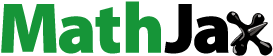
Abstract
Asthma is a common and prevalent health problem, globally affecting over 300 million individuals. Observational and intervention studies have shown beneficial effects of omega-3 [Eicosapentaenoic Acid (EPA) and Docosahexaenoic acid (DHA)] on asthma and exercise-induced bronchoconstriction (EIB). Due to health side effects with pharmacological medication, use of complementary therapies including omega-3 supplementation is gaining impetus. A double-blinded randomised crossover pilot study with 3 weeks of supplementation (3.2 g EPA and 2.2 g DHA or placebo) was conducted to assess the effect of omega-3 supplementation in physically active males with EIB (n = 9, 21 ± 0.9 years, Forced Expiratory Volume in 1-s/Forced Vital Capacity (FEV1/FVC) = 77 ± 1.4). At the start of study, participants showed abnormal lung function, typical drop of >10% in their FEV1 following exercise-challenge tests; elevated levels of Exhaled-breath Nitric oxide, FeNO (>40 ppb). The 3-week supplementation resulted in a significant improvement in post-exercise pulmonary function (PF) (<10% drop in post-exercise FEV1/FVC), supported by significant reduction in serum IL-6 levels (37% reduction). Although no significant changes were observed for Peripheral Blood Mononuclear Cell (PBMC) total lipid composition for EPA/DHA, non-significant increase in total PBMC EPA/DHA with reduction in omega-6 fatty acid (Arachidonic Acid) was observed. This pilot study shows a beneficial effect of 3 weeks of omega-3 supplementation on PF for EIB-participants.
Public Interest Statement
Fish oil has received much attention within the mainstream press with regard to its beneficial impacts upon health and well-being. This article presents data from a pilot study which focuses upon the impact of 3 weeks of dietary supplementation with daily doses of fish oil (comprising of omega-3 fatty acids) in a group of physically active male participants exercising 2–4 times/week, who suffer from clinically diagnosed asthma and exercise-induced asthma. The results of this study suggest that dietary supplementation with omega-3 could improve exercise-induced asthma symptoms in physically active males; however, a larger population-based study is warranted to confirm the findings and further evaluate the effect of omega-3 on associated markers of inflammation.
Competing interests
The authors declare no competing interests.
1. Background
Asthma affects over 300 million individuals worldwide and continues to be a major health problem globally. It has been reported that up to 18% of the world’s population is affected by asthma (Ober & Yao, Citation2011), while the increase in prevalence in developing countries is attributed to the adoption of a “western lifestyle”, as populations become more urbanised (Bousquet, Godard, & Daures, Citation2005; GINA, Citation2011). Asthma is partly characterised by transient narrowing of the airways (Anderson & Holzer, Citation2000; McFadden & Gilbert, Citation1994) leading to a reduction in pulmonary function (PF). The main diagnostic technique for asthma is an assessment of PF to identify airflow limitation; this method has been used to demonstrate the reversibility of lung function abnormalities. On its own, the lung function test does not lead the clinicians directly to an aetiological diagnosis and requires other measurements for confidence. The measurement of PF is combined with an assessment of symptoms such as dyspnoea and wheezing, together they provide reliable information about the different aspects of asthma control (Miller, Crapo, et al., Citation2005; Miller, Hankinson, et al., Citation2005). Spirometry is the primary method for pulmonary function test (PFT). The most important aspects of spirometry include forced vital capacity (FVC), which is the maximum volume of air an individual can expel from the lungs, during expiration made as forcefully and completely starting from full inspiration. The forced expiratory volume (FEV1) in one second, which is the maximum volume of expired air volume in the first second of a FVC manoeuvre. Predicted values of FEV1, FVC and PEF based on age, sex and height have been derived from population studies (Hankinson, Odencrantz, & Fedan, Citation1999). Conventionally, a fixed criteria of ≤80% (for FEV1/FVC ratio has been used for distinguishing “abnormal pulmonary function” from normal, however for more precise and accurate estimates, the lower limit of normal (LLN) should be considered as a better definition (Cerveri et al., Citation2009; Miller, Quanjer, Swanney, Ruppel, & Enright, Citation2011; Pellegrino et al., Citation2005). This issue is currently being considered by the ATS/ERS task forces who are focusing on designing and updating predictive equations that can be used to obtain LLN to ensure accurate diagnosis is made in a clinical environment without any bias (Miller, Pedersen, Pellegrino, & Brusasco, Citation2009).
Exercise-induced bronchoconstriction (EIB) is characterised by a similar transient narrowing of airways which is specifically triggered by a bout of exercise. For individuals with EIB, a brief period of moderate-high-intensity exercise or hyperventilation triggers airflow obstruction, lasting 30–90 min in the absence of treatment (Anderson & Kippelen, Citation2010; Hallstrand, Citation2012). While up to 90% of people with clinically diagnosed asthma experience EIB at some point during their lives, approximately 10% of the healthy population without a history of asthma also show symptoms of EIB following exercise or hyperventilation (Krafczyk & Asplund, Citation2011; Weiss & Rundell, Citation2009). EIB has been identified by recording a post-exercise reduction in forced expiratory volume in 1 s (FEV1) of 10% of the pre-exercise value (Sterk et al., Citation1993). One of the proposed mechanisms for EIB is that it results from loss of water from the airway surface while inhaling large volumes of air in a short period of time. Asthmatics show an increased production of a number of cytokines and chemokines (Barnes, Citation2008; Robinson, Citation2010). There is also a release of Arachidonic Acid (AA)-derived eicosanoids including Prostaglandins and Leukotrienes which cause bronchoconstriction of the airways; these mediators affect microvascular and bronchial dilation, increase Airway Hyperresponsiveness, and have been implicated in the pathogenesis of asthma (Broughton, Johnson, Pace, Liebman, & Kleppinger, Citation1997; Decaterina & Basta, Citation2001; Kippelen et al., Citation2010; Sastre & del Pozo, Citation2012). Nitric Oxide (NO) is an endogenous regulatory molecule involved in the pathogenesis of asthma, and asthmatics usually exhibit 2–3 times higher levels FeNO compared to healthy adults (Saleh, Ernst, Lim, Barnes, & Giaid, Citation1998; Zitt, Citation2005). At present, FeNO is not used as a diagnostic test for asthma or EIB, however the value of performing FeNO measurements as a supplementary diagnostic test is well established (Dweik et al., Citation2011), and elevated FeNO asthmatics are indicative of eisonophilic inflammation; however, a direct pathogenic role of FeNO in asthma is yet to be fully elucidated (Lim et al., Citation2000; Sandrini, Taylor, Thomas, & Yates, Citation2010).
A range of short- and long-term pharmacological therapies for asthma and EIB are available (Barnes, Citation2010); however, long-term usage of these medications is associated with issues of tachyphylaxis (Barnes, Citation2010; GINA, Citation2011; Mickleborough & Lindley, Citation2013). Evidence from observational and intervention studies suggest a beneficial effect of fish oil (comprising of omega-3 polyunsaturated fatty acids (PUFAs) in inflammatory diseases, (specifically asthma) (Thien, De Luca, Woods, & Abramson, Citation2011). Omega-3 PUFA have therefore been proposed as a possible complimentary/alternative therapy for asthma (Kumar, Mastana, & Lindley, Citation2016). Dietary supplementation with omega-3 PUFA alters cellular fatty acid profiles in a dose-response manner, with an overall increase in Eicosapentaenoic Acid (EPA) and Docosahexaenoic acid (DHA) following supplementation (Damsgaard, Frøkiær, & Lauritzen, Citation2008; Kew et al., Citation2004; Rees et al., Citation2006; Yaqoob, Pala, Cortina-Borja, Newsholme, & Calder, Citation2000). There is a significant incorporation of EPA and DHA in inflammatory cell phospholipid, plasma, erythrocyte membrane content with 3, 4 and 5 weeks of supplementation (Mickleborough, Lindley, Ionescu, & Fly, Citation2006; Schubert et al., Citation2009). It is accepted that EPA and DHA compete with and replace omega-6 PUFAs (primarily AA) within the cell membrane (Calder, Citation2015; Mickleborough et al., Citation2006). The modification of phospholipid content can influence the membrane-linked enzyme systems and cell signalling pathways that affect the physiological responses of cells (Calder, Citation2015; Mickleborough & Rundell, Citation2005; Wada et al., Citation2007). However in asthmatics, there is a paucity of studies examining the mechanisms of EPA and DHA at cellular level and such studies are required to evaluate whether omega-3 can be used in asthma management.
Reduced inflammation attenuates the severity of asthma including symptoms (dyspnoea) and exerts a bronchodilatory effect (Mickleborough, Citation2008; Mickleborough & Lindley, Citation2013). Although there are a number of intervention studies investigating the effect of omega-3 PUFA on asthma participants, there is conflicting evidence in relation to PF outcomes—Peak expiratory flow (PEF) and FEV1 (Emelyanov et al., Citation2002; Hodge et al., Citation1998; Mickleborough, Murray, Ionescu, & Lindley, Citation2003; Mickleborough et al., Citation2006; Schubert et al., Citation2009; Surette, Stull, & Lindemann, Citation2008). EPA/DHA supplementation has been studied in athletic populations (Mickleborough et al., Citation2003, Citation2006); however, research is limited in physically active groups (exercising between 2–4 times per week). Hence, we conducted a pilot study to assess the effect of a 3-week EPA/DHA supplementation in EIB-prone physically active males and to evaluate the incorporation of EPA/DHA in circulating Peripheral Blood Mononuclear Cells (PBMC) total lipids. Additionally, we measured FeNO, dyspnoea and circulating levels IL-6, IL-10 and TNF-α to evaluate the changes in inflammatory cytokines with supplementation. The current study would therefore extend what is already known on physically active asthmatic males by focusing on a subgroup that doesn’t train at an athletic level but represents the general population with an active lifestyle.
2. Methods
2.1. Participants
Participants entered the study on their normal diet (baseline 1), participants were included if they were 18–30 years old, had physician-diagnosed mild-moderate asthma, had no respiratory infection within the past 8 weeks and had not taken steroids of any kind in the last 4 weeks, Hay fever or other allergy medication in the past 7 days, and dietary omega-3 supplementation in the last year. The participant group comprised of physically active males exercising 2–4 times per week. Twelve participants were recruited; however, only nine participants successfully completed the entire protocol (mean age (SEM) 21 ± 0.9 years, mean height (SEM) 1.8 ± 0.1 m). One participant did not complete the study due to injury; another participant was excluded because his asthma symptoms worsened (self-reported) for unexplained reasons during the study; a recommendation was made to this participant to discuss his situation with his clinician. Another participant withdrew from the study on the basis of cold and flu symptoms. Participants were recruited based on abnormal lung function (measured FEV1/FVC ratio of ≤80%) and also fell below the “Lower Limit of normal” (LLN) (82%) derived from a matched population of healthy individuals recruited in a previous study (mean age (SEM) 21.6 ± 1.6, mean height (SEM) 1.7 ± 0.02 m). All participants showed positive diagnosis for EIB (≥10% drop in post exercise FEV1) and had elevated FeNO (≥40 ppb) at baseline.
At recruitment, all participants reported accounts of shortness of breath, chest tightness, and intermittent wheezing post exercise on a regular basis, which was normally relieved by administration of a bronchodilator. There were four participants currently reliant on bronchodilators (β2 agonist i.e. Salbutamol or albuterol), and five participants were using a preventative medication and bronchodilators (Salbutamol or albuterol and short acting steroids i.e. beclometasone/fluticasone). Participants voluntarily refrained from all their medication 48 h prior to testing and were advised to discuss the situation with their medical practitioner prior to the participation in the study. Testing was rescheduled if participants used medication 48 h prior to testing and a log of medicine usage was maintained until the participant was tested. Participants were also asked to refrain from caffeine intake (for 8 h) and physical activity (for 24 h) prior to the exercise challenge test. The research design for this study was such that the blood samples were collected at the same time of the day at each collection point (±30 min) and the sample analysis was conducted within 2 h of collection. There were no dietary restrictions during the study other than personal omega-3 supplementation. The participants maintained a diet diary and recorded their daily food intake during supplementation to ensure that they maintained their regular diet (data not shown).
2.2. Study design
A double-blinded, placebo-controlled, randomised, crossover trial was carried out to assess the effects of a 3-week supplementation with omega-3 (EPA/DHA) on EIB participants. It is shown that there is a progressive decline in the levels of EPA and DHA after the completion of supplementation period with a steep decline within the first 2 weeks in various cell types including plasma phospholipids, platelets and leukocytes, neutrophils and RBC membranes (Cao, Schwichtenberg, Hanson, & Tsai, Citation2006; Mickleborough et al., Citation2006; Roulet, Frascarolo, Pilet, & Chapuis, Citation1997; Senkal et al., Citation2007). Therefore, a 3-week washout was considered appropriate for our study. A power analysis was completed to approximate the appropriate sample size based on previous studies showing the effect of omega-3 PUFAs on PF in athletic EIB subjects (Mickleborough et al., Citation2003, Citation2006). The design aimed to observe a minimum detectable difference or change of 300 millilitres in FEV1 after exercise with the supplementation. The significance level was set to α = 0.05 and the power at 0.80. Using these data, a sample size of eight participants was necessary to detect a difference of this magnitude (final sample size was nine subjects). Considering the sample size was small, the participants were randomly allocated using a minimisation scheme (Altman & Bland, Citation2005), whereby next allocation of supplementation capsules was dependent on the supplementation provided to the previous participant. This scheme of randomisation has been found to be appropriate to achieve balance between treatment groups. The study protocol was approved by the Loughborough University’s ethics committee (reference number R09-158) responsible for approval of human experimentation as per the International Declaration of Helsinki.
3. Pulmonary function tests
Spirometry was performed using a calibrated spirometer (Superspiro; Micro Medical; Rochester, Kent, UK) according to ATS/ERS recommendations (Miller, Hankinson, et al., Citation2005). This was done before the exercise test started. This involved three readings, and the best of the three readings was included in all calculations. All participants were given an opportunity to practice and familiarise with the spirometer before the actual tests were conducted. For the exercise challenge test, each participant ran on a motorised treadmill until they achieved 80–90% of their estimated maximum heart rate (220-Age), they then continued running at this steady state for 8 min.(Miller, Crapo, et al., Citation2005; Miller, Hankinson, et al., Citation2005). A warm-up was included at the start and the participants’ heart rate was constantly monitored throughout the test using Polar F4 Heart Rate Monitor (Polar Ltd, USA. The percentage drop in post-exercise FEV1 compared to pre-exercise values was calculated based on the following formula (Equation 1). PFTs were assessed pre-exercise and post exercise at 1, 5, 10, 15, 30 and 45 min or until FEV1 reached ±5% of baseline FEV1 (whichever was earlier). Changes in post-exercise FEV1 and pre-exercise PF measures (FEV1, FVC, FEV1/FVC ratio and PEF) were recorded. Borg analogue scale was used to record the perception of breathlessness (dyspnoea) before and after exercise at 1, 5, 10, 15, 30 and 45 min or until FEV1 reached ±5% of baseline FEV1 (Borg, Citation1982).
3.1. Fraction of exhaled breath nitric oxide (FeNO) measurement
FeNO measurements were conducted using a portable NIOX MINO analyser (Aerocrine Inc., Solna, Sweden) pre and post exercise at each time point. Three acceptable manoeuvres were performed to control intra subject variability based on ATS/ERS recommendations and values expressed in parts per billion (ppb) (Miller, Crapo, et al., Citation2005).
3.2. Biochemical tests
3.2.1. PBMC total lipid analysis
PBMC total lipid analysis was carried out before and after all supplementation phases using 9 ml of anti-coagulated venous blood. Blood was collected before exercise from each participant for this study. PBMC were extracted using a density centrifugation method and esterified to fatty acid methyl esters (FAMEs) and stored at −20°C prior to analysis by GC-FID using a method modified from Kumar, Lindley, and Mastana (Citation2014) and Masood, Stark, and Salem (Citation2005). The area under the curve was measured for the samples and the results were expressed as percentage of each fatty acid content relative to the total fatty acid content.
3.2.2. Circulating cytokine measurements
For sample preparation, whole blood was collected in a serum tube (with no anti-coagulant) and allowed to coagulate for up to an hour. The tubes were centrifuged at 1300× g for 10 min. Serum was separated and aliquots were made and stored at −20°C until further analysis. To minimise the effect of inter-plate variation, all the samples from each individual collected at different time points of the study were analysed on the same ELISA plate. Circulating levels for TNF-α and IL-10 were measured using an ELISA kit (Biolegend, UK) while IL-6 was measured using a high-sensitivity ELISA kit ((Tepnel/Diaclone), UK) as per manufacture’s specifications. The Biolegend kit for TNF-α and IL-10 were reported to provide a sensitivity of less than 2 and 0.04 pg/mL respectively, and guaranteed to have no cross-reactivity or interference. The sensitivity of the Human IL-6 High-Sensitivity ELISA (Diaclone) was 0.81 pg/mL, with guaranteed no cross-reactivity or interference in the assay. The samples were run in duplicate on two plates for reproducibility and the intra-assay variation (coefficient of variation, CV %) was calculated as <10%, which was acceptable as per manufacturer’s guidelines. All ELISA kits tested for cytokine analysis performed up to the recommended specifications provided by the manufacturers, determined by a linear standard curve with correlation coefficient (r) r ≥ 0.99. The intra-plate variation was acceptable for each cytokine kit analysed, determined by an acceptable correlation for standard curves generated on different plates (r ≥ 0.90). The samples were run in duplicate on each plate and any samples with a CV of >10% were excluded from analysis or repeat analysis was conducted to confirm the values.
3.2.3. Compliance monitoring
The participants were provided with a greater number of capsules than necessary to complete the supplementation phases and were asked to return the remaining capsules after the omega-3 supplementation and placebo phase. The remaining capsules were counted to estimate compliance. The participants were given clear instructions about the supplementation (omega-3 and placebo) and were provided with the necessary number of capsules in a plain container. Additionally, the participants were sent text reminders at the end of each week of supplementation and the remaining capsules in the returned containers were counted to estimate compliance.
4. Statistical analysis
Data was analysed using statistical software (SPSS, version 19; SPSS; Chicago, IL, USA). A two-way repeated measures analysis of variance (RM ANOVA) was used to measure changes in physiological and biochemical parameters within the same individuals as a result of omega-3/placebo supplementation over a period of time (within-subject effects). Mauchly’s test was conducted to determine whether sphericity was violated. In the cases where sphericity was violated, the repeated measures analysis of variance was corrected using the Greenhouse–Geiser correction factor. Order effect was assessed using the order as a variable in the analysis. For serum, cytokine analysis using ELISA-based methods, when multiple plates were run, the standard curves were compared for correlation to assess intra assay variations.
5. Results
Out of the twelve participants recruited, nine participants successfully completed the entire study protocol; their basic characteristics are listed in . The participants were given clear instructions about the supplementation (omeag-3 and placebo) and were provided with necessary number of capsules in a pot. Additionally, the participants were sent text reminders at the end of each week of supplementation and the remaining capsules in the returned pots were counted to estimate compliance. All participants had less than five capsules returned, which was considered an acceptable level of compliance. In addition, there was no effect of order in which supplementation was provided (p > 0.05). All results reported in this section are expressed as mean ± SEM unless stated otherwise.
Table 1. Participant characteristics
5.1. Pulmonary function
5.1.1. No carry over effects
There were no differences in either the pre-exercise (rest) or post-exercise FEV1 between the two baselines based on RM ANOVA, demonstrating no carry over effects. Subsequently, all comparisons after supplementation were made with baseline 1. There was also no difference between the two baselines, placebo and the end-of-study washout (p > 0.05).
5.1.2. PFT results
All participants had a >10% drop for post-exercise FEV1 at two time points (between 5 and 15 min) at baseline and placebo representing abnormal PF, and the mean of the two time points where the percentage dropped the lowest (post-exercise FEV1) were used for further analysis of all time points. All participants met the diagnostic criteria for EIB at baseline [mean drop (SEM) = 14.8 (0.50)%] and post placebo phase [mean drop (SEM) = 12.4 (0.44)%; ]. A significant improvement was observed in post-exercise FEV1 at 5, 10 and 15 min post exercise after the EPA/DHA supplementation phase compared to baseline for all participants (p < 0.05). The mean percentage drop in FEV1 (SEM) post omega-3 intervention was 6.6 (0.45)%, which is indicative of attenuated EIB response. No significant changes were observed in pre-exercise resting mean FEV1, FVC, PEF and FEV1/FVC ratio across all time points (p > 0.05), and no carry over effects were observed (p > 0.05).
Figure 1. The percentage change in FEV1 before and after exercise at baseline, placebo and omega-3 (EPA/DHA) phase for all participants. Reduction in FEV1 > 10% shows a positive diagnosis for EIB.
*Significant improvement from baseline (p < 0.05).
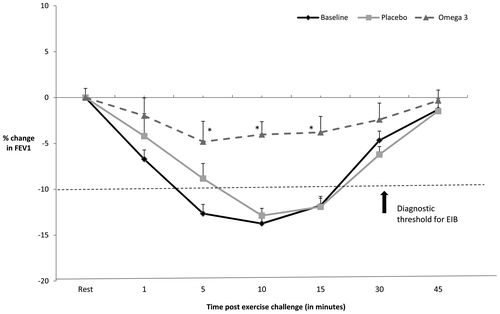
The total bronchodilator usage during omega-3 supplementation phase (4 times, 8 inhalations) was lower than the total usage during placebo phase (7 times, 14 inhalations). Significant reduction (50%) in bronchodilator use was observed during the last week of omega-3 supplementation (1 times, 2 inhalations) compared to placebo phase (2 times, 4 inhalations) (p < 0.05). No changes were observed in the bronchodilator usage between placebo, baseline 2 and the washout (p > 0.05).
5.2. Dyspnoea and FeNO
All participants reported very slight breathlessness before the exercise test at each time point of the study. No improvement was observed in dyspnoea score with omega-3 supplementation compared with other time points (mean score = 1; p > 0.05). At baseline, participants showed elevated levels of resting FeNO (52 ± 3.7 ppb) compared with expected ranges in non-asthmatics (~35 ppb) (Zitt, Citation2005). However, there were no significant changes in pre-exercise or post-exercise FeNO levels measured at each time point compared with baseline (pre exercise values—Omega-3: 57 ± 9.3; Placebo: 47 ± 8; Wash 1: 51 ± 15; Wash 2: 58 ± 6.7; p > 0.05).
5.3. PBMC total lipids and serum cytokines
The mean CV% for all PBMC samples analysed was found to be <10% on the same day. Although there was an increase in overall EPA and DHA levels after supplementation with EPA/DHA, the mean EPA and DHA levels didn’t differ statistically between the time points (p > 0.05, ). However, there was a reduction in omega-6 fatty acid (Arachidonic Acid, AA) levels post omega-3 supplementation, however this change was also not statistically significant (mean(SEM) = 4.74(0.89)%; p > 0.05). Other fatty acids were also analysed, however no statistically significant difference was observed; therefore, these data are not reported. A statistically significant reduction was observed in serum IL-6 levels after omega-3 supplementation, there was an overall reduction of 37% compared to baseline (p < 0.05). The IL-10 and TNF-α levels remain unchanged (p > 0.05) across all time points as shown in .
6. Discussion
Our study for the first time shows significant improvement in post-exercise FEV1 in physically active males with asthma/EIB and these findings are consistent with those observed in athletes in previous studies (Mickleborough et al., Citation2003, Citation2006). The study has demonstrated that a supplementation with omega-3 (3.2 grams of EPA and 2.2 grams of DHA) for three weeks reduces the severity of EIB in participants with mild-moderate asthma. The supplementation improved PF post exercise to below the diagnostic threshold value for EIB, i.e. 10% fall in post-exercise FEV1. There was approximately 71% improvement in FEV1 at 15 min after exercise. The mean level for the two time points where the percentage dropped the lowest in FEV1 after exercise at post omega-3 supplementation was 6.6%, compared to a drop of 14.8% at baseline when participants were on their regular diet.
There was no change in the PF outcomes for FEV1, FVC and PEF before exercise (at rest) after three-week supplementation with omega-3. The adult omega-3 intervention trials in asthma have provided inconclusive information with regard to FEV1 or PEF. In a number of trials with varying dose and duration of omega-3 supplementation, no significant changes have been observed for FEV1 or PEF (Hodge et al., Citation1998; Kirsch et al., Citation1988; Mickleborough et al., Citation2003, Citation2006). There are some studies that have shown positive outcomes; Surette et al. (Citation2008) reported a significant improvement in quality of life and asthma management scores (including symptoms) assessed by questionnaires after 3 weeks of supplementation (0.5 g EPA + 0.75 gram DHA per day). Although some literature and our knowledge of the physiological action of omega-3 fatty acids suggest that we should potentially see effects of supplementation on lung function, there is no consistent effect of omega-3 supplementation on FEV1 or PEF reported. This could be attributed to the heterogeneity between the studies. These studies have used a range of doses, as low as 50 mg to >3 g of EPA/DHA per day and have studied different subgroup of asthmatics such as mild-moderate, EIB and atopic populations from 3 weeks to months. The current study shows improvement in post-exercise FEV1 after 3-week supplementation with no significant changes in resting PF values for FEV1 and PEF, and these results confirm the previous findings by Mickleborough et al. (Citation2003, Citation2006). The evidence suggests that a 3-week supplementation with 3.2 g EPA and 2.2 g DHA is sufficient to improve exercise-induced FEV1 supported by reduction in inflammatory cytokine profiles and marked by reduced bronchodilator usage. However, it is likely that a 3-week supplementation is not sufficient to alter baseline PF values especially if mild-moderate asthmatic populations are studied; consequently, benefits on overall PF values may emerge with longer duration of supplementation. In populations with severe and uncontrolled asthma, the benefits of EPA/DHA supplementation are required to be evaluated along with appropriate duration required for supplementation.
In previous studies, omega-3 supplementation has been accompanied by some improvement in symptoms assessed by reduction in daytime wheezing and quality of life questionnaires. Two studies have shown an improvement in mean daytime wheeze, morning PEF and improvement in quality of life (Emelyanov et al., Citation2002; Surette et al., Citation2008). The study by Emelyanov et al. (Citation2002) comprised of a group of mild-moderate atopic asthmatics (18–56 years, n = 46) supplemented with green-lipped mussel extract with 50 mg of EPA + DHA/day for 8 weeks. Despite the relatively lower dose of EPA and DHA used by Emelyanov et al. (Citation2002), the longer duration of the study could be a reason why beneficial effects were seen in mean daytime wheeze and morning PEF. Surette et al. (Citation2008) had a 3-week supplementation period with 0.75 gram Gamma linolenic acid (GLA) + 0.5 g EPA or 1.3 g GLA + 0.75 g EPA in mild-moderate asthmatics (n = 65) and showed improvement in self-reported status and medication use, and based on quality of life questionnaires there was an improvement in symptoms. The major limitation for this study was that no PFT measurements were taken and the results are based on self-reported outcomes. Since symptoms (or perception) have been suggested to be not a true indicator of the status of PF, the results from the Surette et al. (Citation2008) study can be considered to have serious limitations.
Furthermore, the lack of improvement in PFT outcomes in the omega-3 supplementation studies conducted in the last two decades could be due to the nature of asthmatics used. The current study also showed no improvement in dyspnoea score with supplementation, therefore is in agreement with previous studies. These results only further justify the argument that there is very low concordance between symptoms and spirometry (Cowie, Underwood, & Field, Citation2007; Schifano, Hollenbach, & Cloutier, Citation2014); neither symptoms nor asthma history have the potential to predict respiratory abnormalities on their own and need to be used in conjunction with spirometry and other markers such as bronchodilator usage. In the present study, by the third week of omega-3 supplementation there was approximately 50% reduction in bronchodilator usage compared to placebo phase that support the previous findings that omega-3 supplementation exerts bronchodilatory effects within 2–3 weeks of supplementation. Improvements in symptoms assessed by reduction in daytime wheezing and quality of life questionnaires are reported (Emelyanov et al., Citation2002; Surette et al., Citation2008). The average dyspnoea score reported in our study at baseline was already very low. All participants displayed a score between 0 and 3, which corresponds to no symptoms to mild symptoms of perceived breathlessness (the mean was 1 on a 0–10 scale). This fits well with mild-moderate asthma status of the individuals. The likelihood of improvement in the mean symptom score from this already very low value to reporting no symptoms on average was unlikely. All participants had mild-moderate asthma and had been living with and managing the condition for several years. Because of this, the perception of symptoms was also possibly under reported by the participants. Though self-reported symptoms are widely used in asthma diagnosis it is suggested that symptom score cannot be independently used to assess the severity of asthma, thus these need to be used in conjunction with standard PFTs (Parshall et al., Citation2012). Despite no changes in symptoms, this reduced bronchodilator usage with omega-3 supplementation suggests a possibility of reducing the reliance on pharmacological medications.
It is likely that the individuals in these published studies were mild-moderate asthmatics or not asthmatic at all. If incorrect characterisation was the case, then it is not likely to see a major change in the PFT values. To see substantial changes in PFT outcomes, a larger sample size with participants with severe airflow obstruction are required to assess the effect of EPA/DHA on PFT outcomes. Our study involved participants previously characterised as mild-moderate asthmatics using an 80% FEV1/FVC ratio, who were confirmed as mild-moderate asthmatics using the LLN criteria, in addition, the inclusion criteria included EIB (with exercise testing) and high FeNO levels (>40 ppb). Thus, the asthmatics recruited in the study were representative of a mild-moderate asthmatic young population (18–30 years) with EIB being the major characteristic. Moreover, our asthma characterisation methods support the validity of using LLN alongside fixed cut-off by providing a reliable characterisation of mild-moderate asthmatics. Therefore, improvement in post-exercise FEV1 is indicative of the beneficial effect of omega-3 supplementation.
FeNO measurements are now recognised as a supplementary asthma characterisation measurement in clinical setting (Dweik et al., Citation2011; Szefler et al., Citation2012). Our study showed no changes in FeNO levels across all time points which match results by Moreira et al. (Citation2007). Attenuated FeNO levels with low-dose allergen exposure after 3-week omega-3 supplementation compared with placebo have been reported (Schubert et al., Citation2009). These positive results with a relatively lower dose of omega-3 supplementation could be due to the mild inflammation in the asthma study group (Schubert et al., Citation2009). Conversely, our participants showed substantially elevated levels of FeNO and it is likely that 3-week supplementation with the chosen dose is not sufficient for reduction in FeNO levels. It is hypothesised that higher dietary intake of omega-3 (0.94 g/day of EPA + DHA) and ALA (1.96 g/day) can reduce the odds of non-controlled asthma (Barros et al., Citation2008). However, the prevalence of non-controlled asthma in this study was high, subsequently it is likely that risk was overestimated (Barros et al., Citation2008). In clinical practice, long-term medication use (corticosteroids) are shown to stabilise FeNO (Dweik et al., Citation2011; Zitt, Citation2005). In our study, since some participants were on short-acting steroid medications; they voluntarily refrained from medication usage 48 h prior to the testing. Hence any improvements in FeNO due to omega-3 supplementation would have been complimentary to the effect of pharmacological treatments. Subsequently, it is possible that the effect of EPA/DHA supplementation if measured over a longer period may improve FeNO levels.
A possible limitation of our study was that the FeNO measurements were conducted using a handheld portable device that was available within the budget of this research. The major advantage of these devices is that they can be used to monitor airway inflammation in clinics, in the field and in the patient’s home. There are some concerns about the reliability of these devices due to their limited ability to control flow rates (Kim, Kercsmar, & Davis, Citation2015; Schiller, Hammer, Barben, & Trachsel, Citation2009), and it is suggested that a stationary chemiluminescence device such as “Sievers nitric oxide analyser” may be a more reliable method for assessment. While there are a number of studies showing a good association between FeNO values measured with the two devices, a few studies reported that there were differences in the FeNO values obtained with the handheld NIOX MINO compared to those measured with stationary devices (Menzies, Nair, & Lipworth, Citation2007; Schiller et al., Citation2009). Kim et al. (Citation2012) demonstrated that there is a good correlation (r = 0.876, p < 0.001) but only moderate agreement between the FeNO values measured by the handheld and stationary devices. The NIOX MINO values are significantly lower than stationary devices, thus it is important that results must be carefully interpreted (Kim et al., Citation2012), however since the results are reliable and reproducible the changes in FeNO over time can be monitored using the same device. The mean value of the asthmatics in this study was >40 ppb and if these measurements were conducted using a stationary device, as Kim et al. (Citation2012) suggests, it would have been relatively higher. This suggests high levels of inflammation in the study population compared to healthy individuals (25 ppb). Consequently, the duration and the dose of supplementation given here may not have been sufficient to reduce FeNO values. Dose response and time course studies are required to evaluate the dose and duration required to reduce FeNO levels significantly in groups with high levels of inflammation.
Dietary supplementation with fish oil is shown to alter cellular fatty acid profiles in a dose-response manner; EPA and DHA have the ability to compete and replace AA and there is an increase in overall EPA and DHA in different cells and tissues (Kew et al., Citation2004; Rees et al., Citation2006; Yaqoob et al., Citation2000). Incorporation of fatty acids in PBMC membrane reaches near maximum in 4 weeks (Calder, Citation2015; Rees et al., Citation2006), hence it was expected that with 3 weeks there would be a substantial incorporation of EPA and DHA into the PBMC. The lack of significant change in EPA, DHA and AA could be attributed to measurement error, shorter duration of supplementation (than has been used in other studies with positive outcome) or small sample size and low power. It is demonstrated that incorporation of fatty acids in PBMC membrane reaches near maximum in 4 weeks (Rees et al., Citation2006; Thies et al., Citation2001; Yaqoob et al., Citation2000), and so it was expected that after 3 weeks there would be a substantial incorporation of EPA and DHA into the PBMC. The results from this study do show a positive trend in the incorporation of EPA alongside a reduction in AA levels, demonstrating a positive direction in the study and the likelihood that with continued supplementation the findings would have reached significance. The absence of significant changes in outcome could be attributed to possible limitations of the GC-FID method, particularly low recovery of AA and DHA due to possible loses in esterification and/or due to high temperature of GC parameters. In future studies, to address these issues longer duration of supplementation can be included, also an improvement in the GC-FID method can improve the findings.
EPA and DHA supplementation in healthy volunteers have shown decreased production of TNF-α, of IL-1β and IL-6 by endotoxin-stimulated monocytes or mononuclear cells (Caughey, Mantzioris, Gibson, Cleland, & James, Citation1996; Trebble et al., Citation2003). With omega-3 supplementation, we report a significant reduction in the levels of IL-6, which is in line with recent findings (Gray, Gabriel, Thies, & Gray, Citation2012). Mickleborough et al. (Citation2003) have shown changes in PF with only limited changes in inflammatory mediators (no changes in the circulating levels of IL-6 with 3-week supplementation at a dose and duration similar to the present study with a significant reduction in TNF-α). In our study, no changes were observed in IL-10 levels. IL-10 is critical in limiting immune response to pathogens and is involved in regulation of inflammatory cytokines and inflammatory enzymes (Baine et al., Citation2011; Holgate & Douglass, Citation2010; Larché, Citation2007). In animal models of inflammatory bowel disease, supplementation with omega-3 has shown to increase levels of IL-10 (Barros et al., Citation2011; Folador, de Lima-Salgado, Hirabara, Aikawa, et al., Citation2009). Furthermore, relative effect of EPA and DHA is considered important in determining effects associated with fish oil supplementation. In a cell-culture study, 120 mM pure EPA and EPA-rich media are reported to significantly suppress TNF-α and IL-1β mRNA expression and the production of LTB4, PGD2 and TNF-α and IL-1β in stimulated alveolar macrophages from asthmatics (Mickleborough, Tecklenburg, Montgomery, & Lindley, Citation2009). A review of a number of studies looking at inflammation and exercise propose that circulating IL-6 levels are affected by exercise and this effect is hypothesised to be facilitated by a reduction in visceral fat mass and the anti-inflammatory environment created by exercise regime (Gleeson et al., Citation2011). Therefore, changes in inflammatory mediators could be influenced by diet and exercise concurrently or independently. Multiplex approaches for gene expression are providing insight into the regulation of inflammatory genes. With 26 weeks of omega-3 supplementation approximately 1,000 genes are known to be regulated, with pro-inflammatory TNF-α and IL-6 being down regulated (Bouwens et al., Citation2009). Therefore, it is likely that the effect of omega-3 PUFA on pro-inflammatory cytokines is independent of eicosanoid activity and is associated with regulation of signalling pathways and transcription factors (Afman & Müller, Citation2012; Tai et al., Citation2005).
7. Summary and conclusions
Since asthma is a growing health issue, public health interventions are becoming increasingly important to reduce its severity and prevalence. Diet and nutrition are potential factors that could affect the pathophysiology of asthma, decrease reliance on pharmaceutical drugs, and promote health and independence in later life. This study has confirmed the beneficial effects of EPA/DHA supplementation in decreasing EIB severity thus, there is a potential for using omega-3 as a complimentary therapy for improving PF. The supplementation trials in asthma have been limited by sample size; however, there is a positive relationship of omega-3 PUFA supplementation on various inflammatory outcomes in asthma. Based on our results which confirm the previous findings of various studies in asthma, there is likelihood that if asthmatics with EIB compliment their daily diets with omega-3 either in the form of oily fish or supplements, it may ameliorate their EIB symptoms. A number of asthmatics do not engage in physical activity as it triggers their asthma. For this population, a dietary omega-3 intervention may potentially change their lifestyle and activity levels with wide-ranging health benefits. A large-scale RCT in EIB patients with a daily omega-3 intervention may be instrumental to understand the response of dietary omega-3 at a population level, giving the ability to determine effects in different age groups, different body mass classes, with gender and ethnicity.
This pilot study shows beneficial effects of omega-3 supplementation in asthma and EIB in recreationally active males (training <4times/week) with clinically diagnosed asthma. Although omega-3 supplementation did not alter FeNO levels and cytokine profiles for TNF-α and IL-10, there was a reduction in IL-6 levels suggesting a potential role of EPA/DHA in inflammatory mediator regulation. There was no conclusive evidence about changes in PBMC lipid composition for EPA/DHA, with a trend showing an increase in EPA and a reduction in AA following supplementation. The improvement in PF demonstrates a benefit to mild-moderate asthmatics of EPA/DHA supplementation in the management and treatment for EIB, which would significantly improve the quality of life and prove beneficial in physical activity, particularly to help achieve the recommended levels proposed by the Department of Health for maintaining a healthy lifestyle. However, the lack of consistent inflammatory mediator data means that possible mechanisms are still not elucidated. A larger population-based study is warranted to confirm the findings and evaluate the effect of omega-3 on associated markers of inflammation.
Acknowledgements
The authors are grateful to all participants and University support staff who contributed to the study. The authors also thank Biocare, UK and Bionovate, UK for providing free supplements to support the study.
Additional information
Funding
Notes on contributors
Aishwarya Kumar
Aishwarya Kumar is a senior research scientist at Clinical Trial Service Unit, Oxford University. This work is part of her doctoral research. She has been a part of research group led by Martin R. Lindley, a senior lecturer in Human-Biology and Exercise-Physiology at Loughborough University, UK. He is the academic lead for Translational Chemical Biology, a cross-discipline group focused upon interrogating mechanism of action of naturally occurring anti-inflammatory agents (e.g. fish oil). Aishwarya’s been a part of a research group led by Sarabjit S Mastana, a senior lecturer in Human Biology at Loughborough University. His work extends to Human Genomic Studies particularly focusing on genetic epidemiological analyses of Rheumatoid Arthritis, Diabetes, Heart Disease, Hypertension and Osteoporosis among native and migrant populations. Aishwarya’s current research at CTSU involves development of new high-throughput automated analytical techniques for use in large-scale blood-based epidemiological studies.
References
- Afman, L. A., & Müller, M. (2012). Human nutrigenomics of gene regulation by dietary fatty acids. Progress in Lipid Research, 51, 63–70.10.1016/j.plipres.2011.11.005
- Altman, D. G., & Bland, J. M. (2005). Treatment allocation by minimisation. British Medical Journal, 330, 843.
- Anderson, S. D., & Holzer, K. (2000). Exercise-induced asthma: Is it the right diagnosis in elite athletes? Journal of Allergy and Clinical Immunology, 106, 419–428.
- Anderson, S. D., & Kippelen, P. (2010). Stimulus and mechanisms of exercise-induced bronchoconstriction. Breathe, 7, 25–33.10.1183/18106838.0701.025
- Baine, M. J., Chakraborty, S., Smith, L. M., Mallya, K., Sasson, A. R., Brand, R. E., & Batra, S. K. (2011). Transcriptional profiling of peripheral blood mononuclear cells in pancreatic cancer patients identifies novel genes with potential diagnostic utility. PLoS ONE, 6(2), e17014. doi:10.1371/journal.pone.0017014
- Barnes, P. J. (2008). The cytokine network in asthma and chronic obstructive pulmonary disease. Journal of Clinical Investigation, 118, 3546–3556.10.1172/JCI36130
- Barnes, P. J. (2010). New therapies for asthma: Is there any progress? Trends in Pharmacological Sciences, 31, 335–343.
- Barros, R., Moreira, A., Fonseca, J., Delgado, L., Graça Castel-Branco, M., Haahtela, T., … Moreira, P. (2011). Dietary intake of α-linolenic acid and low ratio of n-6:n-3 PUFA are associated with decreased exhaled NO and improved asthma control. British Journal of Nutrition, 106, 441–450.10.1017/S0007114511000328
- Barros, R., Moreira, A., Fonseca, J., Ferraz de Oliveira, J., Delgado, L., Castel-Branco, M. G., & Lopes, C. (2008). Adherence to the Mediterranean diet and fresh fruit intake are associated with improved asthma control. Allergy, 63, 917–923.10.1111/all.2008.63.issue-7
- Borg, G. A. (1982). Psychophysical bases of perceived exertion. Medicine and Science in Sports and Exercise, 14, 377–381.
- Bousquet, J. P., Godard, P., & Daures, J. P. (2005). The public health implications of asthma. Bulletin of the World Health Organisation, 83, 548–554.
- Bouwens, M., van de Rest, O., Dellschaft, N., Bromhaar, M. G., de Groot, L. C., Geleijnse, J. M., … Afman, L. A.. (2009). Fish-oil supplementation induces antiinflammatory gene expression profiles in human blood mononuclear cells. American Journal of Clinical Nutrition, 90, 415–424.10.3945/ajcn.2009.27680
- Broughton, K. S., Johnson, C. S., Pace, B. K., Liebman, M., & Kleppinger, K. M. (1997). Reduced asthma symptoms with n-3 fatty acid ingestion are related to 5-series leukotriene production. American Journal of Clinical Nutrition, 65, 1011–1017.
- Calder, P. C. (2015). Marine omega-3 fatty acids and inflammatory processes: Effects, mechanisms and clinical relevance. Biochimica et Biophysica Acta (BBA) - Molecular and Cell Biology of Lipids, 1851, 469–484.10.1016/j.bbalip.2014.08.010
- Cao, J., Schwichtenberg, K. A., Hanson, N. Q., & Tsai, M. Y. (2006). Incorporation and clearance of omega-3 fatty acids in erythrocyte membranes and plasma phospholipids. Clinical Chemistry, 52, 2265–2272.10.1373/clinchem.2006.072322
- Caughey, G. E., Mantzioris, E., Gibson, R. A., Cleland, L. G., & James, M. J. (1996). The effect on human tumor necrosis factor a and interleukin 1b production of diets enriched in n-3 fatty acids from vegetable oil or fish oil. American Journal of Clinical Nutrition, 63, 116–122.
- Cerveri, I., Corsico, A. G., Accordini, S., Cervio, G., Ansaldo, E., Grosso, A., … de Marco, R. (2009). What defines airflow obstruction in asthma? European Respiratory Journal, 34, 568–573.10.1183/09031936.00172908
- Cowie, R. L., Underwood, M. F., & Field, S. K. (2007). Asthma symptoms do not predict spirometry. Canadian Respiratory Journal, 14, 339–342.10.1155/2007/816132
- Damsgaard, C. T., Frøkiær, H., & Lauritzen, L. (2008). The effects of fish oil and high or low linoleic acid intake on fatty acid composition of human peripheral blood mononuclear cells. British Journal of Nutrition, 99, 147–154.
- Decaterina, R., & Basta, G. (2001). n-3 Fatty acids and the inflammatory response? Biological background. European Heart Journal Supplements, 3, D42–D49.10.1016/S1520-765X(01)90118-X
- Dweik, R. A., Boggs, P. B., Erzurum, S. C., Irvin, C. G., Leigh, M. W., Lundberg, J. O., et al., on behalf of the American Thoracic Society Committee on Interpretation of Exhaled Nitric Oxide Levels (FENO) for Clinical Applications. (2011). An official ATS clinical practice guideline: Interpretation of exhaled nitric oxide levels (FENO) for clinical applications. American Journal of Respiratory and Critical Care Medicine, 184, 602–615.
- Emelyanov, A., Fedoseev, G., Krasnoschekova, O., Abulimity, A., Trendeleva, T., & Barnes, P. J. (2002). Treatment of asthma with lipid extract of New Zealand green-lipped mussel: A randomised clinical trial. European Respiratory Journal, 20, 596–600.10.1183/09031936.02.02632001
- Folador, A., de Lima-Salgado, T. M., Hirabara, S. M., Aikawa, J., Yamazaki, R. K., Martins, E. F., … Curi, R. (2009). Effect of fish oil supplementation for two generations on changes of lymphocyte function induced by Walker 256 cancer cachexia in rats. Nutrition and Cancer, 61, 670–679.10.1080/01635580902825548
- GINA. Global Initiative for Asthma GINA. (2011). From the global strategy for asthma management and prevention. Retrieved August, 2012, from http://www.ginasthma.org/
- Gleeson, M., Bishop, N. C., Stensel, D. J., Lindley, M. R., Mastana, S. S., & Nimmo, M. A. (2011). The anti-inflammatory effects of exercise: Mechanisms and implications for the prevention and treatment of disease. Nature Reviews Immunology, 11, 607–615. doi:10.1038/nri3041
- Gray, P., Gabriel, B., Thies, F., & Gray, S. R. (2012). Fish oil supplementation augments post-exercise immune function in young males. Brain, Behavior, and Immunity, 26, 1265–1272.10.1016/j.bbi.2012.08.002
- Hallstrand, T. S. (2012). New insights into pathogenesis of exercise-induced bronchoconstriction. Current Opinion in Allergy and Clinical Immunology, 12, 42–48.10.1097/ACI.0b013e32834ecc67
- Hankinson, J. L., Odencrantz, J. R., & Fedan, K. B. (1999). Spirometric reference values from a sample of the general U.S. population. American Journal of Respiratory and Critical Care Medicine, 159, 179–187.10.1164/ajrccm.159.1.9712108
- Hodge, L., Salome, C., Hughes, J., Liu-Brennan, D., Rimmer, J., Allman, M., … Woolcock, A. J. (1998). Effect of dietary intake of omega-3 and omega-6 fatty acids on severity of asthma in children. European Respiratory Journal, 11, 361–365.10.1183/09031936.98.11020361
- Holgate, S. T., & Douglass, J. (2010). Fast facts: Asthma (3rd ed.). Oxford: Health Press.
- Kew, S., Mesa, M. D., Tricon, S., Buckley, R., Minihane, A. M., & Yaqoob, B. (2004). Effects of oils rich in eicosapentaenoic and docosahexaenoic acids on immune cell composition and function in healthy humans. American Journal of Clinical Nutrition, 79, 674–681.
- Kim, S. H., Moon, J. Y., Kwak, H. J., Kim, S. I., Park, D. W., Kim, J. W., … Yoon, H. J. (2012). Comparison of two exhaled nitric oxide analyzers: The NIOX MINO hand-held electrochemical analyzer and the NOA280i stationary chemiluminescence analyzer. Respirology, 17, 830–834.10.1111/j.1440-1843.2012.02163.x
- Kim, Y. J., Kercsmar, M. C., & Davis, S. D. (2015). Diagnostic tests in pediatric pulmonology applications and interpretation (respiratory medicine). In S. D. Davies, E. Eber, & A. C. Koumbourlis (Eds.), Fractional exhaled nitric oxide: Indications and interpretation (Chap. 14, pp. 285–308). Humana Press. ISBN 978-1-4939-1801-0. doi:10.1007/978-1-4939-1801-0_14
- Kippelen, P., Larsson, J., Anderson, S. D., Brannan, J. D., Delin, I., Dahlen, B., & Dahlen, S. E. (2010). Acute effects of beclomethasone on hyperpnea-induced bronchoconstriction. Medicine & Science in Sports & Exercise, 42, 273–280.10.1249/MSS.0b013e3181b541b1
- Kirsch, C. M., Payan, D. G., Wong, Y. S., Dohlman, J. G., Blake, V. A., Petri, M. A., … Gold, W. M. (1988). Effect of eicosapentaenoic acid in asthma. Clinical & Experimental Allergy, 18, 177–187.
- Krafczyk, M. A., & Asplund, C. A. (2011). Exercise-induced bronchoconstriction: Diagnosis and management. American Family Physician, 84, 427–434.
- Kumar, A., Lindley, M. R., & Mastana, S. (2014). A time efficient adaptation of GC-FID method for the analysis of PBMC lipid composition. Journal of Biochemical Techniques, 5, 760–764. ISSN: 0974-2328.
- Kumar, A., Mastana, S. S., & Lindley, M. R. (2016). n-3 Fatty acids and asthma. Nutrition Research Reviews, 1–16.10.1017/S0954422415000116
- Larché, M. (2007). Regulatory T cells in allergy and asthma. Chest, 132, 1007–1014.10.1378/chest.06-2434
- Lim, S., Jatakanon, A., Meah, S., Oates, T., Chung, K. F., & Barnes, P. J. (2000). Relationship between exhaled nitric oxide and mucosal eosinophilic inflammation in mild to moderately severe asthma. Thorax, 55, 184–188.10.1136/thorax.55.3.184
- Masood, A., Stark, K. D., & Salem, Jr., N. (2005). A simplified and efficient method for the analysis of fatty acid methyl esters suitable for large clinical studies. The Journal of Lipid Research, 46, 2299–2305.10.1194/jlr.D500022-JLR200
- McFadden, Jr., E. R., & Gilbert, I. A. (1994). Exercise-induced asthma. New England Journal of Medicine, 330, 1362–1367.10.1056/NEJM199405123301907
- Menzies, D., Nair, A., & Lipworth, B. J. (2007). Portable exhaled nitric oxide measurement. Chest, 131, 410–414.10.1378/chest.06-1335
- Mickleborough, T. D. (2008). A nutritional approach to managing exercise-induced asthma. Exercise and Sport Sciences Reviews, 36, 135–144.10.1097/JES.0b013e31817be827
- Mickleborough, T. D., & Lindley, M. R. (2013). Omega-3 fatty acids: A potential future treatment for asthma? Expert Review of Respiratory Medicine, 7, 577–580.10.1586/17476348.2013.841080
- Mickleborough, T. D., Lindley, M. R., Ionescu, A. A., & Fly, A. D. (2006). Protective effect of fish oil supplementation on exercise-induced bronchoconstriction in asthma. Chest, 129, 39–49.10.1378/chest.129.1.39
- Mickleborough, T. D., Murray, R. L., Ionescu, A. A., & Lindley, M. R. (2003). Fish oil supplementation reduces severity of exercise-induced bronchoconstriction in elite athletes. American Journal of Respiratory and Critical Care Medicine, 168, 1181–1189.10.1164/rccm.200303-373OC
- Mickleborough, T. D., & Rundell, K. W. (2005). Dietary polyunsaturated fatty acids in asthma- and exercise-induced bronchoconstriction. European Journal of Clinical Nutrition, 59, 1335–1346.
- Mickleborough, T. D., Tecklenburg, S. L., Montgomery, G. S., & Lindley, M. R. (2009). Eicosapentaenoic acid is more effective than docosahexaenoic acid in inhibiting proinflammatory mediator production and transcription from LPS-induced human asthmatic alveolar macrophage cells. Clinical Nutrition, 28, 71–77.10.1016/j.clnu.2008.10.012
- Miller, M. R., Crapo, R., Hankinson, J., Brusasco, V., Burgos, F., Casaburi, R., … ATS/ERS Task Force. (2005). General considerations for lung function testing. European Respiratory Journal, 26, 153–161.10.1183/09031936.05.00034505
- Miller, M. R., Hankinson, J., Brusasco, V., Burgos, F., Casaburi, R., Coates, A., … Wanger, J. (2005). Standardisation of spirometry. European Respiratory Journal, 26, 319–338.10.1183/09031936.05.00034805
- Miller, M. R., Pedersen, O. F., Pellegrino, R., & Brusasco, V. (2009). Debating the definition of airflow obstruction: Time to move on? European Respiratory Journal, 34, 527–528.10.1183/09031936.00103309
- Miller, M. R., Quanjer, P. H., Swanney, M. P., Ruppel, G., & Enright, P. L. (2011). Interpreting lung function data using 80% predicted and fixed thresholds misclassifies more than 20% of patients. Chest, 139, 52–59.
- Moreira, A., Moreira, P., Delgado, L., Fonseca, J., Teixeira, V., Padrão, P., & Branco, C. (2007). Pilot study of the effects of n-3 polyunsaturated fatty acids on exhaled nitric oxide in patients with stable asthma. Journal of Investigational Allergology and Clinical Immunology, 7, 309–313.
- Ober, C., & Yao, T. C. (2011). The genetics of asthma and allergic disease: A 21st century perspective. Immunological Reviews, 242, 10–30.10.1111/j.1600-065X.2011.01029.x
- Parshall, M. B., Schwartzstein, R. M., Adams, L., Banzett, R. B., Manning, H. L., Bourbeau, J., … on behalf of the ATS Committee on Dyspnea An Official American Thoracic Society Statement. (2012). An Official American Thoracic Society Statement: Update on the mechanisms, assessment, and management of dyspnea. American Journal of Respiratory and Critical Care Medicine, 185, 435–452.10.1164/rccm.201111-2042ST
- Pellegrino, R., Viegi, G., Brusasco, V., Crapo, R. O., Burgos, F., Casaburi, R., … Wanger, J. (2005) Interpretative strategies for lung function tests. European Respiratory Journal, 26, 948–968.10.1183/09031936.05.00035205
- Rees, D., Miles, E. A., Banerjee, T., Wells, S. J., Roynette, C. E., Wahle, K. W., & Calder, P. C. (2006). Dose-related effects of eicosapentaenoic acid on innate immune function in healthy humans: a comparison of young and older men. American Journal of Clinical Nutrition, 83, 331–342.
- Robinson, D. S. (2010). The role of the T cell in asthma. Journal of Allergy and Clinical Immunology,126, 1081–1091, quiz 1092–1093.10.1016/j.jaci.2010.06.025
- Roulet, M., Frascarolo, P., Pilet, M., & Chapuis, G. (1997). Effects of intravenously infused fish oil on platelet fatty acid phospholipid composition and on platelet function in postoperative trauma. Journal of Parenteral and Enteral Nutrition, 21, 296–301.10.1177/0148607197021005296
- Saleh, D., Ernst, P., Lim, S., Barnes, P. J., & Giaid, A. (1998). Increased Formation of the potent oxidant peroxynitrite in the airways of asthmatic patients is associated with induction of nitric oxide synthase: Effect of inhaled glucocorticoid. Federation of American Societies for Experimental Biology, 12, 929–937.
- Sandrini, A., Taylor, D. R., Thomas, P. S., & Yates, D. H. (2010). Fractional exhaled nitric oxide in asthma: An update. Respirology, 15, 57–70.10.1111/resp.2010.15.issue-1
- Sastre, B., & del Pozo, V. (2012). Role of PGE2 in asthma and nonasthmatic eosinophilic bronchitis. Hindawi Publishing Corporation Mediators of Inflammation, 2012, Article ID 645383, 9 p.
- Schifano, E. D., Hollenbach, J. P., & Cloutier, M. M. (2014). Mismatch between asthma symptoms and spirometry: Implications for managing asthma in children. The Journal of Pediatrics, 165, 997–1002.10.1016/j.jpeds.2014.07.026
- Schiller, B., Hammer, J., Barben, J., & Trachsel, D. (2009). Comparability of a hand-held nitric oxide analyser with online and offline chemiluminescence-based nitric oxide measurement. Pediatric Allergy and Immunology, 20, 679–685.10.1111/pai.2009.20.issue-7
- Schubert, R., Kitz, R., Beermann, C., Rose, M. A., Lieb, A., Sommerer, P. C., … Zielen, S. (2009). Effect of n-3 polyunsaturated fatty acids in asthma after low-dose allergen challenge. International Archives of Allergy and Immunology, 148, 321–329.10.1159/000170386
- Senkal, M., Geier, B., Hannemann, M., Deska, T., Linseisen, J., Wolfram, G., & Adolph, M. (2007). Supplementation of ω-3 fatty acids in parenteral nutrition beneficially alters phospholipid fatty acid pattern. Journal of Parenteral and Enteral Nutrition, 31, 12–17.10.1177/014860710703100112
- Sterk, P. J., Fabbri, L. M., Quanjer, P. H., Cockcroft, D. W., O’Byrne, P. M., Anderson, S. D., … Malo, J. L. (1993). Standardized challenge testing with pharmacological, physical and sensitizing stimuli in adults. European Respiratory Journal, 6, 53–83.10.1183/09041950.053s1693
- Surette, M. E., Stull, D., & Lindemann, J. (2008). The impact of a medical food containing gammalinolenic and eicosapentaenoic acids on asthma management and quality of life of adult asthma patients. Current Medical Research and Opinion, 24, 559–567.
- Szefler, S. J., Wenzel, S., Brown, R., Erzurum, S. C., Fahy, J. V., Hamilton, R. G., … Minnicozzi, M. (2012). Asthma outcomes: Biomarkers. Journal of Allergy and Clinical Immunology, 129, S9–23.
- Tai, E. S., Corella, D., Demissie, S., Cupples, L. A., Coltell, O., Schaefer, E. J., … Framingham Heart Study. (2005). Polyunsaturated fatty acids interact with the PPARA-L162 V polymorphism to affect plasma triglyceride and apolipoprotein C-III concentrations in the Framingham Heart Study. Journal of Nutrition, 135, 397–403.
- Thien, F. C., De Luca, S., Woods, R. K., & Abramson, M. J. (2011). Cochrane review: Dietary marine fatty acids (fish oil) for asthma in adults and children. Evidence-Based Child Health: A Cochrane Review Journal, 6, 984–1012.10.1002/ebch.v6.3
- Thies, F., Nebe-von-Caron, G., Powell, J. R., Yaqoob, P., Newsholme, E. A., & Calder, P. C. (2001). Dietary supplementation with w-linolenic acid or fish oil decreases T lymphocyte proliferation in healthy older humans. Journal of Nutrition, 131, 1918–1927.
- Trebble, T., Arden, N. K., Stroud, M. A., Wootton, S. A., Burdge, G. C., Miles, E. A., … Calder, P. C. (2003). Inhibition of tumour necrosis factor-α and interleukin 6 production by mononuclear cells following dietary fish-oil supplementation in healthy men and response to antioxidant co-supplementation. British Journal of Nutrition, 90, 405–412.10.1079/BJN2003892
- Wada, M., DeLong, C. J., Hong, Y. H., Rieke, C. J., Song, I., Sidhu, R. S., & Yuan, C. (2007). Enzymes and receptors of prostaglandin pathways with arachidonic acid-derived versus eicosapentaenoic acid-derived substrates and products. Journal of Biological Chemistry, 282, 22254–22266.10.1074/jbc.M703169200
- Weiss, P., & Rundell, K. W. (2009). Imitators of exercise-induced bronchoconstriction. Allergy, Asthma & Clinical Immunology, 5, 7.10.1186/1710-1492-5-7
- Yaqoob, P., Pala, H. S., Cortina-Borja, M., Newsholme, E. A., & Calder, P. C. (2000). Encapsulated fish oil enriched in alpha-tocopherol alters plasma phospholipid and mononuclear cell fatty acid compositions but not mononuclear cell functions. European Journal of Clinical Investigation, 30, 260–274.10.1046/j.1365-2362.2000.00623.x
- Zitt, M. (2005). Clinical applications of exhaled nitric oxide for the diagnosis and management of asthma: A consensus report. Clinical Therapeutics, 27, 1238–1250.10.1016/j.clinthera.2005.07.005