Abstract
We examined in laboratory settings the effects of power-frequency electric (14 kV/m) and magnetic (150 µT) field exposures, mimicking that of high voltage overhead transmission lines, on the viability of resting eggs of a cosmopolitan freshwater ostracod Heterocypris incongruens. The experiment was carried out in two consecutive phases: (I) an incubation (dry) phase, and (II) a hatching and development (wet) phase. In the first phase, eggs were incubated dry in one of three conditions: electric field, magnetic field and control, and then, in the second phase, the eggs were inundated with water and assigned to one of two hatching conditions: either the same as in incubation or control. Eggs incubated in control conditions were assigned either to the electric field, magnetic field or remained in control conditions. Differences in: hatching success, dynamic of hatchability, and juvenile survival between control eggs/juveniles and those exposed to each of the stress treatment combinations were compared in the balanced design. The magnetic field significantly affected the dynamic of hatchability: 40% of neonates appeared at 4 days in magnetic field treatment vs. 8 days in control; however, final hatching success was equal in all combinations of treatments. Incubation in both magnetic and electric fields significantly affected juvenile survival: only 21.7% (vs. 51.7% in control) and 16.7% (vs. 41.7% in control) of juveniles reached the adult stage in magnetic and electric fields, respectively. We conclude that the power frequency electromagnetic field created by high voltage overhead transmission lines can have important consequences for fitness-related life history traits of aquatic invertebrates.
Introduction
As a result of the increased use of electricity and the long distances between energy production and consumption, a grid of new high-voltage overhead transmission power lines is going to increase dramatically its expansion, covering and crossing a substantial number of habitats, including priority areas for wetland conservation. The possible adverse biological effects on humans and agricultural or laboratory animals due to exposure to the 50–60 Hz electromagnetic fields generated by transmission lines have been of concern for over four decades; however, the results of the previous studies have been as yet inconclusive or controversial (reviewed in U.S. Congress OTA Citation1989; Fernie & Reynolds Citation2005; WHO Citation2007; Lewczuk et al. Citation2014; Redlarski et al. Citation2015).
Due to the health hazard and other probable negative effects of electromagnetic fields on living organisms, the Council of the European Union issued a Recommendation 1999/519/EC (Recommendation Citation1999) and a Directive 2004/40 EC (Directive Citation2004) specifying limitation of exposure of the general public and occupational zones to electromagnetic fields, based on limit values from guidelines of the International Commission on Non-Ionizing Radiation Protection (Vecchia Citation2007; ICNIPR Citation2010). According to these two EU legislative documents (Recommendation Citation1999; Directive Citation2004) exposure limits for the general public for power frequency (50 Hz) electromagnetic fields are set as 5000 V/m for electric field strength and as 100 μT (microTesla) for magnetic flux density, whereas occupational exposure limits are 10,000 V/m and 500 μT, respectively. Because neither the Recommendation (Citation1999) nor the Directive (Citation2004) has been transposed in binding national legislation in all EU member states yet, there is still a variety of restrictive policies associated with electromagnetic fields in the EU countries. Some member states decided to implement these regulations or even more rigorous values, while in others there are more lenient limits or there is no regulation. Diversity of national rules and limits in the member states and some other countries are reviewed by Stam (Citation2018). However, even in the states where the national limit regulations are in force, real values exceed the reference levels (Goiceanu & Danulescu Citation2006; WHO Citation2007).
Although electromagnetic fields easily enter all shallow aquatic ecosystems, the impacts of power lines on freshwater biodiversity have been poorly addressed, and relatively little is known about how electric and magnetic components of electromagnetic fields generated by power wires may influence the life history characteristics of freshwater invertebrates. Some notable exceptions are laboratory studies on the deleterious consequences on survival, development and reproduction parameters under electromagnetic field exposures in a water flea Daphnia magna Straus, a key species in many lentic freshwater habitats (Krylov Citation2008, Citation2010; Krylov & Osipova Citation2013).
It should be also stressed that most studies have examined either the coupled effects of two primary components of electromagnetic fields or focused on just one of these, electric or magnetic, and ignoring the other. However, for low-frequency fields of the power transmission system the wavelength is much larger than the distance to the source, and therefore the two fields (electric and magnetic) are uncoupled (Hanningan Citation2013), and their effects may be studied separately.
In the present paper we examined in laboratory settings effects of power frequency (50 Hz) intense electric (14 kV/m) and magnetic (150 μT) components of electromagnetic field, simulating and/or exceeding parameters existing in the immediate proximity to high voltage overhead transmission lines, on fitness-related life history traits (hatchability dynamic, hatching success and juvenile development) during incubation and hatching of a cosmopolitan ostracod Heterocypris incogruens (Ramdohr). This is a fairly ubiquitous and euryvalent microcrustacean species recorded from various fresh- and slightly brackishwater habitats, however, it clearly prefers small and shallow freshwaters. Reproducing mainly parthenogenetically and producing eggs highly resistant to desiccation and freezing, H. incongruens easily colonizes new water bodies, has the capacity to develop populations of great size and is a key species in seasonal wetland ecosystems (Meisch Citation2000).
Materials and methods
Material of test species
The experiment was conducted using resting (dormant or diapausing) parthenogenetic eggs of Heterocypris incongruens obtained as Ostracodtoxkit FTM from MicroBioTest Inc., Belgium. Given that H. incongruens displays considerable genetic clonal diversity, we consider high-quality eggs of Ostracodtoxkit FTM, produced in strictly controlled conditions, a kind of reference material of this species as the test conforms to the ISO Standard 14371 and has already been used extensively in ecotoxicology to perform highly standardized bioassays for toxicity screening with a sensitivity similar to that of other common freshwater invertebrates. See web pages of the International Organization for Standardization (ISO Citation2012) and that of the MicroBio Tests Inc. company (Microbiotests Citation2020) for additional references.
Electric and magnetic fields exposure system
In order to produce an electric field specially designed cube-shaped chambers were used with the following dimensions (length x width x height) 605 × 170 × 180 mm. Every chamber was made of acrylic glass (Plexiglass) plates. The bottom (605 × 170 mm) and smaller wall (170 × 180 mm) of the chamber were one-layer Plexiglass plates, whereas the bigger side walls (605 × 180 mm) were formed of two layers of Plexiglass plates, between which cooper plates of a 0.1 mm thickness were inserted, and thus forming a flat condenser (with a space for ostracod culture multiplates inside). The copper plates were connected to a high voltage power supply producing a 14,000 ± 700 V/m electric field between the walls inside the chamber.
Chambers producing a magnetic field expressed as a magnetic flux density of 150 ± 7.5 μT were cylindrical glass containers with an outer diameter of 250 mm and a height of 80 mm. The transformer wire was wound on the cylinder forming a magnetic coil and the two ends of the wire were connected to the low voltage power supply through a resistor. Both cubic (producing electric field) and cylindrical (producing magnetic field) chambers were placed on a rack with integrated lighting and controlled temperature.
Electric field and magnetic flux density were measured by means of a calibrated device PMM 8053 with a PMM EHP-50C probe, and the final parameters of such power frequency (50 Hz) electric and magnetic fields as well as the control conditions during the experiment were as follows: electric field of 14,000 ± 700 V/m and <0.1 μT, magnetic field of <10 V/m and 150 ± 7.5 μT, and control of <10 V/m and <0.1 μT. The parameters of the electric and magnetic fields generated in our experiment were equivalent to conditions existing under high voltage overhead transmission lines.
Experimental design
The experiment was carried out in two consecutive condition phases: (I) an incubation (dry) phase, and (II) a hatching and development (wet) phase. Eggs in the incubation phase were incubated dry in 6-well polystyrene multiplates (mean ± standard error = 69 ± 5.6 eggs per well) at 16°C in the dark for 6 weeks in one of two field treatments: electric field (EF) or magnetic field (MF), and control (C). After the incubation phase, eggs were inundated with mineral water (Primavera, Polska Woda Sp. z o.o., Poland) and assigned to hatching conditions, so that six replicates from each stress (field) incubation treatment remained for hatching in the same conditions as they had been incubated, and the other six were transferred to C, while eggs incubated in C were assigned to one of three hatching treatments: EF, MF and C (each of also six replicates). The hatching phase of the experiment lasted 22 days and was conducted at 25ºC, at a light intensity of 5500 lx (6500 K) with a 14:10-hour light:dark photoperiod. The first 30 neonates from each of seven treatment combinations (incubation x hatching: C x C, C x EF, C x MF, EF x C, EF x EF, MF x C, MF x MF) were transferred to new 6-well multiplates with water (5 neonates per replicate well), fed by Spirulina powder from blue-green algae, and the experiment was continued in the same treatment combinations until ostracods reached adulthood or died. Every day (except day 9) during the first 15 days and then every other day of the hatching phase, hatching and mortality were recorded by counting the number of emerging and surviving ostracod individuals in each replicate.
Tested life history traits and statistical analysis
We tested three fitness related life history traits: (I) dynamic of hatchability (shape of cumulative hatching curves), (II) the total hatching success (proportion of resting eggs that hatched), and (III) proportion of juvenile survival to the adult stage. Difference in the life history traits between control eggs or juveniles and those exposed to the stress treatments (either to electric or magnetic field) was tested separately for each combination of the incubation and hatching conditions in the balanced design by two-way PERMANOVA (with Euclidean distance as resemblance measure in case of hatching success and juvenile survival, and maximum distance in case of hatchability curves) using the software package PRIMER v. 7 (Anderson et al. Citation2008; Clarke & Gorley Citation2015).
Results
In total, 2907 resting eggs were incubated and subsequently inundated, of which 1335 (45.9%) hatched within the 22-day monitoring period of the hatching phase of the experiment. The proportion of resting eggs that successfully hatched varied only inconsiderably among treatments and neither exposure of electric nor magnetic field affected total hatching success (Pseudo-F = 0.029–3.260, p(perm) ≥ 0.084; ).
Table I. Statistics of two-way crossed PERMANOVA (Pseudo-F value and p(perm) value) testing in the balanced design for effects of incubation condition treatments (IN), hatching condition treatments (HA) and their interaction (IN x HA) on the dynamics of hatchability (similarity between cumulative hatching curves), the total hatching success (proportion of resting eggs that hatched), and the juvenile survival to the adult stage of Heterocypris incongruens in the exposure of intense electric (EF) and magnetic (MF) fields versus control conditions (C). Data are mean and standard error (SE)
In case of electric field exposure, there were no main and interaction effects of incubation and hatching conditions on the hatchability (Pseudo-F = 0.757–2.210, p(perm) ≥0.089; ). However, magnetic field exposure affected significantly the hatchability of resting eggs of H. incongruens. The cumulative hatching curve in magnetic field (irrespective of incubation condition) was significantly different (Pseudo-F = 9.409, p(perm) = 0.002; ) from that in control condition (). Hatching of juveniles in the magnetic field was faster, 40% of neonates appeared at 4 days, whereas in control it took two times longer – after 8 days. However, in spite of the significant main effect of hatching conditions, there was no main effect of incubation conditions (Pseudo-F = 0.875, p(perm) = 0.420) and finally, there was also no interaction between incubation and hatching conditions (Pseudo-F = 0.384, p(perm) = 0.726; ), and the final hatching success was also the same in magnetic field and control ().
Figure 1. Cumulative hatching curves for Heterocypris incongruens resting eggs as a function of time in control and in magnetic field hatching conditions. Lines show mean cumulative hatching success of 12 replicates per each of the two treatments of hatching conditions without differentiation between the incubation conditions; whiskers display 1 × SE (standard error)
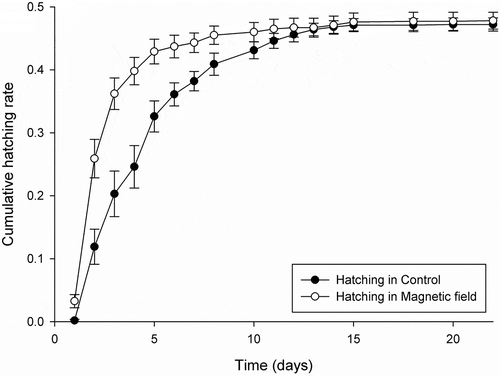
Despite no effect of the incubation condition in magnetic and electric field exposures vs. control on hatchability dynamic and the final hatching success, the incubation conditions in both, the magnetic field and electric field did significantly decrease juvenile survival to the adult stage (in magnetic field Pseudo-F = 8.526, p(perm) = 0.008, and in electric field Pseudo-F = 6.148, p(perm) = 0.029; ). When eggs where incubated in a magnetic field, only 21.7% of juveniles became females, whereas if eggs where incubated in control as much as 51.7% of juveniles reached adulthood. After incubation in electric field juvenile survival to the adult stage was lowered to 16.7% vs. 41.7% in the control condition ().
Discussion
Resting eggs of ostracods inhabiting inland waters are well known to be highly resistant to a range of adverse conditions, e.g. prolonged desiccation, freezing and high temperatures, low atmospheric pressure, UV-B radiation, hypoxia, vertebrate digestive enzymes, high salinity or pesticides and insecticides (Proctor Citation1964; Kornicker & Sohn Citation1971; Sohn & Kornicker Citation1979; Angell & Hancock Citation1989; Martins et al. Citation2008; Van den Broecke et al. Citation2012; Vandekerkhove et al. Citation2013 and reference therein). Our data are the first to demonstrate also the high tolerance of ostracod resting eggs to an intense electric field (14,000 V/m) and a magnetic field (150 µT), mimicking that which is generated by high-voltage overhead transmission lines. We did not detect any effect of the studied field exposures on the hatching success of H. incongruens ().
However, we detected some sensitivity of the hatching dynamics to the magnetic component of the electromagnetic field. In particular, even if there was no significant difference in the final hatching success between the magnetic field and control conditions (), the hatching rate since hydration was accelerated in the magnetic field compared to the control. Comparison of the hatching accumulation curves suggests that eggs exposed to the magnetic field hatched during the first 2 weeks of the experiment faster and more synchronously than control eggs (). Such a hatching strategy may enhance the success of completion of the reproductive cycle, reducing the competition for resources. On the other hand, fast hatching response or a high proportion of ostracod eggs hatching immediately after the first hydration may cause dramatic fitness decrease in case the hydroperiod is too short for completion of the life cycle (Martins et al. Citation2009). In fact, asynchronous hatching (or some kind of bet-hedging strategy) was hypothesized as an advantageous strategy for sexually reproducing populations of an ostracod Eucypris virens (Jurine) compared to their asexual conspecifics, tailoring the hatching response to the unpredictability of the hydroperiod cycle in the Mediterranean area (Martins et al. Citation2008). The mechanism by which hatching dynamic upon inundation was accelerated and synchronized under the magnetic field exposure remains unclear. However, we can speculate that electromagnetic field which is perceived as light (Reiter Citation1993; Fernie & Reynolds Citation2005) may be an additional stimulus (as lengthened photoperiod) to the presence of water, triggering the hastened hatching of resting eggs of ostracods inhabiting temporary inland waters. It is also difficult to explain why the magnetic field had a significant impact on the hatchability dynamics, while we did not detect any effect of the electric field on the hatchability of our test species. It appears, however, that of the two components of the power frequency electromagnetic field, the magnetic component is more important biologically than the electric one. The magnetic field can easier and without attenuation penetrate inside biological tissues than the electric filed which is significantly absorbed by water present in living cells (Kaune Citation1993).
Our study clearly showed that both magnetic field and electric field were also detrimental for ostracod juvenile survival when eggs were incubated dry under the magnetic or electric field exposures (). It is difficult, however, to compare our results with other studies using different experimental parameters (frequency, field strength, magnetic flux density or exposure duration). Nonetheless, it should be emphasized that even considering only the effects of electromagnetic fields of parameters similar to those used in our study (power frequency 50/60 Hz, magnetic flux density of 10–500 (1000) μT and electric field strength of around 10–20 kV/m) on fitness components of invertebrate juvenile or larval stages (hatching dynamic and viability during postembryonic development), the current knowledge is clearly inconclusive.
Similarly to the results found in our study, negative effects upon survival have been associated with the magnetic field exposure for gastropods, crustaceans and insects. Ossenkopp et al. (Citation1990) show that mortality levels in a grove snail Cepaea nemoralis (L.) increase with the duration of exposure to a 60 Hz, 100 μT magnetic field. Krylov (Citation2008) demonstrates that magnetic field with a density of 150 μT and frequencies 17 Hz and 500 Hz exposed during 7 days has the most deleterious consequences on survival and maturation of Daphnia magna, while a 21 days exposure to a frequency of 500 Hz during the juvenile stage decreases body size and viability of the next generation. The same author in other studies on D. magna (Krylov Citation2010) documents decreased number of viable offspring in the first brood of females that develop from exposed eggs (45–175 Hz electromagnetic field), despite no significant differences in mortality in either embryos or adult females of the parental generation between the test specimens and control. Panagopoulos et al. (Citation2013) found that the number of pupae of Drosophila melanogaster Meigen decreases with the strength of the 50 Hz, 100–2100 μT magnetic fields applied for 5 days, although the effects were only statistically significant with high magnetic flux densities of 1.1 and 2.1 mT. Similarly, Ramírez et al. (Citation1983) report increased mortality of eggs, larvae, pupae and adults as well as reduced oviposition by D. melanogaster exposed to 60 Hz, 1000 μT magnetic field for 48 h. Finally, increased mortality has been also documented in strong magnetic field exposures: larvae of a large pig roundworm Ascaris suum (Goeze) (Kuźna-Grygiel et al. Citation2005) and of a common liver fluke Fasciola hepatica L. (Kołodziejczyk et al. Citation2010) both in 2-mT fields as well as larvae of a mill moth Ephestia kuehniella Zeller in 1.4-T field (Pandir et al. Citation2013).
Contrary to our results, no effects of the electromagnetic field exposure on viability have been found for a wrinkled snail Helicella caperata (Montagu) (60 Hz, 100 μT – Child et al. Citation1992), Drosophila melanogaster (60 Hz, 1 mT – Walters & Carstensen Citation1987) and Drosophila subobscura Collin (50 Hz, 500 μT – Zmejkoski et al. Citation2017). For the latter species even increased viability has been revealed under the magnetic field exposure (50 Hz, 500 μT – Dimitrijevic et al. Citation2014). See also above about the results on Daphnia magna by Krylov (Citation2010).
Effects of low-frequency magnetic fields on the development have also been demonstrated for invertebrates and again, both retardation (Zimmerman et al. Citation1990: a purple sea urchin Strongylocentrotus purpuratus (Stimpson) in 60 Hz, 100 µT; Gutzeit Citation2001: D. melanogaster in 50 Hz, 150 µT) and acceleration have been recorded (Krylov Citation2010: Daphnia magna in 45–175 Hz; Dimitrijevic et al. Citation2014; Zmejkoski et al. Citation2017: Drosophila subobscura in 50 Hz, 500 μT). Moreover, the magnetic field (60 Hz, 80 μT) causes significantly lower developmental stability measured by fluctuating asymmetry and a reduction in the weight of D. melanogaster (Graham et al. Citation2000). On the other hand, no significant changes have been found in lifespan, reproduction and hatching rate of a free-living nematode Caenorhabidis elegans (Maupas) exposed to 50 Hz magnetic fields at intensities of 500–3000 μT compared with that of the control (Shi et al. Citation2015).
Excepting differences in laboratory parameters (frequency, exposure duration, magnetic flux density, electric field strength) and/or environmental conditions in field investigations (voltage of power lines, distance from the power lines, etc.), the above inconsistencies between our results and those of other studies as well as between various studies can be attributed to several causes of various natures. First, as laboratory and field experimental data have shown, different species may respond differentially to the exposure of electromagnetic fields (Levin & Ernst Citation1997; Doherty & Grubb Citation1998). In addition, data also suggest that electromagnetic fields might have different effects at various developmental stages and depend on the genetic background (Zmejkoski et al. Citation2017). A further problem is that unlike most biohazard chemical compounds, both components of the electromagnetic field may produce no clear dose-response (non-linear and/or “window”) effects (Doherty & Grubb Citation1998). The experimental results also suggest that the biological effects of electromagnetic fields could be transient or at least partially reversible, synergistic with other factors or depend on some threshold values (Pan Citation1996; Doherty & Grubb Citation1998). We cannot also rule out the possibility that some of the published effects are the results of experimental artifacts, as suggested by Walters and Carstensen (Citation1987). Finally, some effects or no response could be due to the fact that the tested animals have lived for generations under laboratory conditions where they were exposed to the electromagnetic fields and may have adapted to these conditions (Krylov Citation2008).
To conclude, our results show that both components of the power frequency electromagnetic field (50 Hz), equivalent to that created by high voltage overhead power transmission lines, can have important consequences for ostracod reproduction. Further long-term experiments are clearly needed to clarify the results obtained so far and to shed light on the long-term consequences of the environmental pollution by electromagnetic field exposure associated with power transmission lines on fitness-related life history traits of ostracods and other aquatic invertebrates.
Acknowledgements
We are grateful to the Regional Inspectorate for Environmental Protection in Gdansk for the measurement of the electric and magnetic field intensities as well as to the Institute of Experimental Physics, University of Gdansk for help in the designing of the culture containers. We also gratefully acknowledge the help of Adam Green (Gdansk), who improved the English in the manuscript.
Disclosure statement
No potential conflict of interest was reported by the authors.
Additional information
Funding
References
- Anderson MJ, Gorley RN, Clarke KR. 2008. PERMANOVA+ for PRIMER: Guide to software and statistical methods. Plymouth: PRIMER-E.
- Angell RW, Hancock JW. 1989. Response of eggs of Heterocypris incongruens (Ostracoda) to experimental stress. Journal of Crustacean Biology 9:381–386. DOI: 10.2307/1548561.
- Child SZ, Hartman CL, Schery L, Carstensen EL. 1992. A test for increased lethality in land snails exposed to 60 Hz magnetic fields. Neuroscience Letters 134:169–170. DOI: 10.1016/0304-3940(92)90508-5.
- Clarke KR, Gorley RN. 2015. PRIMER v7: User manual/tutorial. Plymouth: PRIMER-E.
- Dimitrijevic D, Savic T, Andelkovic M, Prolic Z, Janac B. 2014. Extremely low frequency magnetic field (50 Hz, 0.5 mT) modifies fitness components and locomotor activity of Drosophila subobscura. International Journal of Radiation Biology 90:337–343. DOI: 10.3109/09553002.2014.888105.
- Directive. 2004. Directive 2004/40/EC of the European Parliament and of the Council on the minimum health and safety requirements regarding the exposure of workers to the risks arising from physical agents (electromagnetic fields). Official Journal of the European Union L 159:1–26.
- Doherty PF, Grubb TC. 1998. Reproductive success of cavity-nesting birds breeding under high-voltage powerlines. The American Midland Naturalist 140:122–128. DOI: 10.1674/0003-0031(1998)140[0122:RSOCNB]2.0.CO;2.
- Fernie KJ, Reynolds SJ. 2005. The effects of electromagnetic fields from power lines on avian reproductive biology and physiology: A review. Journal of Toxicology and Environmental Health, Part B: Critical Reviews 8:127–140. DOI: 10.1080/10937400590909022.
- Goiceanu C, Danulescu R. 2006. Occupational exposure to power frequency fields in some electrical transformation stations in Romania. International Journal of Occupational Safety and Ergonomics 12:149–153. DOI: 10.1080/10803548.2006.11076676.
- Graham JH, Fletcher D, Tigue J, McDonald M. 2000. Growth and developmental stability of Drosophila melanogaster in low frequency magnetic fields. Bioelectromagnetics 21:465–472. DOI: 10.1002/()1521-186X.
- Gutzeit HO. 2001. Biological effects of ELF-EMF enhanced stress response: New insights and new questions. Electro- and Magnetobiology 20:15–26. DOI: 10.1081/JBC-100103157.
- Hanningan J. 2013. Effects of electric and magnetic fields on transmission line design. Electric Energy T & D Magazine 17:33–36.
- ICNIPR. 2010. International commission on non-ionizing radiation protection. Guidelines for limiting exposure to time‐varying electric and magnetic fields (1 HZ-100 kHZ). Health Physics 99:818–836. DOI: 10.1097/HP.0b013e3181f06c86.
- ISO. 2012. International Organization for Standardization: ISO standard 14371:2012 water quality – Determination of fresh water sediment toxicity to Heterocypris incongruens (Crustacea, Ostracoda) – Bibliography. Available: https://www.iso.org/obp/ui/#iso:std:iso:14371:ed-1:v1:en. Accessed Jan 2020 5.
- Kaune WT. 1993. Introduction to power-frequency electric and magnetic fields. Environmental Health Perspectives 101(Suppl. 4):73–81. DOI: 10.1289/ehp.93101s473.
- Kołodziejczyk L, Kuźna-Grygiel W, Gonet B, Podraza W. 2010. Extremely low frequency magnetic field and the hatching rate of Fasciola hepatica eggs, the fecundity and survival of liver fluke-infected snail, Lymnaea truncatula. Folia Biologica (Krakow) 58:157–161. DOI: 10.3409/fb58_3-4.157-161.
- Kornicker LS, Sohn IG. 1971. Viability of ostracode eggs egested by fish and effect of digestive fluids on ostracode shells – Ecologic and paleoecologic implications. Bulletin du Centre de Recherches 5 Suppl:285–293.
- Krylov VV. 2008. Impact of alternating electromagnetic field of ultralow and low frequencies upon survival, development, and production parameters in Daphnia magna Straus (Crustacea, Cladocera). Inland Water Biology 1:134–140. DOI: 10.1134/S1995082908020053.
- Krylov VV. 2010. Effects of electromagnetic fields on parthenogenetic eggs of Daphnia magna Straus. Ecotoxicology and Environmental Safety 73:62–66. DOI: 10.1016/j.ecoenv.2009.03.005.
- Krylov VV, Osipova EA. 2013. The response of Daphnia magna Straus to the long-term action of low-frequency magnetic fields. Ecotoxicology and Environmental Safety 96:213–219. DOI: 10.1016/j.ecoenv.2013.06.012.
- Kuźna-Grygiel W, Gonet B, Jaborowska M, Kołodziejczyk L. 2005. Effect of power network frequency magnetic field on embryonic development of Ascaris suum (Nematoda). Folia Biologica (Krakow) 53:101–105. DOI: 10.3409/1734916054663393.
- Levin M, Ernst SG. 1997. Applied DC magnetic fields cause alterations in the time of cell divisions and developmental abnormalities in early sea urchin embryos. Bioelectromagnetics 18:255–263. DOI: 10.1002/()1521-186X.
- Lewczuk B, Redlarski G, Żak A, Ziółkowska N, Przybylska-Gornowicz B, Krawczuk M. 2014. Influence of electric, magnetic, and electromagnetic fields on the circadian system: Current stage of knowledge. BioMed Research International 69459. DOI: 10.1155/2014/169459.
- Martins MJF, Vandekerkhove J, Mezquita F, Schmit O, Rueda J, Rossetti G, Namiotko T. 2009. Dynamics of sexual and parthenogenetic populations of Eucypris virens (Crustacea: Ostracoda) in three European ponds. Hydrobiologia 636:219–232. DOI: 10.1007/s10750-009-9952-0.
- Martins MJF, Vandekerkhove J, Namiotko T. 2008. Environmental stability and the distribution of the sexes: Insights from life history experiments with the geographic parthenogen Eucypris virens (Crustacea: Ostracoda). Oikos 117:829–836. DOI: 10.1111/oik.2008.117.issue-6.
- Meisch C. 2000. Freshwater Ostracoda of Western and central Europe. Heidelberg-Berlin: Spektrum Akademischer Verlag.
- Microbiotests. 2020. MicroBio Tests Inc.: Toxkits publications. Available: https://www.microbiotests.com/information/publications/. Accessed Jan 2020 5.
- Ossenkopp KP, Kavaliers M, Lipa S. 1990. Increased mortality in land snails (Cepaea nemoralis) exposed to powerline (60-Hz) magnetic fields and effects of the light-dark cycle. Neuroscience Letters 114:89–94. DOI: 10.1016/0304-3940(90)90433-A.
- Pan H. 1996. The effect of a 7 T magnetic field on the egg hatching of Heliothis virescens. Journal of Magnetic Resonance Imaging 14:673–677. DOI: 10.1016/0730-725X(96)00057-4.
- Panagopoulos DJ, Karabarbounis A, Lioliousis C. 2013. ELF alternating magnetic field decreases reproduction by DNA damage induction. Cell Biochemistry and Biophysics 67:703–716. DOI: 10.1007/s12013-013-9560-5.
- Pandir D, Sahingoz R, Ercan FS. 2013. Mediterranean flour moth Ephestia kuehniella eggs and larvae exposed to a static magnetic field and preference by Trichogramma embryophagum. Biocontrol Science and Technology 23:1402–1411. DOI: 10.1080/09583157.2013.835789.
- Proctor VW. 1964. Viability of crustacean eggs recovered from ducks. Ecology 45:656–658. DOI: 10.2307/1936124.
- Ramírez E, Monteagudo JL, García-Gracia M, Delgado JMR. 1983. Oviposition and development of Drosophila modified by magnetic fields. Bioelectromagnetics 4:315–326. DOI: 10.1002/bem.2250040404.
- Recommendation. 1999. Recommendation 1999/519/EC of the European Council on the limitation of exposure of the general public to electromagnetic fields (0 Hz to 300 GHz). Official Journal of the European Union L 159:59–70.
- Redlarski G, Lewczuk B, Żak A, Koncicki A, Krawczuk M, Piechocki J, Jakubiuk K, Tojza P, Jaworski J, Ambroziak D, Skarbek Ł, Gradolewski D. 2015. The influence of electromagnetic pollution on living organisms: Historical trends and forecasting changes. BioMed Research International 234098. DOI: 10.1155/2015/234098.
- Reiter RJ. 1993. Static and extremely low frequency electromagnetic field exposure: Reported effects on the circadian production of melatonin. Journal of Cellular Biochemistry 51:394–403. DOI: 10.1002/jcb.v51.4.
- Shi Z, Yu H, Sun Y, Yang C, Lian H, Cai P. 2015. The energy metabolism in Caenorhabditis elegans under the extremely low-frequency electromagnetic field exposure. Scientific Reports 5:8471. DOI: 10.1038/srep08471.
- Sohn IG, Kornicker LS. 1979. Viability of freeze-dried eggs of the freshwater Heterocypris incongruens. In: Krstić N, editor. Taxonomy, biostratigraphy and distribution of ostracodes: Proceedings of the VII International Symposium on Ostracodes. Belgrade: Serbian Geological Society. pp. 1–3.
- Stam R. 2018. Comparison of international policies on electromagnetic fields (power frequency and radiofrequency fields). Bilthoven: The Netherlands National Institute for Public Health and the Environment.
- U.S. Congress Office of Technology Assessment. 1989. Biological effects of power frequency electric and magnetic fields. Background paper OTA-BP-E-53. Washington DC: U.S. Government Printing Office.
- Van den Broecke L, Martens K, Pieri V, Schön I. 2012. Ostracod valves as efficient UV protection. Journal of Limnology 71:119–124. DOI: 10.4081/jlimnol.2012.e12.
- Vandekerkhove J, Martens K, Rossetti G, Mesquita-Joanes F, Namiotko T. 2013. Extreme tolerance to environmental stress of sexual and parthenogenetic resting eggs of Eucypris virens (Crustacea,Ostracoda). Freshwater Biology 58:237–247. DOI: 10.1111/fwb.2012.58.issue-2.
- Vecchia P. 2007. Exposure of humans to electromagnetic fields. Standards and regulations. Annali dell’Istituto Superiore di Sanità 43:260–267.
- Walters E, Carstensen EL. 1987. Test for the effects of 60-Hz magnetic fields on fecundity and development in Drosophila. Bioelectromagnetics 8:351–354. DOI: 10.1002/()1521-186X.
- World Health Organization. 2007. Extremely low frequency fields. Geneva: World Health Organization Press.
- Zimmerman S, Zimmerman AM, Winters WD, Cameron IL. 1990. Influence of 60-Hz magnetic fields on sea urchin development. Bioelectromagnetics 11:37–45. DOI: 10.1002/()1521-186X.
- Zmejkoski D, Petkovic B, Pavkovic-Lucic S, Prolic Z, Andelkovic M, Savic T. 2017. Different responses of Drosophila subobscura isofemale lines to extremely low frequency magnetic field (50 Hz, 0.5 mT): Fitness components and locomotor activity. International Journal of Radiation Biology 93:544–552. DOI: 10.1080/09553002.2017.1268281.