ABSTRACT
Air pollution in cities disproportionately affects children and those living in economically-deprived areas near busy roadways. Walls are effective in deflecting particulate matter but the addition of shaping either at the design stage, or as retrofit, improves performance. High-wall baffles reduce distal vortex accumulations; On pavements, low-level baffles can deflect suspended particulates back towards the road surface. These shaped structures can scaffold urban plantings and, in tandem, improve the effectiveness of urban green in this context. Shaped baffles are immediately effective, inexpensive and create a win-win that engages stakeholders. This awareness will drive collaborations between planners, designers and modellers for effective and beautiful street furniture elements that reduce pollution exposure.
Introduction
Air pollution in cities is a globally important health issue and is especially pronounced for vulnerable populations, such as children attending schools close to major roads or pedestrians near road junctions where waiting-time constitutes a significant exposure hazard (Woodward et al. Citation2019). The health consequences of vehicle-generated air pollution are serious and fall more grievously on lower-income urban areas as these are more likely to be within attenuation distance of busy roads (Wheeler and Ben-Shlomo Citation2005). This contributes to health and social inequities, the risks of which are borne disproportionately both by those most physiologically vulnerable: children (Künzli et al. Citation2000, Jerrett et al. Citation2014), and those in the most economically vulnerable social classes who are frequently minority ethnic (Mitchell and Dorling Citation2003, Tonne et al. Citation2018).
Substantial effort is directed at long-term source reduction of vehicle emissions and there is the promise of widespread progress with exhaust sources (Amato et al. Citation2014). Other air-borne particulates such as brake dust and tyre wear remnants will remain problematic over a much longer time-frame and at increasing levels due to the greater weight of electric cars (Timmers and Achten Citation2016). Deposited road dusts are additionally prone to local re-suspension and, due to their larger size fraction, disperse less effectively than gaseous pollutants exacerbating local exposures (Thorpe and Harrison Citation2008). This ongoing challenge means that we cannot rest on the laurels of reductions in exhaust-sourced pollution, but must continue to find solutions to reduce the risks and impacts of particulate matters inhalation by pedestrians and residents of urban environments.
High walls along roads can reduce the spread of particulates substantially, but, in real-world conditions, vortices can create areas of high concentration behind these (Gallagher et al. Citation2015). Open spaces between such barrier walls and set-back structures, including schools, homes and transport infrastructure such as stations and bus stops, become areas of elevated toxicity and exposure (Lim et al. Citation2015, Holgate Citation2017). Pavements too are areas of particular vulnerability, additionally so when street-tree canopies constrain air-mixing (Salmond et al. Citation2013). Here again, children are the most vulnerable to inhalation risk of heavier fractions of particulate matter both when walking or when in push-chairs. Low boundary walls have demonstrated substantial efficacity (40–70% abatement) and optimising the design and use of these is a subject of great interest (McNabola et al. Citation2007, Gallagher et al. Citation2015). The analogous green infrastructure, hedges, have also received attention and offer some attenuation benefits as well as other ecosystem services that such ‘urban green’ can deliver (Varshney and Mitra Citation1993, Blanusa et al. Citation2019).
Field evaluations of extant infrastructure and modelling studies have contributed substantially to developing ‘field-testable’ barrier designs (McNabola et al. Citation2009, Hagler et al. Citation2011, Jeanjean et al. Citation2017, Abhijith and Kumar Citation2019). These latter have largely focussed on simple geometrical shapes and mimics of the typical canopy and vegetation structures. Here we extend this modelling to explore whether deliberate shaping of infrastructure can contribute to additional mitigation by diverting a greater fraction of the particulates away from people. Shaped elements at street-level might then be designed to effectively manipulate airflows so that particulate emissions from low-level proximate sources such as cars are deflected away from pedestrian areas. In their simplest form, appropriately angled and placed baffles may have the potential to instantly reduce exposure and consequent ill-health outcomes whilst being rapidly retrofittable to existing walls. Similar baffle structures have been demonstrated as effective in the horizontal plane around airports (Bennett et al. Citation2013) and topological shaping has been recommended to attenuate sound (Dühring et al. Citation2008).
Here, we use air flow modelling to evaluate and quantify the potential of shaped, retrofit baffles to immediately attenuate levels of air pollution at an idealised roadside site. Whilst the modelling conditions are undoubtedly simplifications of any real-world scenario, this serves as a ‘proof of principle’ and allows us to do more than speculate over the potential effectiveness of such ‘shaping’.
Methods
We used the open-source computational fluid dynamics (CFD) software, Fluidity (https://fluidityproject.github.io/) to simulate the dispersion of traffic emissions. Fluidity uses the large eddy simulation (LES) method to resolve the larger turbulent features of the air flow while smaller scale eddies are modelled as additional viscosity using a Smagorinsky-type model (Bentham Citation2003). This method captures the highly dynamic and unsteady nature of the air flow, including mixing with the urban boundary layers above building height. The traffic emissions are modelled as passive tracers. Details of the equations solved can be found in previous examples of the application of Fluidity to simulate the dispersion of emissions in urban environments (e.g. Aristodemou et al. Citation2009, Citation2018, Woodward et al. Citation2019).
The dispersion of traffic emissions within six matched canyons aligned perpendicular to the wind direction was simulated. The dimensions and configuration of two of the canyons are shown in . Periodic (cyclical) boundary conditions are applied at the inlet and outlet of the domain. A forcing term is used to drive the flow in the x direction and a fully turbulent flow is allowed to develop and reach a statistical steady state before the traffic emissions are considered. As is frequent in exploratory studies, the emissions are modelled as constant volume sources with equal magnitude in each canyon and concentrations are analysed after 30 minutes.
Figure 1. Schematic of the ‘typical’ street canyon configuration specified for the initial modelling trials with the example of leeward wall (left) and leeward wall and baffle (right)
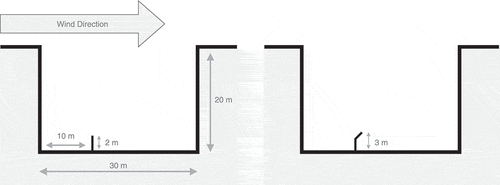
In addition to the ‘control’, no wall, situation, four different structure configurations are considered; windward wall, windward wall with baffle, leeward wall and leeward wall with baffle. In each case, we are concerned with the concentrations on the same and opposing side of the road as the obstacle. Due to the symmetry of the canyon, by considering both windward and leeward configurations we are simulating the impact of the structures at two opposite wind directions, both perpendicular to the canyon. The control canyons contain no barriers, providing a comparison in the absence of any passive mitigation. Both walls and baffles are modelled as solid boundaries.
Results
Leeward side barriers
displays a cross section of the time-averaged wind flow vertices on a plane cut across the centre of the canyon with a prevailing wind direction from left to right. In each case, a classic street canyon vortex is formed within the canyon. This vortex leads to the dispersion of traffic emissions to the leeward side of the canyon. With the presence of a barrier wall as in and (c), the vortex is forced upward over the barrier, carrying the traffic emissions over and away from the roadside. The addition of the baffle onto the wall enhances this effect. A second vortex is seen near the ground on the leeward side for each case. This leeward vortex is much larger in the presence of any barrier and entrains a portion of the traffic emissions into the pedestrian area from the flow passing over and above the barrier. In the case with a baffle, the vortex area is larger leading to a greater volume of air into which the emissions are entrained and diluted. A much stronger vortex on the traffic side of the barrier is seen for the wall with baffle case (). This vortex serves as a recirculation region for the traffic emissions.
Figure 2. Relative pollutant concentrations in the modelled canyon street with (a) no barrier wall, (b) a leeward roadside wall, (c) a leeward roadside wall with baffle. Pollutant levels are time-averaged, normalised concentrations; the white fine-scale arrows indicate wind flow vectors at y = 0 m within the street canyon; see for streetscape geometry
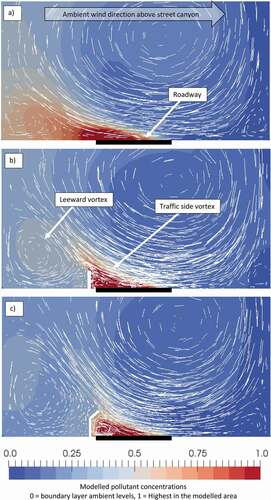
These effects are reflected in the concentrations seen at the roadside. A clear reduction in concentrations is measured behind the barriers and relative to the no wall case. Lower concentrations are seen with a baffle ()) than with a wall only ()). A spatial average of the concentrations was calculated from a 5 m × 20 m × 2 m volume on either side of the road. These concentrations are shown in for the no barrier case, while the reduction relative to the base case is given for each other case.
Table 1. Spatially averaged concentrations in the pedestrian space at roadside in the no barrier case and relative percentage change for each barrier and barrier + baffle combination
Windward side barriers
As with the Leeward side barriers, the presence of a windward side barrier influences the airflow in the canyon. Substantially lower concentrations are seen on the leeward side of the canyon in the presence of a windward side barrier (). This is due to the deflection of the main vortex upwards, such that it does not flow directly over the road, along with the formation of a vortex on the traffic side of the wall. This allows the traffic emissions to recirculate in situ rather than disperse to the roadside. In the case of a wall with a baffle, two traffic side vortices are seen, and a greater upward deflection of the main vortex, leading to greater reductions in concentrations on the leeward roadside. Concentrations on the windward side of the road remain low in each case, although a reduction is seen for the wall with baffle case (−22%, ).
Figure 3. Relative pollutant concentrations in the modelled canyon street with (a) no barrier wall, (b) a windward roadside wall, (c) a windward roadside wall with baffle. Pollutant levels are time-averaged, normalised concentrations; the white fine-scale arrows indicate wind flow vectors at y = 0 m within the street canyon; see for streetscape geometry
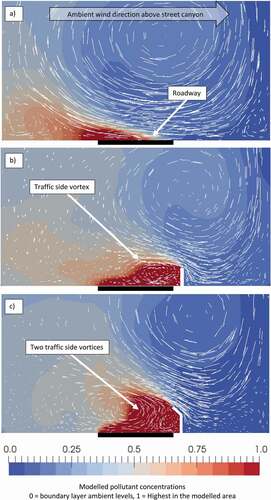
Discussion
This initial exploration of the influence of specific shaping of barriers on particulate matter pollution behaviour in pedestrian-relevant space indicates substantial promise and opportunity. The effects of vertical walls on particulate behaviour modelled here are in-line with other studies (Hagler et al. Citation2011, Gromke et al. Citation2016). However, others (McNabola et al. Citation2009) using similar CFD studies found that the addition of short barriers (0.5 m in this case) can lead to greater pedestrian exposure when the wind is perpendicular. It is clear that more work is required to fully understand the effects of roadside barriers on ambient concentrations, but there is sufficient evidence to suggest that airflows, and their air-borne pollutants can be more effectively managed by adding shaping. This observation and demonstration opens the doors to further studies that may work towards optimising the shapes for maximum impact in applied contexts. Such work will also contribute to managing trade-offs: if the particulates are not dispersing into pedestrian areas, they may concentrate in the roadways. People in vehicles do, though, have greater capacity than adjacent pedestrians to reduce their exposure and mostly benefit from air filtration and the option to close their windows.
One important opportunity created by shaping is the inclusion of shaping frames within urban greening. Walls are more impermeable to pollutants than vegetation barriers, though a combination of barrier and hedge provides even greater efficacity (Tong et al. Citation2016). Thick hedges, either evergreen (e.g. Ilex, Ligustrum or Photinia spp.) or of marcescent deciduous species (e.g. Fagus, Carpinus or Quercus spp.) which, when hedge-trimmed, retain their foliage until subsequent leaves emerge in spring, can already be semi-effective barriers and confer a substantial suite of further ecosystem services to the urban ‘grey’ (Blanusa et al. Citation2019). Combining a shaped barrier with entwined green ( and (b)) may deliver the best attenuation of all passive methods. Sturdy, evergreen climbing plants such as ivies (Hedera spp.), which offer biodiversity services (shelter and food to many birds and insects) can be grown over and through perforated, explicitly shaped frames at road sides. Future modelling accompanied by validation studies will allow clear advice to practitioners on the location and shaping of scaffolded green elements designed to meet specific purposes. For the air quality considered here, using the lower hybrid scheme ()) in breaking and accelerating zones near junctions could contribute to reduced particulate exposure and road safety to pedestrians as well as some wider ecosystem services.
Figure 4. Shaping of low boundary walls (left) and in baffles retrofit to existing infrastructure (right) can substantially reduce particulate matter dispersion into pedestrian areas. Figure preparation by @AzizaIllustrates
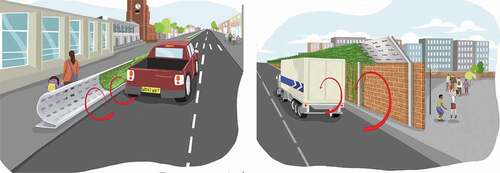
Perforated frames and baffles have other benefits – the perforations reduce weight and prevent water retention and, while we modelled a solid baffle, there is evidence that up to 20% barrier porosity continues to be effective in pollution management (Shan et al. Citation2007). Weight reduction and permeability to water also contribute to ease of retrofit where existing walls may have limited structural capacity for additional ‘sail’ or mass. Children using many of the schools and playgrounds that abut the arterial flows of urban areas could be rapidly further protected from particulate pollution by the addition of deflection baffles ().
The dispersion of traffic emissions in urban areas is a highly complex process that depends on many factors including street and building topology and weather conditions. Here we considered only an idealized street canyon; the added complexity of real urban topology would also affect dispersion. Further, local weather conditions vary daily and seasonally. Our simplified representation is of wind directions perpendicular to the street canyon, in other situations, the oblique wind is likely to form a helical vortex down the length of the canyon and baffle shaping may, or may not, be more effective. We have also not considered buoyancy-driven convective flow and assumed a neutral atmospheric boundary layer. The growing success of many cities in reducing exhaust emissions refocuses the vehicle challenge to particulate fractions from brakes, tyres and other road dusts (Luhana et al. Citation2004, Thorpe and Harrison Citation2008) and here this shaping may have much to contribute. The configuration of the urban streetscape will change much in years to come and by using models to estimate specific shapes and contexts, we may be able to help practitioners address the elements of poor air quality that are predicted to remain.
The effectiveness of baffles has an additional benefit in another dimension. Not only may pollution be attenuated for specific and sensitive audiences, but public confidence is enhanced by seeing responsive and immediate actions taken by partner agencies. From such obvious actions, wide and positive stakeholder confidence will grow.
Reducing unnecessary traffic and managing flows in cities needs a whole systems approach and will take time to be delivered. More rapidly, a collaboration between urban planners, air flow modellers, designers and planting experts could lead to rapid delivery of street furniture and shaped greenery that acted to divert particulates from people and thus contributed to population health and social equity in urban areas. Shape matters to their efficacity and beauty.
Acknowledgments
We wish to thank Helen Ap-Simon and Audrey de Nazelle for their support and encouragement; Rosalind O’Driscoll of APRIL (Air Pollution Research In London) for the opportunity to present and get feedback on this work; Peter Childs of the Dyson School of Design Engineering at Imperial College London for a very helpful conversation and Derek Wilson of TFL (Transport for London) for an opportunity to test these ideas. We also wish to thank the anonymous reviewers who have helped us to improve the work.
Disclosure statement
No potential conflict of interest was reported by the authors.
Additional information
Funding
Notes on contributors
C Matilda Collins
Dr C M (Tilly) Collins is an arborist, ecologist and entomologist with a great interest in urban greenspace. She is a Senior Fellow of the Centre for Environmental Policy at Imperial College London where she leads a number of projects. Tilly has a passion for trees and insects leading to diverse research interests, most of which have a common theme of using well-gathered data to support sustainable decision-making. She likes to chat and make links between people and concepts.
Agamemnon Otero
Agamemnon Otero MBE is a founding director of Repowering, Energy Garden and Brixton Energy. He co-founded industry advocacy body Community Energy England and sat on the Business, Energy and Industrial Strategy Office’s Community Energy Contact Group. He has a M.Sc Architecture: Advanced Environment and Energy and previously worked as the director for Renewable Energy Project Finance and Social Responsibility at a financial institution. In 2014 was made a London Leader, in 2016 he was awarded an MBE for services to community energy and in 2017 was named one of the 51 Most Impactful Green Leaders globally by the CSR Congress.
Huw Woodward
Dr Huw Woodward is a Research Associate at the Centre for Environmental Policy at Imperial College London. His research focuses on pollution dispersion modelling. This includes both national scale modelling using the UK Integrated Assessment Model (UKIAM) to estimate future projections of UK air quality and ecosystem health, and also microscale dispersion modelling, using computational fluid dynamics to investigate the effect of traffic induced turbulence, trees and roof geometry on the dispersion of pollutants within urban areas.
References
- Abhijith, K.V. and Kumar, P., 2019. Field investigations for evaluating green infrastructure effects on air quality in open-road conditions. Atmospheric environment, 201 (December 2018), 132–147. Elsevier. doi:10.1016/j.atmosenv.2018.12.036
- Amato, F., et al., 2014. Urban air quality: the challenge of traffic non-exhaust emissions. Journal of hazardous materials, 275, 31–36. doi:10.1016/j.jhazmat.2014.04.053
- Aristodemou, E., et al., 2009. A comparison of mesh-adaptive LES with wind tunnel data for flow past buildings: mean flows and velocity fluctuations. Atmospheric environment, 6238–6253. doi:10.1016/j.atmosenv.2009.07.014
- Aristodemou, E., et al., 2018. How tall buildings affect turbulent air flows and dispersion of pollution within a neighbourhood. Environmental pollution, 233, 782–796. doi:10.1016/j.envpol.2017.10.041
- Bennett, M., et al., 2013. Abatement of an aircraft exhaust plume using aerodynamic baffles. Environmental science & technology, 47, 2346–2352. doi:10.1021/es303586x
- Bentham, J.H.T., 2003. Microscale modelling of air flow and pollutant dispersion in the urban environment. London: Imperial College.
- Blanusa, T., et al., 2019. Urban hedges: A review of plant species and cultivars for ecosystem service delivery in north-west Europe. Urban forestry and urban greening, 44, 126391. doi:10.1016/j.ufug.2019.126391
- Dühring, M.B., Jensen, J.S., and Sigmund, O., 2008. Acoustic design by topology optimization. Journal of sound and vibration, 317, 557–575. doi:10.1016/j.jsv.2008.03.042
- Gallagher, J., et al., 2015. Passive methods for improving air quality in the built environment: A review of porous and solid barriers. Atmospheric environment, 120, 61–70. doi:10.1016/j.atmosenv.2015.08.075
- Gromke, C., Jamarkattel, N., and Ruck, B., 2016. Influence of roadside hedgerows on air quality in urban street canyons. Atmospheric environment, 139, 75–86. doi:10.1016/j.atmosenv.2016.05.014
- Hagler, G.S.W., et al., 2011. Model evaluation of roadside barrier impact on near-road air pollution. Atmospheric environment, 45, 2522–2530. doi:10.1016/j.atmosenv.2011.02.030
- Holgate, S.T., 2017. Every breath we take: the lifelong impact of air pollution’ - A call for action. Clinical medicine, journal of the royal college of physicians of London. doi:10.7861/clinmedicine.17-1-8
- Jeanjean, A.P.R., et al., 2017. Ranking current and prospective NO2 pollution mitigation strategies: an environmental and economic modelling investigation in Oxford Street, London. Environmental Pollution, 225, 587–597. doi:10.1016/j.envpol.2017.03.027
- Jerrett, M., et al., 2014. Traffic-related air pollution and obesity formation in children: A longitudinal, multilevel analysis. Environmental health: A global access science source, 13. doi:10.1186/1476-069X-13-49
- Künzli, N., et al., 2000. Public-health impact of outdoor and traffic-related air pollution: A European assessment. Lancet, 356, 795–801. doi:10.1016/S0140-6736(00)02653-2
- Lim, S., et al., 2015. Determinants of spikes in ultrafine particle concentration whilst commuting by bus. Atmospheric environment, 112, 1–8. doi:10.1016/j.atmosenv.2015.04.025
- Luhana, L., et al., 2004. Characterisation of exhaust particulate emissions from road vehicles - Measurement of non-exhaust particulate matter, Fifth framework programme competitive and sustainable growth sustainable mobility and intermodality. Bruxelles, Belgium: The European Commission.
- McNabola, A., Broderick, B.M., and Gill, L.W., 2007. Reduced exposure to air pollution on the boardwalk in Dublin, Ireland. Measurement and prediction. Environment international, 33 (9), 86–93. doi:10.1016/j.envint.2007.07.006
- McNabola, A., Broderick, B.M., and Gill, L.W., 2009. A numerical investigation of the impact of low boundary walls on pedestrian exposure to air pollutants in urban street canyons. Science of the total environment, 407, 760–769. doi:10.1016/j.scitotenv.2008.09.036
- Mitchell, G. and Dorling, D., 2003. An environmental justice analysis of British air quality. Environment & planning A, 35, 909–929. doi:10.1068/a35240
- Salmond, J.A., et al., 2013. The influence of vegetation on the horizontal and vertical distribution of pollutants in a street canyon. Science of the total environment, 443, 287–298. doi:10.1016/j.scitotenv.2012.10.101
- Shan, Y., et al., 2007. Effects of vegetation status in urban green spaces on particle removal in a street canyon atmosphere. Acta Ecologica Sinica, 27 (11), 4590–4595. doi:10.1016/S1872-2032(08)60007-4
- Thorpe, A. and Harrison, R.M., 2008. Sources and properties of non-exhaust particulate matter from road traffic: A review. Science of the total environment, 400, 270–282. doi:10.1016/j.scitotenv.2008.06.007
- Timmers, V.R.J.H. and Achten, P.A.J., 2016. Non-exhaust PM emissions from electric vehicles. Atmospheric environment. doi:10.1016/j.atmosenv.2016.03.017
- Tong, Z., et al., 2016. Roadside vegetation barrier designs to mitigate near-road air pollution impacts. Science of the total environment, 541 (15), 920–927. doi:10.1016/j.scitotenv.2015.09.067
- Tonne, C., et al., 2018. Socioeconomic and ethnic inequalities in exposure to air and noise pollution in London. Environment international, 115, 170–179. doi:10.1016/j.envint.2018.03.023
- Varshney, C.K. and Mitra, I., 1993. Importance of hedges in improving urban air quality. Landscape and urban planning, 25, 85–93. doi:10.1016/0169-2046(93)90124-V
- Wheeler, B.W. and Ben-Shlomo, Y., 2005. Environmental equity, air quality, socioeconomic status, and respiratory health: A linkage analysis of routine data from the health survey for England. Journal of epidemiology and community health, 59, 948–954. doi:10.1136/jech.2005.036418
- Woodward, H., et al., 2019. A large eddy simulation of the dispersion of traffic emissions by moving vehicles at an intersection. Atmospheric environment, 215, 116891. doi:10.1016/j.atmosenv.2019.116891