Abstract
Freshwater habitats and species have been lost because of river constructions, particularly regarding hydropower plants. Such losses may be mitigated, for example, by providing compensatory habitats. We present a case which had the objective to promote reproduction of a key species. Imatra City Brook in Finland was constructed in 2014 at a hydropower dam as a new reproduction area for brown trout (Salmo trutta L.). In the planning phase, the objective was to maximize suitable habitat types and areas with limited discharge. Monitoring shows promising densities of brown trout juveniles within only three years, inspiring hope for high smolt production in the main river. The increase in total macroinvertebrate abundances and taxa numbers in the second year of monitoring showed that species richness was comparable to that in natural rivers, and the brook was in a good ecological state. This case confirms the expected high potential of spawning and rearing channels to improve migratory fish stocks and compensate for lost habitats in constructed rivers.
1. Compensatory spawning and rearing areas for improving fish stocks
River management worldwide faces conflicting interests, such as the use of hydropower, fisheries, and tourism. In many countries with constructed rivers, improving reduced migratory fish stocks has attracted considerable attention, and actions to remove dams and restore rapids for fisheries and recreation are ongoing. In rivers with power plants, adverse impacts on fish stocks can be mitigated by fish passes, if suitable reproduction areas are available. Furthermore, restoration of river sections can be achieved by defining environmental flows. However, in many rivers original reproduction sites remain under damming or have been dredged. Destruction of habitats may thus be compensated for by creating new habitats, preferably on-site or nearby. Artificial waterbodies that substitute natural or semi-natural ecosystems are a means of replacing destroyed habitats (Katopodis and Kemp Citation2018). The construction of bypass channels is one such option for habitat compensation, which is frequently underestimated. Bypass channels can provide key habitats for rheophilic species in constructed rivers (Pander et al. Citation2013). From a population perspective, the overall functioning of compensatory habitats benefits from the addition of rearing and overwintering habitats (Clarke Citation2016).
In Finland, the policy of maintaining migratory fish stocks in permission process of hydropower plants has been based mainly on stocking with Atlantic salmon (Salmo salar L.) and brown trout (Salmo trutta L.); however, their stocking performance has declined in the recent decades (Romakkaniemi Citation2008). In the Finnish fish pass strategy of 2012, a new policy for reviving valuable fish stocks by natural reproduction was presented (Ministry of Agriculture and Forestry Citation2012). Near-natural fish passes which can be suitable habitats were a preferred strategy. Planning guidelines for spawning and rearing habitat recreation have been published with estimations of reproduction rates (e.g. Järvenpää et al. Citation2010). In practice, however, the possibility of creating new habitats has rarely been considered in fish pass projects where facilitating migration is the main target. The objective of this study was to determine the possibilities of promoting juvenile production and to assess the ecological value of a channel specifically designed to produce habitats.
2. Reproduction channels in Canada and Europe
2.1. Canada
In Canada, spawning and rearing channels for salmonids were already established in the 1950s (Roos Citation1991), with the objective to mitigate adverse impacts of hydropower plants and log floating. Spawning channels have been constructed to enhance the stocks of Pacific salmon species such as pink salmon (Oncorhynchus gorbuscha) and red salmon (Oncorhynchus nerka). Their fry migrate from the spawning site to the sea or a nearby lake soon after hatching (National Park Service Citation2019). Because of this behaviour, the main target of spawning channels was to increase the incubation rate of eggs, compared to that in natural rivers, which was beneficial for fish stocks. In the Seton River spawning channel (3.6 km length), the total production of pink salmon was enhanced beyond the original capacity of the natural spawning grounds prior to hydropower plant construction (Roos Citation1991). The channel was modified in 2003 to function as a rearing channel for silver salmon (Oncorhynchus kisutch) and king salmon (Oncorhynchus tshawytscha), which spend two to three years in the channel, similar to Atlantic salmon and brown trout. The term “rearing channel” is typically used for channels where juveniles spend extended periods of time after hatching (Adolph Citation2003). The slope in the Seton River channel was 0.1%–0.7%, and its mean depth was 0.38 m. Rearing channels in Canada frequently include wetland habitats, which are considered important for benthic macroinvertebrates and are feeding habitats for Pacific salmon species (Adolph Citation2003).
There is also an example of Atlantic salmon rearing channels in Canada, which is located at the Granite Canal power plant in Newfoundland (McCarthy and Sellars Citation2009). The rearing channel was constructed to compensate for the loss of reproduction habitats by constructing 4.5 hectares of reproduction area in a channel of 2.5 km length. This constituted compensation of the lost area in a ratio of 1:1, which was required for obtaining the hydropower license. Juvenile densities ranged from 50 to 155 individuals/100 m2, which was higher than those in natural rivers (McCarthy and Sellars Citation2009). This case represents ideal policy implementation: the requirement for full compensation of the lost habitat facilitated fish reproduction was equal to or even exceeding natural juvenile production. This channel provided spawning habitats for a large proportion of landlocked salmonid populations. Moreover, invertebrate colonization of newly constructed diversion channels has shown that such channels can become productive small-stream habitats within a relatively short period of time (Chapelsky et al. Citation2020).
2.2. Europe
In Europe, the requirement to mitigate adverse impacts of water construction on the migration, spawning, and reproduction of fish is included in the Water Framework Directive (WFD), i.e. in the requirements for heavily modified water bodies (European Parliament Citation2000). Mitigation of damage and compensation of ecologically valuable areas in construction projects have been included in the environmental legislation of many European countries, e.g. in Germany since the 1980s (ELAW Citation2002). One good example of the establishment of new habitats is the reproduction channel of the Rheinfelden power plant at the Rhine River upstream of Basel, Switzerland. The channel was constructed as a compensatory measure when the power plant was renovated and dam height was increased (Ulrich Citation2013). The channel is 1 km long, 50 m wide, and has a discharge of 10–35 m3 s−1. A total of 34,000 fish belonging to 33 species were observed in the channel in 2012 (Ulrich Citation2013).
Near-natural fish bypass channels have proven valuable for ecological continuity and as habitats. Monitoring of three bypass channels at two tributaries of the Main river, Germany, showed that bypass channels provided migration corridors and key habitats for juvenile and small rheophilic fish in a constructed river, compared to the sections of the upstream and downstream main channel (Pander et al. Citation2013). This seemed to be due to the high variability of flow velocity and water depth (Pander et al. Citation2013).
In Scotland, the Dunglass side channel was constructed in 2003 through an island of the river Conon (Wallace Citation2013). The channel is approximately 1 km long, and the minimum discharge is 0.5 m3 s−1. Atlantic salmon entered the channel soon after construction and began to use it as the lowest spawning site of the river. Juvenile densities were as high as 160 yearling juveniles/100 m2 in the first summer and 70 juveniles/100 m2 in the second summer (McKelvey Citation2008).
In Sweden, Nyqvist et al. (Citation2017) noted that when a technical fish pass was replaced by a near-natural bypass channel, the migration of adult salmon increased in upstream and downstream directions. In a study on 23 Swedish bypass channels, the channels were found to host the same fish communities as natural river sections, indicating their potential as compensatory habitats (Tamario et al. Citation2018). The nature-like fishway at the Eldforsen Power Plant, Sweden, was constructed with an emphasis on creating diverse aquatic habitats (Gustafsson Citation2017). Within two years, the channel contained macroinvertebrates with a functional feeding group (FFG) composition similar to that of natural brooks. The ratio of different groups showed that, rather than resembling a small forest brook, the channel was more similar to the main stem of a large river. Large woody debris was preferred in the habitat selection of introduced brown trout, which indicated that new channels benefit from added wood material.
3. Materials and methods
3.1. Imatra City Brook at the Vuoksi river
The Imatra City Brook in south-eastern Finland was constructed in autumn 2014 to establish a new spawning and rearing area for brown trout of the Vuoksi river (). Here, the results of development and performance during the first years of its existence are presented. The main questions included a) what is the density of different age classes (0+ and 1+) of brown trout juveniles in the brook, and b) how did the macroinvertebrate diversity in this artificial stream develop during its first years.
The Vuoksi river flows from Lake Saimaa, the largest lake system in Finland, to the Russian Lake Ladoga. The height of the Imatra power plant dam is 24 m. After the construction of the Imatra power plant in 1929, the Imatra rapids, a famous tourist attraction since the 19th century, have mostly been dry, except when water is released for short shows. The Imatra rapids served as an important spawning site in this river. Upstream of Lake Saimaa, there is a vulnerable stock population of landlocked salmon (Salmo salar sebago) which occurred there since the last ice age (Seppovaara Citation1962). Due to the construction of power plant dams, most natural reproduction sites in the upper part of the river were no longer available. A brown trout stock which inhabits the dammed sections of the river is mainly managed by regular restocking.
In southern Finland, where the Vuoksi river is located, the spawning season of brown trout is from October to November. Suitable spawning sites and habitats for juveniles regarding water depth, substrate type (gravel), and flow velocity (Mäki-Petäys et al. Citation1997, Louhi et al. Citation2008) are found mainly in tributaries and at only a few restoration sites of the main stream between power plants. Fry hatch from eggs deposited in gravel substrate in May and move to nearby sites which offer rocks and small cobbles for shelter.
The implementation of the European WFD is based on typification and classification of the ecological status of all inland water bodies. The main channel of the Vuoksi is a very large river in regions with mineral soils, i.e. a very large clearwater river, according to the Finnish river typology classification. It has been defined as a heavily modified water body but has been attributed with good ecological potential in the Vuoksi River Basin Management Plan (RBMP) for the future period of 2022–2027. Measures to increase natural brown trout reproduction are promoted, and compensatory habitats are supported by the RBMP.
The construction of the brook was an initiative of the city of Imatra in order to promote fishing in the river and to create a new water feature for tourism. The new brook enables residents and tourists to experience flowing water in this particular area at any time in the year.
3.2. Characteristics of the Imatra City Brook
The length of the brook is 1 km, with an average slope of 2.5%. Water flows through two pipes into two ponds in the upstream section of the brook (). A subsequent section of 400 m follows a rather straight course of a former log-floating chute. The brook flows in a tunnel under the main street with a pier for pedestrians. As a memorial of the former log floating chute, a 30-m long wooden aqueduct was constructed to lead the brook over a valley containing a seepage brook, which is also the emergency spillway of the power plant. The downstream section was designed with small gradients to increase the length of the channel and to provide suitable habitats (). The wetted width of the brook varies from 1 to 5 m, and the widths of the first two ponds and the original wetland are up to 10 m.
Figure 3. The habitat section was designed with a winding channel and small gradients, with deeper pools in between.
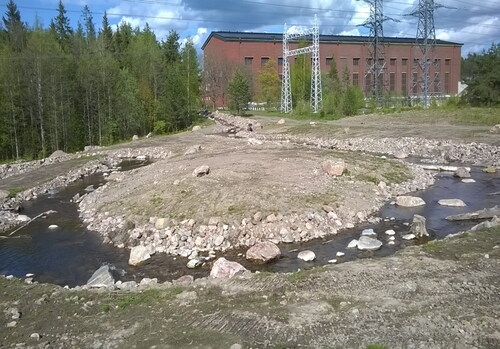
Following an open tender, planning of the brook was performed by a consortium that included the landscape architecture bureau MA-arkkitehdit, the Finnish Environment Institute SYKE, Ecoriver Ltd and other specialists. The construction agency was VRJ South Finland, and the channel was finalized by the Southeast Finland ELY Centre. The brook provided an opportunity to compare the goals of the plan with the constructed situation, hydraulics, and later, the performance of the new channel as a suitable spawning and rearing habitat for fish.
This case serves as an example of how compensative reproduction can be achieved through design and construction. The habitats were planned according to optimal habitat preferences of brown trout and Atlantic salmon in natural rivers, with respect to water velocity, depth, and substrate types (Mäki-Petäys et al. Citation1997, Citation2002 CitationLouhi et al. 2008). As suitable reproduction habitats are rare in the constructed Vuoksi river, special attention was paid to spawning sites with gravel and habitats with gentle slopes that are suitable especially for yearlings (0+) juveniles (Jormola et al. Citation2016; ). Steeper sections and deeper pools were designed for larger fish and for overwintering. Flow and habitat models were created during planning, and they showed a vast extent of suitable brown trout habitats. The target flow gradients were 0.25%–1%, with pools and steeper sections of up to 5% slope. The slope of the straight upstream section was, on average, 2%– 3% and contained mostly riffles and small pools. As the downstream section flowing to the Vuoksi river was very steep with partly up to 16%, the ability of fish to migrate upstream was a concern in the beginning.
Trees, especially alder (Alnus sp.) and birch (Betula sp.), have also started to grow on the banks of the downstream section through natural seeding, however, they have not yet had a marked effect on the brook. Shade and shelter for fish are provided by coarse woody debris, such as tree trunks and stumps that were placed in the brook. The amount of leaf litter entering the brook or the amount of coarse particulate organic matter in macroinvertebrate samples were not evaluated.
We identified 11 taxa of submerged plants, and Potamogeton, Callitriche, and Myriophyllym were among the most common ones. Most aquatic plants originated from the upstream river basin and were most abundant in the two ponds with inlet pipes. We found dense growth of Potamogeton alpinus in the old wetland of the seepage brook, which appeared to provide protection to juvenile brown trout. Elodea canadensis started to form dense growths at downstream site B (), where it is currently the most common submerged plant. By contrast, the aquatic moss Fontinalis antipyretica, which is known to host macroinvertebrates and provide shelter to juvenile fish, established very slowly and occurred only at sites where it was introduced in 2016.
3.3. Hydraulics and physicochemical water quality
The discharge of the brook was planned to be 300 l s−1 in summer and 180 l s−1 in winter, as released by Fortum Power Company. A total of 30 l s−1 drained into the seepage brook. Owing to reparation works of the power plant, the discharge was limited to < 250 l s−1 during this study. Laser scanning of channel topography and water levels was performed after construction. The longitudinal flow gradients were close to those initially planned. The hydraulic parameters were compared with the goals of the plan and the model. Walter velocities and depths of the constructed channels were measured in 2015. The velocities at the respective habitats showed values between 0.1 and 0.7 m s−1, which was in accordance with the plan and habitat preferences of juvenile brown trout (Mäki-Petäys et al. Citation2002). These measurements suggested that the constructed channel offered suitable habitats, as there were stable hydraulics without extreme discharges, compared to natural streams (Koljonen et al. Citation2016). Water velocity in the steep downstream section was 0.7–2.0 m s−1.
According to the WFD physico-chemical status classification, water quality in the Vuoksi river was excellent. Water colouration ranged from oligo- to mesohumic (median water colouration was 35, ranging from 25 to 40 MgPt l−1, in the years 2012–2017; N = 47). The trophic status in Lake Saimaa was oligotrophy, whereas Vuoksi river was oligo-mesotrophic. The median total phosphorous concentration during the past five years was 7 µg l−1, and the median total nitrogen concentration was 420 µg l−1. There was very little annual variation in pH levels for an outlet stream of this size: the range during the past five years was 7.0 to 7.3, with alkalinity of 0.19–0.24 mmol l−1. The low concentration of ammonia (median 2 µg l−1and maximum 18 µg l−1) and very small amounts of suspended solids (median 1.0; maximum 1.7 mg l−1; N = 47; 2012–2017) indicated good water quality. The lowest concentration of oxygen during this same period was 8.5 mg l−1 which was observed during late summer 2014, when also the highest water temperature of 19.8 °C was recorded. The median values of 11.7 mg l−1 for oxygen and 7.6 °C for water temperature were ideal for salmonid fish. These data were collected at the nearest water quality station at the Vuoksi river main channel, situated 3 km upstream of the inlet into the Imatra City Brook (source: Hertta Environment database of the Finnish Environmental Institute). As only minor amounts of urban storm water and no sewage water were released into the river between the station and the brook and the discharge of the Vuoksi river was large (mean annual flow 684 m3 s−1), the water quality parameters were assumed to reflect those in the brook.
3.4. Monitoring of fish and macroinvertebrates
The brook was included in the electrofishing monitoring program of the Vuoksi river in 2016, when the first generation of brown trout from natural spawning was observed. Initial electrofishing was conducted in August 2015 to determine the species which had been visually observed first. Combined monitoring of fish and macroinvertebrates was conducted in October 2016 and September 2017. Five monitoring sites with different habitat structures were used for electrofishing and four for surveying benthic macroinvertebrates; two of these sites were identical sites (). Downstream site A for surveying macroinvertebrates was in the steep section of the brook flowing to the Vuoksi river. Electrofishing site 1 included part of the original wetland of the seepage brook, which existed before the construction of the Imatra City Brook. Site 2 for electrofishing and site B for macroinvertebrate monitoring represented a special habitat section with a gentle slope of approximately 0.5%. Sites 3 and 4 for electrofishing and site C for macroinvertebrate monitoring were located in the straight section with a slope of approximately 2% and with a diversified mix of riffles, pools, and some sections with low gradients. The uppermost sites (5 and D) were in the upstream section of the brook, under the lower pond, with mostly riffles with approximately 3% slope. We calculated the data of electrofishing collected by one-time fishing and estimated the population densities (according to Junge and Libosvarsky Citation1965) using the average catchability of brown trout (catchability = 0.6) and averaged the densities as individuals/100 m2. The average catchability was estimated by repeated three-time fishing at Imatra City Brook in several sampling sites in three consequent years (Syrjänen et al. Citation2021, unpublished).
Macroinvertebrates were monitored using kick-net sampling (net mesh size 0.5 mm). We collected four subsamples (for 30 sec, each, over an area of ca. 0.3 m2) covering only riffle habitats (according to the monitoring scheme of the WFD) from each inventory site A–D () and placed macroinvertebrates and associated bottom material in 70% ethanol for preservation in the field. We processed the samples in the laboratory and identified macroinvertebrates at the species or genus level in 2016 and 2017. We identified the FFG (Merritt et al. Citation2008) of each taxon according to the latest knowledge of species with different feeding types; macroinvertebrates were thus divided into gatherers/collectors, scrapers, and filter feeders (including active and passive filter feeders), shredders predators, and others.
We recorded the average number of individuals per sample at each sampling site and the total number of taxa per sampling site. Furthermore, the following indices were produced to describe the overall ecological quality: occurrence of type-specific (EPT; Ephemeroptera, Plecoptera, and Trichoptera; Lenat and Crawford Citation1994) families for small clearwater rivers (catchment area less than 100 km2) and very large clearwater rivers (catchment area more than 10 000 km2), and, according to Aroviita et al. (Citation2012), the occurrence of stream type-specific taxa (TT) for the two river types above; the percent model affinity (PMA) for these river types was also produced. PMA compares the observed taxonomic composition (measured as the relative abundances of taxa) at a site to the taxonomic composition of a reference model assemblage (Aroviita et al. Citation2012).
The indices were determined for small and large clearwater rivers as the Imatra City Brook is, according to its width, a small brook; however, its waters originate from the Vuoksi river, thus it may be considered a side channel of a very large river. The EPT, TT, and PMA indices were compared to the respective values representative for south Finland. These indices are typically used in river basin management for the classification of water bodies (European Commission Citation2008, Vuori et al. Citation2009, Aroviita et al. Citation2012) and facilitate a comparison of existing diversity in the Imatra City Brook to that in natural rivers. To estimate diversity at different sampling sites and years, we calculated the Shannon diversity index (Shannon Citation1948). Additionally, we produced an overview of the stream bank and aquatic vegetation coverage in 2017 to evaluate the correlation of vegetation with macroinvertebrates and fish. and show the overall dominance of large trees near the brook in the upper section and the openness of the downstream habitat section. As the development of aquatic vegetation was in its initial stage, we produced only a qualitative inventory.
4 Results
4.1. Fish in the brook
The initial brown trout population was introduced in the Imatra City Brook in the summer of 2015, and it originated from a small brook hosting a natural population (transfer from the other side of the power plant, distance 0,5 km). This introduced population comprised 88 individuals aged 1+ to 2+ years. Of these, 20 individuals were PIT-tagged for monitoring, however, none of the marked fish were caught from the brook during subsequent electrofishing.
The predominant fish caught by electrofishing in 2016 and 2017 were juvenile brown trout. Based on the electrofishing results, we calculated the age group densities of juvenile brown trout (0+ and 1+) separately for each of the five sampling sites ( and ). The yearling 0+ average was 40 and 43 individuals/100 m2 in 2016 and 2017, respectively, resulting in a mean 0+ brown trout density of 41.5 individuals/100 m2.
Table 1. Brown trout density estimations for age groups 0+ and 1+ juvenile densities (per 100 m2), according to electrofishing results.
With an average density of 30.6 for age class 1+ in 2017, the calculated average survival rate of the year class 2016 was 76.5%. The average total density of two year classes (0+ and 1+) in 2017 varied between sites (1–5) from 34 to 150 individuals/100 m2.
The electrofishing catch in 2017 contained one burbot individual (Lota lota; weight 266 g) caught at the highest site. Some individuals of small rheophilic fish such as European bullhead (Cottus gobio) and ninespine stickleback (Pungitius pungitius) were caught in 2016, and stone loach (Barbatula barbatula) were caught in 2017. In the first year (2015), hundreds of bleak (Alburnus alburnus) and common roach (Rutilus rutilus) were visually observed, which migrated into the brook, showing rapid fish colonization. Juvenile bleaks and roaches and one perch (Perca fluviatilis) were caught during initial electrofishing in August 2015.
4.2. Macroinvertebrates
In 2016 1,711 macroinvertebrate individuals were collected, whereas in 2017, 8,601 individuals were collected (); the total number had thus increased markedly over the two years. The total number of taxa at all sampling sites increased from 26 to 47.
Table 2. Number of macroinvertebrate taxa and individuals in 2016 and 2017, divided in FFG.
In 2016, the most abundant species was the caddisfly Neureclipsis bimaculata, which was predominant at the highest site D and represented almost one-third of all collected individuals. In 2017, the number of mayflies Baetis rhodani and B. niger increased considerably. Stoneflies (Plecoptera) clearly increased in number in 2017, as their taxa number tripled and the average number of individuals per sample doubled. Leuctra and Isoperla were new genera in 2017, and there were also new species of Trichoptera caddisflies, such as Ceraclea dissimilis, Hydropsyche newae, and Psychomyia pusilla.
In the upstream part of stream site D, where the water comes from the Vuoksi river (), macroinvertebrate samples showed the richest taxonomic composition. Site D produced 31 taxa from 2017, with a large number of Neureclipsis bimaculata, mayfly Heptagenia sulphurea, Isopod Asellus aquaticus, and Chironomidae midges. It produced some taxa which were only present on this site of the stream, such as the amphipod Pallaseopsis quadrispinosa, which typically occurs in deep lakes and, in this case, moved upstream from larger water bodies.
Site C produced 21 taxa in 2017, with high numbers of B. rhodani, Hydropsyche siltalai, Polycentropus flavomaculatus, and Chironomidae. Site B produced 17 taxa, mainly B. rhodani, Paraleptophlebia sp., and Polycentropus flavomaculatus. Site A in the steep downstream section produced 26 taxa, and B. rhodani represented the largest number (1,799 individuals); many other taxa were represented by only one or two individuals. The abundances of taxa and individuals divided into FFG’s are presented in and .
Figure 5. Percentages of individuals attributed to FFGs in Imatra City Brook in 2016 (left) and 2017 (right).
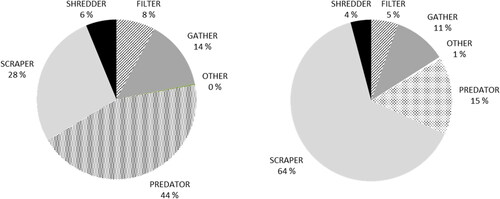
In 2016, the largest group was predators, according to the large number of Neureclipsis bimaculata and Rhyacophila nubila at the highest site D. The proportion of scrapers in total individual numbers increased from 28% in 2016 to almost two thirds (64%) of the total numbers in 2017. Baetis rhodani was the most common taxon in this group. At the same time, the proportion of predators which were predominant in 2016, decreased from 44% to 15% in 2017, even though the numbers of individuals and taxa doubled. The relative number of filter feeders, shredders, and gatherers showed a minor decrease. There were three to four times more scrapers in the completely open downstream sections A and B than at the partly shaded upstream site D in 2017. The species inhabiting numerously the upstream site D were N. bimaculata and Heptagenia sulphurea, and in 2016 also R. nubila; of these, N. bimaculata was either missing or very rare at sites other than site D.
The calculated indices representing the ecological diversity of Imatra City Brook are shown in . In 2016, the number of EPT families for both small and large rivers was moderate at sites A, B, and C, and it was good at site D. In 2017, EPT families for both river types increased by one category so that site D showed high ecological quality; however, there were three more taxa in the index for large rivers at sites A, B, and D than for small rivers.
Table 3. Ecological and diversity indices of the macroinvertebrate community in the City Brook in years 2016 and 2017.
The TT index for small rivers, which was still poor in 2016 at all sites, increased in 2017 to “moderate” at sites B and C, and to “good” at sites A and D. For large rivers, the TT index in 2016 was “moderate” at site D. In 2017, the TT index for large rivers increased to “moderate” at sites B and C, to “good” at site A, and to “high” at site D. As a general conclusion according to the EPT index, the Imatra City Brook reached a good or even high ecological status in 2017 and at the highest site D already in 2016. Similarly, according to the TT index, the brook partly reached a good or high ecological status in 2017. The values showed high diversity at the highest site D at the origin of the brook near the water inflow, with influences from the main watercourse.
The PMA index showed moderate values only for small rivers at downstream sites A–C and, accordingly, only for large rivers at the highest site D, indicating differences in the composition of taxa near the entrance pipe and the rest of the brook. The overall PMA values did not increase between study years, apart from site C, where the community composition in 2017 approached a good status and that of the model community category of small rivers.
The Shannon diversity index, which indicates overall diversity, was comparably low at the downstream sites A and B, and it was slightly higher at the upstream sites C and D in both years. Diversity varied slightly between the two years. The Shannon diversity index values were comparable to those observed at Eldforsen (Gustafsson Citation2017).
5. Discussion
The Imatra City Brook is an example of an artificial stream, designed according to preference conditions of a key species, the brown trout. The brook represents one solution of ecohydraulics (Katopodis and Kemp Citation2018). Our key finding was that a new brook can be colonized rapidly by species originating from the surrounding watercourse and, thus representing a new habitat with ecological value.
The spawning migration of brown trout into the Imatra City Brook began during the first autumn after construction of the brook (2015); however, only mature brown trout individuals were caught by the preliminary electrofishing. During the study years only few three-year-old or older fish were caught from the study sites.
The electrofishing results indicated high densities of juvenile brown trout from 2016 onwards. The brook flows freely into the river, thus a part of both year groups, especially of the older more mobile one, may have been able to migrate out of the brook into the river. There were very few suitable spawning sites in the main river, and there was no evidence that juveniles of both year classes migrated into the brook, thereby increasing the number of juveniles in the brook. We assume that comparing the numbers of year classes provides a rough estimate of the calculated survival rates. We did not perform exact monitoring of individual movements, but comparison of the age classes at each monitoring site showed tendencies of probable changes in the location of the fish from 2016 to 2017 more upstream.
Estimated average survival rates of brown trout yearlings to 1+ individuals was 76.5%, which was considerably higher than normal survival under natural settings, indicating a suitable habitat, low predation-mediated mortality, and stable environmental conditions. In addition, the average densities were high, compared to the long-term monitoring of 28 natural rapids in Central Finland (Heinimaa et al. Citation2016, Syrjänen et al. Citation2021); brown trout densities in Imatra City Brook in 2016 and 2017 observed in the current study were similar to the average densities of the two sites with the highest densities between 1996 and 2017. The high density of first-summer juveniles at one site downstream of the tunnel suggested the influence of special channel characteristics providing protection to spawning adults and small juveniles. With a habitat area of 2,500 m2, the estimated brown trout population size of Imatra City Brook comprised over 1,000 individuals of age class 0+ and approximately 750 juveniles of class 1+; this juvenile age class reached the age of smoltification and migration to the main river, which is the main purpose of the brook.
The fish community of the brook comprised eight species after natural colonization during three years since construction. The great majority of fish were juvenile brown trout, which is considered an endangered species in inland waters in Finland. Three small species also colonized the brook,. i.e. European bullhead, stone loach, and ninespine stickleback. The steep section downstream did not seem to be a hindrance to these species, which are frequently considered weak swimmers. Apparently, the nature-like channel structure, resembling natural rapids with diverse vortexes, provided migration routes for most species and facilitated a mixed composition of species in the brook. This result is in line with the findings of Pander et al. (Citation2013) showing that bypass channels can be essential new habitats for juvenile life stages and small rheophilic species in heavily modified rivers.
Macroinvertebrate colonization tended to increase during the two study years, as shown by increasing total numbers of individuals and taxa. Overall, the highest rates of colonization were observed regarding Baetis rhodani, and at the upstream site, especially regarding Neureclipsis bimaculata. In general, colonization rates differ between invertebrate groups; for example, caddisflies (Trichoptera) and mayflies (Ephemeroptera) disperse faster than leaches and Mollusca.
Scrapers were the predominant group among FFGs, however predators (in the first study year) and filter feeders were also abundant. FFG categorization has been criticized previously because it neglects ontogenic changes, seasonality, and omnivory (Friberg and Jacobsen Citation1994; Ledger and Hildrew Citation2000); however, this categorization was also accepted as a suitable approach to simplify high taxonomic diversity to ecologically meaningful entities. The reason for the dramatic increase in scrapers in 2017 may be partly the result of their fast rate of colonization (especially regarding mayflies). The colonization process itself may play the most prominent role in these changes as no differences in physicochemical water quality or in the amount of water in Imatra City Brook were observed.
This may be partly associated with the overall openness of the channel, which led to an increase in algae from 2016 to 2017. The brook had mostly no canopy cover at most of the sampling sites, which resulted in very small amounts of terrestrial leaf litter entering the brook, thus keeping the abundance of shredders low. However, lacking canopy cover allows more solar radiation to penetrate the water, thereby increasing algal production in the downstream parts of the brook and at site D, thus promoting scrapers. We had no quantitative estimation of dry mass of algae vs. that of leaf litter in any part of the stream, thus prohibiting further conclusions on the relationships between autotrophy and heterotrophy. During the first years after construction of the brook, a bloom of the diatom Didymosphenia geminata was observed at the beginning of the brook and at the highest site D, indicating oligotrophy (see section 3.3). This bloom appeared to be beneficial for several macroinvertebrate species (see also Rost and Fritsen Citation2014, Fenoglio et al. Citation2020). Trees provided shade and debris at most upstream areas, however, there were also areas almost barren of streamside vegetation, such as macroinvertebrate sampling site D.
Colonization started at the highest sampling site of the City Brook simply as the inlet pipe from the Vuoksi mainstream was nearest to site D. Most indices reflecting community composition, especially those considering aquatic insects, supported this phenomenon. The absolute number of taxa and individuals as well as the overall ecological quality (based on EPT, TT, and PMA indices of the large river type) were highest at site D in both years.
Over less than three years, the average number of taxa increased to 32, and the average number of individuals was approximately 500 per sample. All ecological quality indices improved in the upstream site D, and the Shannon diversity index increased slightly. The increase in the number of taxa was two-fold from 2016 to 2017 at site D, indicating high rates of drift and colonization from the inlet pipe. Furthermore, there may be colonization by crawling and other mechanisms (e.g. oviposition by flying insects) at the downstream end, as the taxa number tripled from 2016 to 2017, notably raising the EPT number and doubling the number of TT (). The monitoring of macroinvertebrates has been performed annually since 2017. The increase in the number of individuals continued; however, this was most evident at the upstream site D (Haapala et al., unpublished). Colonization thus continues as an ongoing process.
Overall, our results are in line with those of Gustafsson (Citation2017) regarding the rate of colonization and the dominance of scrapers in the earlier phases of brook colonization. Both the number of EPT families and TT showed higher values for large and very large river types, when compared to small rivers. The PMA index corroborated these results in the uppermost part; this phenomenon may explain why colonization started there and why the community at this site most closely resembled the model community of a very large river. The findings that the overall community composition better resembled a model community of a very large clearwater river than that of small rivers (despite the fact that the average channel width in the City Brook is < 5 m and the discharge is 0.2–0.3 m3/sec) were also in line with previous observations (Gustafsson Citation2017).
Microhabitat selection models for macroinvertebrates, presented by Forcellini et al. (Citation2022), could suit well for predicting the development of constructed habitats. In our case, biological traits like colonization and fast growth of algae seem to explain most of the macroinvertebrate assemblage. The role of hydrological parameters like velocity and shear stress may become more important in the future when the environmental conditions stabilize – these factors were not monitored so far in our study.
6. Conclusions
The fish densities of brown trout as a key fish species in the Imatra City Brook are, despite its short existence, comparable to the best natural streams in Central Finland (Heinimaa et al. Citation2016). Survival rates of the two age classes were also higher than those in natural streams. This indicates that constructed reproduction channels have a high potential as compensative reproduction areas for brown trout near power plants where natural reproduction sites are often destroyed.
The colonization of macroinvertebrates indicates the rapid development of Imatra City Brook. Scrapers were the predominant FFG, which may be an indirect indication of high autochthonous primary production in the brook with very limited streamside vegetation.
Drift from the main river may play a major role in invertebrate colonization, as indicated by the highest number of taxa and one lake species from profundal zones, Pallaseopsis quadrispinosa, in the uppermost sampling site in both study years, as well as by the highest number of individuals in the first year. Diversity indices (compared to values reported by Gustafsson et al. Citation2013) showed good or even high diversity at all three upstream sites after a short time of existence of the brook. Presence of benthic macroinvertebrates, together with colonization by several fish species, shows that constructed reproduction channels can rapidly develop into diverse ecosystems with considerable value as compensative habitats (see also Huttunen et al. Citation2018, Chapelsky et al. Citation2020).
This study shows that targeted planning and construction of artificial channels according to the requirements of key species can strongly support natural fish production. It also shows that such measures should be recommended for policies to support river basin management and to revive fish communities and increase the ecological value of constructed rivers.
Acknowledgements
The Finnish Environment Institute SYKE and the Centre for Economic Development, Transport, and Environment of South-Eastern Finland provided possibilities for the research and monitoring of fishes and other biota through different project finances. Fishery Manager Tomi Menna from Imatra City assisted in the electrofishing studies; he was originally the key initiator of the construction of the brook and is a current riverkeeper of this stream. Pinja Kasvio from SYKE assisted with sampling macroinvertebrates. Senior research scientist Heikki Mykrä from SYKE provided comments on the indices. Jukka Syrjänen from the University of Jyväskylä offered valuable help with the electrofishing data processing. We would like to thank the reviewers for taking the time and effort to review the manuscript. We sincerely appreciate all your valuable comments and suggestions, which helped us in improving the quality of the manuscript.
Disclosure statement
No potential conflict of interest was reported by the author.
References
- Adolph B. 2003. Lower Seton spawning channel complexing project. Lillooet, B.C., Canada: Cayoose Creek Fisheries.
- Aroviita J, Hellsten S, Jyväsjärvi J, Järvenpää L, Järvinen M, Karjalainen SM, Kauppila P, Keto A, Kuoppala M, Manni K, et al. 2012. Guidelines for the ecological and chemical status classification of surface waters for 2012–2013 – updated assessment criteria and their application. Environ Administration Guidelines. 7/2012:1–144. (in Finnish).
- Aroviita J, Mitikka S, Vienonen S. 2019. Pintavesien tilan luokittelu ja arviointiperusteet vesienhoidon kolmannella kaudella. Suomen Ympäristökeskuksen Raportteja. 37:1–182. (in Finnish). https://helda.helsinki.fi/handle/10138/306745.
- Chapelsky AJ, Guzzo MM, Hrenchuk LE, Blanchfield PJ. 2020. Invertebrate colonization of a newly constructed diversion channel in the Canadian Shield. Can J Fish Aquat Sci. 77(9):1477–1486.
- Clarke KD. 2016. Evaluating habitat compensation in insular newfoundland rivers: what have we learned? Fisheries and Oceans Canada, Canadian Science Advisory Secretariat. Research Document 2016/069. https://www.researchgate.net/publication/306077640_Evaluating_Habitat_Compensation_in_Insular_Newfoundland_Rivers_What_have_we_Learned.
- ELAW. 2002. Federal Nature Conservation Act of 25 March 2002. https://www.elaw.org/content/germany-federal-nature-conservation-act-25-march-2002.
- European Commission. 2008. Commission decision of 30 October 2008 establishing the values of the Member State monitoring system classifications as a result of the intercalibration exercise (2008/915/EC). Official J European Union 332:20–44.
- European Parliament. 2000. 2000/60/EC of the European Parliament and of the Council of 23 October 2000 establishing a framework for Community action in the field of water policy. https://eur-lex.europa.eu/legal-content/EN/TXT/?uri=CELEX:32000L0060.
- Fenoglio S, Tierno de Figueroa JM, Doretto A, Falasco E, Bona F. 2020. Aquatic insects and benthic diatoms: a history of biotic relationships in freshwater ecosystems. Water. 12(10):2934.
- Forcellini M, Plichard L, Dolédec S, Mérigoux S, Olivier J-M, Cauvy-Fraunié S, Lamouroux N. 2022. Microhabitat selection by macroinvertebrates: generality among rivers and functional interpretation. J Ecohydraulics. 7(1):28–41.
- Friberg N, Jacobsen D. 1994. Feeding plasticity of two detritivore-shredders. Freshwater Biol. 32(1):133–142.
- Gustafsson S. 2017. Habitat compensation in nature-like fishways – effect on benthos and fish [doctoral thesis]. Karlstad University Studies; p. 46. http://kau.diva-portal.org/smash/record.jsf?pid=diva2%3A1153328&dswid=3387.
- Gustafsson S, Österling M, Skurdal J, Schneider LD. 2013. Macroinvertebrate colonization of a nature-like fishway: the effects of adding habitat heterogeneity. Ecol Eng J Ecosys Restor. 61:345–353.
- Heinimaa P, Syrjänen J, Kivinen J, Sivonen O, Sivonen K, Keskinen T, Valkeajärvi P. 2016. Keski-Suomen taimenseuranta vuonna 2015 (Brown trout monitoring in Central Finland in 2016). In Finnish. Reports of Konneveden kalatutkimus ry 1/2016. http://konnevedenkalatutkimus.fi/media/Keski-Suomen_taimenseuranta_2015_%282%29.pdf.
- Huttunen K-L, Mykrä H, Paavola R, Muotka T. 2018. Estimates of benthic invertebrate community variability and its environmental determinants differ between snapshot and trajectory designs. Freshwater Sci. 37(4):769–779.
- Jormola J, Koljonen S, Koskiaho J, Tammela S, Tapaninen M. 2016. Planning and construction of compensative reproduction channels for salmonid fish. Proceedings of the International Conference on Ecohydraulics, ISE 2016, Melbourne. http://ise-2016.m.asnevents.com.au/schedule/session/8110/abstract/26097.
- Junge CO, Libosvarsky J. 1965. Effects of size selectivity on population estimates based on successive removals with electrical fishing gear. Zool. Listy. 14:171–178.
- Järvenpää L, Jormola J, Tammela S. 2010. Planning of nature-like bypass channels in a constructed river. (In Finnish, with figure texts and reference in English). The Finnish Environment 5/2010. https://helda.helsinki.fi/handle/10138/37987.
- Katopodis C, Kemp PS. 2018. Challenges of integrating habitat for aquatic life and morphodynamics offer a plethora of opportunities for advances in ecohydraulics. Journal of Ecohydraulics. 3(1):1–3.
- Koljonen S, Jormola J, Koskiaho J. 2016. Bypass channels can serve as compensative reproduction habitat for salmonids. Proceedings of the International Conference on Ecohydraulics ISE 2016, Melbourne http://ise-2016.m.asnevents.com.au/schedule/track/1019/session/8133/abstract/26089.
- Ledger M, Hildrew AG. 2000. Herbivory on acid stream. Freshwater Biol. 43(4):545–556. http://www.creeweb.org/wp-content/uploads/2012/01/Compensation_guidance.pdf.
- Lenat DR, Crawford JK. 1994. Effects of land use on water quality and aquatic biota of three North Carolina streams. Hydrobiol. 294:85–199.
- Louhi P, Mäki-Petäys A, Erkinaro J. 2008. Spawning habitat of Atlantic salmon and brown trout: general criteria and intragravel factors. River Res Applic. 24(3):330–339.
- Mäki-Petäys A, Muotka T, Huusko A, Tikkanen P, Kreivi P. 1997. Seasonal changes in habitat use and preference by juvenile brown trout, Salmo trutta, in a northern boreal river. Can J Fish Aquat Sci. 54:520–520.
- Mäki-Petäys A, Huusko A, Erkinaro J, Muotka T. 2002. Transferability of habitat preference criteria of juvenile Atlantic salmon (Salmo salar). Can J Fish Aquat Sci. 59(2):218–228.
- McCarthy JH, Sellars B. 2009. Can engineering drawings create fish habitat?. Waterpower XVI. Spokane, Washington: PennWell Corporation.
- McKelvey S. 2008. Dunglass electro-fishing summaries 2004-2006. Unpublished.
- Merritt RW, Cummins KW, Berg MB. 2008. An Introduction to the aquatic insects of North America. Kendall/Hunt, Iowa.
- Ministry of Agriculture and Forestry. 2012. Kansallinen kalatiestrategia (National fishpass strategy, in Finnish). https://mmm.fi/kalat/strategiat-ja-ohjelmat/kalatiestrategia.
- National Park Service. 2019. The Salmon Life Cycle. Olympic National Park Washington. https://www.nps.gov/olym/learn/nature/the-salmon-life-cycle.htm.
- Nyqvist D, Nilsson PA, Alenäs I, Elghagen J, Hebrand M, Karlsson S, Kläppe S, Calles O. 2017. Upstream and downstream passage of migrating adult Atlantic salmon: remedial measures improve passage performance at a hydropower dam. Ecol Eng. 102:331–343.
- Pander J, Mueller M, Geist J. 2013. Ecological functions of fish bypass channels in streams: Migration corridor and habitat for rheophilic species. River Res Applic. 29(4):441–450.
- Romakkaniemi A. 2008. Conservation of Atlantic salmon by supplementary stocking of juvenile fish [academic dissertation]. Department of Biological and Environmental Sciences, Faculty of Biosciences, University of Helsinki and Finnish Game and Fisheries research Institute. http://ethesis.helsinki.fi.
- Roos JF. 1991. Restoring Fraser River salmon. A History of the International Pacific Salmon Comission 1937-1985. Vancouver, British Columbia, Canada: The Pacific Salmon Commission.
- Rost A, Fritsen CH. 2014. Influence of a tributary stream on benthic communities in a Didymosphenia geminata impacted stream in the Sierra Nevada, USA. Diatom Res. 29(3):249–257.
- Seppovaara O. 1962. Zur Systematik und Ökologie des Lachsen und der Forellen in den Binnengewässern in Finland. Ann. Zool. Soc. Vanamo. 24:1–86.
- Shannon CE. 1948. A mathematical theory of communication. Bell Syst Tech J. 27(3):379–423.
- Syrjänen J, Eloranta A, Sivonen K, Keskinen T, Ruokonen T, Valkeajärvi P. 2021. Brown trout monitoring results of Kivikoski and Simunankoksi rapids in 1983–2021. Unpublished.
- Tamario C, Degerman E, Donadi S, Spjut D, Sandin L. 2018. Nature-like fishways as compensatory lotic habitats. River Res Appl. 34(3):253–261.
- Ulrich J. 2013. Zwishenergebnis der Fischzählung am neuen Wasserkraftwerk Rheinfelden. Wasserwirtsch. 103(6):54–57.
- Vuori KM, Mitikka S, Vuoristo H, 2009. Guidance on ecological classification of surface waters in Finland. Part1 Aroviita J, Hellsten S, Jyväsjärvi J, Järvenpää L, Järvinen M, Karjalainen SM, Kauppila P, et al. 2009. Reference conditions and classification criteria, Part 2: guidelines for the ecological and chemical status classification of surface waters for 2012-2013. Environ Administration Guidelines 7/2012:1–144. (in Finnish).
- Wallace C. 2013. River channel, through Dunglass island https://www.geograph.org.uk/photo/3342834