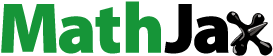
ABSTRACT
This study investigated the potential use of biochar produced from Iraqi date kernels (Khestawi) as an adsorbent for methylene blue (MB) removal from polluted water. A full factorial design methodology was employed to examine the effects of heating rate, pyrolysis temperature and holding time on the performance of the produced biochar. The results showed that pyrolysis temperature and time had a strong effect on the biochar performance, while the heating rate had a weaker effect. The biochar produced at pyrolysis temperatures of 350°C and 550°C exhibited MB removal percentages (% R) of 48.9% and 30.9%, respectively. Similarly, biochar production through pyrolysis durations of 30 and 90 minutes resulted in MB removal rates of 47.5% and 30.3%, respectively. The adsorption experimental data was well-fitted by the Freundlich isotherm model, with a high value of the Freundlich constant (KF = 1.64), indicating a high adsorption capacity of the biochar. The activation of biochar using bio-oil (organic-1) led to a substantial enhancement in the adsorption capacity, with the activated biochar (ABC-1) exhibiting a higher adsorption capability and a higher value of the Freundlich constant (KF = 7.12). Overall, the results suggest that biochar produced from date kernels has the potential as an effective and sustainable adsorbent for MB removal from polluted water, with the potential for further enhancement through biochar activation.
Introduction
According to data from the Food and Agriculture Organization (FAO), date palm production globally covers an area estimated at more than 1 million hectares. The total production exceeds 8 million tons annually, while there are about 2.5 thousand varieties of date palms around the world (El Hadrami & Al-Khayri, Citation2012). Palm cultivation is concentrated globally, particularly in the Asia region, which accounts for 55.8% of global production, followed by Africa, which accounts for 43.4% of production. The Arab region accounts for more than 77% of date production, or about 6.6 million tons annually, and there are about 160 million palm trees in the region.
Several Arab countries are major producers of dates, including Saudi Arabia, Egypt, Iraq, Tunisia, Algeria, and the United Arab Emirates (Erskine et al., Citation2004). According to the data of the Central Statistical Organization of the Iraqi Ministry of Planning, the volume of production for the past year amounted to about 735 thousand tons from about 17 million palm trees (CSO, Citation2020). The volume of local consumption for all Iraqi governorates ranges between 150 and 200 thousand tons annually of dates. Among the types of Iraqi dates that still appeal to citizens are as follows: Khestawi, Khadrawi, Ashrassi, Jamali, Halawi, Sayer, Maktoum, Barhi, and Zahdi (CSO, Citation2020).
Date kernels, which are the seeds found inside the fruit of the date palm tree, have several uses. The kernels can be exploited for animal feed production for livestock, such as sheep, goats, and camels (Attia et al., Citation2021). Ground date kernels are sometimes used as a natural exfoliant in skincare products such as scrubs and soaps (Jahan & Happy, Citation2022). Date kernel oil, which is extracted from the kernels, is used in the cosmetics industry as a moisturizer and hair conditioner. It can also be used in the production of soaps, detergents, and lubricants. In some cultures, date kernels are used in traditional medicine for their purported health benefits, such as for digestive issues or as a diuretic (Younas et al., Citation2020). Date kernels can be used to produce biofuel via pyrolysis, which involves heating the kernels in the absence of oxygen to produce biochar, bio-oil, and gases such as hydrogen and methane (Aljeradat et al., Citation2021, Citation2022, Citation2023). Biochar produced from date kernel pyrolysis can be used as an amendment for soil to improve the fertility of soil and its water retention (Abid & Ammar, Citation2022).
Biochar derived from date kernels is an effective adsorbent that can be used to remove contaminants from air, water, and soil (Tahir et al., Citation2020). The utilization of date kernel biochar as an adsorbent offers several advantages over conventional adsorbents. Date kernel biochar is often less expensive to produce than activated carbon, which makes it a more cost-effective alternative. In addition, the properties of biochar can be tailored to specific applications through the use of different pyrolysis conditions. This allows for the customization of biochar for specific adsorption needs (Foong et al., Citation2020). Furthermore, biochar is stable and can be used for long periods without losing its effectiveness (Al-Wabel et al., Citation2019).
Activation of biochar is a process that involves modifying the surface properties of biochar for better adsorption properties. Biochar activation can affect the physical and chemical properties of the biochar, such as its surface area, pore size distribution, and surface chemistry (Sakhiya et al., Citation2020). During chemical activation, the biochar is treated with a chemical agent to create pores on the surface of the biochar. Several chemical agents can be used for activation, such as: acids, bases, or salts. The biochar is impregnated with the chemical agent and then heated to activate the pores. Physical activation may involve heating the biochar in the presence of an inert gas, such as carbon dioxide or nitrogen, to create pores on the surface of the biochar. The heating process is believed to cause the volatiles in the biochar to be released, which creates voids and channels on the surface of the biochar (Cha et al., Citation2016).
In this study, the primary objective is to investigate the effectiveness of date kernels as an adsorbent for MB removal from polluted water. To achieve this primary objective, we employed a factorial design methodology to systematically analyze and understand how variations in key process variables, namely pyrolysis temperature, holding time, and heating rate, impact the performance of date kernel-based biochar as a dye adsorbent. Additionally, we examined possible interaction effects among these process variables.
The novelty of using date kernels as an adsorbent for MB removal from water lies in its sustainability, natural adsorption properties, local resource utilization, cultural relevance, and reduced environmental impact, making it a promising and unique approach in the field of water treatment.
Materials and methods
Date kernels preparation and characterization
Date kernel samples were collected from Iraqi date known as Khestawi, which were collected from palm farms located between the governorates of Baghdad and Babylon in Iraq, in 2021.
The samples were washed in deionized water several times and cleaned manually to remove dust, impurities, and remnants of date fruits. The date kernels were then air-dried for 48 hours at room temperature before being packaged in airtight bags to prevent any contamination.
The moisture content, volatile matter, fixed carbon, and ash content of date kernels were all determined using the conventional proximate analysis method (ASTM D3172, Citation2013). A representative sample of date kernels is weighed and dried in an oven at 105°C until a constant weight is obtained. The weight loss during drying is recorded as the moisture content of the sample. To drive out the volatile matter, a dried sample from the moisture determination phase is heated in a controlled environment in a muffle furnace at 550°C for 15 minutes. The weight loss during this step is recorded as the volatile matter content of the sample. The residue from the volatile matter determination phase is ashed in the muffle furnace at 900°C until all organic matter has been burned off and only inorganic ash remains. The weight of the ash is recorded as the ash content of the sample. The fixed carbon content is calculated as the difference between 100% and the sum of the moisture, volatile matter, and ash content. Moreover, the date kernels were subjected to ultimate analysis to determine the elements: carbon (C), hydrogen (H), oxygen (O), and nitrogen (N) following ASTM method (ASTM D3176, Citation2015).
Biochar production
In a bench-scale facility, biochar is produced using pyrolysis. The pyrolytic reactor is a stainless-steel tube (30 cm long and 4 cm in diameter). The tube is designed with a flange at one end, which serves to facilitate the feeding of date kernels into the reactor. In this batch process, the feedstock is charged into the reactor through this flange. Once the date kernels are loaded, the reactor is closed and heating is initiated. Once the process is complete and the system is shut down, the reactor is opened, and the resulting biochar is collected. The flange not only aids in the initial loading of the feedstock but also ensures that the material is properly contained during the heating process, thereby enhancing the overall efficiency and safety of the operation. The reactor is heated using a tubular furnace (Nabertherm, Germany). The heating rate and final temperature can be controlled using the furnace’s built-in software.
A typical experimental procedure consists of charging 50 g in the reactor, fastening the flanges, placing the reactor in the furnace, and starting pyrolysis at low nitrogen flow and at a given heating rate and final temperature. Several heating rates, final temperatures, and holding periods have been used in this study as will be demonstrated later.
The pyrolysis products were routed into a downstream oil and gas capture system via a silicon tube. The capturing system consists of two sequentially connected impingers. The two impingers are immersed in an ice bath to ensure perfect condensation and capturing of the bio-oil. The non-condensable gases were collected using sampling bags.
Biochar activation
Biochar samples obtained at pyrolysis conditions of 20°C/min heating rate, 450°C final temperature, and one-hour holding time were subjected to chemical activation. The activation process comprised the impregnation of the biochar samples with the bio-oil obtained from the same pyrolysis process after refinement. The collected bio-oil was first mixed with deionized water in a volume ratio of 1:4. The mixture was vigorously mixed for 30 min then allowed to settle. Two distinct layers were obtained, namely the bio-oil organic phase (Organic 1) and the bio-oil aqueous phase (BOAP-1). BOAP-1 was then mixed with chloroform in a volume ratio of 1:2. The mixture was vigorously mixed for 30 min then allowed to settle. Two distinct layers were obtained, namely: the solvent phase (Organic 2) and the second bio-oil aqueous phase (BOAP-2). Both organic 1 and organic 2 phases were used as activating agents for biochar.
The impregnated biochar samples were subjected to pyrolysis conditions of 20°C/min heating rate, 150°C final temperature and one-hour holding time. Biochar activated with organic 1 is designated as ABC-1, while biochar activated with organic 2 is designated as ABC-2.
The adsorption capacity for each activated biochar sample was determined by obtaining the adsorption isotherms at room temperature.
Full factorial design methodology
Full factorial design methodology was followed to study the effect of three different operational conditions on biochars’ adsorption capacity. The three operational conditions investigated in this study were as follows: heating rate (HR), final reactor temperature (Temp), and the holding time (Time). A full factorial design methodology of the type 23 was used, including three experiments at the center points. The process parameters and their associated levels are given in .
Table 1. Operating conditions employed in the full factorial design methodology.
shows the design matrix that will be used for the factorial design process.
Table 2. Experimental matrix based on full factorial design for three process variables: pyrolysis temperature, holding time, and heating rate.
The adsorption capacity for each produced biochar sample was determined by obtaining the adsorption isotherms at room temperature.
To analyze the data and assess the impact of key process variables on the performance of date kernel-based biochar as a dye adsorbent, a factorial design approach was employed. The experimental design included varying pyrolysis temperature, holding time, and heating rate as independent variables.
After conducting the experiments, the data were subjected to analysis of variance (ANOVA). ANOVA was used to test the main effects of each process variable (pyrolysis temperature, holding time, and heating rate) as well as the interaction effects between these variables.
The significance of the effects was determined by calculating p-values, with statistical significance set at an alpha level of 0.05. Variables with p-values less than 0.05 were considered statistically significant, indicating that they have a significant impact on the adsorption performance of the date kernel-based biochar.
This approach allows us to systematically evaluate how variations in process parameters influence the effectiveness of date kernels as an adsorbent for MB removal from polluted water, providing insights into optimal conditions for biochar production and application.
Batch adsorption experiments
A methylene blue solution is prepared by dissolving a known amount of methylene blue (MB) in deionized water. A feedstock solution with 100 ppm MB concentration was first prepared, then other lower MB concentration solutions (50, 25, 12.5, and 6.25 ppm) were prepared following subsequent dilutions.
A series of adsorption experiments is conducted in batch mode, where 500 ml of the adsorbate solution is mixed with 2 g of biochar in a glass container. The container is then tightly sealed to prevent evaporation or leakage. The solution pH value is maintained at 10. The adsorbate and adsorbent are allowed to equilibrate for four hours, with gentle agitation (200 rpm) to promote contact between the adsorbate and the adsorbent. This allows the adsorption process to reach equilibrium, where no further adsorption or desorption occurs. After the equilibration period, a small aliquot of the mixture is collected at the end of the equilibration period to determine the concentration of the adsorbate remaining in the solution (Ce). This is typically done by filtering the mixture to separate the solid adsorbent from the solution, and then analyzing the concentration of the adsorbate in the filtrate using UV-spectrometry at a wavelength of 664 nm.
The percentage removal of MB (% R) is calculated as follows:
where Ce is the equilibrium concentration.
The equilibrium uptake (Qe) is estimated as follows (Aliedeh et al., Citation2021):
where Co is the initial concentration of MB, V is the solution volume, and m is the mass of biochar.
The adsorption data obtained from the batch adsorption experiments is analyzed to determine the adsorption capacity and other adsorption parameters. Adsorption isotherms, namely Langmuir and Freundlich, were chosen to model the adsorption behavior and obtain insights into the adsorption mechanisms.
The linearized Langmuir isotherm is presented as follows (Aljbour et al., Citation2017):
where, b is Langmuir constant (L/mg), and Qm is the maximum adsorption capacity (mg/g).
The linearized Freundlich isotherm model is presented as (Aljbour et al., Citation2017):
where, KF is the adsorption capacity, and n is the adsorption intensity.
Results and discussion
Date kernels characterization
shows the results of the proximate and ultimate analyses of Khestawi date kernels.
Table 3. Proximate and ultimate analyses of Khestawi date kernels.
The proximate analysis of the date kernels indicates that the kernels have high volatile matter content (79.51 wt%). The volatile matter typically consists of organic compounds that can vaporize or decompose upon heating, including volatile gases, liquids, and low-molecular-weight organic compounds. The release of volatile matter during pyrolysis can affect the surface morphology of the produced biochar. The high volatile matter content can result in the formation of voids, cracks, or other surface features on the biochar, which can affect its surface roughness, texture, and structure. These changes in surface morphology can influence the adsorption behavior of the biochar by affecting the surface area, porosity, and accessibility of adsorption sites.
The fixed carbon content for Khestawi date kernels is 19.46 wt%, because its content does not volatilize during pyrolysis, and therefore, it remains in the biochar as solid carbonaceous material. This can contribute to the overall surface area and porosity of the biochar. Higher fixed carbon content can result in a biochar product with higher surface area and porosity, which can enhance its adsorption capacity and accessibility for adsorbates.
The oxygen content for Khestawi date kernels is 51.75 wt%. The high oxygen content in the biomass can result in bio-oil with a higher oxygen content, which can affect its properties, such as viscosity, heating value, and stability. Bio-oil with higher oxygen content tends to have higher viscosity and lower heating value, as oxygen is less energy-dense compared to carbon and hydrogen. Moreover, high oxygen content in the biomass can also affect the composition and properties of the pyrolysis gas. Furthermore, oxygen in the biomass can react with the pyrolysis products, such as hydrogen and carbon, during pyrolysis, resulting in a higher concentration of carbon monoxide (CO) and lower concentration of hydrogen (H2) in the syngas.
It is important to note that date kernels can vary in composition based on factors like the date palm variety, climate, soil conditions, and post-harvest processing.
Full factorial design analysis
Biochar samples produced at the operating conditions as specified by the full factorial design methodology are examined toward MB removal. The performance of the produced biochar samples in the removal of MB from polluted water is given in .
Table 4. Percentage of MB removal from polluted water using biochar.
The full factorial analysis allows us to extract the primary impacts of individual process variables on the biochar’s performance, specifically in terms of the percentage removal (% R) of MB. This analysis provides insight into the isolated influence of each variable on the dependent variable, disregarding the influence of other variables. Furthermore, the full factorial analysis facilitates the detection of interaction effects among the independent variables, elucidating how one variable’s impact on the dependent variable evolves as the level of another variable undergoes alterations.
shows the interaction effect plot for the process variables.
Figure 1. Interaction effects plot showing the influence of pyrolysis temperature, holding time, and heating rate on date kernel-based biochar adsorption capacity for methylene blue (MB) removal.
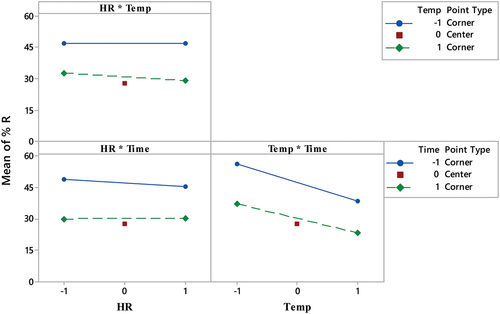
The interaction effect plot indicates a weak interaction effect among the process variables. This implies that the impact of each process variable (heating rate, temperature, and time) on the biochar’s performance remains consistent regardless of the levels of the other process variables. The influence of each independent variable on the dependent variable remains unaffected by the specific levels of the other independent variables.
It is essential to emphasize that a lack of interaction effect does not imply that the two independent variables have no effect on the dependent variable. Instead, it signifies that the influence of each independent variable operates independently, irrespective of the levels of the other independent variables (Box et al., Citation2005). Therefore, it is important to consider the main effects of each process variable on the performance of the produced biochar, even in the absence of any interaction effects. shows the main effects for each process variable.
Figure 2. Main effect plots illustrating the influence of pyrolysis temperature, holding time, and heating rate on date kernel-based biochar adsorption capacity for methylene blue (MB) removal.
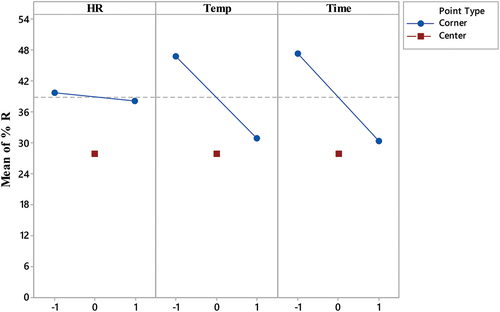
The main effect plots for each process variable indicate strong effect for pyrolysis temperature and time and week effect for the heating rate on the performance of biochar. The results indicate that increasing the pyrolysis temperature from 350°C to 550°C produced biochar samples exhibiting a decrease in the % R of MB from 48.9% to 30.9%, respectively. In addition, increasing the pyrolysis time from 30 to 90 min produced biochar samples exhibiting a decrease in the % R of MB from 47.5% to 30.3%, respectively. On the other hand, increasing the heating rate from 10°C to 30°C/min slightly affected the performance of the produced biochar, and the average % R of MB was almost 39%. Therefore, the pyrolysis temperature might affect the final biochar porosity and its surface chemistry.
Increasing the pyrolysis temperature will cause the removal of the volatile matter from the biomass matrix, thereby, causing the formation of voids, cracks, or other surface features on the biochar. It is likely that the formation of voids and cracks does not significantly contribute to the removal of MB from the polluted water. On the other hand, the high % R of MB obtained with biochar samples produced at low level of pyrolysis temperature suggests that adsorption was enhanced due to biochar surface modification. The release of functional groups, such as hydroxyl, carboxyl, or amino groups as a result of low temperature pyrolysis influenced the adsorption characteristics of the biochar. These functional groups can play a role in adsorption by providing adsorption sites for MB or promoting surface reactions. The same promotion effect can be envisaged for the pyrolysis time.
ANOVA is used to test the main effects of each process variable and the interaction effects between the process variables. Results of the ANOVA are given in .
Table 5. Results of the ANOVA (analysis of variance) for process variables.
The ANOVA results indicate that the calculated p-value for the process variables (temperature and time) is less than the alpha level (0.05), which means that the effect of both temperature and time is statistically significant. On the other hand, the effect of heating rate and the binary interaction effects are statistically significant for alpha level (0.2), which means they are estimated at 80% confidence interval for the estimated effect.
Adsorption isotherm of MB
Adsorption isotherm for MB adsorption on biochar produced at the settings of the center-point level is obtained. Langmuir and Freundlich adsorption isotherm models were implemented for analyzing the adsorption behavior and get insights into the adsorption processes. ().
Figure 3. Adsorption isotherms of methylene blue (MB) on Biochar Obtained at pyrolysis conditions: heating rate of 20°C/min, pyrolysis temperature of 450°C, and holding time of 60 min.
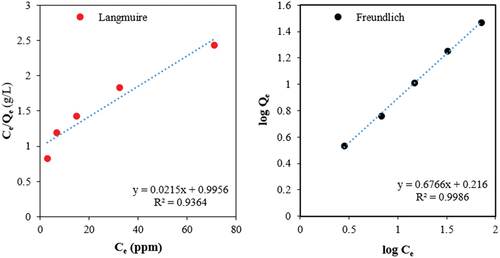
The model parameters for the adsorption isotherms are given in .
Table 6. Model parameters of the adsorption isotherms for methylene blue (MB) adsorption on biochar.
The Freundlich adsorption isotherm model perfectly fitted the adsorption experimental data, compared to the Langmuir adsorption isotherm model. The adsorption process is likely to be heterogeneous, with a distribution of adsorption energies on the surface of the biochar. The adsorption capacity of the biochar is considered high, as indicated by the high value of the Freundlich constant (KF = 1.64). The adsorption intensity is high since the Freundlich exponent (n = 1.48) is greater than 1, indicating that the adsorption process is favorable.
Performance of activated biochar
Adsorption isotherm for MB adsorption on activated biochar samples (ABC-1 and ABC-2) are obtained. The Freundlich adsorption isotherms were used to model the adsorption behavior ().
Figure 4. Adsorption isotherms of methylene blue (MB) on Activated Biochar Samples: ABC-1 (Biochar Activated with organic 1) and ABC-2 (Biochar Activated with organic 2).
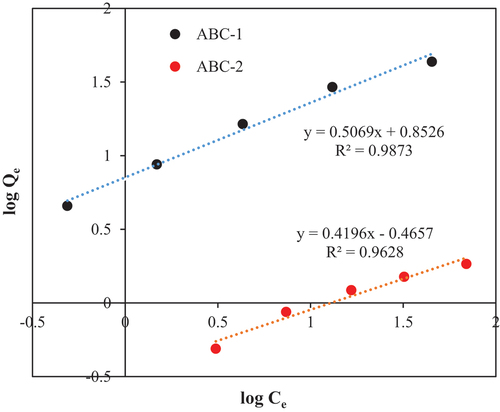
The Freundlich adsorption isotherm model perfectly fitted the adsorption experimental data for both ABC-1 and ABC-2. The high value of the Freundlich constant (KF = 7.12) indicates increased adsorption capability of ABC-1 compared to biochar without activation. The Freundlich exponent (n = 1.97) is larger than 1, suggesting that the adsorption process is favorable, and the adsorption intensity is high.
It is clear that biochar activation via the bio-oil (organic-1) substantially enhanced the biochar adsorption capacity. It is worth mentioning that organic-1 comprised mainly lignin (Aljeradat et al., Citation2022). Lignin is a complex polymer composed of several functional groups, including aromatic hydroxyl groups, methoxy groups, carbonyl groups, carboxyl groups, and ether linkages (Brunow & Lundquist, Citation2010). Modification of the surface of biochar by introducing functional groups obtained from the lignin could enhance the adsorption of MB. Functional groups on the surface of adsorbent can form hydrogen bonds, ionic interactions, or covalent bonds with adsorbates, leading to their retention (Punyapalakul et al., Citation2013; Suriyanon et al., Citation2013; Tong et al., Citation2019). Adsorption at pH = 10, means that the solution is basic, and the hydroxyl group can become deprotonated and carry a negative charge. On the other hand, the carboxyl groups are acidic and can be deprotonated at high pH. In this case, they carry a net negative charge. MB is a cationic dye, meaning that it has a positive charge. Because of its positive charge, MB can engage electrostatically with the negatively charged functional groups on the surface of biochar.
Biochar activated with Organic 2 (ABC-2) exhibited low adsorption capacity (KF = 0.34). It is worth mentioning that organic-2 comprised mainly furans, phenolics, alcohols, and ketones (Aljeradat et al., Citation2022). These chemical compounds adversely affected the surface and morphology of biochar. It is possible that these compounds formed a layer, which could block the adsorption sites and reduce the available surface area for adsorption. Another possible explanation that these compounds caused the degradation of the adsorbent surface.
Performance of different adsorbents in the removal of MB from polluted water
The performance of the date kernel-derived biochar with and without activation in the removal of MB from polluted water was compared with the performance of other adsorbents ().
Table 7. Performance of different adsorbents in the removal of MB from polluted water.
While the performance of activated biochar (ABC-1) was generally high compared to the other adsorbents, there were some systems that exhibited even higher values. The advantages of ABC-1 as adsorbent for MB removal from polluted water include its ease of preparation and its ability to contribute to efficient utilization of resources and effective management of waste.
Biochar derived from date kernels offers a variety of applications as well as prospective future research direction. It may be used to treat water and wastewater. According to the current investigation, biochar produced from date kernels has a good adsorption capacity for MB from polluted water. Other potential practical applications of the produced biochar include soil fertility enhancement and renewable energy production. It may be burnt to generate both heat and power. It has the potential to be a long-term carbon-sequestration technique, particularly in arid and semi-arid areas.
Future research areas for date kernel biochar include more extensive characterization of date kernel biochar’s physicochemical properties, which can aid in optimizing its use in various applications. Temperature, residence time, and heating rate during biochar activation can all have an impact on the characteristics of activated biochar. These characteristics can be optimized to produce biochar with superior qualities for specific purposes.
Conclusions
The study involved the production of biochar from Iraqi Khestawi date kernels through pyrolysis under various operational parameters. Utilizing a full factorial design methodology, the influence of heating rate, pyrolysis temperature, and holding time on the biochar’s efficacy in removing MB from contaminated water was systematically explored.
The findings of this study revealed that pyrolysis temperature and holding time had substantial effects on the biochar’s performance, resulting in notable MB removal percentages of 48.9% and 30.9% at pyrolysis temperatures of 350°C and 550°C, respectively. Conversely, the impact of heating rate, was relatively weak.
Furthermore, the application of Freundlich adsorption isotherm model excellently matched the experimental data. Notably, the biochar exhibited a high adsorption capacity, as evidenced by the elevated Freundlich constant (KF = 1.64), indicating favorable adsorption intensity and a beneficial adsorption process.
Moreover, the activation of biochar through bio-oil (organic-1) significantly enhanced its adsorption capacity, as reflected in the substantially increased Freundlich constant (KF = 7.12) for ABC-1 compared to non-activated biochar. This underscores the favorable and high-intensity adsorption of MB on ABC-1.
This study provides valuable insights into the potential of date kernel-derived biochar for effective MB removal from polluted water, with a focus on the critical influence of process parameters. These findings contribute to the broader understanding of biochar as some eco-friendly adsorbent and open avenues for further research in environmental remediation applications.
Declaration of generative AI and AI-assisted technologies in the writing process
During the preparation of this work, the authors used AI-tools in order to improve the language and readability of the manuscript. After using these tools, the authors reviewed and edited the content as needed and took full responsibility for the content of the publication.
Disclosure statement
No potential conflict of interest was reported by the author(s).
References
- Abid, W., & Ammar, E. (2022). Date palm (Phoenix dactylifera L.) wastes valorization: A circular economy approach. In M. F. Ramadan & M. A. Farag (Eds.), Mediterranean fruits bio-wastes: Chemistry, functionality and technological applications (pp. 403–430). Springer.
- Aliedeh, M. A., Aljbour, S. H., Al-Harahsheh, A. M., Al-Zboon, K., & Al-Harahsheh, S. (2021). Implementing 24-1 fractional factorial design for filling the gap in OVAT sorption studies of nitrate ions onto Jordanian Zeolitic Tuff. Journal of Chemical Technology and Metallurgy, 56(2), 331–341.
- Aljbour, S. H., Al-Harahsheh, A. M., Aliedeh, M. A., Al-Zboon, K., & Al-Harahsheh, S. (2017). Phosphate removal from aqueous solutions by using natural Jordanian zeolitic tuff. Adsorption Science & Technology, 35(3–4), 284–299. https://doi.org/10.1177/0263617416675176
- Aljeradat, R. A., Aljbour, S. H., & Jarrah, N. A. (2021). Natural minerals as potential catalysts for the pyrolysis of date kernels: Effect of catalysts on products yield and bio-oil quality. Energy Sources, Part A: Recovery, Utilization, Environmental Effects, 1–9. https://doi.org/10.1080/15567036.2021.2003485
- Aljeradat, R. A., Aljbour, S. H., & Jarrah, N. A. (2022). Pyrolysis of date kernels using natural Jordanian Tripoli as a catalyst under different operational conditions. Case Studies in Chemical and Environmental Engineering, 6, 100212. https://doi.org/10.1016/j.cscee.2022.100212
- Aljeradat, R. A., Aljbour, S. H., & Jarrah, N. A. (2023). Performance of chemically modified Tripoli in catalytic pyrolysis of date kernels. Case Studies in Chemical and Environmental Engineering, 7, 100319. https://doi.org/10.1016/j.cscee.2023.100319
- Al-Wabel, M. I., Usman, A. R. A., Al-Farraj, A. S., Ok, Y. S., Abduljabbar, A., Al-Faraj, A. I., & Sallam, A. S. (2019). Date palm waste biochars alter a soil respiration, microbial biomass carbon, and heavy metal mobility in contaminated mined soil. Environmental Geochemistry and Health, 41(4), 1705–1722. https://doi.org/10.1007/s10653-017-9955-0
- ASTM D3172. (2013). Standard practice for proximate analysis of coal and Coke (pp. D3172–13). ASTM International.
- ASTM D3176. (2015). Standard practice for ultimate analysis of coal and Coke (pp. D3176–15). ASTM International.
- Attia, A. I., Reda, F. M., Patra, A. K., Elnesr, S. S., Attia, Y. A., & Alagawany, M. (2021). Date (Phoenix dactylifera L.) by-products: Chemical composition, nutritive value and applications in poultry nutrition, an updating review. Animals, 11(4), 1133. https://doi.org/10.3390/ani11041133
- Belhachemi, M., & Addoun, F. (2011). Comparative adsorption isotherms and modeling of methylene blue onto activated carbons. Applied Water Science, 1(3–4), 111–117. https://doi.org/10.1007/s13201-011-0014-1
- Bhattacharyya, K. G., & Sharma, A. (2005). Kinetics and thermodynamics of methylene blue adsorption on neem (azadirachta indica) leaf powder. Dyes and Pigments, 65(1), 51–59. https://doi.org/10.1016/j.dyepig.2004.06.016
- Box, G. E., Hunter, J. S., & Hunter, W. G. (2005). Statistics for experimenters. Wiley.
- Brunow, G., & Lundquist, K. (2010). Functional groups and bonding patterns in lignin (including the lignin-carbohydrate complexes). In C. Heitner, D. Dimmel, & J. Schmidt (Eds.), Lignin and lignans, lignin (pp. 268–291). New York: CRC Press, Taylor Francis Group.
- Bulut, Y., & Aydın, H. (2006). A kinetics and thermodynamics study of methylene blue adsorption on wheat shells. Desalination, 194(1–3), 259–267. https://doi.org/10.1016/j.desal.2005.10.032
- Cha, J. S., Park, S. H., Jung, S.-C., Ryu, C., Jeon, J.-K., Shin, M.-C., & Park, Y.-K. (2016). Production and utilization of biochar: A review. Journal of Industrial and Engineering Chemistry, 40, 1–15. https://doi.org/10.1016/j.jiec.2016.06.002
- CSO. (2020). Palm date production statistics. Iraqi Central Statistical Organization.
- El Hadrami, A., & Al-Khayri, J. M. (2012). Socioeconomic and traditional importance of date palm. Emirates Journal of Food and Agriculture, 24(5), 371–385.
- Erskine, W., Moustafa, A. T., Osman, A. E., Lashine, Z., Nejatian, A., Badawi, T., & Ragy, S. M. (2004). Date palm in the GCC countries of the Arabian Peninsula. Proceedings of Regional Workshop on Date Palm Development in the Arabian Peninsula (pp. 29–31). Abu Dhabi, UAE.
- Foong, S. Y., Liew, R. K., Yang, Y., Cheng, Y. W., Yek, P. N. Y., Mahari, W. A. W., Lee, X. Y., Han, C. S., Vo, D.-V. N., Van Le, Q., Aghbashlo, M., Tabatabaei, M., Sonne, C., Peng, W., & Lam, S. S. (2020). Valorization of biomass waste to engineered activated biochar by microwave pyrolysis: Progress, challenges, and future directions. Chemical Engineering Journal, 389, 124401. https://doi.org/10.1016/j.cej.2020.124401
- Franca, A. S., Oliveira, L. S., & Ferreira, M. E. (2009). Kinetics and equilibrium studies of methylene blue adsorption by spent coffee grounds. Desalination, 249(1), 267–272. https://doi.org/10.1016/j.desal.2008.11.017
- Ghosh, D., & Bhattacharyya, K. G. (2002). Adsorption of methylene blue on kaolinite. Applied Clay Science, 20(6), 295–300. https://doi.org/10.1016/S0169-1317(01)00081-3
- Jahan, F., & Happy, A. A. (2022). Revolutionizing plant-based extracts for skin care and therapeutics. In S. H. M. Setapar, A. Ahmad, & M. Jawaid (Eds.), Nanotechnology for the preparation of cosmetics using plant-based extracts (pp. 75–130). Elsevier.
- Okeola, F. O., & Odebunmi, E. O. (2010). Freundlich and Langmuir isotherms parameters for adsorption of methylene blue by activated carbon derived from agrowastes. Advances in Natural Applied Sciences, 4(3), 281–289.
- Öktem, Y. A., Soylu, S. G. P., & Aytan, N. (2012). The adsorption of methylene blue from aqueous solution by using waste potato peels; equilibrium and kinetic studies. Journal of Scientific & Industrial Research, 71(12), 817–821. http://nopr.niscpr.res.in/handle/123456789/15131
- Punyapalakul, P., Suksomboon, K., Prarat, P., & Khaodhiar, S. (2013). Effects of surface functional groups and porous structures on adsorption and recovery of perfluorinated compounds by inorganic porous silicas. Separation Science and Technology, 48(5), 775–788. https://doi.org/10.1080/01496395.2012.710888
- Sakhiya, A. K., Anand, A., & Kaushal, P. (2020). Production, activation, and applications of biochar in recent times. Biochar, 2(3), 253–285. https://doi.org/10.1007/s42773-020-00047-1
- Sarioglu, M., & Atay, U. (2006). Removal of methylene blue by using biosolid. Global NEST Journal, 8(2), 113–120. https://doi.org/10.30955/gnj.000351
- Suriyanon, N., Punyapalakul, P., & Ngamcharussrivichai, C. (2013). Mechanistic study of diclofenac and carbamazepine adsorption on functionalized silica-based porous materials. Chemical Engineering Journal, 214, 208–218. https://doi.org/10.1016/j.cej.2012.10.052
- Tahir, A. H., Al-Obaidy, A. H. M., & Mohammed, F. H. (2020). Biochar from date palm waste, production, characteristics and use in the treatment of pollutants: A review. In IOP Conference Series: Materials Science and Engineering. 4th International Conference on Buildings, Construction and Environmental Engineering, Istanbul, Turkey, October 7–9, 2019 (Vol. 737, p. 0121171). IOP Publishing. https://doi.org/10.1088/1757-899X/737/1/012171
- Tong, Y., Mcnamara, P. J., & Mayer, B. K. (2019). Adsorption of organic micropollutants onto biochar: A review of relevant kinetics, mechanisms and equilibrium. Environmental Science: Water Research & Technology, 5(5), 821–838. https://doi.org/10.1039/C8EW00938D
- Younas, A., Naqvi, S. A., Khan, M. R., Shabbir, M. A., Jatoi, M. A., Anwar, F., Inam‐Ur‐Raheem, M., Saari, N., & Aadil, R. M. (2020). Functional food and nutra-pharmaceutical perspectives of date (Phoenix dactylifera L.) fruit. Journal of Food Biochemistry, 44(9), e13332. https://doi.org/10.1111/jfbc.13332