Abstract
Purpose was to describe the perioperative isometry testing in anatomic reconstructions of the anterior cruciate ligament of the knee (ACL) using a single-bundle technique (MAXTEST). Anatomic ACL transtibial tunnel reconstruction was performed in 16 human cadaver knees. To assess the isometry, the length of Ethibond 2 ® suture thread passed through the tibial tunnel and attached to a guidewire placed at the estimated center point of the original ACL footprint on the lateral femoral condyle was measured one centimeter from the external tibial tunnel exit in the knee maximal 26 flexion and extension. The femoral entrance was modified before the perforation of the femoral tunnel to an anterior or posterior position until an isometric behaviour was observed. We called this assessment the MAXTEST. The obtained measurements were statistically analyzed. Additionally, a correlation test was conducted between the following: (1) the height from the guidewire exit point to the anterior surface of the lateral femoral condyle and the anteroposterior diameter of the lateral femoral condyle; (2) the distance from the articular surface of the tibia to the intercondylar roof and the distance between the guidewire and the intercondylar roof.
The performed statistical analysis showed that the anatomic reconstruction pattern could be isometric (p-value=0.654). A linear positive correlation was found between the height from the guidewire exit point to the anterior surface of the lateral femoral condyle and the anteroposterior diameter of the lateral femoral condyle (R2=0.677, multiple R=0.823, adjusted R2=-1.154, standard error=2.72). Additionally, a positive correlation was observed between the distance from the articular surface of the tibia to the intercondylar roof and the distance between the guidewire and the intercondylar roof (R2=0.96, multiple R=0.98, adjusted R2=-1.143, standard error=0.319). We concluded that “MAXTEST” is a reproducible isometry test in anatomic single- bundle ACL reconstructions when performed before the femoral tunnel perforation.
Public Interest Statement
This paper describes the perioperative isometry testing in anatomic reconstructions of the anterior cruciate ligament of the knee (ACL) using a single-bundle technique (MAXTEST). Anatomic ACL transtibial tunnel reconstruction was performed in 16 human cadaver knees. A linear positive correlation was found between the height from the guidewire exit point to the anterior surface of the lateral femoral condyle and the anteroposterior diameter of the lateral femoral condyle. Additionally, a positive correlation was observed between the distance from the articular surface of the tibia to the intercondylar roof and the distance between the guidewire and the intercondylar roof showing that the “MAXTEST” is a reproducible isometry test in anatomic single-bundle ACL reconstructions when performed before the femoral tunnel perforation.
1. Introduction
The anterior cruciate ligament (ACL) is the main restrictor of the anterior translation of the tibia;1 additionally, it facilitates the restriction of varus and valgus alignment when the knee is in extension (Butler, Noyes, & Grood, Citation1980; Grood, Suntay, Noyes, & Butler, Citation1984). It originates on the medial surface of the lateral condyle and has an intra-articular and extra-synovial anteroposterior insertion to the anterior tibial spine (Fukubayashi, Torzilli, Sherman, & Warren, Citation1982; Grood et al., Citation1984). It has two very distinct bundles: the anteromedial bundle is tight in flexion and important in translation, while the posterolateral bundle is tight in extension and important in rotation (Fukubayashi et al., Citation1982).
Since the initial description of the intra-articular ACL reconstruction technique, the concept regarding the ligament bundles, their functions, and the golden surgical approach for this reconstruction has changed several times (Jones, Citation1963). The isometric technique aims at the reconstruction of the anteromedial bundle (11 h—right, 1 h—left), aiming to obtain a negative translation, while the anatomic technique prioritized the reconstruction of the posterolateral bundle, which guided the graft most horizontally (9 h—right, 3 h—left), in order to obtain a negative rotation. (Bonamo, Krinick, & Sporn, Citation1984; Jones, Citation1963; Ristanis et al., Citation2009).
However, no definitive pattern is currently available regarding the choice of the bundle to be reconstructed or the reproduction of one (single bundle) or two bundles (double bundle) of the ligament (Bonamo et al., Citation1984; DeLee & Craviotto, Citation1991; Jones, Citation1963; Ristanis et al., Citation2009). The authors used a cadaveric model to study the possibility of an anatomic ACL reconstruction with an isometric biomechanical behavior and tried to reproduce a perioperative test in order to choose the appropriate graft placement that would meet this requirement in a single-bundle ACL reconstruction. Therefore, the objective of this study was to describe a perioperative isometry assessment, which the authors called “MAXTEST,” in order to choose the femoral tunnel perforation point in the anatomic reconstruction of the ACL.
2. Methods
2.1. Research subjects
Between May and July 2014, 16 human cadaver knees (eight right knees and eight left knees) preserved in formaldehyde were studied at the Medical School Anatomy Laboratory of the Federal University of Rio de Janeiro (Unirio). Of the total number of studied cadavers, five were female and three were male, and the presumed age was 20–40 years. The inclusion criteria were as follows: adult knees without previous surgical manipulation or dissection; minimum knee joint flexion mobility of 120°; the right and left knees; both sexes. The exclusion criteria were as follows: adult knees previously manipulated in surgery or dissected; knees that did not reach a minimum of 120° of flexion; knees with degenerative intra-articular disease or previous ACL lesion.
2.2. Methodology
The cadavers were removed from formaldehyde vats 3 h before the beginning of the study. The identification of the medial collateral ligament (MCL) was performed through a medial skin incision and medial and lateral capsulotomy. The quadriceps tendon was proximally folded and medial soft tissues were dissected. Subsequently, the knee were positioned in maximum flexion and fixed on the dissection table. The ACL was then identified, and the anteromedial and posterolateral bundles were dissected for visualization of their origin and insertion points for correct reproduction of the footprint center. A 55° Arthrex® guide (Arthrex, Naples, FL) was placed immediately anterior to the MCL, allowing an adequate anatomical angular orientation to insert the two bundles in the tibia–tibial footprint. The 2.5-mm-diameter guidewire was passed using an electric drill, with visualization of the exit point in the tibial footprint, and then the tibial tunnel was perforated using a 9-mm drill bit. The common origin of the two bundles was identified and the guidewire was passed through the femur, the transtibial tunnel, the lateral intercondylar wall, and the point between the origin of the native ACL bundles, considered the anatomic femoral footprint (Figure ). An Ethibond® Ethicon 2 suture (Ethicon Inc., Somerville, NJ) thread was inserted into the guidewire hole and slowly drawn until the complete entry into the cortical articulation of the femoral condyle (Figure ). Isometry was assessed using a hemostatic forceps placed 1 cm from the external origin of the tibial tunnel, with the knee positioned at 90°. This distance was measured with a pachymeter; subsequently, the measurement was repeated in the maximum extension and flexion of the knee, and the distance between the forceps and the tibial tunnel entrance was assessed. When non-isometric behavior was observed and the forceps was moved closer to the entrance of the external tibial tunnel during flexion, showing that the femoral tunnel was at an excessively posterior position, the thread was removed and repositioned in a more posterior point. This procedure was repeated until the thread maintained the same measure in maximum knee flexion and extension (Figures and ). In addition, several measurements of the femur and tibia were attempted to evaluate their correlation with the measured anatomical evaluations.
Figure 1. Guidewire passing between the anteromedial and posterolateral bundles of the ACL.
Source: Anatomy Laboratory–UNIRIO, 2015.
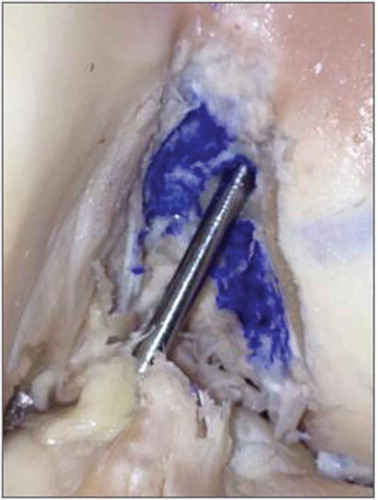
Figure 2. Ethibond 2 suture thread passing between the anteromedial and posterolateral bundles of the ACL.
Source: Anatomy Laboratory–UNIRIO, 2015.
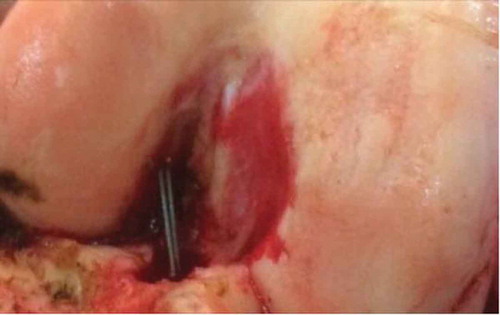
Figure 3. Hemostatic forceps placed on the suture thread, 1 cm from the tibial cortex with the knee in flexion.
Source: Anatomy Laboratory–UNIRIO, 2015.
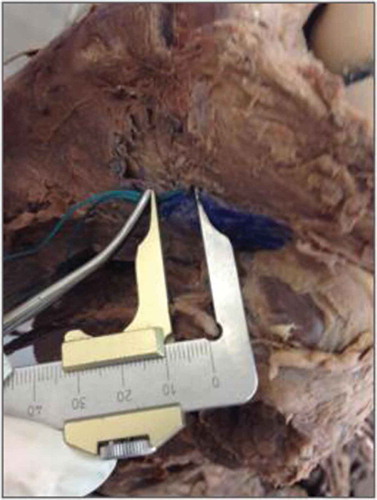
Tibial measurements:
a. Laterolateral measurement (immediately anterior to the MCL).
b. Height from the guidewire to the articular surface (one end of the pachymeter was positioned on the guidewire and the other on the anterior aspect of the tibia).
c. Distance from the guidewire passage in the tibial footprint to the posterior cruciate ligament (PCL) (Figure ).
Figure 4. Thread measurement with the knee in extension.
Source: Anatomy Laboratory–UNIRIO, 2014–2015.
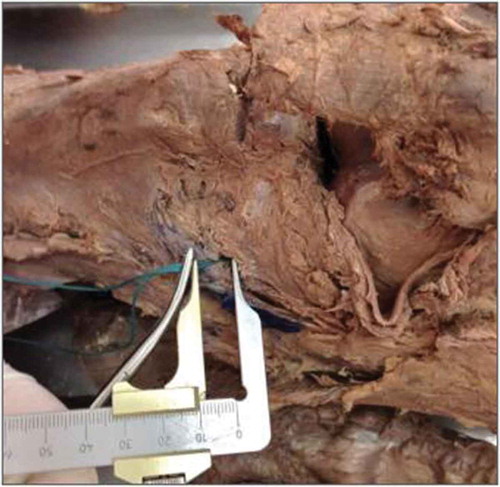
Femoral measurements:
d. Laterolateral measurement (at the level of the epicondyle).
e. Anteroposterior measurement (posterolateral femoral condyle to uppermost point of the femoral condyle) (Figure ).
f. Height from the guidewire exit point in relation to the anteroposterior measurement (Figure ).
Figure 5. Distance from the guidewire placed in the tibial “footprint” to the PCL.
Source: Anatomy Laboratory–UNIRIO, 2014–2015.
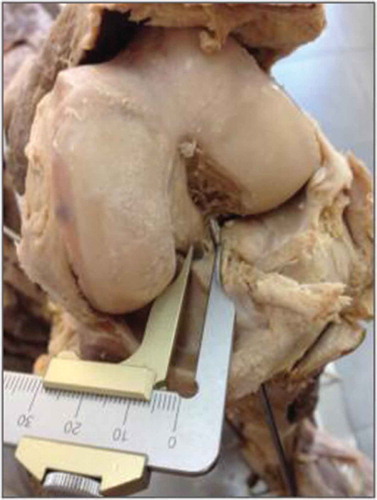
g. Height from the articular surface of the tibia to the intercondylar roof (Figure ).
Figure 6. Height of the guidewire exit point in relation to the anteroposterior measurement of the lateral femoral condyle.
Source: Anatomy Laboratory–UNIRIO, 2014–2015.
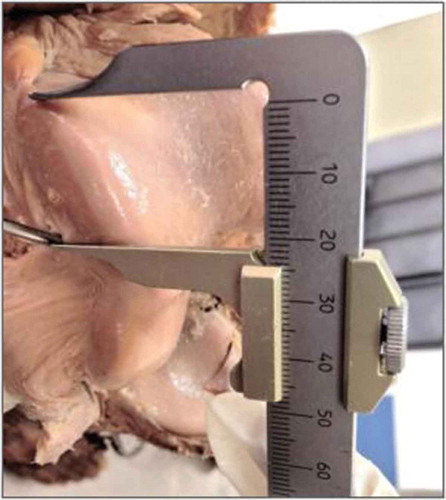
h. Guidewire length up to the intercondylar roof (Figure ).
2.3. Statistical evaluation
The anatomo-topographic measurements were submitted to descriptive analysis and expressed in tables and graphs. Isometry was assessed through a statistical analysis of the length of the threads in extension and flexion. As the data were paired, the Wilcoxon test was used, starting from the null hypothesis of equality for the population mean, that is, that the length of the threads of the evaluated knees in extension and flexion follows a normal distribution. The nonparametric test was chosen because of the non-Gaussian sample, as evidenced by the Anderson–Darling normality test (p ≤ 0.0005). The correlation test was conducted for the measurements of the following: (1) the height from the exit point of the guidewire to the anterior surface of the lateral femoral condyle and the anteroposterior diameter of the lateral femoral condyle; and (2) the distance from the articular surface of the tibia to the intercondylar roof and the distance between the guidewire and the intercondylar roof.
3. Results
The anatomic measurement of laterolateral distance of the samples varies from 68 to 88 mm, average 79 mm, mode 68, SD 6.2822; anteroposterior average distance was 23.43, varying from 16 to 38, mode 16, SD 15.715 mm. The distance from the guidewire passage in the tibial footprint to the PCL was 6 mm in all specimens. The mean femoral laterolateral distance was 79.87 mm, ranging from 72 to 86 mm; anteroposterior measurement was 69.56 mm, varying from 61 to 76 mm. The height from the articular surface of the tibia to the intercondilar roof was 31.68 mm (from 29 to 35 mm, SD 1.74, mode 32). The height from the guidewire in relation to the anteroposterior axis was 25.18 mm (22–27, SD 1.72). The guidewire length was 15.75 mm (15–17). (Table and Graphs 1 and 2). The statistical analysis of the thread length values of the knees in extension and flexion, with a significance level of 5% (α = 0.05), showed that the reconstruction pattern was isometric since the p-value was 0.654. A positive linear correlation was found between the height from the guidewire exit point to the anterior surface of the lateral femoral condyle and the anteroposterior diameter of the lateral femoral condyle (R2 = 0.677, multiple R = 0.823, adjusted R2 = 1.154, and standard error = 2.72). Additionally, a positive correlation was observed between the distance from the tibial articular surface to the intercondylar roof and the distance between the guidewire and the intercondylar roof (R2 = 0.96, multiple R = 0.98, adjusted R2 = −1.143, and standard error = 0.319). The descriptive and graphic analysis of these parameters showed that the measurements of the distance between the guidewire and the intercondylar roof were very close to or equal to half of the values of distance from the articular surface of the tibia to the intercondylar roof (Table ).
Table 1. Obtained values from eight measurements performed on the 16 studied knees
Table 2. Descriptive analysis of the distance between the guidewire and the intercondylar 341 roof and between the articular surface of the tibia and the femoral intercondylar roof 342
4. Discussion
Several intra-articular ACL reconstruction techniques in order to obtain a more physiological stability of the knee in case of a deficient ACL have been reported (Bonamo et al., Citation1984; Cooper, Deng, Burstein, & Warren, Citation1993; DeLee & Craviotto, Citation1991; Milano et al., Citation2006; Shelbourne & Nitz, Citation1990). Both the anatomic and isometric reconstructions tend to achieve the ideal positioning of the tunnels, thereby allowing the graft to achieve a maximum complex stabilization of the native ACL and to restore the normal knee kinematics. Despite the several ACL reconstruction techniques and possibilities, no consensus regarding the best surgical option is currently available (Fukubayashi et al., Citation1982). Studies found that both the single- and double-bundle techniques can restore the internal rotation and the anterior translation of the knee joint with a 15–30% probability of revision per year in the USA (Butler, Mellecker, Rudert, & Albright, Citation2013). Previous studies did not find clinical or statistical differences between the single- and double-bundle reconstruction methods and the double-bundle reconstruction was not found to provide better control of knee rotation (Desai et al., Citation2016; Martins, Kropf, Shen, van Eck, & Fu, Citation2012; Massada & Silva, Citation2013; Meredick, Vance, Appleby, & Lubowitz, Citation2008; Zantop et al., Citation2008). Reproducible patterns remain unclear and most surgical techniques show different results. Although the real reason for the detected inconsistencies remains unknown, authors present the anatomical variations as the main cause, but no perioperative dynamic test methodology was found to predict the biomechanical result after the reconstruction (Desai et al., Citation2016; Meredick et al., Citation2008). Considering the traditional reconstruction techniques, which use a single graft, and the new understanding based on the anatomy of the studied bundles, there is a current tendency to customize the surgery based on each patient’s anatomy, location of the ligament insertion, size of the intercondylar fossa, and shape of the native ACL (Murawski et al., Citation2013). However, the question is how to customize the surgery without the used surgical method confirmation and how to minimize the incidence of misconceptions. It is important to highlight that studies have already confirmed that when an ACL is not anatomically reconstructed the knee becomes susceptible to osteoarthritis; conversely, a correctly performed surgical procedure should prevent osteoarthritis and allow reassuming sports activities (Murawski et al., Citation2013). Generally, the previously described single-bundle techniques promote the anterior translation (isometric) or the stabilization of rotation (anatomic) (Zantop, Herbort, Raschke, Fu, & Petersen, Citation2007). The anatomic reconstructions conducted in this study were submitted to the perioperative isometry test, “MAXTEST,” and showed a possible isometric biomechanical behavior (p = 0.669) in these experimental models, with the possibility to modify the femoral tunnel perforation point to a more anterior or posterior position in order to obtain a reconstruction pattern if the footprint anatomical parameters were maintained. A positive correlation was observed in the present study between the distance from the tibial articular surface to the intercondylar roof and the distance between the guidewire and the intercondylar roof in the models with unaltered length of the threads by the biomechanical behavior of the anatomical reconstruction. The descriptive analysis of these models showed that the measurements of the distance between the guidewire and the intercondylar roof are very close to or equal to half of the distance from the articular surface of the tibia to the intercondylar roof, which directs the ideal positioning of the femoral tunnel approximately to the midpoint of the height of the intercondylar lateral wall, with a strong tendency to graft horizontalization. No study with this design or with similar measurement evaluations was found in the performed literature review (PubMed, SciELO, and LILACS). The cadaver model reproduces in vivo patterns. The results obtained and the efficacy of MAXTEST should be reproducible in real patients.
5. Conclusion
The presented technique for measuring the biomechanical behavior prior to the femoral tunnel perforation in ACL reconstruction proved reproducible, accurate and easy to perform, and can be adapted to any methodology and material reported to date.
Competing Interests
The authors declare no competing interest.
Citation information
Cite this article as: Topographic study of the isometrytesting in anatomic reconstructions of the anterior cruciate ligament of the knee, Joao Gabriel Villardi et al., Cogent Medicine (2018), 5: 1470067.
Additional information
Funding
Notes on contributors
Joao Gabriel Villardi
João Gabriel de Cerqueira Campos Villardi, M.D, MSc, is an orthopaedic surgeon, knee surgery specialist, member of the SBOT, Master in Medicine, staff of the Knee & Shoulder Team at the Gafrée Guinle University Hospital (HUGG - UNIRIO).
Alfredo Villardi
Alfredo Marques Villardi MD, PhD, is an orthopaedic surgeon, knee surgery specialist, Doctor in Medical Science, professor at the Veiga de Almeida University.
Leonardo Antunes Bellot de Souza
Leonardo Antunes Bellot de Souza MD, MSc is an orthopaedic surgeon, knee surgery specialist, member of the SBOT, Master in Medicine, staff of the Knee & Shoulder Team at the Gafrée Guinle University Hospital (HUGG - UNIRIO).
Yonder Archanjo Ching San Junior
Yonder Archanjo Ching San Junior, MD, MSc, orthopaedic surgeon, shoulder & elbow specialist, Master in Medicine at UNIRIO.
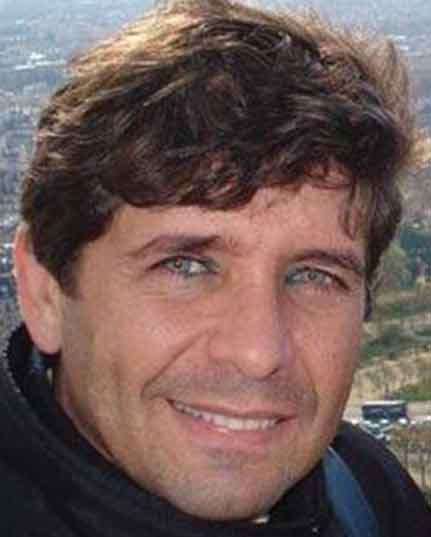
Max Ramos
Max Ramos, MD, PhD, is Associate Professor in UNIRIO, CEO of residence program in orthopedic, surgery and MSc program coordinator at UNIRIO, chief surgeon in knee, shoulder and sport’s medicine at HUGG-UNIRIO, master’s at Ciências Cirúrgicas from Universidade Federal do Rio de Janeiro (1997), and Ph.D. at Ciências Cirúrgicas from Universidade Federal do Rio de Janeiro (2004).
References
- Bonamo, J. J., Krinick, R. M., & Sporn, A. A. (1984). Rupture of the patellar ligament after use of its central third for anterior cruciate reconstruction. A report of two cases. The Journal of Bone & Joint Surgery, 66, 1294–12.
- Butler, D. L., Noyes, F. R., & Grood, E. S. (1980). Ligamentous restraints to anterior- posterior drawer in the human knee. A biomechanical study. The Journal of Bone & Joint Surgery, 62, 259–270.
- Butler, P. D., Mellecker, C. J., Rudert, M. J., & Albright, J. P. (2013). Single-bundle versus double-bundle ACL reconstructions in isolation and in conjunction with extra-articular iliotibial band tenodesis. The Iowa Orthopaedic Journal, 33, 97–106.
- Cooper, D. E., Deng, X. H., Burstein, A. L., & Warren, R. F. (1993). The strength of the central third patellar. tendon graft. The American Journal of Sports Medicine, 21, 818–824.
- DeLee, J. C., & Craviotto, D. F. (1991). Rupture of the quadriceps tendon after a central third patellar tendon anterior cruciate ligament reconstruction. The American Journal of Sports Medicine, 19, 415–416.
- Desai, N., Alentorn-Geli, E., van Eck, C. F., Musahl, V., Fu, F. H., Karlsson, J., & Samuelsson, K. (2016). A systematic review of single- versus double-bundle ACL reconstruction using the anatomic anterior cruciate ligament reconstruction scoring checklist. Knee Surgery, Sports Traumatology, Arthroscopy, 24, 862–872.
- Fukubayashi, T., Torzilli, P. A., Sherman, M. F., & Warren, R. F. (1982). An in vitro biomechanical evaluation of anterior-posterior motion of the knee. Tibial displacement, rotation, and torque. The Journal of Bone & Joint Surgery, 64, 258–264.
- Grood, E. S., Suntay, W. J., Noyes, F. R., & Butler, D. L. (1984). Biomechanics of the knee- extension exercise. Effect of cutting the anterior cruciate ligament. The Journal of Bone & Joint Surgery, 66, 725–734.
- Jones, K. G. (1963). Reconstruction of the anterior cruciate ligament. A technique using the central one third of the patellar ligament. The Journal of Bone & Joint Surgery, 45, 925–932.
- Martins, C. A. Q., Kropf, E. J., Shen, W., van Eck, C. F., & Fu, F. H. (2012). The concept of anatomic anterior cruciate ligament reconstruction. Operative Techniques in Sports Medicine, 20, 7–18.
- Massada, M., & Silva, A. (2013). Reconstrução anatômica do LCA com duplo túnel. Onde nos encontramos hoje? Rev Portuguesa Ortop Traum, 21, 479–485.
- Meredick, R. B., Vance, K. J., Appleby, D., & Lubowitz, J. H. (2008). Winner of the 2007 systematic review competition: Outcome of single-bundle versus double-bundle reconstruction of the anterior cruciate ligament: A meta- analysis. The American Journal of Sports Medicine, 36, 1414–1421.
- Milano, G., Mulas, P. D., Ziranu, F., Piras, S., Manunta, A., & Fabbriciani, C. (2006). Comparison between different femoral fixation devices for ACL reconstruction with doubled hamstring tendon graft: A biomechanical analysis. Arthroscopy: The Journal of Arthroscopic & Related Surgery, 22, 660–668.
- Murawski, C. D., Wolf, M. R., Araki, D., Muller, B., Tashman, S., & Fu, F. H. (2013). Anatomic anterior cruciate ligament reconstruction. Cartilage, 4, 27S–37S.
- Ristanis, S., Stergiou, N., Siarava, E., Ntoulia, A., Mitsionis, G., & Georgoulis, A. D. (2009). Effect of femoral tunnel placement for reconstruction of the anterior cruciate ligament on tibial rotation. The Journal of Bone and Joint Surgery-American Volume, 91, 2151–2158.
- Shelbourne, K. D., & Nitz, P. (1990). Accelerated rehabilitation after anterior cruciate ligament reconstruction. The American Journal of Sports Medicine, 18, 292–299.
- Zantop, T., Diermann, N., Schumacher, T., Schanz, S., Fu, F. H., & Petersen, W. (2008). Anatomical and nonanatomical double-bundle anterior cruciate ligament reconstruction: Importance of femoral tunnel location on knee kinematics. The American Journal of Sports Medicine, 36, 678–685.
- Zantop, T., Herbort, M., Raschke, M., Fu, F. H., & Petersen, W. (2007). The role of the anteromedial and posterolateral bundles of the anterior cruciate ligament in anterior tibial translation and internal rotation. The American Journal of Sports Medicine, 35, 223–227.