ABSTRACT
The genus Lachemilla in Ecuador consists of 27 species distributed along the páramos and superpáramos of the Andes. With 11 species, it is one of the most species-rich genera in the superpáramo zone. We report the presence of apomixis in Lachemilla hirta and L. hispidula. Both species were regularly visited by entomofauna, where Diptera were the most representative group. Three treatments (enclosing the inflorescences with mesh bags, wax paper bags and the open-pollinated control) were used to determine the presence of pollination, apomixis and both processes respectively. In the fruiting assays with the mesh bags, L. hirta showed no significant differences when compared to the control treatments; whereas highly significant differences between treatments were found in L. hispidula, where the control treatment was the treatment with more fruit production, which according to the apomixis results, could mean and accidental pollination activity from the insects found in this study. The flow cytometric screen of seeds from all three treatments showed that these species are predominantly autonomous apomicts. Additionally, pseudogamous seeds in L. hirta, and triploid embryos in L. hispidula were also found though in smaller quantities. Interestingly, these results indicate Lachemilla as the very first case of documented apomixis from the páramo ecosystem. Our results suggest that populations of both species are asexual. Furthermore, insects found on these plants use them strictly as feeding sites, for protection and for reproduction, and rarely act as accidental pollinators.
Introduction
Lachemilla is a neotropical genus pertaining to the Rosaceae family. It comprises approximately 80 species distributed from southern California to northern Argentina and Chile [Citation1–Citation4]. The region between Venezuela and Ecuador is the area of highest diversity with 36 species [Citation2]. In Ecuador, Lachemilla has 27 species distributed within an altitude of 2000 and 5000 m.a.s.l. in the Andes [Citation5–Citation9] .
Pollination is defined as the transfer of pollen from the anthers to the stigma of the same or of another individual, which can be accomplished by the action of abiotic factors, water or wind, or biotic factors such as animals [Citation10]. Within Rosaceae, we can find animal-pollinated genera such as Duchesnea, Fragaria, Potentilla, Rubus, Amelanchier, Crataegus, Malus and Sorbus [Citation11–Citation13] and wind-pollinated plants such as Polylepis [Citation14]. Moreover, studies on the sister genera of Lachemilla, such as Alchemilla and Aphanes [Citation12], found that these are possibly pollinated biotically. In Alchemilla vulgaris, Pont [Citation15] determined that Muscidae present feeding activity, wherein they make contact with the anthers of this species. However, there is currently no published work on pollination studies in Lachemilla; therefore, we first made observations of the possible pollinators that we may found in these species.
Apomixis (sensu agamospermy), a process present in angiosperms, is defined as the asexual production of seeds from the maternal tissue of the ovule which gives rise to an embryo, even when fertilization and meiosis are circumvented [Citation16–Citation18]. Apomixis may be a facultative event, occurring simultaneously with sexual events [Citation19]. Bicknell and Koltunow [Citation17] observed that evergreen species that live in disturbed ecosystems and present mechanisms of vegetative reproduction are more likely to have apomictic events in their life cycle [Citation17]. Apomictic species are characterized by the formation of microspecies (i.e. populations with morphological variation), which are often problematic for taxonomic determination [Citation10,Citation17,Citation20].
Gametophytic apomixis is characteristic in Rosaceae, wherein the formation of the endosperm can be pseudogamous, when there is incentive fertilization; or autonomous, when it develops spontaneously [Citation17,Citation19,Citation21]. Alchemilla is characterized by autonomous apomixis, while Aphanes, by pseudogamy [Citation4,Citation22]. The presence of apomixis has not yet been established in Lachemilla.
Apomixis is associated with plants that live in extreme environmental conditions because it ensures a successful reproduction. The conditions present in the páramos, such as extreme cold, high UV radiation and occasional frosts constantly threaten the action of pollinators and the development of seeds. Apomixis has allowed plants to colonize sites that have been disturbed or covered by glaciers and increase their distribution at an altitudinal and latitudinal level, even more than related taxa that reproduced sexually. It has been seen that in these regions the autonomous apomixis is more prevalent than the pseudogamy due to the weather conditions [Citation23–Citation25].
Two species were chosen to carry out this research, Lachemilla hirta (L. M. Perry) Rothm., distributed from Venezuela to Ecuador; and Lachemilla hispidula (L. M. Perry) Rothm., found in Colombia and Ecuador [Citation2,Citation5]. Our aim was to document the processes of pollination and apomixis in both species in order to have an overview of what happens in the genus Lachemilla, whose reproductive biology has been unknown.
Materials and methods
Study population and treatments
Collections were conducted in the páramos of Oyacachi, in Cayambe Coca National Park located in the province of Napo, Ecuador. Two Lachemilla populations located approximately 350 m away from the Oyacachi park checkpoint were chosen. The first population corresponds to L. hispidula, located near the edge of the road (S 0° 11ʹ 4.6ʹ’ and W 78° 6ʹ 10.3ʹ’) at 3858 m.a.s.l.; and the second, to L. hirta, located within the Polylepis forest (S 0° 11ʹ 10.2ʹ’ and W 78° 6ʹ 7.8ʹ’) at 3865 m.a.s.l.
A total of 24 Lachemilla hirta and 24 L. hispidula individuals were included in the study. One inflorescence per treatment was selected in an individual of each species and it was enclosed when they presented buttons, so they were not simultaneously performed. Three treatments were applied in order to collect seeds and study their reproductive biology. Each treatment consisted of: (1) enclosing the inflorescences within mesh bags to isolate biotic pollinators and determine pollination [Citation26–Citation30]; (2) enclosing the inflorescences within wax paper bags to completely isolate the biotic and abiotic plant pollinators and, by flow cytometry seed screen (FCSS), determine apomixis; treatment (3) was the control (open pollination) which implied no enclosing of the inflorescence and was used to determine additive effect of pollination and apomixis. Each inflorescence of Lachemilla hirta possess (2) 3–9 (12) flowers and L. hispidula, (5) 7–10 (13) flowers. The achenes produced by Lachemilla are small single seed indehiscent fruits [Citation2,Citation10]. Thus, the separation of the seeds from the fruits was not required for the analyses conducted in this study.
Pollination
Seeds
Lachemilla hirta inflorescences were enclosed within mesh bags during the dry season (October–December) and wet season (January–September), while the inflorescences of L. hispidula were only enclosed during the wet season due to the difficulties of preserving the treatments intact.
Mature flowers from the mesh bags and control treatments were collected and dissected in the laboratory, and the fruits formed were counted [Citation27]. A one-way ANOVA and the Mann–Whitney test were performed based on the number of fruits obtained from each treatment using IBM SPSS Statistics 18® software.
Observation and pollinator collection
A total of 20 h of direct observation of each species, during midday hours (from 10:00 a.m. to 12:00 p.m.), was conducted throughout the wet and dry season of 2013. For 10 min, insects visiting the flowers were observed, and for another 10 min insects found upon them were collected by means of a vacuum cleaner, tweezers and brush. Insects found anywhere in the studied plants, except the flowers, were also collected. The insects were preserved in 70% ethanol, and laboratory identification was made to the level of order, family and subfamily [Citation26,Citation28,Citation31–Citation37]. Specimens were deposited in the Museum of invertebrates (QCAZ) at the Pontificia Universidad Católica del Ecuador (PUCE).
Pollen plates
Pollen was removed from the insect collections and plated as described by Wodehouse [Citation38]. The pollen plates were then compared with photographic pollen samples of Lachemilla hispidula, L. jamesonii, L. nivalis, L. orbiculata and L. vulcanica from the Paleobotany Laboratory (QCA) at PUCE.
Apomixis
Mature flowers enclosed within the wax paper bags were collected, and the seeds were extracted and dried to determine the mode of reproduction in both species, whether apomictic or sexual. Seeds obtained from the wax bag treatment, together with seeds from the mesh and control treatments, were used in the FCSS as described by Matzk et al. [Citation16]. Each achene and maternal plant was analyzed separately using propidium iodide flow cytometry following the simplified two-step protocol using Otto buffers [Citation39]. Pisum sativum cv. Ctirad (2C = 9.09 pg [Citation39]) was used as the internal standard. Together with 0.5 cm2 of internal standard leaf each achene was chopped up using a sharp razor blade in a Petri dish containing 0.5 ml of ice-cold Otto I buffer (0.1 Mcitric acid, 0.5% Tween-20 [Citation40]). The suspension was filtered through a 42-μm nylon mesh and incubated for ~30 min at room temperature. The staining solution consisted of 1 ml of Otto II buffer (0.4 M Na2HPO4.12 H2O) supplemented with 2-mercaptoethanol (final concentration of 2 μl/ml), propidium iodide and RNase II type IIA (both at a final concentrations of 50 μg/ml). After a short incubation, the samples were analyzed using Cyflow SL instrument (Partec GmbH, Münster, Germany) equipped with a green solid-state laser (Cobolt Samba, 532 nm, 100 mW). Fluorescence intensity of 3000 particles was recorded.
Additionally, two adult mother plants per species were collected to determine the level of ploidy of the parent plants [Citation39–Citation41].
Results
Pollination
Fruit formation
A total of 315 fruits from the Lachemilla hirta inflorescences enclosed within the mesh bags during the wet season as well as 314 control treatment fruits were recovered. Each flower produced between 0–3 fruits (achenes), with an average production of 0.82 in the mesh bag treatment and of 0.72 in the control treatment. The Mann–Whitney test found no significant differences in fruit formation between treatments (p > 0.05) ( and ). Regarding L. hirta inflorescences enclosed during the dry season, 128 fruits from the mesh bag treatment and 128 control treatment fruits were counted. Each flower produced between 0–3 fruits, with an average production of 0.85 for the mesh bag treatment and of 0.71 for the control treatment. The Mann–Whitney test found no significant differences in fruit formation between treatments (p > 0.05) ( and ). The existence of flowers with no fruits or undeveloped fruits was also observed in high percentages, 41% of the total of flowers analyzed in the mesh bags and 48% in the control treatment ().
Table 1. Count of seeds in Lachemilla hirta and Lachemilla hispidula obtained in the mesh bag treatment and control treatment during wet and dry season.
Table 2. Average fruit formation and comparison of fruit formation between treatments.
Two hundred and thirteen flowers extracted from the mesh bags and control treatments were analyzed in Lachemilla hispidula. Each flower produced between 1–4 fruits (achenes), with an average fruit formation of 1.90 for the mesh bag treatment, and of 2.30 for the control treatment. The Mann–Whitney test determined that there were highly significant differences in fruit formation between treatments (p < 0.01) ( and ). The seeds of L. hispidula used in the study correspond only to the wet season due to the difficulties of preserving the treatments intact from the livestock and human activity. Flowers with no fruits were not registered ()
Visitors, parasitoids and hyperparasitoids
Twenty-one collections (the term collection refers to all the insects that were collected in 1 day) were made, 12 corresponding to Lachemilla hirta and 9 to L. hispidula (). The insects were classified, by bibliography, as visitors (predators, detritivores, herbivores, flower visitors), parasitoids and hyperparasitoids in order to determine their functional role with the plants studied ( and ) [Citation33,Citation34].
Table 3. List of the total of arthropods collected in L. hirta and L. hispidula.
Table 4. List of visitors with its possible parasitoids and hyperparasitoids found in Lachemilla hirta and L. hispidula.
As flowers visitors, Diptera was the predominant order in the collections of Lachemilla hirta (68% of all insects) and L. hispidula (65% of all insects), followed by Hymenoptera (). Scatopsidae and Sciaridae were always found in greater proportions than other fly families collected (). Scatopsidae forms large groups on flowers during the dry season; however, they have a passive behavior (). Conversely, Sciaridae has an active behavior and is found throughout the year. Insects from both families were observed sucking the floral discs of the flowers when these presented a green color in Lachemilla hirta; or green with pink, in L. hispidula. The flowers that turned red were evaded by insects.
Less abundance and lower entomofauna activity was recorded throughout the investigation in Lachemilla hispidula except for Scatopsidae which was always found in large numbers ().
Thirteen pollen plates from Lachemilla hispidula and eight from L. hirta were obtained; additionally, pollen from other plant families was also found occasionally (it was not measured) among which were Bromeliaceae (Puya sp.), Poaceae and the Moraceae/Urticaceae complex.
Apomixis
All 28 successfully analyzed Lachemilla hirta seeds extracted from the wax paper bag, mesh bag and control treatments contained diploid embryos (i.e. exhibiting 2C DNA content similar to that of the mother plant) (). Of the 26 seeds in which the ploidy of both embryo and endosperm were determined, 23 exhibited mean endosperm: embryo ratio of 1.98, consistent with autonomous apomixis (i.e. 4n:2n) ()). Additionally, three seeds exhibited embryo: endosperm ratio of 2.91 which is closest to a scenario of pseudogamy involving pollination by unreduced pollen grain (i.e. 6n:2n) ()). The Lachemilla hispidula sample comprised 39 diploid embryos (i.e. with genome size corresponding to that of the mother plant), while three seeds had putative triploid embryo with on average 1.47-fold larger DNA content ()). The 15 successfully analyzed samples in which also endosperm was detected consistently showed and endosperm: embryo ratio of on average 2.03, suggesting that autonomous apomixis is the predominant mode of reproduction also in L. hispidula (, )). In sum, seeds of both Lachemilla species analyzed were of apomictic origin, 92% of L. hirta and all L. hispidula seeds originated by autonomous apomixis. Eight percent of the seeds were originated by pseudogamous apomixis, possibly due to wind or insect activity.
Table 5. Flow cytometric seed screen of the achenes of the investigated Lachemilla species.
Figure 2. (a) Histogram of autonomous apomixis in Lachemilla hirta. The first peak corresponds to the embryo 2x while the second peak corresponds to the endosperm 4x. The third peak corresponds to the standard sample (Pisum sativum). (b) Histogram of pseudogamous apomixis in L. hirta. The first peak corresponds to the embryo 2x while the second peak corresponds to the endosperm 6x. The third peak corresponds to the standard sample (Pisum sativum).
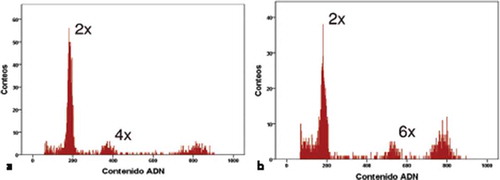
Figure 3. (a) Histogram of autonomous apomixis in Lachemilla hispidula. The first peak corresponds to the embryo 2x while the second peak corresponds to the endosperm 4x. The third peak corresponds to the standard sample (Pisum sativum). (b) Histogram of triploid embryos in L. hispidula. The first peak corresponds to the embryo 3x while the second peak corresponds to the standard sample (Pisum sativum).
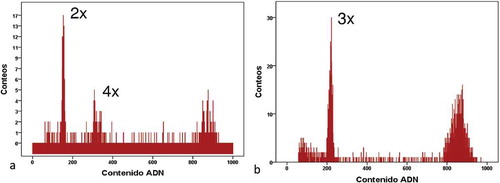
Discussion
Pollination
Fruit formation
The treatments determine the type of pollination present in a plant; that is, if there is fruit production in the mesh bag then it is assumed that plants are pollinated by the wind. However, if there is fruit formation in the control treatment it would mean that plants are pollinated by animals [Citation30,Citation31,Citation45]. Lachemilla hirta presented no significant differences in fruit production when compared to the control treatment, during both wet and dry season. As for L. hispidula, highly significant differences between treatments were obtained, with more fruit production found in the control when compared to the mesh bags. There was always fruit formation in the results obtained, both in the mesh bags and in the control treatment; therefore, and according to the results from the FCSS ( and ), it is suggested that both species are apomictic.
Scarce formation of fruits, a high frequency of flowers that did not produce fruit and the existence of undeveloped fruits was evidenced in Lachemilla hirta (). The formation of fruits and seeds depends on several factors including: the number of female flowers and ovules present, the amount of pollinated flowers and fertilized ovules, predation of fruits and seeds, abiotic factors such as climate, and the ability of the parent plants to provide the necessary resources for the development of the fruits and seeds [Citation46]. When a damaged and/or an unfeasible fruit is formed it is aborted as it is unnecessary to invest in fruits that contain nonviable seed, and thus initiates the formation of new fruits if available resources are sufficient [Citation46].
Visitors, parasitoids and hyperparasitoids
Although Diptera was the predominant order in the collections, with 67% of all the individuals collected from both species, the composition of the genera within each order vary due to their genera-specific nutritional and shelter requirements and due to changes in the composition of the flora [Citation47]. Changes in other abiotic factors such as in the water can also modify the entomofauna. For instance, Chironomidae (Diptera) was only collected from Lachemilla hispidula as there was a lake nearby and necessary for it to complete its life cycle ( and ) [Citation33,Citation45].
The functional role of each group of insects was determined based on bibliography and field observations, where detritivores (Acari and Collembola,), flower visiting insects (Diptera, Lepidoptera), herbivorous insects (Diptera larvae and Coleoptera), predatory Arthropods (Araneae and Neuroptera), parasitoids and hyperparasitoids coexist with the studied plants ( and ). Diptera, the most abundant order of the study area, corresponds to the second largest group of visitors and pollinators worldwide following Hymenoptera [Citation48–Citation51] (). Furthermore, Diptera is the main group of visitors and pollinators at high altitudes and latitudes due to its greater plasticity for adaptation to harsh weather conditions (e.g. low temperature, heavy rainfall and strong winds), less dependence on floral rewards, and longer foraging time as they do not feed progeny unlike Hymenoptera [Citation15,Citation23,Citation48,Citation51,Citation52]. Because, they do not pollinate Lachemilla hirta and L. hispidula, their importance lies in that they are the main source of food for many predators and parasitoids present in these plant species [Citation33,Citation34,Citation48].
Insects usually visit flowers for two reasons: the first, is related to food as nectar and pollen provide them with energy and proteins respectively; secondly, because it is an excellent place for reproduction, oviposition and for protection from harsh weather and predators [Citation49,Citation51,Citation53]. Although no nectar was found in neither Lachemilla hirta nor L. hispidula, information on its brother genus, Alchemilla vulgaris, demonstrates that Muscidae feed on its nectar [Citation15]; the main Diptera that visit Lachemilla, Scatopsidae and Sciaridae are nectarivores [Citation49].
The insects collected from Lachemilla hirta were constantly found perched on green flowers; the flowers were green with pink in the case of L. hispidula (). In a study on butterflies, the changing of flower color was associated with guiding the pollinators to flowers that were not pollinated, red being the color of evasion [Citation50].
Pollen from Bromeliaceae (Puya sp.), Poaceae and the Moraceae/Urticaceae complex were also found scarcely (not measure) on the pollen plates. This is corroborated by direct observation of Scatopsidae specimens foraging other plant species.
Apomixis
The FCSS applied to Lachemilla hirta and L. hispidula indicated that these species are mostly autonomous apomictic () and )). In addition, three seeds of L. hirta were likely apomictic with fertilization of the endosperm by an unreduced sperm cell (i.e. originated by pseudogamy) ()). Autonomous apomictic seeds presented a 2n peak for the embryo and a 4n peak for the endosperm (i.e. the observed ratio of the two peaks was ~2) (, ) and ). These values suggest that an unreduced female gamete was solely involved in the formation of the embryo (2n) while two unreduced central embryo sac nuclei merged (2n+ 2n = 4n) during formation of the endosperm. No male cell intervention was required in the formation of these apomictic seeds [Citation16,Citation19,Citation23,Citation54]. A similar autonomous apomictic mechanism has also been registered in the sister genus, Alchemilla [Citation4,Citation16], to our knowledge our result is the first case of such reproductive system in the New World genus Lachemilla.
In the three cases of pseudogamy, 2n peaks were determined for the embryo and 6n for the endosperm ()). The formation of the embryo is equally parthenogenetic; however, the endosperm is formed by merger of the two unreduced nuclear cells (2n+ 2n = 4n) plus an unreduced sperm cell (2n). In other words, the endosperm must be fertilized [Citation16,Citation19,Citation39,Citation54]. These pseudogamous events were only reported for Lachemilla hirta seeds, and were present in mesh bags and control treatments, which could indicate accidental activity by insect visitors or by wind. Such presence of both autonomous apomixis and pseudogamy within the same species was previously documented from European flora (e.g. [Citation22]). The FCSS also confirmed the presence of three triploid (2n = 3x) embryos in Lachemilla hispidula ()).
A chromosome count study performed in the Alchemillinae clade determined that its base number is x = 8 and that polyploidy and alloploiploidy are common events within it [Citation55,Citation56]. Lachemilla is a genus mostly polyploid (with the exception of L. mandoniana x = 16), and within the species selected for this study only L. hispidula has chromosome count data 2n = 24 (3x) and 2n = 32 (4x) [Citation55,Citation56]. According to Sharbel and Mitchell-Olds [Citation57], there are two theories for the association of polyploidy with apomictic species: one of which is that polyploidy is a requirement for apomixis because this way certain genes related to this reproductive mechanism can be transmitted more easily; or that polyploidy is non-essential for apomixis, but simply selected more easily in polyploid organisms than in diploid. Richards [Citation18], on the other hand, explains that polyploidy is a consequence of apomixis, and arises due to the absence of gene recombination; thus, the occurrence of hybrids is also favorable. Diploidy in apomixis favors the accumulation of disadvantageous mutations, for this reason, it is rare to find apomictic diploid plants. Some genera may contain species with different ploidy levels, and often vary geographically in their reproductive mechanisms [Citation56].
Triploid plants are unlikely to produce stable triploid seed through normal sexual mechanisms as genetically unbalanced gametes are formed; therefore, apomixis is the only viable mechanism for reproduction in these cases [Citation56–Citation58]. Triploid organisms play an important role in the formation of polyploidy [Citation58].
With this article we propose a baseline research about the reproductive biology of one population of Lachemilla hirta and one of L. hispidula in the páramo of Oyacachi, there are still many other questions to answer and other methodologies to be use to understand with clarity all the reproductive and ecological aspects of this genus. What happens with other populations of Lachemilla hirta and L. hispidula in the páramos of Oyacachi? In other locations? Is the apomixis present for the entire Lachemilla genus? Is the pollination necessary for the formation of pseudogamous seeds (from which they came), self-fertilization, wind and insects? What is the complete network of interactions of the insects found with the plants studied?
Acknowledgments
We thank Andrea Villota for her help in the development and identification of pollen plates, Julio Sánchez for his advice in the statistical design, Diego Morales for his advice and comments, Petr Sklenář for his upholder on the project and the Ministry of Environment of Ecuador for the research permit MAE No. 03-2012-IC-FLO-CPAR-MA.
Associate Editor: Luis D Llambi
Disclosure statement
No potential conflict of interest was reported by the authors.
Additional information
Funding
References
- Gaviria J, Sinópsis del género Lachemilla (Focke) Rydberg (Rosaceae) para Venezuela. [Synopsis of the genus Lachemilla (Focke) Rydberg (Rosaceae) for Venezuela]. Plantula [Internet]. 1996 [cited 2013 Nov 2];1(3):189–212. Available from: http://www.paramo.org/files/recursos/sinopsis_Lachemilla.pdf
- Romoleroux K, The genus Lachemilla (Rosaceae) in the northern Andes of South America. Lyonia [Internet]. 2004 [cited 2013 Nov 2];7(1):21–32. Available from: http://www.lyonia.org/articles/rbussmann/article_232/pdf/article.pdf
- Sklenář P, Luteyn JL, Ulloa Ulloa C, et al. Flora genérica de los Páramos: guía ilustrada de las plantas vasculares. [Generic flora of the Páramos: illustrated guide to vascular plants]. The New York Botanical Garden Press; 2005.
- Gehrke B, Bräuchler C, Romoleroux K, et al., Molecular phylogenetics of Alchemilla, Aphanes and Lachemilla (Rosaceae) inferred from plastid and nuclear intron and spacer DNA sequences, with comments on generic classification. Mol Phylogenet Evol [Internet]. 2008 [cited 2013 Nov 2];47(3):1030–1044. Available from: http://www.sciencedirect.com/science/article/pii/S1055790308001000
- Romoleroux K. Rosaceae 79. In: Harling G, Andersson L, editors. Flora of Ecuador vol 56. Stockholm: Publishing House of the Swedish Research Council; 1996. p. 89–132.
- Sklenář P, Jørgensen PM. Distribution patterns of páramo plants in Ecuador. J Biogeogr [Internet]. 1999;26(4):681–691.
- Luteyn JL. Páramos: a checklist of plant diversity, geographical distribution, and botanical literature. New York: New York Botanical Garden Press; 1999. p. 124–125.
- Morales-Briones DF Relaciones filogenéticas de Lachemilla (Focke) Rydb. (Rosaceae) inferidas a partir de secuencias de ADN nuclear y cloroplástico, con énfasis en las especies del norte de Sudamérica. [Phylogenetic relationships of Lachemilla (Focke) Rydb. (Rosaceae) inferred from nuclear and chloroplastic DNA sequences, with emphasis on northern species of South America] [Thesis] Pontificia Universidad Católica del Ecuador; 2009.
- Romoleroux K, Morales-Briones DF. Lachemilla jaramilloi and L. talamanquensis spp. nov. (Rosaceae) from Ecuador and Costa Rica. Nord J Bot [Internet]. Blackwell Publishing Ltd. 2012;30(6):732–736.
- Judd WS, Campbell CS, Kellogg EA, et al. Plant Systematics: a phylogenetic approach. Third ed. Sunderland (MA): Sinauer Associates, Inc.; 2008.
- Campbell CS, Greene CW, Dickinson TA. Reproductive biology in Subfam. Maloideae (Rosaceae). Syst Bot [Internet]. American Society of Plant Taxonomists. 1991;16(2):333–349. Available from: http://www.jstor.org/stable/2419284
- Bolmgren K, Eriksson O, Linder HP. Contrasting flowering phenology and species richness in abiotically and biotically pollinated angiosperms. Evolution (N Y) [Internet]. 2003;57(9):2001–2011.
- Brown AO, McNeil JN, Pollination ecology of the high latitude, dioecious cloudberry (Rubus chamaemorus; Rosaceae). Am J Bot [Internet]. 2009 [cited 2013 Nov 2];96(6):1096–1107. Available from: http://www.amjbot.org/content/96/6/1096.short
- Domic AI, Mamani E, Camilo G, Fenología reproductiva de la kewiña (Polylepis tomentella, Rosaceae) en la puna semihúmeda de Chuquisaca (Bolivia). [Reproductive phenology of the Kewiña (Polylepis tomentella, Rosaceae) in the semi-humid puna of Chuquisaca (Bolivia)]. Ecol En Boliv [Internet]. 2013 [cited 2013 Nov 2];48(1):31–45. Available from: http://www.scielo.org.bo/scielo.php?script=sci_arttext&pid=&lng=es&nrm=iso&tlng=
- Pont AC. Observations on anthophilous Muscidae and other Diptera (Insecta) in Abisko National Park, Sweden. J Nat Hist [Internet]. Taylor & Francis. 1993 [cited 2013 Nov 1];27(3):631–643.
- Matzk F, Meister A, Schubert I. An efficient screen for reproductive pathways using mature seeds of monocots and dicots. Plant J [Internet]. Blackwell Science Ltd. 2000;21(1):97–108.
- Bicknell RA, Koltunow AM, Understanding apomixis: recent advances and remaining conundrums. Plant Cell [Internet]. 2004 [cited 2013 Nov 1];16(Suppl(suppl_1)):S228–45. Available from: http://www.plantcell.org/content/16/suppl_1/S228
- Richards AJ, Apomixis in flowering plants: an overview. Philos Trans R Soc Lond B Biol Sci [Internet]. 2003 [cited 2013 Nov 2];358(1434):1085–1093. Available from: http://rstb.royalsocietypublishing.org/content/358/1434/1085.short
- Matzk F. Reproduction mode screening. Flow Cytometry with Plant Cells [Internet]. Wiley-VCH Verlag GmbH & Co. KGaA. 2007;131–152. DOI:10.1002/9783527610921.ch6
- Pihu S, Hõimra J, Köster E, et al. Environmentally dependent morphological variability in seven apomictic microspecies from Alchemilla L. (Rosaceae). Folia Geobot [Internet]. 2009 [cited 2013 Nov 2];44(2):159–176.
- Carneiro V, Dusi D, Ortiz J. Apomixis: occurrence, applications and improvements. Floric Ornam Plant Biotechnol [Internet]. 2006 [cited 2013 Nov 2];564–571. Available from: http://www17.plala.or.jp/gsbjapan/images/Vol1_sample.pdf
- Czapik R. Problems of apomictic reproduction in the families Compositae and Rosaceae. Folia Geobot [Internet]. 1996;31(3):381–387.
- Heenan PB, Molloy BPJ, Bicknell RA, et al. Levels of apomictic and amphimictic seed formation in a natural population of Coprosma robusta (Rubiaceae) in Riccarton Bush, Christchurch, New Zealand. New Zeal J Bot [Internet]. Taylor & Francis. 2003;41(2):287–291.
- Hörandl E, Dobeš C, Suda J, et al. Apomixis is not prevalent in subnival to nival plants of the European Alps. Ann Bot [Internet]. 2011 [cited 2013 Nov 2]; 108(2):381–390. Available from: http://aob.oxfordjournals.org/content/108/2/381.short
- Hörandl E, Cosendai A-C, Temsch EM. Understanding the geographic distributions of apomictic plants: a case for a pluralistic approach. Plant Ecol Divers [Internet]. Taylor & Francis. 2008 [cited 2013 Oct 30];1(2):309–320.
- Arroyo MTK, Primack R, Armesto J. Community studies in pollination ecology in the high temperate Andes of Central Chile. I. Pollination mechanisms and altitudinal variation. Am J Bot [Internet]. Botanical Society of America. 1982;69(1):82–97. Available from: http://www.jstor.org/stable/2442833
- Pendleton BK, Pendleton RL. Pollination Biology of Coleogyne ramosissima (Rosaceae). Southwest Nat [Internet]. Southwestern Association of Naturalists. 1998;43(3):376–380. Available from: http://www.jstor.org/stable/30055383
- Sakai S, Thrips pollination of androdioecious Castilla elastica (Moraceae) in a seasonal tropical forest. Am J Bot [Internet]. 2001 [cited 2013 Nov 2];88(9):1527–1534. Available from: http://www.amjbot.org/content/88/9/1527.short
- Pías B, Guitián P, Breeding system and pollen limitation in the masting tree Sorbus aucuparia L. (Rosaceae) in the NW Iberian Peninsula. Acta Oecologica [Internet]. 2006 [cited 2013 Nov 2];29(1):97–103. Available from: http://www.sciencedirect.com/science/article/pii/S1146609X05000949
- Fagua JC, Gonzalez VH. Growth rates, reproductive phenology, and pollination ecology of Espeletia grandiflora (Asteraceae), a giant andean caulescent rosette. Plant Biol [Internet]. Blackwell Publishing Ltd. 2007;9(1):127–135.
- Herrera J. Pollination relationships in southern spanish mediterranean shrublands. J Ecol [Internet]. British Ecological Society. 1988;76(1):274–287. Available from: http://www.jstor.org/stable/2260469
- Blanco MA, Barboza G, Pseudocopulatory pollination in Lepanthes (Orchidaceae: pleurothallidinae) by fungus gnats. Ann Bot [Internet]. 2005 [cited 2013 Nov 2];95(5):763–772. Available from: http://aob.oxfordjournals.org/content/95/5/763.short
- Triplehorn CA, Johnson NF. Borror and Delong’s introduction to the study of insects. Seventh ed. Belmont (CA): Thomson Brooks/ Cole; 2005.
- Hanson PE, Gauld ID. Hymenoptera de la región neotropical. [Hymenoptera of the neotropical region]. Vol. 77. Madison, WI: Memoirs of the American Entomological Institute; 2006.
- Etcheverry AV, Alemán MM, Fleming TF, Flower morphology, pollination biology and mating system of the complex flower of Vigna caracalla (Fabaceae: papilionoideae). Ann Bot [Internet]. 2008 [cited 2013 Nov 2];102(3):305–316. Available from: http://aob.oxfordjournals.org/content/102/3/305.short
- Ellis AG, Johnson SD, The evolution of floral variation without pollinator shifts in Gorteria diffusa (Asteraceae). Am J Bot [Internet]. 2009 [cited 2013 Oct 31];96(4):793–801. Available from: http://www.amjbot.org/content/96/4/793.short
- Ollerton J, Masinde S, Meve U, et al., Fly pollination in Ceropegia (Apocynaceae: asclepiadoideae): biogeographic and phylogenetic perspectives. Ann Bot [Internet]. 2009 [cited 2013 Nov 2];103(9):1501–1514. Available from: http://aob.oxfordjournals.org/content/103/9/1501.short
- Wodehouse RP. Preparation of pollen for microscopic examination. Bull Torrey Bot Club [Internet]. Torrey Botanical Society. 1933;60(6):417–421. Available from: http://www.jstor.org/stable/2480494
- Doležell J, Greilhuber J, Suda J. Estimation of nuclear DNA content in plants using flow cytometry. Nat Protoc [Internet]. 2007;2(9):2233–2244. Available from: http://www.ncbi.nlm.nih.gov/pubmed/17853881
- Otto F. DAPI staining of fixed cells for high-resolution flow cytometry of nuclear DNA. Methods Cell Biol. 1990;33:105–110.
- Doležell J, Greilhuber J, Meister A, et al. Plant genome size estimation by flow cytometry: inter-laboratory comparison. Ann Bot. 1998;82:17–26.
- Schranz ME, Dobes C, Koch MA, et al., Sexual reproduction, hybridization, apomixis, and polyploidization in the genus Boechera (Brassicaceae). Am J Bot [Internet]. 2005 [cited 2013 Nov 4];92(11):1797–1810. Available from: http://www.amjbot.org/content/92/11/1797.short
- Dobeš C, Lückl A, Hülber K, et al., Prospects and limits of the flow cytometric seed screen-insights from Potentilla sensu lato (Potentilleae, Rosaceae). New Phytol [Internet]. 2013 [cited 2013 Nov 1];198(2):605–616. Available from: http://www.pubmedcentral.nih.gov/articlerender.fcgi?artid=3618378&tool=pmcentrez&rendertype=abstract
- Loureiro J, Suda J, Doležel J, et al. FLOWER: A Plant DNA Flow Cytometry Database. In: Flow Cytometry with Plant Cells [Internet]. Wiley-VCH Verlag GmbH & Co. KGaA; 2007. p. 423–438. DOI:10.1002/9783527610921.ch18
- Grey J, Kelly A, Ward S, et al. Seasonal changes in the stable isotope values of lake-dwelling chironomid larvae in relation to feeding and life cycle variability. Freshw Biol [Internet]. Blackwell Science Ltd. 2004;49(6):681–689.
- Stephenson AG. Flower and fruit abortion: proximate causes and ultimate functions. Annu Rev Ecol Syst [Internet]. Annual Reviews. 1981;12:253–279. Available from: http://www.jstor.org/stable/2097112
- Smithers P, Ramsay PM, Bond AN, et al. Macro-arthropod communities of the giant rosette plant, Espeletia pycnophylla subsp. angelensis. In: Pm R, editor. The ecology of Volcán Chiles: high-altitude ecosystems on the Ecuador-Colombia border. Plymouth: Pebble & Shell; 2001. p. 169–175.
- Kevan PG, Baker HG. Insects as flower visitors and pollinators. Annu Rev Entomol [Internet]. Annual Reviews. 1983;28(1):407–453.
- Larson BMH, Kevan PG, Inouye DW. Flies and flowers: taxonomic diversity of anthophiles and pollinators. Can Entomol. Cambridge Journals Online. 2001;133(04):439–465.
- Campbell J, Hanula JL. Efficiency of malaise traps and colored pan traps for collecting flower visiting insects from three forested ecosystems. J Insect Conserv [Internet]. Springer Netherlands. 2007;11(4):399–408.
- Ssymank A, Ca K, Pape T, et al. Pollinating Flies (Diptera): A major contribution to plant diversity and agricultural production. Biodiversity [Internet]. Taylor & Francis. 2008;9(1–2):86–89.
- Dupont YL, Hansen DM, Olesen JM. Structure of a plant–flower-visitor network in the high-altitude sub-alpine desert of Tenerife, Canary Islands. Ecography (Cop) [Internet]. Munksgaard International Publishers. 2003;26(3):301–310.
- Sakai S. Aristolochia spp. (Aristolochiaceae) pollinated by flies breeding on decomposing flowers in Panama. Am J Bot [Internet]. 2002;89(3):527–534. Available from: http://www.amjbot.org/content/89/3/527.abstract
- Vinkenoog R, Scott R. Autonomous endosperm development in flowering plants: how to overcome the imprinting problem? Sex Plant Reprod [Internet]. Springer-Verlag. 2001;14(4):189–194.
- Morales-Briones DF, Romoleroux K, Kollar F, et al. Phylogeny and evolution of the neotropical radiation of Lachemilla (Rosaceae): uncovering a history of reticulate evolution and implications for infrageneric classification. Systematic Botany. 2018;43(1):17–34.
- Dickinson TA, Lo E, Talent N. Polyploidy, reproductive biology, and Rosaceae: understanding evolution and making classifications. Plant Syst Evol [Internet]. 2007;266(1–2):59–78.
- Sharbel TF, Mitchell-Olds T. Recurrent polyploid origins and chloroplast phylogeography in the Arabis holboellii complex (Brassicaceae). Heredity (Edinb) [Internet]. The Genetical Society of Great Britain. 2001;87(1):59–68.
- Burgess MB, Cushman KR, Doucette ET, et al. Effects of apomixis and polyploidy on diversification and geographic distribution in Amelanchier (Rosaceae). Am J Bot. 2014;101(8):1375–1387.