Abstract
Tillandsia landbeckii Phil. is a vulnerable species belonging to the terrestrial Bromeliaceae family; it is highly adapted to extremely hyper-arid conditions of the Atacama Desert and Peruvian deserts. In this study, we sequenced, assembled, and annotated its chloroplast genome. T. landbeckii chloroplast genome is 159,131 bp in length, containing a large single-copy region of 87,164 bp, a small single-copy region of 18,521 bp, and a pair of inverted repeat regions of 26,723 bp. The GC content of the chloroplast genome is 37.33%. It encodes a total of 132 genes, including 86 protein-coding genes, 38 tRNA genes and 8 rRNA genes. The phylogenetic tree indicates that T. landbeckii is placed within the Bromeliaceae family and has a close relationship with T. marconae with 100% support.
Keywords:
Tillandsia landbeckii is a perennial herbaceous species highly adapted to the Atacama Desert and South-Peruvian deserted areas' extremely hyper-arid conditions. It is distributed naturally from Lima Department, central of Peru, to Coquimbo region, north of Chile (Rundel and Dillon Citation1998; Pinto Citation2005). Due to the absence of functional roots, this species grows unattached to the sandy soil surface and absorbs the regular fog humidity through specialized leaf trichomes to survive in the arid coastal region of the Atacama and Peruvian desert (Rundel et al. Citation1997; Pinto et al. Citation2006). T. landbeckii occupies a unique ecological niche, which makes it particularly vulnerable to the effects of climate change and habitat destruction. Because of its extreme specialization and dependence on fog humidity, this species is considered as a‘vulnerable species’ in both countries (Zizka et al. Citation2009; Whaley et al. Citation2019; MMA Citation2021). So far, the chloroplast genome of T. landbeckii is not available. In this study, we sequenced and characterized the chloroplast genome of T. landbeckii to serve as a genetic resource for future studies on the taxonomy and to get a better understanding of phylogenetic relationships in the Tillandsia genus.
Sample of T. landbeckii was collected from Pampa Camarones, Arica, Chile (18° 52′ 31.40′ S; 70° 6′ 32.30′ W) and a specimen of T. landbeckii is conserved in the herbarium of Universidad de Concepción, Chile (http://www2.udec.cl/∼herbconc/, Dra. Alicia Marticorena, [email protected]) under the voucher CONC-184217. Total genomic DNA was extracted from fresh leaves using the DNeasy Plant Mini Kit (Qiagen, Hilden, Germany) following the manufacturer's instructions. DNA library was sequenced, and 150 pb paired-end reads were generated on an Illumina NovaSeq PE150 platform. After base quality control using FASTQC v01 (Andrews Citation2010), the remaining high-quality reads were used to assemble the chloroplast genome by SPAdes (Bankevich et al. Citation2012) and NOVOPlasty v01 (Dierckxsens et al. Citation2017), using T. usneoides chloroplast genome as a reference (KY293680.1). The chloroplast genome annotation was performed by GeSeq (Tillich et al. Citation2017) ().
Figure 1. Maximum likelihood (ML) tree based on chloroplast genome sequences of 20 species. Values along branches correspond to ML bootstrap percentages.
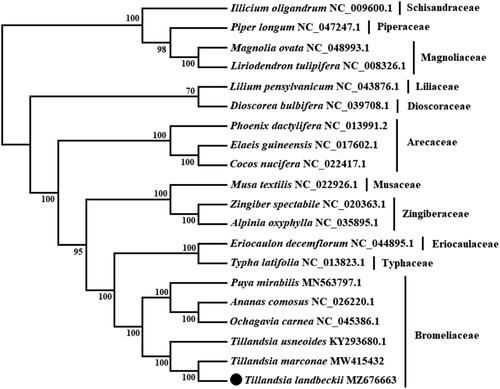
The chloroplast genome of T. landbeckii (GenBank accession number: MZ676663) is 159,131 bp in length with 37.33% GC content, displaying a quadripartite structure that contains a pair of inverted repeats (IR) regions (26,723 bp, GC content 42.68%), separated by a large single-copy (LSC) region (87,164 bp, GC content 35.31%) and a small single-copy (SSC) region (18,521 bp, GC content 31.46%). A total of 132 genes were predicted, including 38 tRNA genes, 8 rRNA genes, and 86 protein-coding genes.
A phylogenetic analysis was performed using chloroplast genomes from 20 species and with Magnolia ovata, Liriodendron tulipifera, Illicium oligandrum, and Piper longum as outgroup. The genomes were aligned using MAFFT version 7.475 software (Katoh and Standley Citation2013), involving the concatenation of 74 orthologue protein-coding genes. Five species from Bromeliaceae, three from Arecaceae, two from Zingiberaceae, and one each from Typhaceae, Eriocaulaceae, Musaceae, Liliaceae, and Dioscoreaceae corresponding to Liliopsida class were included in the analysis. The phylogenetic analysis was conducted based on maximum-likelihood (ML) analysis using IQ-Tree software (Nguyen et al. Citation2015) under GTR + I + G nucleotide substitution model, which was selected by Jmodeltest 2 (Darriba et al. Citation2012). The ML tree was inferred by bootstrapping with 1000 replicates. The phylogenetic tree showed that T. landbeckii is placed under the Bromeliaceae family and has a close relationship with T. marconae with 100% support. This chloroplast genome will contribute to conservation, phylogenetic and evolutionary studies in desert plant species.
Disclosure statement
No potential conflict of interest was reported by the authors.
Data availability statement
The data that supports this study is openly available in GenBank of NCBI (https://www.ncbi.nlm.nih.gov) under the accession number MZ676663. The associated Bio project, Biosample, and SRA numbers are PRJNA742530, SAMN19959867, and SRR15015612, respectively.
Additional information
Funding
References
- Andrews S. 2010. FastQC: a quality control tool for high throughput sequence data [Online]. http://www.bioinformatics.babraham.ac.uk/projects/fastqc/
- Bankevich A, Nurk S, Antipov D, Gurevich AA, Dvorkin M, Kulikov AS, Lesin VM, Nikolenko SI, Pham S, Prjibelski AD, et al. 2012. SPAdes: a new genome assembly algorithm and its applications to single-cell sequencing. J Comput Biol. 19(5):455–477.
- Darriba D, Taboada G, Doallo R, Posada D. 2012. jModelTest 2: more models, new heuristics and parallel computing. Nat Methods. 9(8):772.
- Dierckxsens N, Mardulyn P, Smits G. 2017. NOVOPlasty: de novo assembly of organelle genomes from whole genome data. Nucleic Acids Res. 45(4):e18.
- Katoh K, Standley DM. 2013. MAFFT multiple sequence alignment software version 7: improvements in performance and usability. Mol Biol Evol. 30(4):772–780.
- MMA. 2021. Inventario nacional de especies de Chile. Ministerio del Medio Ambiente. http://especies.mma.gob.cl/CNMWeb/Web/WebCiudadana/ficha_indepen.aspx?EspecieId=927&Version=1
- Nguyen L-T, Schmidt HA, von Haeseler A, Minh BQ. 2015. IQ-TREE: a fast and effective stochastic algorithm for estimating maximum-likelihood phylogenies. Mol Biol Evol. 32(1):268–274.
- Pinto R. 2005. Tillandsia del Norte de Chile y del extremo sur del Peru. Raquel Pinto (eds). Santiago, Chile: Gas Atacama. p. 15-21.
- Pinto R, Barría I, Marquet PA. 2006. Geographical distribution of Tillandsia lomas in the Atacama Desert, northern Chile. J Arid Environ. 65(4):543–552.
- Rundel PW, Dillon MO. 1998. Ecological patterns in the Bromeliaceae of the Lomas formations of Coastal Chile and Peru. Pl Syst Evol. 212(3–4):261–278.
- Rundel PW, Palma B, Dillon MO, Sharifi MR, Boonpragob K. 1997. Tillandsia landbeckii in the coastal Atacama Desert of northern Chile. Rev Chil Hist Nat. 70:341–349.
- Tillich M, Lehwark P, Pellizzer T, Ulbricht-Jones ES, Fischer A, Bock R, Greiner S. 2017. GeSeq – versatile and accurate annotation of organelle genomes. Nucleic Acids Res. 45(W1):W6–W11.
- Whaley O, Orellana-Garcia A, Octavio Pecho-Quispe J. 2019. An annotated checklist to vascular flora of the Ica Region, Peru—with notes on endemic species, habitat, climate and agrobiodiversity. Phytotaxa. 389(1):1–125.
- Zizka G, Schmidt M, Schulte K, Novoa P, Pinto R, König K. 2009. Chilean Bromeliaceae: diversity, distribution and evaluation of conservation status. Biodivers Conserv. 18(9):2449–2471.