Abstract
Purpose: Evaluate if etomidate modulates adrenal apoptosis and if this influences the development of critical illness-related corticosteroid insufficiency (CIRCI) in hemorrhagic shock (HS). Material and methods: Four groups of 16 male Wistar rats: G0 (control group anesthetized with isoflurane and mechanical ventilation), G1 (like G0, but with buprenorphine), G2 (like G1 with HS), and G3 (like G2 with etomidate 1 mg/kg, IV, before HS). HS induced by collecting 30% of blood volume. Resuscitation performed 90 min later with the collected blood and normal saline. Hemodynamic parameters, blood gas analysis, adrenocorticotropic hormone (ACTH), corticosterone (CS), and TNF-α, IL6, IL10 were determined at 0, 90, 150, and 240 min post-HS induction (at the corresponding time points in G0 and G1). Apoptosis and necrosis were determined by TUNEL and caspase-3 immunofluorescence and a necrosis score, respectively. Results: HS groups had significantly higher levels of apoptosis and necrosis than G1 and G0. Compared with G2, etomidate-treated animals had significantly lower levels of CS (compatible with CIRCI), PO2, PO2/FiO2, BE, HCO3, apoptosis, and necrosis and significantly higher cytokine levels. Conclusions: Etomidate was associated with CIRCI. HS was associated with adrenal gland apoptosis and necrosis. The latter were decreased by etomidate, possibly by both direct and indirect mechanisms.
Public Interest Statement
Hemorrhagic shock (HS) is still a significant Health problem, being responsible for more than a third of deaths associated to trauma. Etomidate is an anesthetic commonly used for intubation in HS. However, its use has been recently questioned in critically ill patients because it affects adrenal function. Apoptosis is a type of cell death which has been associated to development of organ dysfunction in critical illness. In this study, the effects of etomidate on adrenal apoptosis and necrosis in a rat model of HS were evaluated. The study found that etomidate attenuated significantly the development of adrenal apoptosis and necrosis. Etomidate also increased morbidity and was associated to significant adrenal dysfunction. Thus, etomidate´s effects in HS are complex and can be beneficial and deleterious. Until these effects are more thoroughly evaluated and its clinical significance determined, etomidate should be used cautiously in HS and only in specific circumstances.
Competing Interests
The authors declare no competing interest.
1. Introduction
Etomidate is an anesthetic commonly used in critical conditions, such as Hemorrhagic Shock (HS), due to its favorable hemodynamic profile (Forman, Citation2011). However, it also directly inhibits adrenal gland steroidogenesis, even after single bolus administration (Allolio et al., Citation1985; Wagner, White, Kan, Rosenthal, & Feldman, Citation1984). This “pharmacologic adrenal suppression” has been associated to development of critical illness-related corticosteroid insufficiency (CIRCI) in several conditions, including sepsis and septic shock (Cherfan et al., Citation2011; den Brinker et al., Citation2008), trauma (Cotton et al., Citation2008) and burns (Mosier, Lasinski, & Gamelli, Citation2015). CIRCI is defined as the inadequate glucocorticoid intracellular anti-inflammatory activity for the severity of the patient’s illness (Marik, Citation2009). The clinical significance of etomidate-associated CIRCI has been extensively debated and it is still incompletely understood. The most recently available meta-analysis, however, have reached the conclusion that etomidate administration to critically ill patients is associated with increased risk of Multi-Organ Failure (Bruder, Ball, Ridi, Pickett, & Hohl, Citation2015).
The development of CIRCI following HS has been demonstrated in both clinical (Cotton et al., Citation2008; Hoen et al., Citation2002; Stein et al., Citation2013) and experimental models (Rushing, Britt, & Britt, Citation2006; Wang et al., Citation1999). How HS induces CIRCI is still incompletely understood although several mechanisms have been suggested. These include the following: adrenal necrosis caused by HS-associated ischemia (Kajihara, Malliwah, Matsumura, Taguchi, & Iijima, Citation1983; Rushing et al., Citation2006); reduced adrenal levels of corticosterone (CS) and cyclic adenosine monophosphate; reduced CS release following corticotropin stimulation; decreased adrenocorticotropic hormone (ACTH) secretion (Wang et al., Citation1999) and adrenal dysfunction due to HS-associated inflammation (Hoen et al., Citation2002). Etomidate administration has also been suggested as a possible mechanism by some but not all authors. For example, Hoen et al. (Citation2002) did not found any relation between CIRCI’s occurrence and etomidate administration when studying trauma patients with HS. In contrast, and also in trauma with HS, another study found that etomidate was the only modifiable factor associated to CIRCI (Cotton et al., Citation2008).
In recent years, experimental studies have shown that increased adrenal apoptosis can cause adrenal dysfunction in several types of critical illnesses, including acute necrotizing pancreatitis (ANP) and sepsis (Liu et al., Citation2016; Polito et al., Citation2010; Yu et al., Citation2012, Citation2016). To our knowledge only one study described the development of adrenal apoptosis in HS, and this did not report any occurrence of CIRCI (Rushing & Britt, Citation2007).
Etomidate has been shown to modulate apoptosis in several cell types (Wu et al., Citation2011; Xu, Chen, Luo, & Firoj, Citation2014). This has also been found in the adrenal gland of both normal and septic animals, where etomidate’s modulation of apoptosis seems to be a dose- and time-dependent (Liu, Zhang, Han, Lv, & Xiong, Citation2015; Liu et al., Citation2016; Zhang et al., Citation2015). The influence of etomidate in apoptosis can be both direct, through direct interference in the intra-signaling pathways and proteins associated to the apoptotic process and indirect, through modulation of oxidative and nitrosative stress and inflammation (Liu et al., Citation2015, Citation2016; Zhang et al., Citation2015).
To our knowledge, the contribution of adrenal apoptosis to HS-associated CIRCI and the effects of etomidate in this process have not been described. Therefore, we conducted an experimental rat model of HS to evaluate several hypotheses: that HS is associated to development of adrenal gland apoptosis contributing to CIRCI; that etomidate significantly modulates adrenal apoptosis in HS; that etomidate will exert mainly an anti-apoptotic effect in the adrenal.
2. Clinical significance
• | Etomidate is associated with development of CIRCI in critically ill patients although the clinical significance of this is still being debated. | ||||
• | Few studies have addressed the development of etomidate-associated CIRCI in HS and none in an experimental model. | ||||
• | This study demonstrated significant morbidity associated with etomidate-associated CIRCI in experimental HS. | ||||
• | In addition, the study demonstrated for the first time that HS-associated adrenal apoptosis and necrosis were decreased in etomidate-treated groups. | ||||
• | This finding launches the possibility of using etomidate therapeutically to decrease adrenal apoptosis and necrosis in HS. |
3. Methods
All experiments were performed accordingly with the ethical standards of Faculdade de Medicina Veterinária da Universidade de Lisboa, in compliance with the Portuguese legislation for the use of animals for experimental purposes (Decreto-Lei nº 129/92 and Portaria nº 1005/92, DR nº 245, série I-B, 4930-42), and with the European Union legislation (EU Directive 2010/63/EU).
3.1. Experimental animals
Twelve-week-old male Wistar rats (Rattus norvegicus) (Charles Rivers, Barcelona, Spain), weighing 250–450 g were used. Animals were housed (n = 3 per cage) in a climate-controlled room under standard conditions (20–24°C; 12 h light/dark cycle). Water was provided ad libitum and food consisted of rat chow (Harlan ®). All animals were acclimatized for seven days before the experiments.
3.2. Study groups
Rats were randomly and blindly allocated to one of four body weight-matched groups: G0 (n = 16), G1 (n = 16), G2 (n = 16), and G3 (n = 16). Rats from G0 (the control group) were subjected to general anesthesia (GA), mechanical ventilation, and surgical intervention. Rats from G1 had the same procedures as G0, but also received buprenorphine. Rats from G2 had the same procedures as G1 but were subjected to HS. Rats from G3 had the same procedures as G2, but also received etomidate.
3.3. Experimental procedures
The experimental protocol is displayed in Figure .
Figure 1. Experimental time line.
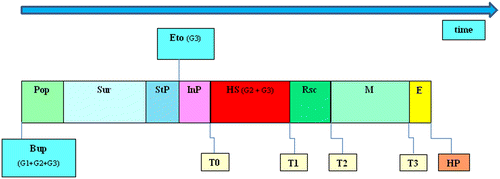
3.4. Anesthesia and surgical procedure
Rats from all experimental groups except G0 were pre-medicated with 0.05 mg/kg buprenorphine (Budale®, Dechra, UK) to induce pre-emptive analgesia. Buprenorphine was injected subcutaneously (SC), 20 min prior induction of GA. GA was initiated by placing the animals in an induction chamber (World Precision Instruments, UK, Europe) previously saturated with 100% oxygen and 5% isoflurane (IsoFLo®, Abbott, USA). Once anesthetized, rats were moved and placed in dorsal recumbency over a water-based heated pad. Isoflurane anesthesia was maintained through face mask until a tracheostomy tube was placed. ECG was registered continuously through lead wire probes (ECG; ML136 Animal Bio Amp, ADInstruments, UK). A respiratory sensor was placed over the thoracic wall to measure respiratory rate. A rectal probe (MLT1403, ADInstruments, UK) and an oximetry tail sensor (ADInstruments, UK,) were placed to continuously record rectal temperature (kept between 35 and 38°C) and pulse oximetry, respectively. The pedal withdrawal reflex was used to help in assessing the depth of anesthesia. The anesthetic plane was considered adequate if a toe pinch did not induce the withdrawal reflex. An intravenous catheter (Introcan®, 26 Gauge, B. Braun Medical, Portugal) was placed in the left femoral vein for fluid and drug administration. Another catheter was placed in the right external carotid artery and connected to a three-way stopcock for blood sampling and arterial blood pressure measurement. The carotid catheter and the three-way stopcock were connected to a fluid-filled pressure transducer (MLT844, ADInstruments, UK) which in turn was connected to blood pressure amplifiers (ML221 Bridge Amp, ADInstruments, UK). Arterial blood pressure [systolic, diastolic and mean arterial pressure (MAP)], ECG, rectal temperature, pulse oximetry, and respiratory frequency data were transmitted to a data acquisition unit (PowerLab®, ADInstruments, UK), to be analyzed by a specific software (LabChart Pro®, ADInstruments, UK).
After placement of a carotid catheter, the tracheostomy tube was placed. Volume-controlled ventilation was initiated with a small animal ventilator (CWE Small Animal Ventilator, World Precision Instruments, UK). General anesthesia was maintained with isoflurane at concentrations of 1.5–2%, administered through the tracheostomy tube. Oxygen was also delivered by the tracheostomy tube. The inspiratory fraction of O2 (FiO2) was kept at 100% until the end of the experiment. Initial ventilator settings consisted in a tidal volume of 10 ml/kg and a respiratory rate of 110–125 breaths/min. These parameters were adjusted as the experiment progressed, accordingly to the results of arterial blood gas analysis, with the aim of maintaining normocapnia (35–45 mm Hg) and normoxemia [arterial pressure of O2 (PO2)>85 mm Hg]. Once the surgical procedure was completed, a stabilization period of 10 min (15 min in G2) was allowed to happen before inducing HS in G2 and G3. The purpose of the stabilization period was to permit the recovery of the hemodynamic instability caused by initiation of mechanical ventilation. In G3, the stabilization period was followed by the intermediate period of 5 min in order that HS was not initiated immediately after etomidate injection. At T3 and after blood sampling, euthanasia was performed by pentobarbital administration (Eutasil®, Ceva, Portugal) at 100–150 mg.kg−1 through the femoral catheter.
3.5. Sample collection and anesthesia monitoring
At four time points (T0, T1, T2, and T3), a sample of blood (500 μl) was collected for analysis. To prevent activation of the hypothalamic-pituitary axis following blood collection 1 ml of normal saline was administered IV following each blood sampling, except at T0 in G2 and G3, when blood collection coincided with HS induction. At T0, T1, T2, and T3, MAP, heart rate (HR), temperature, ECG, ventilator parameters, and depth of anesthesia were recorded. T0, the initial time point, was set at the end of the stabilization period (intermediate period in G3). T1, T2, and T3 were set at 90, 150, and 240 min post-T0, respectively. HR and MAP collected at each time point were used for analysis and constituted the hemodynamic variables.
3.6. Immunological and hormonal variables
To determine hormone and cytokine levels, 200 μl of the collected blood were placed in sterile heparin-coated tubes (FactorMed, Portugal) and centrifuged (12,000 rpm for 15 min) to obtain plasma. Plasma was then stored at −20°C until further analysis. ACTH, CS (rat stress hormone panel Millipore, Arium Laboratórios, Portugal) TNF-α, IL6, and IL10 (Rat Cytokine/Chemokine, Millipore, Arium Laboratórios, Portugal) plasma levels were determined by Multiplex/Luminex technology (Vignali, Citation2000).
3.7. Metabolic variables
At all time points, 100 μl of blood was used to measure metabolic variables, including pH, PO2, arterial pressure of CO2 (PCO2), BE, HCO3, and lactate, through a portable blood gas analyzer (I-STAT Analyzer 300, I-STAT Corporation, Abbot, USA). The arterial pressure of O2/Inspiratory Fraction of O2 (PO2/FiO2) ratio was calculated as indicator of lung dysfunction.
3.8. HS induction and resuscitation
In G2 and G3, at T0 and after blood sampling, HS was induced by collecting 30% of blood volume (estimated as 54 ml/kg) (Rushing et al., Citation2006) from the carotid artery. Blood collection was divided into two stages. In the first stage, 15% of blood volume was collected in 10 min. The remaining 15% was collected on the following 20 min. We divided blood collection in two stages of different velocities, to induce a more natural model of hypovolemia. Hemorrhage was stopped when 30% of the estimated blood volume was removed and/or when MAP reached and stabilized at 45 mm Hg. The blood collected was placed into 1 ml sterile aliquots previously filled with 0.12 ml of sodium citrate until it was used in the resuscitation phase.
Resuscitation was initiated at T1, after blood sampling for analysis. It was performed by administrating the blood which was removed to induce HS added to normal saline in a 1:3 ratio, respectively. Both fluids were warmed at body temperature and administered by a syringe pump (Perfusor® fm (MFC), B. Braun Medical, Portugal) through the femoral vein catheter. Resuscitation lasted 30 min and once completed, a constant-rate infusion of warmed normal saline was administered at maintenance rate (2 ml/kg/h) until the end of the experiment.
3.9. Etomidate administration
In G3, etomidate (Etomidate®, Lipuro, 2 mg/ml, B. Braun Medical, Portugal) was administered by bolus at 1 mg/kg, IV, 5 min before T0. The total volume of anesthetic solution was 0.5 ml/kg for each animal. In the other groups, the same amount of normal saline was given instead of etomidate.
3.10. Histopathological variables
3.10.1. Adrenal gland necrosis assessment
After euthanasia both adrenals were collected and placed in 1% formaldehyde. Several 10 μm-thick tissue sections were than obtained and stained with hematoxylin and eosin. Two slides of each adrenal gland were evaluated by a pathologist blinded to the study, which looked for changes such as tissue edema, lipid vacuolization, and inflammatory cell infiltration. The degree of adrenal necrosis was quantified in a score adapted from a score used in other study (Rushing et al., Citation2006). In our scoring system, necrosis was quantified in five categories depending on the percentage of gland affected. Thus, score 0 represented a complete absence of necrosis and scores 1, 2, 3, and 4 corresponded to 25%, 25–50%, 50–75%, and more than 75% of the gland being affected by necrosis, respectively. The necrosis score was first determined in each of the two original slides of the adrenal gland. This first score was used to determine the average necrosis score of each gland. The scores of the two glands were used to calculate the average necrosis score of each animal. A final average necrosis score for each group calculated the necrosis scores of all animals belonging to the correspondent group.
3.10.2. TUNEL assay
TUNEL staining was performed according to the manufacturer’s instructions (ApopTag® Plus Peroxidase In Situ Apoptosis Kit, #S7101, Merck KGaA, Darmstadt, Germany). Tissue specimens were examined using an Axioskop bright-field microscope (Carl Zeiss GmbH, Gena, Germany). Tissue sections 10 μm thick were deparaffinized in three changes of xylene, and next hydrated with two changes of absolute ethanol, followed by three consecutive washes in 95%, in 70% ethanol, and in phosphate buffer saline (PBS), 5 min per wash. Then, samples were incubated with freshly diluted proteinase K (20 μg/ml) in PBS, for 15 min at room temperature, followed by two washes in ddH2O, for 2 min also at room temperature. In the following step samples were treated with 3% hydrogen peroxide to quench endogenous peroxidase activity. After adding the equilibration buffer, sections were treated with terminal deoxynucleotidyltransferase for 60 min at 37°C. This was followed by incubation with anti-digoxigenin peroxidase conjugate for 30 min at 37°C, staining with 3, 3′-diaminobenzidine substrate, and counterstaining with 0.5% methyl green. Finally, slides were rinsed, dehydrated, and mounted. A negative control was prepared by omitting the TdT enzyme to control for non-specific incorporation of nucleotides or binding of enzyme-conjugate.
The slides were observed and photo documented using an Olympus BH-2 microscope. To quantify TUNEL-positive nuclei, a total of 12 high-power fields (x400) were randomly examined for each animal, six from each adrenal gland (three fields from the adrenal cortex and three from the adrenal medulla). The apoptotic index (in percentage) was obtained from the ratio between the number of apoptotic and total number of cells counted in each field, as described by others (Yu et al., Citation2012). The cell count in each field was determined using Image J software (NIH, http://rsb.info.nih.gov/ij/), especially adapted for this purpose. The apoptotic index obtained in each field was used to calculate the average cortical and medullary apoptotic index of each adrenal gland. The average cortical and medullary apoptotic indexes of each animal were subsequently obtained from the results of the two adrenal glands . Finally, the average cortical and medullary apoptotic index for each group was calculated from the values of all animals belonging to each specific group. These were then compared through statistically methods.
3.10.3. Caspase-3 immunofluorescence microscopy analysis
Apoptosis was confirmed by determining the presence of active caspase-3 (Porter & Jänicke, Citation1999; Yu et al., Citation2012). For this assessment, 10 μm thick paraffin-embedded adrenal sections were deparaffinized, rehydrated, and boiled three times in 10 mM citrate buffer, pH 6. Sections were then incubated for 60 min in blocking buffer, containing 10% (v/v) normal donkey serum (Jackson ImmunoResearch Laboratories Inc., West Grove, PA, USA) in PBS with 0.1% (v/v) Triton X-100 (Sigma-Aldrich), and subsequently in diluted primary antibodies overnight at 4°C. After rinsing, the primary antibody was developed by incubating with DyLight 488- (Jackson ImmunoResearch) or Alexa Fluor 594- (Invitrogen, Grand Island, NY, USA) conjugated secondary antibodies against the corresponding species, for 2 h at room temperature. To confirm the apoptotic phenotype, we used a rabbit polyclonal active caspase-3 antibody (R&D Systems, Lille, France; 1:100). No staining was observed in control sections, where primary antibody was replaced by blocking buffer. Immunofluorescence analysis was performed using an epifluorescence microscope (Leica DM R HC model, Wetzlar, Germany). The data-sets were acquired by Adobe Photoshop CS5 software (Adobe Systems, Inc., San Jose, USA) and images were subsequently processed with Image J open source software (version 1.46r).
3.11. Statistical Analysis
Statistical analyses were performed with the Statistica software, version 8.0 (Statsoft Ibérica, Lisboa, Portugal), using a mixed linear model. Data were expressed as mean and standard error. For each variable, a two-way ANOVA was used where variance components were obtained through the Variance Estimation and Precision (VEPAC) Statistica module. Variance components in the model were estimated by Restricted Maximum Likelihood (REML) estimation. Least Squares means and standard errors for Least square means were computed from the solution of the mixed model equations. Correlation analysis between variables was performed with the Spearman rank correlation, considering the complete set of four time points. In all analysis, statistical significance was set at a p < 0.05.
4. Results
Data were obtained in all animals of G0 and G1. Three animals of G2 and two animals of G3 were excluded due to insufficient data.
4.1. Hormonal variables
The statistical analysis is shown in Figure and Supplementary Table 1. In both HS groups, ACTH levels reached its highest peak at T1. The G3’s value of ACTH at T1 was the highest of all groups and time points. ACTH levels were always higher in G3 than in G2 except at T3. ACTH levels were higher in G2 than in G1 at T1 and T2, but not at T0 and T3. G0’s ACTH levels were the lowest of all groups. The statistically significant differences between groups were between G2 and G3 with G1 at T1 (G2 with G1, p = 0.001; G3 with G1, p = 0.000) and between G2 and G3 with G0 at T1 (G2 with G0, p = 0.001; G3 with G0, p = 0.000). The interaction between ACTH and time was also statistically significant (p = 0.006).
Figure 2. Variation of hormonal variables from T0 to T3 in G0, G1, G2, and G3: (a) ACTH and (b) CS.
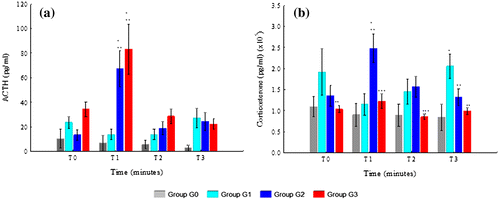
The highest levels of CS were observed in G2 at T1. CS levels were lower in G3 than in G2 at all time points, although this difference was only statistically significant at T1 (p = 0.000) and T2 (p = 0.031). CS levels were also lower in G3 than in G1 at T0, T2, and T3. However, this was only statistically significant at T0 (p = 0.003) and T3 (p = 0.001). The levels of CS in G3 and G0 were similar and not statistically different. In G3, CS levels were lower than10000 pg/ml at T0, T1, and T2.
The levels of CS were higher in G2 than in G1 at T1 and T2, but not at T0 and T3. These differences were only statistically significant at T1 (p = 0.000) and T3 (p = 0.046). The levels of CS in G2 were always higher than in G0, although none of the differences reached statistically significance. The levels of CS in G1 were always higher than in G0, but only at T3 the difference was statistically significant (p = 0.002). The interaction between CS and time was statistically significant (p = 0.015).
4.2. Immunological variables
Immunological variables are displayed in Figure and Supplementary Table 2. TNF-α was only detectable in G3. In this group, it increased from T0 to T2 and then decreased at T3. The differences between G3 with other groups were only statistically significant at T2 (G3 with G0, p = 0.017; G3 with G1, p = 0.008; G3 with G2, p = 0.012) and T3 (G3 with G0, p = 0.040; G3 with G1, p = 0.022; G3 with G2, p = 0.030). The differences between G0, G1, and G2 were not statistically significant.
Figure 3. Immunological variables: (a) TNF-α; (b) IL6 and (c) IL10.
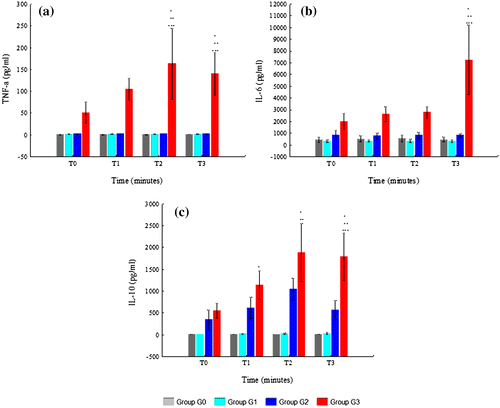
G3 had always higher levels of IL6 than other groups. These differences were only statistically significant at T3 (G3 with G0, p = 0.004; G3 with G1, p = 0.000; G3 with G2, p = 0.002). In G3, the levels of IL6 increased from T0 to T3, which contrasts with the other groups, where they remained stable. The levels of IL6 of G2 were always higher than those of G0 and G1. However none of the differences between G2, G1, and G0 were found to be statistically significant.
IL10 levels were only detectable in G2 and G3. Its highest level was observed in G3 at T2. The levels of IL10 were always higher in G3 than in G2, although the only difference which was statistically significant was at T3 (p = 0.004). The levels of IL10 in G3 also differed significantly from those in G1 at T2 (p = 0.001) and T3 (p = 0.002) and from those in G0 at T1 (p = 0.051), T2 (p = 0.003), and T3 (p = 0.005). The differences between G2, G1, and G0 were not statistically significant.
4.3. Metabolic variables
In metabolic variables the groups differed significantly at several time points (Figure and and Supplementary Table 3).
Figure 4. Variation of metabolic variables (pH, lactate, BE and HCO3) from T0 to T3 in G0, G1, G2, and G3: (a) pH; (b) lactate; (c) BE and (d) HCO3.
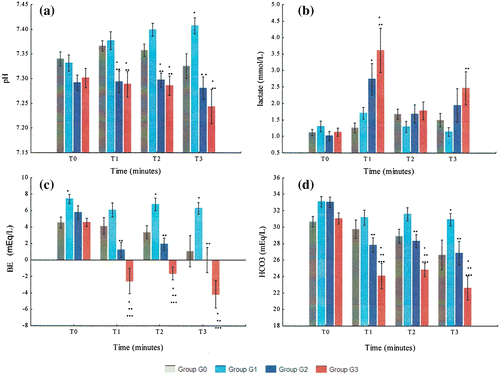
Figure 5. Variation of metabolic variables (PO2 and PO2/FiO2) from T0 to T3 in G0, G1, G2, and G3: (a) PO2 and (b) PO2/FiO2.
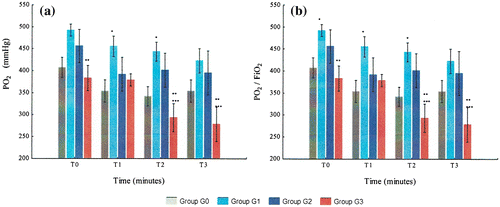
The pH of G2 and G3 was always lower than G1 and G0 at all time points. G3 and G1 were the group with the lowest and highest pH levels, respectively.
The levels of lactate increased after hemorrhage, decreased after resuscitation and increased from T2 to T3 in both G2 and G3. Lactate levels were always higher in G3 than in G2 although the differences were not statistically significant. The levels of lactate in G3 were also higher than in G1 and G0 at T1, T2, and T3.
BE levels decreased markedly with hemorrhage, then improved slightly with resuscitation and finally decreased from T2 to T3, in both G2 and G3. G1 was the group which presented the highest BE levels. BE was always lower in G3 than in G2 and G1. The interaction between time and BE was statistically significant (p = 0.016).The results of HCO3 were similar to BE. G1 had the highest HCO3 levels of all groups. HCO3 levels were lower in G3 than in G2 at all time points.
G3 animals had the lowest PO2 levels of all groups. In G3, PO2 levels did not significantly change with hemorrhage, but then decreased continuously after resuscitation until T3. G2’s PO2 levels decreased especially from T0 to T1, but contrarily to G3, they remained stable from T1 to T3. The PO2/FiO2 ratio in G3 decreased progressively from T0 to T3 and reached levels below 300 at T2 and T3.
4.4. Hemodynamic variables
Hemodynamic variables are shown in Figure and in Supplementary Table 4.
Figure 6. Variation of hemodynamic variables from T0 to T3 in G0, G1, G2, and G3: (a) HR and (b) MAP.
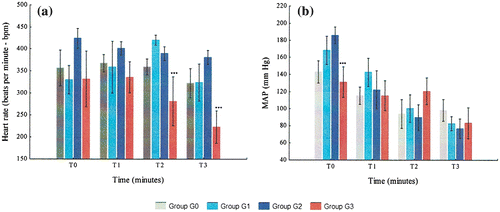
G3 animals had always lower HR than other groups. In contrast, G2 was the group with the highest HR levels. In G3, HR was stable from T0 to T1 and then decreased continuously until T3. In G2, HR was highest at T0 and then decreased continuously until T3. None of the differences regarding HR between the studied groups were statistically significant, except the ones between G3 and G2 at T2 (p = 0.0.037) and T3 (p = 0.003).
G3’s MAP decreased slightly with hemorrhage, increased mildly at T2 and decreased from T2 to T3. At T2, G3 was the group which presented the highest MAP levels. In both G1 and G2, MAP decreased continuously from T0 to T3. The difference between G2 and G3 at T0 was the only one between all groups which was statistically significant (p = 0.014).
4.5. Histopathological variables
4.5.1. Apoptosis
Cortical and medullary apoptosis statistical analysis is described in Supplementary Table 5 and Figures . Figures – display the presence of apoptosis in the adrenal cortex and medulla. In the cortex, TUNEL-positive nuclei were more frequently found in zona reticularis than in zona fasciculata and were infrequently found in zona glomerulosa (Figure –).The statistical analysis of the necrosis score is described in Supplementary Table 5 and Figure .
Figure 7. Variation of the apoptotic index G0, G1, G2, and G3. Apoptosis score in G0, G1, G2, and G3: (a) apoptosis score in the adrenal cortex and (b) apoptosis score in the adrenal medulla.
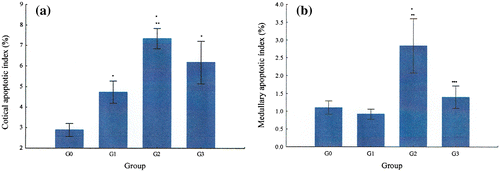
Figure 8. Pictures of TUNEL positive nuclei in the adrenal cortex and medulla and caspase-3 immunofluorescence analysis in G0 and G1.
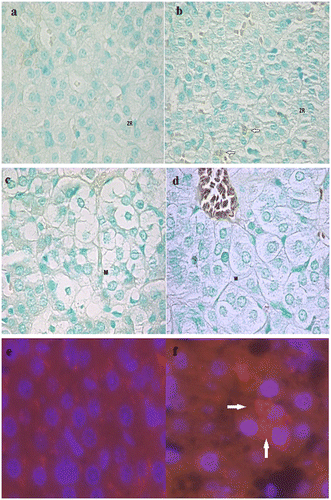
Figure 9. Pictures of TUNEL positive nuclei in the adrenal cortex and medulla and caspase-3 immunofluorescence analysis in G2 and G3.
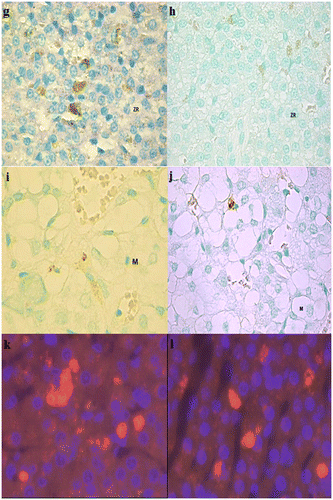
Figure 10. TUNEL positive cells in both adrenal cortex (zona reticularis) and medulla from an animal of G2.
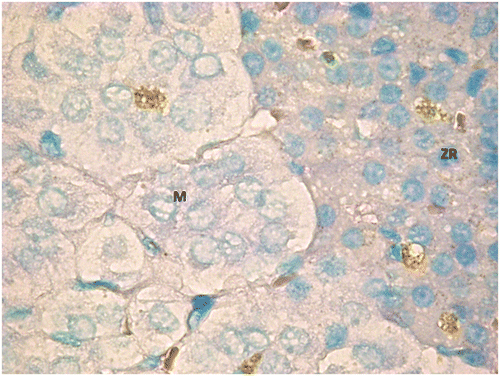
Figure 11. TUNEL positive adrenal cells in high resolution. TUNEL positive adrenal cells in high resolution; (m) zona reticularis of an animal of G2 and (n) adrenal medulla from an animal of G3.
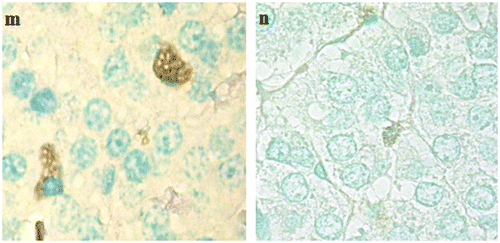
Figure 12. Variation of the necrosis score in G0, G1, G2, and G3.
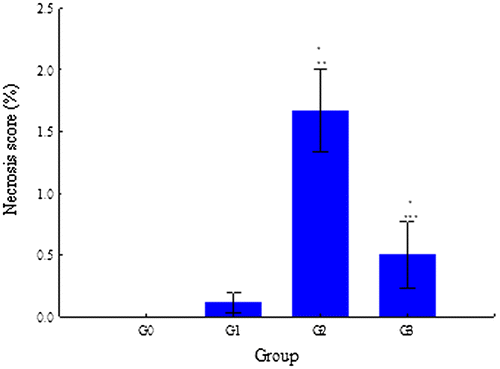
In all groups, apoptotic index was higher in the cortex than in medulla. G2 had the highest cortical apoptotic index, followed by G3, G1, and G0. G2 had also the highest medullary apoptotic index followed by G3, G0, and G1.
Regarding the statistical analysis of cortical apoptotic index, the differences between G0 with G1, G2, and G3 were statistically significant, with the level of significance being p = 0.039, p = 0.000, and p = 0.000, respectively. G2 also differed significantly from G1 (p = 0.008). The difference between G2 and G3 was not statistically significant.
The statistical analysis of the medullary apoptotic index revealed that the one of G2 was significantly different from the one of G0 (p = 0.000), G1 (p = 0.000), and G3 (p = 0.000). The differences between G0, G1, and G3 were not statistically significant.
G2 and G3 were the only groups where adrenal necrosis was found (Figure ). Necrosis was highest in G2, where it reached an average score of 2. The necrosis score of G2 differed significantly from the one of G0 (p = 0.000), G1 (p = 0.000), and G3 (p = 0.000). The necrosis score also differed significantly between G3 and G0 (p = 0.034).
5. Discussion
5.1. Buprenorphine was associated to increased apoptotic rate
In this study, animals of G1 had a higher rate of adrenocortical apoptosis than animals of G0. To our knowledge, no study has described a pro-apoptotic effect of buprenorphine (and of any other opioid) in adrenocortical cells. The underlying mechanisms of buprenorphine’s pro-apoptotic effects could not be determined in this study. However, based in what has been described in nerve and cancer cells, buprenorphine could have promoted apoptosis by increasing mitochondrial permeability and cytochrome c release, by increasing caspase-3 activity and/or by promoting the expression of genes associated with the polyubiquitination of apoptotic proteins (Kugawa, Arae, Ueno, & Aoki, Citation1998; Kugawa, Nakamura, Ueno, & Aoki, Citation2004). In addition, because G1 animals had increased CS levels, buprenorphine could have increased apoptosis indirectly by increasing glucocorticoids levels as these are known to increase adrenal cell apoptosis (Almeida, Matos, & Neves, Citation2007).
5.2. HS increases adrenal gland apoptosis
As expected, HS was associated to increased development of adrenal apoptosis. An increase in adrenal apoptosis has been described in several critical illnesses including trauma (Didenko, Wang, Yang, & Hornsby, Citation1996), ANP (Yu et al., Citation2012, Citation2016), and sepsis (Flierl et al., Citation2008; Kanczkowski, Chatzigeorgiou, Grossklaus, et al., Citation2013, Kanczkowski, Chatzigeorgiou, Samus, et al., Citation2013; Liu et al., Citation2016; Wang et al., Citation2015). In rat models of ANP (Yu et al., Citation2012, Citation2016) and sepsis (Kanczkowski, Chatzigeorgiou, Grossklaus, et al. Citation2013; Liu et al., Citation2016), adrenal apoptosis was also associated to decreased adrenal function. In contrast, although increased adrenal apoptosis was described in HS this was not associated to adrenal dysfunction (Rushing & Britt, Citation2007).
G2 and G3 had several conditions which could have increased the risk of adrenal apoptosis. This include the pro-inflammatory state associated to HS as observed in our study: higher cytokine levels; combination of decreased PO2 and decreased blood pressure, higher lactate and lower BE, HCO3, and pH levels (decreased tissue oxygen delivery); increased risk of developing ischemic/reperfusion (I/R) injury due to resuscitation and, in the case of G2, the increased CS levels (Papathanassoglou, Moynihan, & Ackerman, Citation2000).
In this study, adrenal apoptosis was apparent just 4 h after the induction of a mild state of HS. These results suggest that in this pathologic condition, adrenal apoptosis can develop very early in the disease process and even after a mild insult. If this reflects a specific vulnerability of the adrenal gland to the pro-apoptotic effects of HS or any other reason remains unknown, and to our knowledge, it has never been reported before.
Etomidate-treated animals had a lower apoptotic index than G2, despite having higher TNF-α levels, lower PO2, lower blood pressure, and worse indicators of tissue perfusion following HS. The reasons for these findings were not determined. We hypothesize that this could have been due to two possible mechanisms: a direct anti-apoptotic effect of etomidate; an indirect effect, where the decreased rate of apoptosis was due to etomidate’s effects in other mediators, such as cytokines and hormones.
5.3. Etomidate’s direct anti-apoptotic effects
Studies have shown that etomidate can be both pro- and anti-apoptotic (Roesslein et al., Citation2008; Wang et al., Citation2014; Wu et al., Citation2011; Xu et al., Citation2014; Zhang, Xiong, Jiao, Wang, & Zuo, Citation2010). In addition, recent studies demonstrated that etomidate influences the development of adrenal apoptosis in both normal and septic animals in a time- and dose-dependent manner (Liu et al., Citation2015, Citation2016; Zhang et al., Citation2015). In septic animals when etomidate was administered by constant-rate infusion (2 mg/kg/h) for 2 h or by IV bolus (2 mg/kg), it increased adrenal apoptosis (Liu et al., Citation2016; Zhang et al., Citation2015). In contrast, when administered by IV bolus at 0.6 mg/kg, immediately after the induction of sepsis, it prevented apoptosis (Liu et al., Citation2016; Zhang et al., Citation2015). The results in normal animals paralleled those of septic animals. For example, when etomidate was administrated by constant-rate infusion (1.2 mg/kg/h) for 6 h, it increased adrenal apoptosis in normal rats (Liu et al., Citation2015). However, if the constant-rate infusion of etomidate was preceded by its administration as a bolus of 0.6 mg/kg, than apoptosis was prevented. The results of these studies seem to suggest that etomidate’s anti-apoptotic effects are manifest when the drug is administered at lower doses and before or very shortly after the beginning of the pathologic process. This is compatible with some sort of preconditioning effect induced by etomidate. In fact a preconditioning effect by etomidate to prevent apoptosis has been reported before and seems to involve the activation of the mitochondrial ATP-sensitive potassium channel (Zhang et al., Citation2010).
Etomidate was shown to directly affect apoptosis by several mechanisms. It can interfere directly with cytochrome c levels, increase the opening of the mitochondrial permeability transition pore and modulate the activity of several intracellular transcription factors involved in apoptosis (Liu et al., Citation2015; Wu et al., Citation2011; Zhang et al., Citation2010).
Alternatively etomidate can also affect apoptosis by its direct pro-oxidant effects (Wang et al., Citation2014; Yagmurdur et al., Citation2004). It also has direct activity upon intracellular anti-oxidant systems such as superoxide dismutase, catalase, and glutathione-peroxidase and modulates directly the inflammatory cascade (Liu et al., Citation2016; Zhang et al., Citation2010, Citation2015), including by a direct interference in the NF-kB pathway (Zhang et al., Citation2015). Studies have shown that etomidate’s effects upon oxidative stress and inflammation are also dose- and time-dependent and can also be pro or anti-apoptotic (Liu et al., Citation2015, Citation2016; Zhang et al., Citation2015). Furthermore, by attenuating I/R injury (Ergün, Darendeli, Imrek, Kilinç, & Oksüz, Citation2010; Yu et al., Citation2010), etomidate can also prevent apoptosis.
We believe that using etomidate at the doses of 1 mg/kg, immediately before inducing HS, we were able to prevent adrenal apoptosis in a similar manner to what was described in septic models. We hypothesize that the mechanisms involved could have included a direct preconditioning effect of etomidate or its effects in oxidative stress, I/R injury, and inflammation-related intracellular pathways. However, because the pathophysiology of HS is different from sepsis and our dose was higher than the one used in septic models, this hypothesis needs to be confirmed in future studies.
5.4. Etomidate’s indirect effects in adrenal apoptosis
5.4.1. Could have decreased CS levels contributed to the lower apoptotic rate of etomidate-treated animals?
As expected, etomidate-treated animals had lower levels of CS, because etomidate inhibits glucocorticoid adrenal production (Crozier, Beck, Schlaeger, Wuttke, & Kettler, Citation1987). We hypothesize that the decreased CS levels might have contributed to the decrease in adrenal apoptosis. In fact it has been reported that glucocorticoids augment adrenal apoptosis by increasing the levels of Bcl-2 and caspase-3 (Almeida et al., Citation2007) and especially in zona reticularis, which was the mostly affected by apoptosis in our study. Besides increased glucocorticoid levels were associated to increased parenchymal cell apoptosis in burns (Fukuzuka et al., Citation1999) and sepsis (Kamiyama et al., Citation2008).
5.4.2. Did increased ACTH levels contributed to the lower apoptotic rate of etomidate-treated animals?
ACTH levels were higher in etomidate-treated animals, possibly due to the decreased CS levels (Preziosi & Vacca, Citation1982), increased TNF-α and IL6 levels (John & Buckingham, Citation2003), and the higher degree of hypotension at T0 and T1 (Turnbull & Rivier, Citation1999). We believe that the higher ACTH levels could have contributed to the lower apoptotic rate of etomidate-treated animals. It is known that ACTH has anti-apoptotic effects in adrenal cells (Carsia, Macdonald, Gibney, Tilly, & Tilly, Citation1996; Keramidas, Feige, & Thomas, Citation2004), which are especially manifest in zona reticularis and zona fasciculata (Almeida, Matos, Ferreira, & Neves, Citation2006; Almeida et al., Citation2007; Carsia et al., Citation1996).
How ACTH prevents apoptosis is poorly understood. In part it results from its activation of the JAK/ERK/STAT pathway after ligating to the melanocortin receptors (Ottani et al., Citation2013; Si et al., Citation2013). In addition, ACTH activates the vagus nerve-mediated brain cholinergic anti-inflammatory pathway (Guarini et al., Citation2004). Through this way ACTH prevents vascular dysfunction (Bertuglia & Giusti, Citation2004), decreases leukocyte adhesion and its infiltration into tissues (Bertuglia & Giusti, Citation2004; Guarini et al., Citation1996; Squadrito et al., Citation1999) and inhibits the inflammatory cascade (Bazzani, Bertolini, & Guarini, Citation1997; Guarini et al., Citation2004; Squadrito et al., Citation1999) and ROS production (Guarini et al., Citation1996).
5.4.3. Did increased IL6 and IL10 levels contributed to the lower apoptotic rate of etomidate-treated animals?
Etomidate-treated animals had higher levels of TNF-α, IL6, and IL10 than other groups. Increased cytokine levels following etomidate administration have been previously described in rats (Félix et al., Citation2016; Pejo et al., Citation2012) and Humans (den Brinker et al., Citation2005; Jameson, Desborough, Bryant, & Hall, Citation1997) and they most likely result from etomidate’s suppression of CS production (Félix et al., Citation2016; Pejo et al., Citation2012). It is believed that inappropriately low serum and tissue levels of glucocorticoids can lead to an exaggeration of the immunological response to injury in etomidate-treated animals (Hermoso & Cidlowski, Citation2003; Pejo et al., Citation2012).
The influence of cytokines in apoptosis depends from several factors, including the type of cytokine and the target cell and/or tissue. TNF-α is known to be mainly pro-apoptotic in adrenal cells (Mikhaylova, Kuulasmaa, Jääskeläinen, & Voutilainen, Citation2007; Tkachenko, Jääskeläinen, Jääskeläinen, Palvimo, & Voutilainen, Citation2011). In contrast, to our knowledge, the effects of IL6 and IL10 in adrenal apoptosis have not yet been studied. Nevertheless, their preventive role against apoptosis has been well recognized in other occasions (Dhingra, Bagchi, Ludke, Sharma, & Singal, Citation2011; Moran et al., Citation2009; Rollwagen, Yu, Li, & Pacheco, Citation1998; Thacker, Robinson, Abel, & Tweardy, Citation2013; Thompson, Zurko, Hanna, Hellenbrand, & Hanna, Citation2013). IL6 prevents apoptosis by several mechanisms: by activating STAT3, a transcription factor which down-regulates the expression of pro-apoptotic genes (Hirano, Ishihara, & Hibi, Citation2000; Moran et al., Citation2009; Rollwagen et al., Citation1998; Thacker et al., Citation2013); by increasing the activity of DNA-repair systems (Centurione & Aiello, Citation2016); by suppressing cytosolic Ca2+ increase and oxidative stress; and by preventing mitochondrial dysfunction (Smart et al., Citation2006). Likewise, IL10 also activates STAT3 (Moore, de Waal Malefyt, Coffman, & O’Garra, Citation2001) and prevents apoptosis indirectly, by decreasing TNF-α, ILβ1, IFNγ (Fiorentino et al., Citation1991; Fuchs et al., Citation1996), glucocorticoid (Koldzic-Zivanovic et al., Citation2006), and ROS production (Thompson et al., Citation2013).
Based in these studies, we hypothesize that the increased IL6 and IL10 levels combined with the already cited etomidate’s direct anti-apoptotic effects, and associated increased ACTH and decreased CS levels counteracted the pro-apoptotic effects of HS, including those promoted by increased TNF-levels.
5.5. Apoptotic index was different accordingly to the different areas
The distribution of apoptosis was heterogeneous across the adrenal gland, being more severe in the cortex than in the medulla. In addition, apoptosis was also heterogeneously distributed across the adrenal cortex itself, being particularly predominant in zona reticularis. It was less present in the inner half of zona fasciculata and was infrequently found in zona glomerulosa. The heterogeneous distribution of apoptosis in the adrenal gland has also been described in other studies (Bozzo et al., Citation2006; Wolkersdörfer et al., Citation1996). For instances in rats, each adrenocortical zone has its specific and independent pattern of apoptosis (Bozzo et al., Citation2006) and this zone-specificity is maintained in the presence of exogenous pro-apoptotic stimulus. Two of these are dexamethasone administration and decreased ACTH levels (for example, by hypophysectomy) and rats submitted to these stimuli have increased rate of adrenocortical apoptosis which mainly affects zona reticularis (Almeida et al., Citation2007; Carsia et al., Citation1996).
The mechanisms behind the heterogeneous pattern of apoptosis in the adrenal gland are only partially understood. It may result from different paracrine and/or autocrine regulatory mechanisms which are specific to each adrenal zone (Bozzo et al., Citation2006). In addition, the adrenal distribution of proteins involved in the apoptotic process is not uniform (Vinson, Citation2016). Examples of this include superoxide dismutase (Vinson, Citation2016), the IL6 receptor, (mainly found in zona reticularis and inner zona fasciculata) (Päth, Bornstein, Ehrhart-Bornstein, & Scherbaum, Citation1997), thrombospondin-2 (absent from zona reticularis) (Feige, Keramidas, & Chambaz, Citation1998), and Bak (only found in adrenal cortex) (Krajewski, Krajewska, & Reed, Citation1996). In addition, more than 90% of the arterial blood supply to the adrenal cortex reaches first zona glomerulosa, and only after this, it arrives to zona reticularis and zona fasciculata. This pattern of arterial blood supply increases the risk of ischemia development in zona reticularis and zona fasciculata (Sparrow & Coupland, Citation1987), especially during low-flow states such as HS (Hamaji et al., Citation1986; Jasper, McDermott, Gann, & Engeland, Citation1990).
Two additional factors can predispose the inner adrenocortical zones to develop increased rates of apoptosis. The first is that zona reticularis and fasciculata have capillary walls with larger fenestrations than zona glomerulosa (Motta, Muto, & Fujita, Citation1979).This factor can facilitate the diffusion of pro-apoptotic molecules from the plasma to the adrenal interstitium. The second is that adrenal gland macrophages are especially abundant in the inner cortical regions (Almeida, Ferreira, & Neves, Citation2004). Due to pivotal role of macrophages in intra-adrenal inflammation and apoptosis (Wang et al., Citation2015) it is possible that this heterogeneous distribution also contributes to the higher apoptotic rate of the inner cortical regions.
5.6. HS increased adrenal gland necrosis
In our study, the degree and location of adrenal necrosis (preferentially affecting zona fasciculata and reticularis) were similar to what was found in previous experimental studies involving rats and dogs (Kajihara, Hirata, & Miyoshi, Citation1977; Kajihara et al., Citation1983; Rushing et al., Citation2006). What caused adrenal necrosis in our model remains undetermined. HS-associated adrenal ischemia is one major possibility as necrosis was especially predominant in the inner cortical areas (Rushing et al., Citation2006), as described before. Other possibilities include oxidative stress, I/R injury, and increased systemic and adrenal inflammation. Increased cytokines levels, in particular TNF-α, have been associated with increased cellular necrosis (Galluzzi et al., Citation2012; Vanlangenakker, Berghe, Krysko, Festjens, & Vandenabeele, Citation2008). Furthermore, an experimental study in dogs submitted to HS showed that adrenal necrosis was mainly apparent after fluid resuscitation (Kajihara et al., Citation1983) which may also suggest I/R injury as a contributing factor.
Adrenal necrosis could have also been due to necroptosis, an active form of necrosis which has been recognized in several critical illnesses (Duprez et al., Citation2011; Sharma, Matsuo, Yang, Wang, & Wang, Citation2014). This can be triggered by cytokines such as TNF-α, pathogen-associated molecular patterns, damage-associated molecular patterns, I/R injury, Ca2+ overload, hypoxia, infectious agents, DNA damage, and oxidative and nitrosative stress (Vanlangenakker et al., Citation2008). The presence of necroptosis was not investigated in our setting.
Etomidate-treated animals had a lower necrosis score than G2. The reason for this finding remains unclear. To our knowledge, no study has reported a protective effect of etomidate against cell necrosis. We hypothesize that etomidate might have prevented adrenal necrosis by the same mechanisms that prevented apoptosis, because apoptosis and necrosis share many pathophysiological mechanisms (Formigli et al., Citation2000).
5.7. Did adrenal gland apoptosis or necrosis cause CIRCI in our model?
In experimental septic and ANP models (Liu et al., Citation2016; Polito et al., Citation2010; Yu et al., Citation2012, Citation2016), adrenal apoptosis was associated to development of CIRCI. In our study, the results suggest that G2 animals could have also developed adrenal dysfunction at some degree. For instances, their CS levels were similar to those found in rats considered to have an inadequate adrenal response to HS (Rushing et al., Citation2006; Stein et al., Citation2013). In addition, in G2, the rise in ACTH levels from T2 to T3 was not associated to a correspondent increase of CS levels, suggesting a possible decreased adrenal responsiveness to ACTH’s stimulatory effects. In addition, adrenal apoptosis was mainly concentrated in the areas responsible for glucocorticoid production in rats (Vinson, Citation2016). We hypothesize based on other models (Liu et al., Citation2016; Polito et al., Citation2010; Rushing et al., Citation2006; Yu et al., Citation2012, Citation2016) that the development of adrenal apoptosis (associated to development of adrenal necrosis) could in part explain this adrenal dysfunction,. However, contrarily to what was described in septic and ANP models, the decrease in CS levels was not sufficiently low to classify the adrenal dysfunction as CIRCI, accordingly to current guidelines (Marik, Citation2009). The reason why our results differ from the septic and ANP studies remains unknown but it could be related with differences in experimental design and methodology. Besides, we cannot rule out that maybe the pathophysiology of adrenal apoptosis is different between HS and sepsis or ANP.
In contrast, etomidate-treated animals had CS levels compatible with the diagnosis of CIRCI in Humans (Marik, Citation2009). In G3, we believe that the decreased CS levels were mainly due to the effects of etomidate in CS production. However previous studies have also shown that even in rats treated with etomidate, CIRCI resulted in part from adrenal apoptosis (Liu et al., Citation2015, Citation2016). Thus, we cannot exclude that adrenal apoptosis contributed to CIRCI in G3’s animals. However, if it did, we believe that it played a minor role, because etomidate-treated animals had a lower apoptotic rate.
5.7.1. Implications
Our findings regarding etomidate effects upon adrenal apoptosis and necrosis open the possibility of this drug being used to modulate the adrenal apoptotic and necrotic processes in HS. This modulation can be advantageous to clarify their role in CIRCI development during HS and eventually clinically beneficial. However, before we consider using etomidate therapeutically for this purpose, one should consider that the anesthetic was associated to significant morbidity, including a higher degree of hypoperfusion, hypoxemia, and lung injury, as described more in detail in a previous study by our group (Félix et al., Citation2016). The latter study and others performed in septic models (Pejo et al., Citation2012) suggested that this increased morbidity was partially associated to the significant increase in cytokine levels. This in turn might have resulted from the lower levels of glucocorticoids caused by etomidate, which allowed the immune system to act without restraint. Thus, the literature suggests a cautious approach for the use of etomidate in HS. If the balance between the desired and adverse effects of etomidate administration in HS is time- and dose-dependent as described in septic models can only be answered by future studies.
5.8. Limitations of the study
This study had some limitations. The first was the use of a limited number of animals. In addition the animals were exclusively young male Wistar rats, limiting the translation of our results to other species. Besides, the response to HS is strongly influenced by sex, strain, and age of animals (Klemcke et al., Citation2011; Mees et al., Citation2008). We also induced a mild state of HS. It is possible that a severer and/or a longer model of HS can lead to a higher degree of apoptosis and adrenal dysfunction.
How etomidate modulated apoptosis was not defined. Future studies should address this issue, possibly using different doses of etomidate, its administration at different time points, and eventually by manipulating factors such as ACTH and cytokine levels.
In addition, although the methods chosen to identify the presence of apoptosis and necrosis are commonly used for this purpose, they do not provide information regarding its underlying mechanisms. The quantification of gene expression of bax and bcl2 by PCR, the subsequent calculation of the bax/bcl2 ratio, and the determination of intracellular factors involved in the apoptotic and necrotic pathways could be useful in this context and should be considered in future studies.
6. Conclusion
In this study, a mild form of HS was associated to development of adrenal gland apoptosis and necrosis. Apoptosis was heterogeneously distributed, affecting mostly the adrenal cortex and in particular, zona reticularis and zona fasciculata. Etomidate was associated to decrease rate of adrenal apoptosis, necrosis, and CS levels (compatible with CIRCI). The development of adrenal apoptosis and necrosis was not clearly associated to CIRCI. Etomidate could have modulated apoptosis and necrosis directly and/or indirectly, by decreasing CS levels and by contributing to increased IL6, IL10, and ACTH levels. Future studies should be performed to confirm and clarify the mechanisms of these findings, and eventually to explore the use of etomidate in preventing adrenal apoptosis and necrosis in HS.
Supplementary material
Supplementary material for this article can be accessed here https://doi.org/10.1080/23312025.2017.1393864.
Correction
This article was originally published with errors. This version has been corrected/amended as follows: Competing statement was not included. Now this has been included.
OABI_1393864_Supplementary_Material.doc
Download MS Word (81 KB)Acknowledgements
The authors would like to thank Dr Jeanne Kehren for her assistance and technical advice to prepare the final version of the manuscript and Prof Solange Gil, Prof Luísa Mateus, Dr Sofia Gomes, and Dr Tiago Rafael for their contributions to conduct the experimental procedure.
Additional information
Funding
Notes on contributors
Nuno M. Félix
Nuno M. Félix was licensed as Doctor in Veterinary Medicine in 1999 by the Lisbon Faculty of Veterinary Medicine, completed his master of science (Neuroscience) in 2004 by the Lisbon Faculty of Medicine, became human medical doctor by the Lisbon Faculty of Medicine in 2011, and finished his PhD about hemorrhagic shock in 2017, by the Lisbon Faculty of Veterinary Medicine. He worked as assistant professor of Small Animal Internal Medicine in the same faculty from 2001 to 2014 and is currently a human doctor, resident in Pediatric Medicine. The study here reported was related with his PhD research project, which aimed to evaluate endocrine changes associated to hemorrhagic shock. He and his research group continue to research several critical illnesses in small animals and how these can be translated in the Human field, with clinical benefits for all species.
References
- Allolio, B., Dörr, H., Stuttmann, R., Knorr, D., Engelhardt, D., & Winkelmann, W. (1985). Effect of a single bolus of etomidate upon eight major corticosteroid hormones and plasma ACTH. Clinical Endocrinology, 22(3), 281–286.10.1111/j.1365-2265.1985.tb03241.x
- Almeida, H., Ferreira, J., & Neves, D. (2004). Macrophages of the adrenal cortex: A morphological study of the effects of aging and dexamethasone administration. Annals of the New York Academy of Sciences, 1019, 135–140.10.1196/annals.1297.024
- Almeida, H., Matos, L., Ferreira, J., & Neves, D. (2006). Age-related effects of dexamethasone administration in adrenal zona reticularis. Annals of the New York Academy of Sciences, 1067, 354–360.10.1196/annals.1354.050
- Almeida, H., Matos, L., & Neves, D. (2007). Caspase-3 and Bcl-2 expression in aging in adrenal zona reticularis after dexamethasone administration. Annals of the New York Academy of Sciences, 1119, 190–195.10.1196/annals.1404.021
- Bazzani, C., Bertolini, A., & Guarini, S. (1997). Inhibition of nitric oxide synthases enhances the effect of ACTH in hemorrhagic shock. Life Sciences, 61(19), 1889–1897.10.1016/S0024-3205(97)00828-X
- Bertuglia, S., & Giusti, A. (2004). Influence of ACTH-(1-24) and plasma hyperviscosity on free radical production and capillary perfusion after hemorrhagic shock. Microcirculation, 11(3), 227–238.10.1080/10739680490425930
- Bozzo, A., Soñez, C. A., Mugnaini, M. T., Pastorino, I. C., Rolando, A. N., Romanini, M. C., & Gauna, H. F. (2006). Chronic stress effects on the apoptotic index of the adrenal cortex of pregnant rats. Biocell, 30(3), 439–445.
- Bruder, E. A., Ball, I. M., Ridi, S., Pickett, W., & Hohl, C. (2015). Single induction dose of etomidate versus other induction agents for endotracheal intubation in critically ill patients. The Cochrane database of systematic reviews, 1, CD010225.
- Carsia, R. V., Macdonald, G. J., Gibney, J. A., Tilly, K. I., & Tilly, J. L. (1996). Apoptotic cell death in the rat adrenal gland: An in vivo and in vitro investigation. Cell Tissue Research, 283, 247–254.10.1007/s004410050535
- Centurione, L., & Aiello, F. B. (2016). DNA repair and cytokines: TGF-β, IL-6, and thrombopoietin as different biomarkers of radioresistance. Frontiers Oncology, 6, 175.
- Cherfan, A. J., Tamim, H. M., AlJumah, A., Rishu, A. H., Al-Abdulkareem, A., Al Knawy, B. A., … Arabi, Y. M. (2011). Etomidate and mortality in cirrhotic patients with septic shock. BMC Clinical Pharmacology, 11, 22.10.1186/1472-6904-11-22
- Cotton, B. A., Guillamondegui, O. D., Fleming, S. B., Carpenter, R. O., Patel, S. H., Morris, Jr., J. A., & Arbogast, P. G. (2008). Increased risk of adrenal insufficiency following etomidate exposure in critically injured patients. Archives of Surgery, 143, 62–67.10.1001/archsurg.143.1.62
- Crozier, T. A., Beck, D., Schlaeger, M., Wuttke, W., & Kettler, D. (1987). Endocrinological changes following etomidate, midazolam, or methohexital for minor surgery. Anesthesiology, 66, 628–635.10.1097/00000542-198705000-00006
- den Brinker, M., Joosten, K. F., Liem, O., de Jong, F. H., Hop, W. C., Hazelzet, J. A., … Hokken-Koelega, A. C. (2005). Adrenal insufficiency in meningococcal sepsis: Bioavailable cortisol levels and impact of interleukin-6 levels and intubation with etomidate on adrenal function and mortality. The Journal of Clinical Endocrinology & Metabolism, 90, 5110–5117.10.1210/jc.2005-1107
- den Brinker, M., Hokken-Koelega, A. C., Hazelzet, J. A., de Jong, F. H., Hop, W. C., & Joosten, K. F. (2008). One single dose of etomidate negatively influences adrenocortical performance for at least 24 h in children with meningococcal sepsis. Intensive Care Medicine, 34, 163–168.10.1007/s00134-007-0836-3
- Dhingra, S., Bagchi, A. K., Ludke, A. L., Sharma, A. K., & Singal, P. K. (2011). Akt regulates IL-10 mediated suppression of TNFα-induced cardiomyocyte apoptosis by upregulating Stat3 phosphorylation. PLoS ONE, 6, e25009.10.1371/journal.pone.0025009
- Didenko, V. V., Wang, X., Yang, L., & Hornsby, P. J. (1996). Expression of p21 (WAF1/CIP1/SDI1) and p53 in apoptotic cells in the adrenal cortex and induction by ischemia/reperfusion injury. Journal of Clinical Investigation, 97, 1723–1731.10.1172/JCI118599
- Duprez, L., Takahashi, N., Van Hauwermeiren, F., Vandendriessche, B., Goossens, V., Vanden Berghe, T., … Vandenabeele, P. (2011). RIP kinase-dependent necrosis drives lethal systemic inflammatory response syndrome. Immunity, 35(6), 908–918.10.1016/j.immuni.2011.09.020
- Ergün, Y., Darendeli, S., Imrek, S., Kilinç, M., & Oksüz, H. (2010). The comparison of the effects of anesthetic doses of ketamine, propofol, and etomidate on ischemia-reperfusion injury in skeletal muscle. Fundamentals of Clinical Pharmacology, 24(2), 215–222.
- Feige, J. J., Keramidas, M., & Chambaz, E. M. (1998). Hormonally regulated components of the adrenocortical cell environment and the control of adrenal cortex homeostasis. Hormone and Metabolic Research, 30, 421–425.10.1055/s-2007-978908
- Félix, N. M., Goy-Thollot, I., Walton, R. S., Gil, S. A., Mateus, L. M., Matos, A. S., & Niza, M. M. R. E. (2016). Effects of etomidate in the adrenal and cytokine responses to hemorrhagic shock in rats. European Journal of Inflammation, 14(3), 147–161. doi:10.1177/1721727X16677604
- Fiorentino, D. F., Zlotnik, A., Vieira, P., Mosmann, T. R., Howard, M., Moore, K. W., & O’Garra, A. (1991). IL-10 acts on the antigen-presenting cell to inhibit cytokine production by Th1 cells. Journal of Immunology, 146, 3444–3451.
- Flierl, M. A., Rittirsch, D., Chen, A. J., Nadeau, B. A., Day, D. E., Sarma, J. V., … Ward, P. A. (2008). The complement anaphylatoxin C5a induces apoptosis in adrenomedullary cells during experimental sepsis. PLoS ONE, 3, e2560. doi:10.1371/journal.pone.0002560
- Forman, S. A. (2011). Clinical and molecular pharmacology of etomidate. Anesthesiology, 114, 695–707.10.1097/ALN.0b013e3181ff72b5
- Formigli, L., Papucci, L., Tani, A., Schiavone, N., Tempestini, A., Orlandini, G. E., … Orlandini, S. Z. (2000). Aponecrosis: Morphological and biochemical exploration of a syncretic process of cell death sharing apoptosis and necrosis. Journal of Cellular Physiology, 182, 41–49.10.1002/(ISSN)1097-4652
- Fuchs, A. C., Granowitz, E. V., Shapiro, L., Vannier, E., Lonnemann, G., Angel, J. B., … Dinarello, C. A. (1996). Clinical, hematologic, and immunologic effects of interleukin-10 in humans. Journal of Clinical Immunology, 16, 291–303.10.1007/BF01541395
- Fukuzuka, K., Rosenberg, J. J., Gaines, G. C., Edwards, C. K., 3rd, Clare-Salzler, M., MacKay, S. L., … Mozingo, D. W. (1999). Caspase-3–dependent organ apoptosis early after burn injury. Annals of Surgery, 229, 851–858.10.1097/00000658-199906000-00012
- Galluzzi, L., Vitale, I., Abrams, J. M., Alnemri, E. S., Baehrecke, E. H., Blagosklonny, M. V., … Kroemer, G. (2012). Molecular definitions of cell death subroutines: Recommendations of the Nomenclature Committee on Cell Death 2012. Cell Death and Differentiation, 19, 107–120.10.1038/cdd.2011.96
- Guarini, S., Bazzani, C., Ricigliano, G. M., Bini, A., Tomasi, A., & Bertolini, A. (1996). Influence of ACTH-(1-24) on free radical levels in the blood of haemorrhage-shocked rats: Direct ex vivo detection by electron spin resonance spectrometry. British Journal of Pharmacology, 119(1), 29–34.10.1111/bph.1996.119.issue-1
- Guarini, S., Cainazzo, M. M., Giuliani, D., Mioni, C., Altavilla, D., Marini, H., … Bertolini, A. (2004). Adrenocorticotropin reverses hemorrhagic shock in anesthetized rats through the rapid activation of a vagal anti-inflammatory pathway. Cardiovascular Research, 63(2), 357–365.10.1016/j.cardiores.2004.03.029
- Hamaji, M., Nakamura, M., Izukura, M., Nakaba, H., Hashimoto, T., Tanaka, Y., … Harrison, T. S. (1986). Autoregulation and regional blood flow of the dog during hemorrhagic shock. Circulatory Shock, 19(3), 245–255.
- Hermoso, M. A., & Cidlowski, J. A. (2003). Putting the brake on inflammatory responses: The role of glucocorticoids. IUBMB Life (International Union of Biochemistry and Molecular Biology: Life), 55, 497–504.10.1080/15216540310001642072
- Hirano, T., Ishihara, K., & Hibi, M. (2000). Roles of STAT3 in mediating the cell growth, differentiation and survival signals relayed through the IL-6 family of cytokine receptors. Oncogene, 19, 2548–2556.10.1038/sj.onc.1203551
- Hoen, S., Asehnoune, K., Brailly-Tabard, S., Mazoit, J. X., Benhamou, D., Moine, P., & Edouard, A. R. (2002). Cortisol response to corticotropin stimulation in trauma patients: Influence of hemorrhagic shock. Anesthesiology, 97, 807–813.10.1097/00000542-200210000-00010
- Jameson, P., Desborough, J. P., Bryant, A. E., & Hall, G. M. (1997). The effect of cortisol suppression on interleukin-6 and white blood cell responses to surgery. Acta Anaesthesiologica Scandinavica, 41, 304–308.10.1111/aas.1997.41.issue-2
- Jasper, M. S., McDermott, P., Gann, D. S., & Engeland, W. C. (1990). Measurement of blood flow to the adrenal capsule, cortex and medulla in dogs after hemorrhage by fluorescent microspheres. Journal of the Autonomic Nervous System, 30(2), 159–167.10.1016/0165-1838(90)90140-E
- John, C. D., & Buckingham, J. C. (2003). Cytokines: Regulation of the hypothalamo-pituitary-adrenocortical axis. Current Opinion in Pharmacology, 3(1), 78–84.10.1016/S1471-4892(02)00009-7
- Kajihara, H., Hirata, S., & Miyoshi, N. (1977). Changes in blood catecholamine levels and ultrastructure of dog adrenal medullary cells during hemorrhagic shock. Virchows Archives B Cell Pathology, 23(1), 1–16.
- Kajihara, H., Malliwah, J. A., Matsumura, M., Taguchi, K., & Iijima, S. (1983). Changes in blood cortisol and aldosterone levels and ultrastructure of the adrenal cortex during hemorrhagic shock. Pathology, Research and Practice, 176, 324–340.10.1016/S0344-0338(83)80022-3
- Kamiyama, K., Matsuda, N., Yamamoto, S., Takano, K., Takano, Y., Yamazaki, H., … Hattori, Y. (2008). Modulation of glucocorticoid receptor expression, inflammation, and cell apoptosis in septic guinea pig lungs using methylprednisolone. AJP: Lung Cellular and Molecular Physiology, 295, 998–1006.10.1152/ajplung.00459.2007
- Kanczkowski, W., Chatzigeorgiou, A., Grossklaus, S., Sprott, D., Bornstein, S. R., & Chavakis, T. (2013). Role of the endothelial-derived endogenous anti-inflammatory factor Del-1 in inflammation-mediated adrenal gland dysfunction. Endocrinology, 154(3), 1181–1189.10.1210/en.2012-1617
- Kanczkowski, W., Chatzigeorgiou, A., Samus, M., Tran, N., Zacharowski, K., Chavakis, T., & Bornstein, S. R. (2013). Characterization of the LPS-induced inflammation of the adrenal gland in mice. Molecular and Cellular Endocrinology, 371, 228–235.10.1016/j.mce.2012.12.020
- Keramidas, M., Feige, J. J., & Thomas, M. (2004). Coordinated regression of adrenocortical endocrine and endothelial compartments under adrenocorticotropin deprivation. Endocrine Research, 30(4), 543–549.10.1081/ERC-200043623
- Klemcke, H. G., Joe, B., Calderon, M. L., Rose, R., Oh, T., Aden, J., & Ryan, K. L. (2011). Genetic influences on survival time after severe haemorrhage in inbred rat strains. Physiological Genomics, 43, 758–765.10.1152/physiolgenomics.00245.2010
- Koldzic-Zivanovic, N., Tu, H., Juelich, T. L., Rady, P. L., Tyring, S. K., Hudnall, S. D., … Hughes, T. K. (2006). Regulation of adrenal glucocorticoid synthesis by interleukin-10: A preponderance of IL-10 receptor in the adrenal zona fasciculata. Brain, Behavior, and Immunity, 20(5), 460–468.10.1016/j.bbi.2005.09.003
- Krajewski, S., Krajewska, M., & Reed, J. C. (1996). Immunohistochemical analysis of in vivo patterns of Bak expression, a proapoptotic member of the Bcl-2 protein family. Cancer Research, 56, 2849–2855.
- Kugawa, F., Arae, K., Ueno, A., & Aoki, M. (1998). Buprenorphine hydrochloride induces apoptosis in NG108-15 nerve cells. European Journal of Pharmacology, 347, 105–112.10.1016/S0014-2999(98)00080-6
- Kugawa, F., Nakamura, M., Ueno, A., & Aoki, M. (2004). Over-expressed Bcl-2 cannot suppress apoptosis via the mitochondria in buprenorphine hydrochloride-treated NG108-15 cells. Biological & Pharmaceutical Bulletin, 27, 1340–1347.10.1248/bpb.27.1340
- Liu, N., Zhang, Y., Xiong, J. Y., Liu, S., Zhu, J., & Lv, S. (2016). The pituitary adenylate cyclase-activating polypetide (PACAP) protects adrenal function in septic rats administered etomidate. Neuropeptides, 58, 53–59.10.1016/j.npep.2016.03.005
- Liu, S., Zhang, X. P., Han, N. N., Lv, S., & Xiong, J. Y. (2015). Pretreatment with low dose etomidate prevents etomidate-induced rat adrenal insufficiency by regulating oxidative stress-related MAPKs and apoptosis. Environmental Toxicology and Pharmacology, 39(3), 1212–1220.10.1016/j.etap.2015.04.014
- Marik, P. E. (2009). Critical illness-related corticosteroid insufficiency. Chest, 135, 181–193.10.1378/chest.08-1149
- Mees, S. T., Gwinner, M., Marx, K., Faendrich, F., Schroeder, J., Haier, J., & Kahlke, V. (2008). Influence of sex and age on morphological organ damage after hemorrhagic shock. Shock, 29, 670–674.
- Mikhaylova, I. V., Kuulasmaa, T., Jääskeläinen, J., & Voutilainen, R. (2007). Tumor necrosis factor-alpha regulates steroidogenesis, apoptosis, and cell viability in the human adrenocortical cell line NCI-H295R. Endocrinology, 148(1), 386–392.10.1210/en.2006-0726
- Moore, K. W., de Waal Malefyt, R., Coffman, R. L., & O’Garra, A. (2001). Interleukin-10 and the interleukin-10 receptor. Annual Review of Immunology, 19, 683–765.10.1146/annurev.immunol.19.1.683
- Moran, A., Tsimelzon, A. I., Mastrangelo, M. A., Wu, Y., Yu, B., Hilsenbeck, S. G., … Tweardy, D. J. (2009). Prevention of trauma/hemorrhagic shock-induced lung apoptosis by IL-6-mediated activation of Stat3. Clinical and Translational Science, 2, 41–49.10.1111/cts.2009.2.issue-1
- Mosier, M. J., Lasinski, A. M., & Gamelli, R. L. (2015). Suspected adrenal insufficiency in critically ill burned patients: Etomidate-induced or critical illness-related corticosteroid insufficiency?-A review of the literature. Journal of Burn & Care Research, 36(2), 272–278.10.1097/BCR.0000000000000099
- Motta, P., Muto, M., & Fujita, T. (1979). Three-dimensional organization of mammalian adrenal cortex. A scanning electron microscopic study. Cell Tissue Research, 30, 23–38.
- Ottani, A., Galantucci, M., Ardimento, E., Neri, L., Canalini, F., Calevro, A., … Guarini, S. (2013). Modulation of the JAK/ERK/STAT signaling in melanocortin-induced inhibition of local and systemic responses to myocardial ischemia/reperfusion. Pharmacological Research, 72, 1–8.10.1016/j.phrs.2013.03.005
- Papathanassoglou, E. D., Moynihan, J. A., & Ackerman, M. H. (2000). Does programmed cell death (apoptosis) play a role in the development of multiple organ dysfunction in critically ill patients? A review and a theoretical framework. Critical Care Medicine, 28, 537–549.10.1097/00003246-200002000-00042
- Päth, G., Bornstein, S. R., Ehrhart-Bornstein, M., & Scherbaum, W. A. (1997). Interleukin-6 and the interleukin-6 receptor in the human adrenal gland: Expression and effects on steroidogenesis. Journal of Clinical Endocrinology and Metabolism, 82(7), 2343–2349.
- Pejo, E., Feng, Y., Chao, W., Cotten, J. F., Le Ge, R., & Raines, D. E. (2012). Differential effects of etomidate and its pyrrole analogue carboetomidate on the adrenocortical and cytokine responses to endotoxemia. Critical Care Medicine, 40, 187–192.10.1097/CCM.0b013e31822d7924
- Polito, A., Lorin de la Grandmaison, G., Mansart, A., Louiset, E., Lefebvre, H., Sharshar, T., & Annane, D. (2010). Human and experimental septic shock are characterized by depletion of lipid droplets in the adrenals. Intensive Care Medicine, 36, 1852–1858.10.1007/s00134-010-1987-1
- Porter, A. G., & Jänicke, R. U. (1999). Emerging roles of caspase-3 in apoptosis. Cell Death Differentiation, 6, 99–104.10.1038/sj.cdd.4400476
- Preziosi, P., & Vacca, M. (1982). Etomidate and corticotrophic axis. Archives Internationales de Pharmacodynamie et de Therapie, 256, 308–310.
- Roesslein, M., Schibilsky, D., Muller, L., Goebel, U., Schwer, C., Humar, M., … Loop, T. (2008). Thiopental protects human T lymphocytes from apoptosis in vitro via the expression of heat shock protein 70. Journal of Pharmacology and Experimental Therapeutics, 325, 217–225.10.1124/jpet.107.133108
- Rollwagen, F. M., Yu, Z. Y., Li, Y. Y., & Pacheco, N. D. (1998). IL-6 rescues enterocytes from hemorrhage induced apoptosis in vivo and in vitro by a bcl-2 mediated mechanism. Clinical Immunology Immunopathology, 89, 205–213.10.1006/clin.1998.4600
- Rushing, G. D., & Britt, L. D. (2007). Inhibition of NF-KB does not induce c-Jun N-Terminal kinase-mediated apoptosis in reperfusion injury. Journal of the American College of Surgeons, 204(5), 964–967.10.1016/j.jamcollsurg.2007.01.029
- Rushing, G. D., Britt, R. C., & Britt, L. D. (2006). Effects of hemorrhagic shock on adrenal response in a rat model. Annals of Surgery, 243, 652–656.10.1097/01.sla.0000216759.36819.1b
- Sharma, A., Matsuo, S., Yang, W. L., Wang, Z., & Wang, P. (2014). Receptor-interacting protein kinase 3 deficiency inhibits immune cell infiltration and attenuates organ injury in sepsis. Critical Care, 18(4), R142.10.1186/cc13970
- Si, J., Ge, Y., Zhuang, S., Wang, L.J., Chen, S., & Gong, R. (2013). Adrenocorticotropic hormone ameliorates acute kidney injury by steroidogenic-dependent and -independent mechanisms. Kidney International, 83, 635–646.10.1038/ki.2012.447
- Smart, N., Mojet, M. H., Latchman, D. S., Marber, M. S., Duchen, M. R., & Heads, R. J. (2006). IL-6 induces PI 3-kinase and nitric oxide-dependent protection and preserves mitochondrial function in cardiomyocytes. Cardiovascular Research, 69, 164–177.10.1016/j.cardiores.2005.08.017
- Sparrow, R. A., & Coupland, R. E. (1987). Blood flow to the adrenal gland of the rat: Its distribution between the cortex and the medulla before and after haemorrhage. Journal Anatomy, 155, 51–61.
- Squadrito, F., Guarini, S., Altavilla, D., Squadrito, G., Campo, G. M., Arlotta, M., … Caputi, A. P. (1999). Adrenocorticotropin reverses vascular dysfunction and protects against splanchnic artery occlusion shock. British Journal of Pharmacology, 128(3), 816–822.10.1038/sj.bjp.0702848
- Stein, D. M., Jessie, E. M., Crane, S., Kufera, J. A., Timmons, T., Rodriguez, C. J., … Scalea, T. M. (2013). Hyperacute adrenal insufficiency after hemorrhagic shock exists and is associated with poor outcomes. Journal of Trauma Acute Care Surgery, 74(2), 363–370.10.1097/TA.0b013e31827e2aaf
- Thacker, S. A., Robinson, P., Abel, A., & Tweardy, D. J. (2013). Modulation of the unfolded protein response during hepatocyte and cardiomyocyte apoptosis in trauma/hemorrhagic shock. Scientific Reports, 3, 1.10.1038/srep01187
- Thompson, C. D., Zurko, J. C., Hanna, B. F., Hellenbrand, D. J., & Hanna, A. (2013). The therapeutic role of interleukin-10 after spinal cord injury. Journal of Neurotrauma, 30(15), 1311–1324.10.1089/neu.2012.2651
- Tkachenko, I. V., Jääskeläinen, T., Jääskeläinen, J., Palvimo, J. J., & Voutilainen, R. (2011). Interleukins 1α and 1β as regulators of steroidogenesis in human NCI-H295R adrenocortical cells. Steroids, 76(10-11), 1103–1115.10.1016/j.steroids.2011.04.018
- Turnbull, A. V., & Rivier, C. L. (1999). Regulation of the hypothalamic-pituitary-adrenal axis by cytokines: Actions and mechanisms of action. Physiological Reviews, 79(1), 1–71.
- Vanlangenakker, N., Berghe, T. V., Krysko, D. V., Festjens, N., & Vandenabeele, P. (2008). Molecular mechanisms and pathophysiology of necrotic cell death. Current Molecular Medicine, 8, 207–220.10.2174/156652408784221306
- Vignali, D.A. (2000). Multiplexed particle-based flow cytometric assays. Journal of Immunological Methods, 243, 243–255.10.1016/S0022-1759(00)00238-6
- Vinson, G. P. (2016). Functional zonation of the adult mammalian adrenal cortex. Frontiers Neuroscience, 10, 238.
- Wagner, R. L., White, P. F., Kan, P. B., Rosenthal, M. H., & Feldman, D. (1984). Inhibition of adrenal steroidogenesis by the anesthetic etomidate. New England Journal of Medicine, 310(22), 1415–1421.10.1056/NEJM198405313102202
- Wang, P., Ba, Z. F., Jarrar, D., Cioffi, W.G., Bland, K. I., & Chaudry, I. H. (1999). Mechanism of adrenal insufficiency following trauma and severe hemorrhage: Role of hepatic 11beta-hydroxysteroid dehydrogenase. Archives of Surgery, 134, 394–401.10.1001/archsurg.134.4.394
- Wang, C. N., Duan, G. L., Liu, Y. J., Yu, Q., Tang, X. L., Zhao, W., … Ni, X. (2015). Overproduction of nitric oxide by endothelial cells and macrophages contributes to mitochondrial oxidative stress in adrenocortical cells and adrenal insufficiency during endotoxemia. Free Radical Biology & Medicine, 83, 31–40.10.1016/j.freeradbiomed.2015.02.024
- Wang, X., Jin, A., An, M., Ding, Y., Tuo, Y., & Qiu, Y. (2014). Etomidate deteriorates the toxicity of advanced glycation end products to human endothelial Eahy926 cells. The Journal of Toxicological Sciences, 39(6), 887–896.10.2131/jts.39.887
- Wolkersdörfer, G. W., Ehrhart-Bornstein, M., Brauer, S., Marx, C., Scherbaum, W. A., & Bornstein, S. R. (1996). Differential regulation of apoptosis in the normal human adrenal gland. Journal Clinical Endocrinology Metabolism, 81, 4129–4136.
- Wu, R. S., Wu, K. C., Yang, J. S., Chiou, S. M., Yu, C. S., Chang, S. J., … Chung, J. G. (2011). Etomidate induces cytotoxic effects and gene expression in a murine leukemia macrophage cell line (RAW264.7). Anticancer Research, 31, 2203–2208.
- Xu, H., Chen, S., Luo, W., & Firoj, K. M. (2014). Effect of stress on myocardial apoptosis in ischemic preconditioning in rabbit hearts. Zhong Nan da Xue Xue Bao Yi Xue Ban, 39, 477–482.
- Yagmurdur, H., Cakan, T., Bayrak, A., Arslan, M., Baltaci, B., Inan, N., & Kilinc, K. (2004). The effects of etomidate, thiopental and propofol in induction on hypoperfusion-reperfsuion phenomenon during laparoscopic cholecystectomy. Acta Anaesthesiologica Scandinavica, 48(6), 772–777.10.1111/aas.2004.48.issue-6
- Yu, J., Xu, S., Wang, W. X., Deng, W. H., Jin, H., Chen, X. Y., … Sun, H. T. (2012). Changes of inflammation and apoptosis in adrenal gland after experimental injury in rats with acute necrotizing pancreatitis. Inflammation, 35, 11–22.10.1007/s10753-010-9284-2
- Yu, J., Zuo, T., Deng, W., Shi, Q., Ma, P., Chen, C., … Wang, W. (2016). Poly(ADP-ribose) polymerase inhibition suppresses inflammation and promotes recovery from adrenal injury in a rat model of acute necrotizing pancreatitis. BMC Gastroenterology, 16(1), 11.10.1186/s12876-016-0493-5
- Yu, Q., Zhou, Q., Huang, H., Wang, Y., Tian, S., & Duan, D. (2010). Protective effect of etomidate on spinal cord ischemia–reperfusion injury induced by aortic occlusion in rabbits. Annals of Vascular Surgery, 24(2), 225–232.10.1016/j.avsg.2009.06.023
- Zhang, X., Xiong, J., Jiao, Y., Wang, G., & Zuo, Z. (2010). Involvement of mitochondrial ATP-sensitive potassium channels in etomidate preconditioning-induced protection in human myeloid HL-60 cells. Environmental Toxicology and Pharmacology, 29(3), 320–322.10.1016/j.etap.2010.02.003
- Zhang, Y., Li, R., Zhu, J., Wang, Z., Lv, S., & Xiong, J. Y. (2015). Etomidate increases mortality in septic rats through inhibition of nuclear factor kappa-B rather than by causing adrenal insufficiency. Journal of Surgical Research, 193(1), 399–406.10.1016/j.jss.2014.07.001