The power production settings were configured to 40 kV and 40 mA. To determine the size of the nanoparticles, the Debye-Scherer formula (Brown, Citation2012; Xie et al., Citation2009) was employed, as shown below:
(1)
(1)
where:
D = Crystallite size
k = Proportionality constant (0.9).
λ = X-ray wavelength (1.54178 Å).
β = Full Width at Half Maximum (FWHM) of XRD peaks.
θ = Bragg’s angle.
The Diffrac Plus software tool was used to streamline the computing procedure. A transmission electron microscope (TEM) with ultra-high resolution and fast data collecting capabilities was used to analyze the size of SUZN crystals. Lyophilized SUZN samples were suspended in 20 mM phosphate-buffered saline (PBS) at a pH of 7.4. Using a sputter coater, a drop of the nanoparticles was applied to a transparent glass shaft, allowed to air dry, and then coated with a gold-palladium composite material. The imaging method used a 200 kV accelerating voltage (Khan et al., Citation2020).
The surface morphology of the SUZN was examined using scanning electron microscopy (SEM). The main details are:
A JEOL JSM-6510 LV SEM was utilized to analyze the surface characteristics of SUZN nanoparticles.
SEM examination measured the shape and diameter-to-length ratio of SUZN nanoparticles.
The SEM method allowed for thorough analysis of the surface morphology, shape, and size distribution of the SUZN nanoparticles. The study was conducted in collaboration with Oxford’s energy dispersive spectroscopy (EDS) and SEM. The microscope utilized a 5 kV acceleration voltage and a 10 μA current. A gold sputter coating was applied to enhance the samples’ viability (Khan et al., Citation2014).
The UV-Vis absorption spectroscopy for SUZN was performed with a twin-beam PERKIN-ELMER spectrophotometer in the 300–600 nm range with 1 nm resolution. Measurements were obtained in a quartz cuvette with a 1 cm path length, using DDW as the standard for background correction (Kathirvelu et al., Citation2009).
2.4. Collection and multiplication of J2s of M. incognita
Perineal pattern analysis was used to identify M. incognita from eggplant roots infected with Meloidogyne species that were collected from the campus of Aligarh Muslim University in India (Karssen et al., Citation2013). Second-stage juveniles (J2s) of M. incognita were cultivated in eggplant roots under controlled glasshouse conditions to conduct antinematode activity studies. From the harvested roots of infected eggplant, egg masses were handpicked using sterilized forceps and placed into 25 mm pore-sized sieves. Sieves immersed in water-filled petri plates assisted the hatching of second-stage infective juveniles (J2). Petri plates with egg masses were put in a biochemical oxygen demand (BOD) room at 28 °C for J2s to hatch. The sieves contained the egg masses while enabling hatched J2s to flow through to the plate surface. The hatching J2s were then collected for additional processing and investigation.
2.5. J2s mortality test
The nematicidal action of different concentrations (150, 300, 450, 600, 750 ppm) of SUZN against J2s was performed, and values of 50% lethal concentration (LC50) were determined for all the treatments. To observe the impact of SUZN on J2s mortality, 2 mL DDW containing 65 J2s was poured in 8 mL of different concentrations of SUZN within a Petri plate. Petri plates containing DDW were considered as control. Parafilm was used on the Petri plates to prevent evaporation. After 06, 12, 24, and 48 hours of exposure time, the mortality of J2s was measured with the help of a stereoscopic microscope. Each treatment was performed five times (). El-Rokiek and El-Nagdi (Citation2011) recognized live J2s based on their movement or zigzag behavior, but Caboni et al. (Citation2013) identified dead J2s as having no activity. The mortality data underwent probit analysis to estimate the LC50 values for each therapy, as described by Sakuma (Citation1998) and Behreus and Karbeur (Citation1953). The percent mortality of each treatment was determined separately using the following formula.
2.6. J2s hatching analysis
The egg mass dipping method was used to determine the inhibitory effect of different SUZN concentrations (150, 300, 450, 600, and 750 ppm) on J2s hatching. Six egg masses were carefully removed from diseased eggplant roots using sterilized forceps and put on Petri plates with 8 mL of SUZN solutions. Petri plates were sealed with parafilm to avoid evaporation and incubated at 28 °C. Control plates with egg masses in DDW were also made. Each treatment was performed five times (). After four days, the number of hatched J2s was counted with a binocular microscope. The percentage inhibition of J2 hatching from egg masses was determined using the formula presented by Almutairi et al. (Citation2022).
2.7. J2 penetration analysis
A 7 cm disposable teacup contained 40 g of washed river sand combined with 1 mL of different SUZN concentrations. Two-week-old brinjal seedlings (cv-BR-112) were then put in the cups. Each cup received 50 freshly hatched second-stage juveniles of M. incognita, which were inserted through 3–4 holes in the teacup without damaging the root structure. The moisture of the cups was maintained throughout the test of J2s bioassay. After 3–4 days of inoculation, eggplants were uprooted carefully, and the J2s penetration was observed. Before observation, the eggplant roots were placed on paper to soak extra water and then sliced these roots into small pieces of about 2 mm. A modified acid-fuchsin staining-destaining procedure utilizing sodium hypochlorite (NaOCl) as a pre-staining treatment for nematode-infected plant tissues was adopted to detect J2s (Bybd et al., Citation1983). Finally, sliced roots were kept on the glass slide to observe and count the penetrated J2s under a stereo microscope.
2.8. SEM analysis for meloidogyne species identification
Meloidogyne incognita species were identified using scanning electron microscopy (SEM), and the perineal pattern was characterized. A mature female specimen was obtained from an infected eggplant root and evaluated using the procedure outlined by Abrantes and Santos (Citation1989). SEM examination was performed on a Jeol JSM 6510 LV microscope from Japan, which had a 14 nm gold coating added to the perineal pattern for imaging. The study sought to characterize Meloidogyne species using morphological traits discovered in M. incognita’s perineal pattern. There was a conspicuous dorsal arch with the normal pyriform shape, as well as an angularly oval construction. Distinct striae usually wavy and lateral field weakly demarcated by forked striae. The ventral side’s striae seemed straighter and oval-shaped ().
2.9. Statistical analysis
The data was statistically analyzed using R (version 2.14.1) with the Duncan Multiple Range Test (DMRT) and standard error (±SE) of the mean. Furthermore, ANOVA was performed, and the LC50 value for each treatment was calculated using OPSTAT, as described by Panse and Sukhatme (Citation1954).
3. Results and discussion
The literature reported the effective generation of flower-shaped zinc oxide nanoparticles (ZnONPs) at varied temperatures, utilizing a simple sol-gel synthesis approach that did not need specialized equipment. Furthermore, the study exploring the effect of physical agitation on the morphology of ZnONPs, which resulted in the development of thorn-like structures has also been published (Khan et al., Citation2014, Citation2016, Citation2020). Apart from that, various preparation techniques, including spray-pyrolysis, microwave-assisted technique, hydrothermal technique, and water-assisted synthesis, have been reported in the scientific literature (Gonullu et al., Citation2024; Wojnarowicz et al., Citation2020; Wirunchit et al., Citation2023; Saloga et al., Citation2022) for producing ZnONPs in different shapes and sizes (). In the latest study, the sol-gel approach to create two-dimensional SUZN crystals under regulated centrifugation run and temperature conditions has been conducted. This study focuses on developing SUZN crystals and testing their effectiveness against M. incognita infection and root penetration severity in host plants. Furthermore, the emerging results showed that SUZN caused mortality in J2s and reduced their capacity to enter roots.
Table 1. Comparison of sea urchin-like zinc oxide nanometrics (SUZN) unique characteristics with conventionally synthesized ZnONPs.
3.1. X-ray diffraction Analysis of synthesized SUZN
As shown in X-ray diffraction (XRD) was used to assess the crystallinity and phase properties of the synthesized sea urchins like Zinc oxide nanometrics (SUZN). The diffraction arrangement exhibited a perfect match with the standard peaks listed in the International Centre for Diffraction Data (ICDD) database (card number: 080-0075). The analysis confirmed the presence of a hexagonal wurtzite crystal structure in the SUZN samples, with lattice parameters a & b = 3.24 and c = 5.20, resulting in a c/a ratio of 1.60. The XRD arrangement indicated that the SUZN were obtained through a single-step synthesis process. The characteristic form of the peaks indicated the samples’ small dimensions and high crystallinity level. Notably, no further peaks were detected, indicating that the generated SUZN was devoid of contaminants (Khan et al., Citation2014). The peak corresponding to the (101) crystallographic orientation exhibited the highest intensity at a 2θ angle of 36.2° (Khan et al., Citation2016). Furthermore, the XRD pattern supported the presence of a pure wurtzite structure, as evidenced by the peaks corresponding to the (100), (002), (102), (110), and (103) crystallographic planes, which aligned with the ICDD card no. 080-0075. Different crystal lattice configurations, including wurtzite, cubic zinc-blende, and rock salt, are known to exist for ZnONPs (Amalraj et al., Citation2015; Djurišić et al., Citation2012). According to Morkoç and Özgür (Citation2008), the wurtzite structure of ZnONPs, with its hexagonal unit cell, is non-centrosymmetric and belongs to the space group P63mc in the Hermann-Mauguin systems and C46v in the Schoenflies notation, both of which lack an inversion center. This thermodynamically stable structure is tetrahedral, with four oxygen atoms encircling Zn atoms and vice versa (Amalraj et al., Citation2015; Mohd Adnan et al., Citation2016).
3.2. X-ray Dispersive Energy (EDX) spectrometry analysis of synthesized SUZN
depicts the use of EDX to assess the chemical composition and quality of the generated SUZN samples. The EDX spectrum confirmed the presence of zinc and oxygen in the SUZN samples, which are quantitatively shown as weight percentages in the figure. The background picture in the spectrum was the electron image of the sample utilized for analysis (Khan et al., Citation2016). It is worth mentioning that the occurrence of other peaks, in addition to the Zn and O peaks, may be due to the substrate on which the SUZN samples were mounted during the instrumental examination.
3.3. Scanning Electron Microscopy (SEM) Analysis of synthesized SUZN
SEM analysis revealed the presence of synthesized SUZN, as shown in . The sol-gel technique employed in the synthesis resulted in SUZN with sizes and lengths resembling long spines of sea urchin-like structures. Notably, the extensive lengths of the nanoparticles in comparison to their diameters indicated high aspect ratios, portraying the elongated rod-like shape of the SUZN. The morphology obtained was in agreement with earlier research and was attained by applying 0.15 M gm of zinc acetate precursor in a planned centrifugation run after synthesis at a steady temperature (Wang et al., Citation2017).
3.4. TEM analysis of synthesized SUZN
TEM analysis further elucidated the structural characteristics of SUZN samples, as depicted in . The investigation revealed the presence of a wide range of SUZN single crystals exhibiting a distinctive two-dimensional (2D) shape. The nanoparticles created frames resembling long sea urchin spines, and the elongated geometry of the pointed rods revealed the formation of SUZN's distinctive 2D lattice structure.
3.5. UV-Vis absorption spectroscopy analysis of synthesized SUZN
UV-vis absorption spectroscopy was used to determine the optical characteristics of SUZN samples. To account for background inaccuracies, DDW was utilized as the reference material. A distinct absorption band, characterized by its broad, compact, and sharp nature, was observed at approximately 357 nm in the UV-A region (). The absorption band was notable for its absence of extra peak locations, which suggests the existence of a pure hexagonal wurtzite ZnO structure. This blue shift in λmax during the specified centrifugation run at constant temperature, was attributed to an increase in the aspect ratios of the SUZN, which also influenced their absorbance. These results are in line with other studies that show peak shifts are influenced by solvent type, process temperature, crystal size and shape, and other sample-related factors (Jung et al., Citation2023; Khan et al., Citation2020). Furthermore, keeping a constant temperature while prolonging the centrifugation run after synthesis resulted in a significant increase in absorption peak intensities.
3.6. Characterization of two-dimensional crystal morphology of synthesized SUZN
The measurements of length (l) and diameter (d) were conducted to determine the desired l/d ratios associated with these unique crystals. The SEM pictures provided visual evidence of the variable nature of these ratios, which depended on the synthesis parameters employed during SUZN production (Cheng et al., Citation2006). It is noteworthy that improving the aspect-ratio identity of the crystals has been found by keeping a constant temperature while prolonging the centrifugation run after synthesis () (Khan et al., Citation2014). This phenomenon can be attributed to the fact that alterations in centrifugation runs while maintaining a constant temperature impact both the length and diameter of the SUZN, albeit to varying extents. Specifically, the reduction in diameter (d) is significantly greater than the reduction in length (l) under these conditions. As a result, the synthesis process yields SUZN with distinct long spines of SUZN.
3.7. Growth behaviour of one-dimensional crystals of synthesized SUZN
The growth of synthesized SUZN in distinct directions, specifically along their different polar surfaces viz. [000I] and [000Ī] having different stabilities and properties (Mora-Fonz et al., Citation2017), gives rise to the formation of nanoparticles frames resembling long spines of sea urchin-like, and the elongated shape of the pointed rods suggested the formation of a distinct 2D lattice structure. SUZN having dimensions suggesting elongated spines of sea urchin-like formations were produced during the synthesis using the chemical-solution deposition technique. This led to the augmentation of the l/d aspect proportions of the SUZN in these particular prompts, as shown in . This preferential growth can be attributed to the presence of capping agent (CTAB) surfactant acting as a stabilizer, which promotes binding predominantly in the [0IĪI] as well as [0IĪ0] projections. As a result, the surfactant-induced amendments in free energy restrict the advancement of growth along these axes (Khan et al., Citation2014, Citation2016). Additionally, although maintaining a steady temperature, this growth tendency continues during an extension in the centrifugation run following the synthesis process at constant temperature. Consequently, a significant disparity in the d/l ratios of the SUZN is observed, resulting in a substantial increase in surface area. This attribute may play an important role in nematicidal action against the root-knot nematode, M. incognita. However, it is worth noting that not all SUZN adhere strictly to this growth trend, as growth along different axes may still occur.
3.8. Effects on J2s mortality and egg hatching
J2s mortality caused by SUZN was studied using the direct-contact method. It was noticed that different concentrations (150, 300, 450, 600, and 750 ppm) revealed significant variances in J2s mortality of M. incognita, with all doses exhibiting some amount of toxicity against J2s. exhibited substantial differences in J2s mortality of M. incognita; with all the concentrations showing some level of toxicity against J2s. The mortality rates of J2s were higher upon increasing the SUZN concentrations as well as exposure time. The 750-ppm concentration was observed to be highly toxic for the J2s at 48 h of the incubation period, and this concentration was killing significantly more J2s compared with the other concentrations. Similarly, the duration of the incubation time substantially influences the J2s percent mortality, reaching a maximum at 48 hours incubation period. Furthermore, 150 ppm dosage resulted in significantly higher J2s mortality contrasted with the control (). According to , the LC50 values for SUZN were 273.46, 411.08, 623.80, and 1066.71 ppm at 48, 36, 24, and 12 hours of incubation, respectively, based on the dose-response probabilistic model (PROBIT) evaluation of juvenile motility. The selection of lethal concentrations was informed by the results of the LC50 experiment, which provided preliminary data on concentrations, capable of producing lethal effects.
Table 2. Effect of various concentrations of SUZN on the mortality of J2s of M. incognita over 12, 24, 36 and 48 hours of incubation.
Table 3. Lethal effect concentrations of the SUZN that caused 50% (LC50) mortality of second stage infective juveniles (J2s) of Meloidogyne incognita. Data are means of six replicates.
Findings indicate that all the concentrations of SUZN were found to be highly toxic and caused mortality to J2s, with toxicity ranging from 08% to 54% depending on exposure time and concentration (). Significant findings were achieved in this bioassay when the direct contact approach was used to observe SUZN's prevention of egg hatching. The egg hatching was reduced significantly at all concentrations viz., 150, 300, 450, 600, 750 ppm as compared to the control (). When SUZN concentration increased from 150 to 750 ppm, a corresponding improvement was observed in the inhibition of egg hatching, successively. The maximum inhibition was observed at 750 ppm after five days of incubation, followed by 600, 450, 300, and 150 ppm. The individual inhibition effect of different SUZN concentrations on egg hatching is represented in .
Figure 9. Effect of various concentrations of sea urchins like zinc oxide nanometrics (SUZN) on J2s hatching of M. incognita over 4 days of incubation period. Each value is an average of six replicate. Each bar followed by same letter is not significantly different according to Duncan’s multiple-range test (p ≤ 0.05). [DW- Distilled water (Control); J2s-Second stage juveniles; ppm-parts per million].
![Figure 9. Effect of various concentrations of sea urchins like zinc oxide nanometrics (SUZN) on J2s hatching of M. incognita over 4 days of incubation period. Each value is an average of six replicate. Each bar followed by same letter is not significantly different according to Duncan’s multiple-range test (p ≤ 0.05). [DW- Distilled water (Control); J2s-Second stage juveniles; ppm-parts per million].](/cms/asset/3788c151-f9ae-4d51-be4a-8ce3a9d4c87d/oafa_a_2366396_f0009_c.jpg)
Based on the obtained results of J2s mortality and egg hatching inhibition, the current study proved that all SUZN viz., 150, 300, 450, 600, and 750 ppm significantly exhibited impact. However, 750 ppm had a significant impact on egg hatching and resulted in the highest J2s mortality. It was determined that increasing the exposure time increased SUZN's nematicidal behavior. According to Ch et al. (Citation2019), the J2s mortality of M. incognita was caused by varying quantities of copper (Cu) nanoparticles (NPs). The results of this study are consistent with those of Akhter et al. (Citation2020), who reported that Cu NPs had comparable effects on the J2s and egg hatching of the root-knot worm M. incognita. The nematotoxic role of SUZN against M. incognita can occur by various mechanisms, such as disrupting several cellular mechanisms, membrane permeability, oxidative stress response, and synthesis of ATP (Ellegaard-Jensen et al., Citation2012; Kim & Ryu, Citation2013; Roh et al., Citation2012). Validation regarding the mortality of J2s can disrupt the nematode’s cellular organization, which is induced by the application of nanoparticles. The consequence of the current research investigations reported that the synthesized SUZN against root-knot nematodes could be used directly or indirectly to cause crop yield loss.
3.9. J2s penetration in SUZN treated eggplant seedlings
The investigation demonstrated that different SUZN concentrations inhibited M. incognita J2 penetration to variable degrees when compared to the control. A dosage of 750 ppm significantly reduced J2 penetration in eggplant roots, whereas 150 ppm exhibited modest inhibition. Concentrations of 300, 450, and 600 ppm all dramatically reduced J2 penetration. The inhibitory impact increased at larger doses, showing SUZN's nematostatic and nematicidal qualities, which are promising for long-term M. incognita management. The time and concentration of SUZN affected J2 penetration inhibition. shows how SUZN inhibits J2 penetration in eggplant roots at various doses, demonstrating its potential for nematode control.
Figure 10. Effect of various concentrations of SUZN on the penetration of J2s of M. incognita in the roots of eggplant over 5 days. Each value is an average of six replicate. Each bar followed by same letter is not significantly different according to Duncan’s multiple-range test (p ≤ 0.05). [DW- Distilled water (Control); J2s-Second stage juveniles; ppm-parts per million].
![Figure 10. Effect of various concentrations of SUZN on the penetration of J2s of M. incognita in the roots of eggplant over 5 days. Each value is an average of six replicate. Each bar followed by same letter is not significantly different according to Duncan’s multiple-range test (p ≤ 0.05). [DW- Distilled water (Control); J2s-Second stage juveniles; ppm-parts per million].](/cms/asset/48c462b8-2013-46bf-a76c-ad536878136d/oafa_a_2366396_f0010_c.jpg)
The study focuses on SUZN's outstanding nematostatic and nematicidal capabilities, which is advantageous for long-term nematode treatment. SUZN-treated seedlings showed less migration of second-stage infective juveniles (J2s) toward roots, probably due to root diffusate obstruction, which draws J2s. This is consistent with Baldwin and Bell’s (Citation1981) findings of diminished J2 attraction to roots when root diffusate is limited. Plant-ingested nanoparticles may trigger defensive systems. Interestingly, this contradicts Applerot et al.’s (Citation2012) results that nanomaterials cause DNA damage through oxidative stress from reactive oxygen species (ROS) and lipid-based peroxides. These opposing findings highlight the complexities of nanoparticle-plant-nematode interactions, implying SUZN's potential for innovative nematode control tactics with low environmental effect.
Nanoparticles disrupt cellular mechanisms, allowing them to pierce the nematode egg wall (Madhuri et al., Citation2010). Taha (2016) and Entsar (Citation2016) found that varying doses (20, 40, 200, 500, and 1500 ppm) of silver nanoparticles induced J2s mortality while inhibiting nematode development (gall and egg production and final population) and egg hatchability. Different doses (50, 100, 175, 200, 250, 300, 350 ppm) of silicon nanoparticles inhibited the percentage of egg hatching and caused J2s mortality of M. incognita at different times of exposure (El-Ashry et al., Citation2021). The leading causes of toxic effects have also been recognized as physical stress-induced structural deformation of cells and damage to the cell plasma membrane associated with nanoparticle cell contacts (Balashanmugam & Kalaichelvan, Citation2015).
Zinc oxide nanoparticles (ZnONPs) have received a lot of interest as a viable alternative to conventional chemical pesticides due to their antibacterial, antifungal, and insecticidal capabilities (Hashem et al., Citation2022; Jameel et al., Citation2020). The specific mechanism by which ZnONPs exhibit antagonistic action against M. incognita remains unknown. However, its anti-nematode potential is thought to be due to a blend of physicochemical and biological mechanisms that impair nematode biological functions, causing them to perish. ZnONPs dissolve in soil, releasing Zn2+ ions (Wang et al., Citation2013). They also undergo chemical alterations such as reduction, oxidation, and sulfidation (Dutta et al., Citation2019). These activities improve the bioavailability along with reactivity of ZnONPs in the surrounding environment (Martins et al., Citation2020; González-Moragas et al., Citation2017). The liberated Zn2+ ions can be harmful to nematodes by impeding active transport and interfering with important enzymatic activities, causing physiological malfunction and cell death (Soren et al., Citation2018; Sávoly et al., Citation2016).
Furthermore, the sharp edges and strong surface reactivity of ZnONPs can substantially disrupt the nematode’s cuticle, increasing porosity and triggering the disappearance of vital nutrients and ions, diminishing the pathogen (Khan et al., Citation2015). ZnONPs are also thought to damage the structural proteins of worm juveniles, causing aberrant appearance and mortality. The disruption with protein structure is critical for worm survival (Kalaba et al., Citation2021).
A further mechanism is the generation of reactive oxygen species (ROS) when ZnONPs penetrate cells, particularly under UV irradiation. ROS molecules include superoxide anion (O2−), hydroxyl ion (OH−), and hydrogen peroxide (H2O2). These reactive species connect to biological components including lipids, proteins, and DNA, resulting in oxidative stress, cellular damage, and, eventually, cell death (Daniel et al., Citation2023; Mosquera-Sánchez et al., Citation2020). ROS production breaks bacterial cell membranes and slows cellular growth, which enhances the anti-nematode action (Elshafie et al., Citation2023; Sirelkhatim et al., Citation2015; Wang et al., Citation2023; Yu et al., Citation2020). Furthermore, ZnONPs have the ability to boost plant immunity, enhancing their defenses against infections. This stimulation involves activating defense-related genes and increasing the synthesis of defensive chemicals including phytoalexins and pathogenesis-related proteins. These chemicals help plants resist nematode attacks more efficiently (Singh et al., Citation2024).
ZnONPs are particularly efficient against nematodes due to their combination of actions, which include direct toxicity, physical damage, disruption of critical biological processes, and improved plant immune responses. However, further study is needed to completely understand the specific mechanisms of action to develop in vivo therapy techniques utilizing biocompatible ZnONPs. Understanding these pathways will help to optimize the use of ZnONPs for long-term and efficient nematode management, perhaps replacing existing chemical pesticides with a lower environmental effect.
4. Conclusions
This study deals with the synthesis and characterization of novel SUZN by employing sol-gel technique. Ensuring a consistent temperature during the extension in the centrifugation run after the synthesis process is essential to sustain the growth tendency of SUZN. SEM analysis revealed the presence of SUZN oriented along different crystallographic projections, predominantly in the [000I] as well as [000Ī] axes. The particles produced large aspect ratios, which had typical diameters between ∼11 and ∼62 nm, whereas the lengths ranged from ∼416 nm to over about 1000 nm. The growth pattern of SUZN showed that the [000I] facets exhibited the highest growth rate. The introduction of the capping agent, CTAB, as a stabilizer altered the free energy and suppressed the growth of [0IĪI] and [0IĪ0] facets. SUZN in multiple dosages had strong nematicidal effects, considerably lowering the overall number of M. incognita second-stage infective juveniles (J2s). The results of the investigation showed that SUZN successfully eradicated J2s, preventing them from hatching from egg masses and infiltrating brinjal seedling roots. Notably, larger amounts of synthesized SUZN showed increased effectiveness. These findings emphasize the potential of produced SUZN as an ecologically benign strategy for managing root-knot nematodes (RKNs) for agricultural use in a sustainable manner. The use of SUZN to manage RKNs, particularly M. incognita, is a potential method due to its reduced genotoxicity when compared to chemical nematicides. The incorporation of SUZN into Integrated Pest management (IPM) techniques provides a safe, cost-effective, and environmentally responsible approach for RKN control. In the future, one can advocate investigating SUZN’s effectiveness across different crops and soil types to determine its broad-spectrum nematicidal action. Furthermore, studying its long-term impacts on soil health and non-target species would offer a thorough knowledge of its environmental impact. Furthermore, performing field experiments to evaluate SUZN's effectiveness in real-world agricultural situations and comparing its performance to traditional chemical nematicides will increase its practical utility.
Author contributions
The authors confirm contribution to the paper as follows: study conception and design – A.K, M.F.K, F.A, M.M; data collection – A.K, S.K, M.M, M.F.K.; analysis and interpretation of results – A.K, S.K, M.M, M.F.K.; draft manuscript preparation – A.K, S.K, M.M, M.F.K., M.F.B.M, S.C.S, P.M, F.A,; revised it critically for intellectual content – M.F.B.M, M.F.K., S.C.S, P.M, F.A. All authors reviewed the results and approved the final version of the manuscript.
Acknowledgement
Dr Mohd. Muddassir is grateful to Researchers Supporting Project number (RSP2024R141), King Saud University, Riyadh, Saudi Arabia, for financial assistance. Dr. Faheem Ahmad acknowledges the support and facility of the Department of Botany, Aligarh Muslim University.
Disclosure statement
No potential conflict of interest was reported by the author(s).
Data availability statement
The authors confirm that the data supporting the findings of this study are available within the article.
Additional information
Notes on contributors
Amir Khan
Amir Khan, is an Assistant Professor at SSLD Varshney Institute of Management & Engineering, Aligarh, India. He published more than 25 scientific papers in the journals of international repute. His research interest includes mycology, plant pathology, biological control, microbial biotechnology, bio-nanotechnology and sustainable nematode management.
Manar Fawzi Bani Mfarrej
Manar Fawzi Bani Mfarrej, received her Ph.D. in Sustainabilityand Environmental Studies in 2010 from the University ofJordan. She is currently working as an Assistant Professor of Environmental Science in the College of Natural and Health Sciences at Zayed University, United Arab Emirates. Dr.Manar’s research interests include air quality, environmental sustainability, plant protection, pesticide residues, environ-mental pollution, and waste management.
Mohd. Farhan Khan
Mohd. Farhan Khan, is an Associate Professor in the Faculty of Science at GCMT, RMPSS University, Aligarh, and an Adjunct Professor in the Department of Chemistry at Maulana Azad University, Jodhpur, Rajasthan, India. He graduated from Aligarh Muslim University, Aligarh. His research interests includes material science, photocatalysis, sensors, and other applied areas.
Shahbaz Khan
Shahbaz Khan, is a research scholar at the National Institute of Technology, Calicut, India. His research focuses on nanomaterials, graphene synthesis, and proton exchange membranes to enhance fuel cell performance.
Peiter Malan
Peiter Malan, is an Associate Professor in Unit for Environmental Sciences and Management at North-West University (Mahikeng Campus), South Africa. He published many scientific papers in the journals of international repute.
Saurabh C. Saxena
Saurabh C. Saxena, is an Assistant Professor in the Department of Biochemistry at Central University of Haryana. His research interest includes functional genomics, stress biology, plant transgenics, and enzymology. He has notable publications and a membership in the Society of Biological Chemists.
Mohd. Muddassir
Mohd. Muddassir is an Assistant Professor in the Department of Chemistry, College of Science at King Saud University, Saudi Arabia. He earned his Ph.D. degree in 2010 from Aligarh Muslim University. Dr. Muddassir has also worked as Postdoctoral Fellow at Nanjing University. His research interest includes supramolecular, bioinorganic chemistry and fluorescence Sensing.
Faheem Ahmad
Faheem Ahmad, is a Senior Assistant Professor in the Department of Botany at Aligarh Muslim University, Aligarh, India. He has also worked as a postdoctoral researcher at Ehime University (Japan), North-West University (South Africa), Ege University (Turkey), and National Sun Yat-Sen University (Taiwan). His research interests include pathogen interaction and disease management, as well as agricultural and nano-biotechnology. He published more than 57 papers in the journal of international repute and holds prestigious fellowships and awards.
References
- Abrantes, I. M. O., & Santos, M. (1989). Technique for preparing perineal patterns of root-knot nematodes for scanning electron microscopy. Journal of Nematology, 21(1), 1–16.
- Afzal, A., & Mukhtar, T. (2024). Revolutionizing nematode management to achieve global food security goals – An overview. Heliyon, 10(3), e25325. https://doi.org/10.1016/j.heliyon.2024.e25325
- Akhter, G., Khan, A., Ali, S. G., Khan, T. A., Siddiqi, K. S., & Khan, H. M. (2020). Antibacterial and nematicidal properties of biosynthesised Cu nanoparticles using extract of holoparasitic plant. SN Applied Sciences, 2(7), 1268. https://doi.org/10.1007/s42452-020-3068-6
- Al-Dhabi, N. A., & Valan Arasu, M. (2018). Environmentally-friendly green approach for the production of zinc oxide nanoparticles and their anti-fungal, ovicidal, and larvicidal properties. Nanomaterials, 8(7), 500. https://doi.org/10.3390/nano8070500
- Almutairi, F. M., Khan, A., Ajmal, M. R., Khan, R. H., Khan, M. F., Lal, H., Ullah, M. F., Ahmad, F., Ahamad, L., Khan, A., Arif, H., & Ayaz Ahmad, M. (2022). Phytochemical analysis and binding interaction of cotton seed cake derived compounds with the target protein of Meloidogyne incognita for nematicidal evaluation. Life, 12(12), 2109. https://doi.org/10.3390/life12122109
- Altammar, K. A. (2023). A review on nanoparticles: Characteristics, synthesis, applications, and challenges. Frontiers in Microbiology, 14, 1155622. https://doi.org/10.3389/fmicb.2023.1155622
- Amalraj, A. S., Dharani, A. P., Inbaraj, P. F. H., Sivakumar, V., & Senguttuvan, G. (2015). Influence of pH on structural, morphological and optical properties of chemically deposited Nanocrystalline ZnO Thin Films. Journal of Materials Science, 26(11), 8877–8886. https://doi.org/10.1007/s10854-015-3568-0
- Applerot, G., Lellouche, J., Lipovsky, A., Nitzan, Y., Lubart, R., Gedanken, A., & Banin, E. (2012). Understanding the antibacterial mechanism of CuO nanoparticles: Revealing the route of induced oxidative stress. Small, 8(21), 3326–3337. https://doi.org/10.1002/smll.201200772
- Baig, N., Kammakakam, I., & Falath, W. (2021). Nanomaterials: A review of synthesis methods, properties, recent progress, and challenges. Materials Advances, 2(6), 1821–1871. https://doi.org/10.1039/D0MA00807A
- Balashanmugam, P., & Kalaichelvan, P. T. (2015). Biosynthesis characterization of silver nanoparticles using Cassia roxburghii DC. aqueous extract, and coated on cotton cloth for effective antibacterial activity. International Journal of Nanomedicine, 10(Suppl 1), 87–97. https://doi.org/10.2147/IJN.S79984
- Baldwin, J. G., & Bell, A. H. (1981). Pararotylenchus n. gen. (Pararotylenchinae n. subfam., Hoplolaimidae) with six new species and two new combinations. Journal of Nematology, 13(2), 111–128.
- Balesdent, M. H., Daverdin, G. G., Gout, L. L., Aubertot, J.N., Pinochet, X. X., & Rouxel, T. T. (2012). Molecular evolution of the AvrLm7 avirulence gene of Leptosphaeria maculans under resistance gene selection in the field is driven by its genomic location, sexual reproduction, and cropping practices. Plant Resistance Sustainability International Conference, Oct 2012, La Collesur Loup, France, pp. 54–54.
- Behreus, A. S., & Karbeur, L. (1953). Determination of LD50. Archiv Fur Experimentelle Pathologie Und Pharmakologie, 28, 177–183.
- Brown, J. G. (2012). X-rays and their applications. Springer Science & Business Media.
- Bybd, D. W., Jr, Kirkpatrick, T., & Barker, K. (1983). An improved technique for clearing and staining plant tissues for the detection of nematodes. Journal of Nematology, 15(1), 142–143.
- Caboni, P., Saba, M., Tocco, G., Casu, L., Murgia, A., Maxia, A., Menkissoglu-Spiroudi, U., & Ntalli, N. (2013). Nematicidal activity of mint aqueous extracts against the root-knot nematode Meloidogyne incognita. Journal of Agricultural and Food Chemistry, 61(41), 9784–9788. https://doi.org/10.1021/jf403684h
- Cardozo, T. R., De Carli, R. F., Seeber, A., Flores, W. H., da Rosa, J. A., Kotzal, Q. S., Lehmann, M., da Silva, F. R., & Dihl, R. R. (2019). Genotoxicity of zinc oxide nanoparticles: An in vivo and in silico study. Toxicology Research, 8(2), 277–286. https://doi.org/10.1039/C8TX00255J
- Ch, G., Ntalli, N., Menkissoglu-Spiroudi, U., & Dendrinou-Samara, C. (2019). Essential metal-based nanoparticles (Copper/Iron NPs) as potent nematicidal agents against Meloidogyne spp. Journal of Nanotechnology Research, 01(02), 44–58. https://doi.org/10.26502/jnr.2688-8521004
- Cheng, B., Shi, W., Russell-Tanner, J. M., Zhang, L., & Samulski, E. T. (2006). Synthesis of variable-aspect-ratio, single-crystalline ZnO nanostructures. Inorganic Chemistry, 45(3), 1208–1214. https://doi.org/10.1021/ic051786a
- Daniel, A. I., Keyster, M., & Klein, A. (2023). Biogenic zinc oxide nanoparticles: A viable agricultural tool to control plant pathogenic fungi and its potential effects on soil and plants. The Science of the Total Environment, 897, 165483. https://doi.org/10.1016/j.scitotenv.2023.165483
- Djurišić, A. B., Chen, X., Leung, Y. H., & Ng, A. M. C. (2012). ZnO nanostructures: Growth, properties and applications. Journal of Materials Chemistry, 22(14), 6526–6535. https://doi.org/10.1039/c2jm15548f
- Dutta, T., Bagchi, D., Bera, A., Das, S., Adhikari, T., & Pal, S. K. (2019). Surface engineered ZnO-humic/citrate interfaces: Photoinduced charge carrier dynamics and potential application for smart and sustained delivery of Zn micronutrient. ACS Sustainable Chemistry & Engineering, 7, 10920–10930.
- El-Ansary, M. S. M., Hamouda, R. A., & Elshamy, M. M. (2021). Using biosynthesized zinc oxide nanoparticles as a pesticide to alleviate the toxicity on banana infested with parasitic-nematode. Waste and Biomass Valorization, 13, 405–415. https://doi.org/10.1007/s12649-021-01527-6
- El-Ansary, M. S. M., Hamouda, R. A. F., & Ahmed-Farid, O. A. (2021). Bioremediation of Oxamyl compounds by Algae: Description and traits of root-knot nematode control. Waste and Biomass Valorization, 12(1), 251–261. https://doi.org/10.1007/s12649-020-00950-5
- El-Ashry, R. M., El-Saadony, M. T., El-Sobki, A. E. A., El-Tahan, A. M., Al-Otaibi, S., El-Shehawi, A. M., Saad, A. M., & Elshaer, N. (2021). Biological silicon nanoparticles maximize the efficiency of nematicides against biotic stress induced by Meloidogyne incognita in eggplant. Saudi Journal of Biological Sciences, 29(2), 920–932. https://doi.org/10.1016/j.sjbs.2021.10.013
- Elkobrosy, D. H., Abdelsalam, N. R., El-Saedy, M. A., Shama, S., & Hafez, E. E. (2020). The effect of cyst nematode (Globodera rostochiensis) isolate ddh1 on gene expression in systemic leaves of potato plant. Journal of Microbiology, Biotechnology and Food Sciences, 10(1), 93–97. https://doi.org/10.15414/jmbfs.2020.10.1.93-97
- Ellegaard-Jensen, L., Jensen, K. A., & Johansen, A. (2012). Nano-silver induces dose-response effects on the nematode Caenorhabditis elegans. Ecotoxicology and Environmental Safety, 80, 216–223. https://doi.org/10.1016/j.ecoenv.2012.03.003
- El-Rokiek, K. G., & El-Nagdi, W. M. (2011). Dual effects of leaf extracts of Eucalyptus citriodora on controlling purslane and root-knot nematode in sunflower. Journal of Plant Protection Research, 51(2), 121–129. https://doi.org/10.2478/v10045-011-0021-0
- Elshafie, H. S., Osman, A., El-Saber, M. M., Camele, I., & Abbas, E. (2023). Antifungal activity of green and chemically synthesized ZnO nanoparticles against Alternaria citri, the causal agent citrus black rot. The Plant Pathology Journal, 39(3), 265–274. https://doi.org/10.5423/PPJ.OA.02.2023.0035
- Entsar, E. (2016). Nematicidal effects of silver nanoparticles on root-knot nematodes (Meloidogyne incognita) in laboratory and screenhouse. Journal of Plant Protection and Pathology, 7(5), 333–337. https://doi.org/10.21608/jppp.2016.50566
- Ghareeb, R. Y., Alfy, H., Fahmy, A. A., Ali, H. M., & Abdelsalam, N. R. (2020). Utilization of Cladophora glomerata extract nanoparticles as eco-nematicide and enhancing the defense responses of tomato plants infected by Meloidogyne javanica. Scientific Reports, 10(1), 19968. https://doi.org/10.1038/s41598-020-77005-1
- Gonullu, M. P., Cakil, D. D., & Cetinkaya, C. (2024). Influence of thermal treatment and Fe doping on ZnO films by ultrasonic spray pyrolysis. Thin Solid Films, 793, 140265. https://doi.org/10.1016/j.tsf.2024.140265
- González-Moragas, L., Maurer, L. L., Harms, V. M., Meyer, J. N., Laromaine, A., & Roig, A. (2017). Materials and toxicological approaches to study metal and metal-oxide nanoparticles in the model organism Caenorhabditis elegans. Materials Horizons, 4(5), 719–746. https://doi.org/10.1039/C7MH00166E
- Hashem, A. H., Selim, T. A., Alruhaili, M. H., Selim, S., Alkhalifah, D. H. M., Al Jaouni, S. K., & Salem, S. S. (2022). Unveiling antimicrobial and insecticidal activities of biosynthesized selenium nanoparticles using prickly pear peel waste. Journal of Functional Biomaterials, 13(3), 112. https://doi.org/10.3390/jfb13030112
- Hasnidawani, J. N., Azlina, H. N., Norita, H., Bonnia, N. N., Ratim, S., & Ali, E. S. (2016). Synthesis of ZnO nanostructures using sol-gel method. Procedia Chemistry, 19, 211–216. https://doi.org/10.1016/j.proche.2016.03.095
- Jameel, M., Shoeb, M., Khan, M. T., Ullah, R., Mobin, M., Farooqi, M. K., & Adnan, S. M. (2020). Enhanced insecticidal activity of thiamethoxam by zinc oxide nanoparticles: A novel nanotechnology approach for pest control. ACS Omega, 5(3), 1607–1615. https://doi.org/10.1021/acsomega.9b03680
- Jatala, P. (2019). Biology and management of plant-parasitic nematodes on sweet potato. In Sweet Potato Pest Management (pp. 359–378). CRC Press. https://doi.org/10.1201/9780429308109-19
- Jiang, Z., Liu, B., Yu, L., Tong, Y., Yan, M., Zhang, R., Han, W., Hao, Y., Shangguan, L., Zhang, S., & Li, W. (2023). Research progresses in preparation methods and applications of zinc oxide nanoparticles. Journal of Alloys and Compounds, 956, 170316. https://doi.org/10.1016/j.jallcom.2023.170316
- Jones, J. T., Haegeman, A., Danchin, E. G. J., Gaur, H. S., Helder, J., Jones, M. G. K., Kikuchi, T., Manzanilla-López, R., Palomares-Rius, J. E., Wesemael, W. M. L., & Perry, R. N. (2013). Top 10 plant-parasitic nematodes in molecular plant pathology. Molecular Plant Pathology, 14(9), 946–961. https://doi.org/10.1111/mpp.12057
- Jung, Y., Kim, J., Kim, N. H., & Kim, H. G. (2023). Ag–ZnO nanocomposites as a 3D metal-enhanced fluorescence substrate for the fluorescence detection of DNA. ACS Applied Nano Materials, 6(2), 976–985. https://doi.org/10.1021/acsanm.2c04352
- Kalaba, M. H., Moghannem, S. A., El-Hawary, A. S., Radwan, A. A., Sharaf, M. H., & Shaban, A. S. (2021). Green synthesized ZnO nanoparticles mediated by Streptomyces plicatus: Characterizations, antimicrobial and nematicidal activities and cytogenetic effects. Plants, 10(9), 1760. https://doi.org/10.3390/plants10091760
- Kalia, A., Kaur, J., Tondey, M., Manchanda, P., Bindra, P., Alghuthaymi, M. A., Shami, A., & Abd-Elsalam, K. A. (2021). Differential antimycotic and antioxidant potentials of chemically synthesized zinc-based nanoparticles derived from different reducing/complexing agents against pathogenic fungi of maize crop. Journal of Fungi, 7(3), 223. https://doi.org/10.3390/jof7030223
- Karssen, G., Wesemael, W., & Moens, M. (2013). Root-knot nematodes. In Plant nematology (pp. 73–108). Cabi. https://doi.org/10.1079/9781780641515.0073
- Kathirvelu, S., D’souza, L., & Dhurai, B. (2009). UV protection finishing of textiles using ZnO nanoparticles. Indian Journal of Fibre & Textile Research, 34, 267–273.
- Khan, Y. A., Singh, B. R., Ullah, R., Shoeb, M., Naqvi, A. H., & Abidi, S. M. A. (2015). Anthelmintic effect of biocompatible Zinc Oxide Nanoparticles (ZnO NPs) on Gigantocotyle explanatum, a Neglected Parasite of Indian Water Buffalo. PLoS One, 10(7), e0133086. https://doi.org/10.1371/journal.pone.0133086
- Khan, I., Saeed, K., & Khan, I. (2019). Nanoparticles: Properties, applications and toxicities. Arabian Journal of Chemistry, 12(7), 908–931. https://doi.org/10.1016/j.arabjc.2017.05.011
- Khan, M. F., Ansari, A. H., Hameedullah, M., Ahmad, E., Husain, F. M., Zia, Q., Baig, U., Zaheer, M. R., Alam, M. M., Khan, A. M., AlOthman, Z. A., Ahmad, I., Ashraf, G. M., & Aliev, G. (2016). Sol-Gel synthesis of thorn-like ZnO nanoparticles endorsing mechanical stirring effect and their antimicrobial activities: Potential role as nano-antibiotics. Scientific Reports, 6(1), 27689. https://doi.org/10.1038/srep27689
- Khan, M. F., Husain, F. M., Zia, Q., Ahmad, E., Jamal, A., Alaidarous, M., Banawas, S., Alam, M. M., Alshehri, B. A., Jameel, M., Alam, P., Ahamed, M. I., Ansari, A. H., & Ahmad, I. (2020). Anti-quorum sensing and anti-biofilm activity of zinc oxide nanospikes. ACS Omega, 5(50), 32203–32215. https://doi.org/10.1021/acsomega.0c03634
- Khan, M. F., Hameedullah, M., Ansari, A. H., Ahmad, E., Lohani, M. B., Khan, R. H., Alam, M. M., Khan, W., Husain, F. M., & Ahmad, I. (2014). Flower-shaped ZnO nanoparticles synthesized by a novel approach at near-room temperatures with antibacterial and antifungal properties. International Journal of Nanomedicine, 9, 853–864. https://doi.org/10.2147/IJN.S47351
- Kim, S., & Ryu, D. Y. (2013). Silver nanoparticle-induced oxidative stress, genotoxicity and apoptosis in cultured cells and animal tissues. Journal of Applied Toxicology, 33(2), 78–89. https://doi.org/10.1002/jat.2792
- Lawrence, G. W., Clark, C. A., & Wright, V. L. (1986). Influence of Meloidogyne incognita on resistant and susceptible sweet potato cultivars. Journal of Nematology, 18(1), 59–65.
- Lodha, A. S., Pandya, A., & Shukla, R. K. (2016). Nanotechnology: An applied and robust approach for forensic investigation. Foresic Research & Criminology International Journal, 2(1), 00044. https://doi.org/10.15406/frcij.2016.02.00044
- Madhuri, S., Choudhary, A. K., & Rohit, K. (2010). Nanotechnology in agricultural diseases and food safety. Journal of Phytology, 2(4), 78–82.
- Mandal, A. K., Katuwal, S., Tettey, F., Gupta, A., Bhattarai, S., Jaisi, S., Bhandari, D. P., Shah, A. K., Bhattarai, N., & Parajuli, N. (2022). Current research on zinc oxide nanoparticles: Synthesis, characterization, and biomedical applications. Nanomaterials, 12(17), 3066. https://doi.org/10.3390/nano12173066
- Martins, N. C. T., Avellan, A., Rodrigues, S., Salvador, D., Rodrigues, S. M., & Trindade, T. (2020). Composites of biopolymers and ZnO NPs for controlled release of zinc in agricultural soils and timed delivery for maize. ACS Applied Nano Materials, 3(3), 2134–2148. https://doi.org/10.1021/acsanm.9b01492
- Mohd Adnan, M. A., Julkapli, N. M., & Abd Hamid, S. B. (2016). Review on ZnO hybrid photocatalyst: Impact on photocatalytic activities of water pollutant degradation. Reviews in Inorganic Chemistry, 36(2), 77–104. https://doi.org/10.1515/revic-2015-0015
- Mora-Fonz, D., Lazauskas, T., Farrow, M. R., Catlow, C. R. A., Woodley, S. M., & Sokol, A. A. (2017). Why are polar surfaces of ZnO stable? Chemistry of Materials, 29(12), 5306–5320. https://doi.org/10.1021/acs.chemmater.7b01487
- Morkoç, H., & Özgür, Ü. (2008). Zinc oxide: Fundamentals, materials and device technology. John Wiley & Sons. https://doi.org/10.1002/9783527623945
- Mosquera-Sánchez, L. P., Arciniegas-Grijalba, P. A., Patiño-Portela, M. C., Guerra–Sierra, B. E., Muñoz-Florez, J. E., & Rodríguez-Páez, J. E. (2020). Antifungal effect of zinc oxide nanoparticles (ZnO-NPs) on Colletotrichum sp., causal agent of anthracnose in coffee crops. Biocatalysis and Agricultural Biotechnology, 25, 101579. https://doi.org/10.1016/j.bcab.2020.101579
- Mukhopadhyay, S. S. (2014). Nanotechnology in agriculture: Prospects and constraints. Nanotechnology, Science and Applications, 7, 63–71. https://doi.org/10.2147/NSA.S39409
- Nazir, R. (Ed.). (2018). Nanotechnology applications in environmental engineering. IGI Global. https://doi.org/10.4018/978-1-5225-5745-6
- Overstreet, C. (2009). Nematodes. In G. Loebenstein & G. Ottappilly (Eds.), De Sweet Potato (pp. 135–159). Springer. https://doi.org/10.1007/978-1-4020-9475-0_9
- Pandya, A., & Shukla, R. K. (2018). New perspective of nanotechnology: Role in preventive forensic. Egyptian Journal of Forensic Sciences, 8(1), 57. https://doi.org/10.1186/s41935-018-0088-0
- Panse, V. G., & Sukhatme, P. V. (1954). Statistical methods for agricultural workers. Statistical methods for agricultural workers.
- Roh, J. Y., Eom, H. J., & Choi, J. (2012). Involvement of Caenohabditis elegans MAPK signaling pathways in oxidative stress response induced by silver nanoparticles exposure. Toxicological Research, 28(1), 19–24. https://doi.org/10.5487/TR.2012.28.1.019
- Sakuma, M. (1998). Probit analysis of preference data. Applied Entomology and Zoology, 33(3), 339–347. https://doi.org/10.1303/aez.33.339
- Saloga, P. E., Rybak, T., & Thünemann, A. F. (2022). Microwave-assisted synthesis of ZnO nanoparticles: Phase transfer to water. Advanced Engineering Materials, 24(6), 2101276. https://doi.org/10.1002/adem.202101276
- Sávoly, Z., Hrács, K., Pemmer, B., Streli, C., Záray, G., & Nagy, P. I. (2016). Uptake and toxicity of nano-ZnO in the plant-feeding nematode, Xiphinema vuittenezi: the role of dissolved zinc and nanoparticle-specific effects. Environmental Science and Pollution Research International, 23(10), 9669–9678. https://doi.org/10.1007/s11356-015-5983-4
- Singh, R., Choudhary, P., Kumar, S., & Daima, H. K. (2024). Mechanistic approaches for crosstalk between nanomaterials and plants: Plant immunomodulation, defense mechanisms, stress resilience, toxicity, and perspectives. Environmental Science, 2024, 53. https://doi.org/10.1039/D4EN00053F
- Sirelkhatim, A., Mahmud, S., Seeni, A., Kaus, N. H. M., Ann, L. C., Bakhori, S. K. M., Hasan, H., & Mohamad, D. (2015). Review on zinc oxide nanoparticles: Antibacterial activity and toxicity mechanism. Nano-Micro Letters, 7(3), 219–242. https://doi.org/10.1007/s40820-015-0040-x
- Soren, S., Kumar, S., Mishra, S., Jena, P. K., Verma, S. K., & Parhi, P. (2018). Evaluation of antibacterial and antioxidant potential of the zinc oxide nanoparticles synthesized by aqueous and polyol method. Microbial Pathogenesis, 119, 145–151. https://doi.org/10.1016/j.micpath.2018.03.048
- Subbotin, S. A., Palomares-Rius, J. E., & Castillo, P. (2021). Taxonomic history. In D. J. Hunt & R. N. Perry (Eds.), Systematics of root-knot nematodes (Nematoda: meloidogynidae) (Nematology monographs and Perspectives 14 (pp. 1–15). Brill. https://doi.org/10.1163/9789004387584_002
- Titus, D., Samuel, E. J. J., & Roopan, S. M. (2019). Nanoparticle characterization techniques. In Green synthesis, characterization and applications of nanoparticles (pp. 303–319). Elsevier. https://doi.org/10.1016/B978-0-08-102579-6.00012-5
- Tryfon, P., Kamou, N. N., Ntalli, N., Mourdikoudis, S., Karamanoli, K., Karfaridis, D., Menkissoglu-Spiroudi, U., & Dendrinou-Samara, C. (2022). Coated Cu-doped ZnO and Cu nanoparticles as control agents against plant pathogenic fungi and nematodes. NanoImpact, 28, 100430. https://doi.org/10.1016/j.impact.2022.100430
- Wang, P., Menzies, N. W., Lombi, E., McKenna, B. A., Johannessen, B., Glover, C. J., Kappen, P., & Kopittke, P. M. (2013). Fate of ZnO nanoparticles in soils and cowpea (Vigna unguiculata). Environmental Science & Technology, 47(23), 13822–13830. https://doi.org/10.1021/es403466p
- Wang, X., Deng, L. L., Wang, L. Y., Dai, S. M., Xing, Z., Zhan, X. X., Lu, X. Z., Xie, S. Y., Huang, R. B., & Zheng, L. S. (2017). Cerium oxide standing out as an electron transport layer for efficient and stable perovskite solar cells processed at low temperature. Journal of Materials Chemistry A, 5(4), 1706–1712. https://doi.org/10.1039/C6TA07541J
- Wang, Z., Wang, S., Ma, T., Liang, Y., Huo, Z., & Yang, F. (2023). Synthesis of zinc oxide nanoparticles and their applications in enhancing plant stress resistance: A review. Agronomy, 13(12), 3060. https://doi.org/10.3390/agronomy13123060
- Wirunchit, S., Wonganan, N., & Koetniyom, W. (2023). Multi self-cleaning properties of zinc oxide nanoparticles/polydimethylsiloxane (ZnO/PDMS) composite on polyester textile. Current Applied Science and Technology, 23(5), 10–55003. https://doi.org/10.55003/cast.2023.05.23.015
- Wojnarowicz, J., Chudoba, T., & Lojkowski, W. (2020). A review of microwave synthesis of zinc oxide nanomaterials: Reactants, process parameters and morphologies. Nanomaterials, 10(6), 1086. https://doi.org/10.3390/nano10061086
- Xie, J., Li, P., Li, Y., Wang, Y., & Wei, Y. (2009). Morphology control of ZnO particles via aqueous solution route at low temperature. Materials Chemistry and Physics, 114(2-3), 943–947. https://doi.org/10.1016/j.matchemphys.2008.11.007
- Yazhiniprabha, M., Vaseeharan, B., Sonawane, A., & Behera, A. (2019). In vitro and in vivo toxicity assessment of phytofabricated ZnO nanoparticles showing bacteriostatic effect and larvicidal efficacy against Culex quinquefasciatus. Journal of Photochemistry and Photobiology. B, Biology, 192, 158–169. https://doi.org/10.1016/j.jphotobiol.2019.01.014
- Yu, Z., Li, Q., Wang, J., Yu, Y., Wang, Y., Zhou, Q., & Li, P. (2020). Reactive oxygen species-related nanoparticle toxicity in the biomedical field. Nanoscale Research Letters, 15(1), 115. https://doi.org/10.1186/s11671-020-03344-7
- Zhao, L., Lu, L., Wang, A., Zhang, H., Huang, M., Wu, H., Xing, B., Wang, Z., & Ji, R. (2020). Nano-biotechnology in agriculture: Use of nanomaterials to promote plant growth and stress tolerance. Journal of Agricultural and Food Chemistry, 68(7), 1935–1947. https://doi.org/10.1021/acs.jafc.9b06615